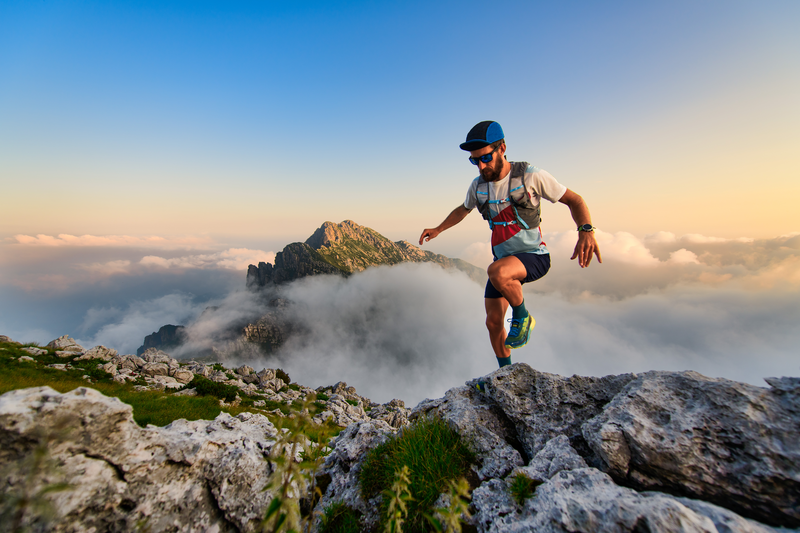
95% of researchers rate our articles as excellent or good
Learn more about the work of our research integrity team to safeguard the quality of each article we publish.
Find out more
ORIGINAL RESEARCH article
Front. Sports Act. Living , 31 March 2025
Sec. Sports Science, Technology and Engineering
Volume 7 - 2025 | https://doi.org/10.3389/fspor.2025.1549763
Background: Rowing is a strength-endurance sport. The Olympic race distance of 2,000-m (2k) requires extensive aerobic and anaerobic energy system contributions, along with sustained high force output.
Aim(s): The primary aim of this study was to evaluate the relationship between rowing ergometer (erg) performance and the force-time curve characteristics of a counter-movement jump (CMJ), and to determine if rowing-related low back pain (LBP) affected these relationships.
Methods: Athletes completed a 2k time trial at the 2023 USRowing Atlantic City Indoor National Championships as well as a CMJ on force plates. Data from the 2k (n = 27) time trial was analyzed to determine significant relationships between CMJ force-time characteristics and 2k rowing ergometer performance. Partial correlations were used to determine the effects rowing-related LBP has on the relationship between 2k erg and CMJ force-time curve characteristics.
Results: Positive Take-off Impulse had the strongest correlation with 2k erg performance (r = 0.71, p < 0.001). Jump height was not significantly related to 2k erg performance (r = −0.13, p = 0.518). Current LBP changed the relationship for Concentric Mean Force (r = 0.74, p < 0.001).
Conclusion: Positive Take-off Impulse has a strong, positive relationship to 2k erg performance. CMJ variables related to impulse and force should be monitored longitudinally to see if changes in these variables coincide with improved 2k rowing erg performance and the assessment of neuromuscular fatigue. Coaches can utilize these findings to individualize strength and power training for potential 2k erg performance improvements.
Rowing has been classified as a strength-endurance sport (1, 2) due to the extensive aerobic and anaerobic demands of racing that have on-water world best times lasting between 5:18.68 and 7:23.36, depending on boat classification (3). The energy system contribution to racing has been determined to be approximately 70%–77% aerobic and 23%–30% anaerobic (4, 5). Ingham et al. had 41 World Rowing Championships finalists perform the following series of tests to identify the physiological determinants of 2k rowing ergometer performance: a 2k ergometer (erg) time trial, a discontinuous incremental test to establish the blood lactate threshold, a seven-stroke test (two building strokes, five maximal) to determine maximal force (Fmax), maximal power (Wmax), and stroke length. WVO2max (power at maximal oxygen consumption) and Wmax were the strongest single correlates to 2k erg performance (6). Further analysis determined that 98% of 2k erg variance could be explained by WVO2max, VO2LT, LSS (oxygen consumption at lactate threshold determined by least sum of squares), Wmax, and W4mmol−1 (power at blood lactate concentration of 4 mmol/L−1) (6). Ingham's study highlighted that rowers require significant development of both aerobic (WVO2max) and anaerobic (Wmax) energy systems for 2k performance. However, all of these tests were conducted on a rowing ergometer. The inclusion of strength and neuromuscular tests that assess and monitor anaerobic characteristics are valuable to the development of rowers to understand the specific anaerobic qualities that may contribute to performance.
Strength has been defined as the ability of the body to produce force to overcome inertia or load (7) whereas maximal force (Fmax) is defined as the maximum amount of force a muscle or muscle group can generate (7, 8). Athletes are often required to exert maximal forces, externally, in several ways: to overcome gravity (e.g., sprinting, high jump), to move a competitor's body mass (e.g., wrestling), to use an instrument (e.g., hockey stick, baseball bat, soccer), and to propel a piece of equipment or an implement (e.g., cycling, rowing, javelin) (9). Therefore, an athletes' Fmax is important to develop for sport performance, as well as the rate of force development (RFD), defined as the greatest magnitude of force an athlete can produce in a time constraint (8, 10). Many endurance sports have a strong correlation between a high RFD and sport performance. For example, running (11) and cycling (12, 13) have utilized the Isometric Mid-Thigh Pull (IMTP) for RFD measurement whereas in on-water rowing it's traditionally been measured at the pin of the oarlock (14, 15). However, one of the practical limitations of the IMTP is that it takes significant time to set-up and execute. This makes the IMTP a challenging test to implement in sports such as rowing that have teams of 40 + athletes (16, 17).
A recent study by Ledergerber et al. investigated the contributions of different strength determinants for different phases (start, middle, end) of 2k erg performance in 14 adolescent rowers (18). Ledergerber found that the RFD of isometric leg press over 150 and 350 milliseconds (ms) was significantly correlated (r = 0.671–0.918, p < 0.05) to the start, middle, end and total 2k race distance (18). A final key finding was that the single greatest predictor for 2k erg performance was attributed to absolute VO2max, maximal isokinetic trunk flexion, and sex, explaining 97.5% of the variance (p < 0.001). This provides evidence that reinforces the importance of developing aerobic metabolism to improve VO2max and Fmax transmission through the legs and trunk.
Strength-endurance athletes, such as rowers, benefit from strength training through improved economy and muscular power (19), contributing to ∼30% of the anaerobic energy supply (4). Reviewing data from Steinacker, for each stroke during on-water competition, elite male rowers produce peak forces of 1,000–1,500 Newtons (N) at the start, 500–700 N during the body of the race, and 600–700 N for the final sprint of a 2k race (20). Analyzing data from each stroke of 47 2k races during two regattas at the Sydney International Rowing Center, Holt et al. found that the mean force across 2k was 261 N in the Men's Single (M1x), 199 N in the Women's Single (W1x), 503 N in the Men's Pair (M2-), and 367 N in the Women's Pair (W2-) (14). Furthermore, the time to peak force from the catch (blade entering the water at the start of each oar stroke) ranged from 0.36 to 0.43 s across these boat classes (14). Taken together, these two studies highlight the importance of Fmax (20) and RFD (14) for on-water rowing performance. To further tie together on-water rowing performance and on-land strength determinants, Ledergerber found that the RFD of the IMTP over 300 ms was significantly correlated (r = 0.769–0.903, p < 0.05) to the start, middle, end and total 2k race distance, but not at 150 ms (r = 0.302, to −0.413, p > 0.05) (18). This shows that regardless of environment (on-water or on-land) rowers have a longer RFD that relates to rowing performance (14, 18).
The countermovement jump (CMJ) is a vertical jump test performed by having an athlete start in a standing upright position, hands on hips, before making a downward movement with a triple flexion of the hips, knees, and ankles, and then aggressively extending the hips, knees, and ankles to jump off the floor as high as possible (21). The CMJ requires a coordinated flexion of the ankles, knees, and hips; followed by a rapid full extension of each joint to accelerate off the floor; then concluding with a landing back on the ground. The CMJ has been used across multiple sports as a proxy measure of muscle force and power (22, 23). Dos' Santos et al. conducted a study investigating IMTP against CMJ, squat jump, and 1 rep maximum power clean in 43 (rowing, soccer, motocross, and hockey) athletes. They found that only force at 250 ms in the IMTP had a significant, yet moderate correlation (Spearman = 0.346, p = 0.016) with CMJ jump height (24). Boullosa et al. investigated the force-time characteristics of different athletic populations and the relationships between them. The study included three groups: endurance (eight runners, six triathletes), 12 sprinters, and 12 fire fighters (controls). The main finding was that the CMJ force-time characteristics were dependent on training background. In terms of absolute values, the sprinters exhibited greater jump height, peak power, normalized vertical stiffness and RFD compared to the endurance and fire fighters (25). However, the strength of the correlations for many of the force-time variables were higher in the endurance group. For example, when examining the relationship between vertical stiffness and the ratio between peak RFD and its time of occurrence they found the endurance group (r = 0.920, p < 0.01) had a significantly stronger relationship compared to the sprinters (r = 0.721, p < 0.01) (25). This indicates that the athletes who could producer higher levels of vertical stiffness at the end of the eccentric phase were able to translate that to earlier and higher RFD values (25). This indicates that different athletic populations not only achieve different levels of absolute force but how they produce this force is distinct to their sporting background.
The CMJ has been extensively used within elite rowing populations for over a decade with key force-time curve characteristics being used to measure both improvements with strength training as well as demonstrate the strong relationship between leg strength and power in 2k erg, a key selection metric for crews (8, 26, 27). Podstawski et al. explored the relationship between CMJ and 2k erg in 200 rowers (female: n = 70, male: n = 130) and found, in both sexes, that 2k erg time was significantly shortened (p < .001) with an increase in peak power (r = −.98 and −.99), relative peak power (r = −.77 and −.76), and Fmax (r = −.59 and −.52) during the CMJ (28). Interestingly, male rowers displayed a significantly shorter 2k erg with an increase in jump height (r = −.36, p < .001) (28). The rowers in this study were 15–22 years old, which limits the ability to generalize findings to other populations. Further research is warranted to establish if a relationship exists between CMJ performance and 2k rowing erg performance within the sport of rowing and between both sexes. Therefore, the purpose of this study was to evaluate the relationship between 2k erg and CMJ, and associated force-time curve characteristics related to force, power, and impulse. A secondary aim was to determine if sex and self-reported rowing-related low back pain (LBP) affected the relationship between 2k erg and CMJ.
The study was completed at the 2023 USRowing Atlantic City Indoor National Championships (2023 USACINC) that were held on February 4th–5th, 2023 in Atlantic City, New Jersey, USA.
A convenience sample of participants who were competing at USACINC were recruited to participate in this study. The inclusion criteria included participants who were ≥18–49 years of age and registered to compete in the 2023 USACINC. Exclusion criteria included 2023 USACINC participants who were <18 years of age, or in the PR3 Down Syndrome (DS) and PR3 Intellectual Impairment (II) competition categories due to concerns around safely landing after performing a CMJ; all other para categories were included. Informed consent was obtained electronically from each participant via an iPad using a secure link from Qualtrics (Seattle, WA). Research approval was obtained from the University of Kansas Institutional Review Board (STUDY00149616).
The 2023 USACINC included events ranging from juniors to masters rowers across multiple distances including max watts testing, 500 m, 2k, four-person team relay across 2k, and triathlon (RowErg, BikeErg, and SkiErg). The data included in our study was participants' publicly available 2k time trial data. All testing was performed on a RowErg (Model D; Concept II, Inc., Morrisville, VT, USA). Per USRowing rules, rowers were allowed to set the drag factor to their desired setting (29).
The research team were initially positioned in an exhibitor's booth by the competition area and then moved to the warm-up area to increase the recruitment of participants. USRowing circulated an email to all indoor rowing competitors the weekend of the event, informing them of the research study, where the study was being conducted, and the testing involved.
After informed consent, participants completed a survey through Qualtrics (Provo, UT). The survey questions included the participant's name, age, competition category at the 2023 USACINC, rowing experience, and history of self-reported rowing-related low back pain. The research team was then able to link participants' survey responses, CMJ tests, and their 2k erg at the 2023 USACINC via the publicly available results on Time-Team Regatta Systems (Amersfoot, Netherlands).
Dual uni-dimensional force plates (VALD ForceDecks, Brisbane, Queensland, Australia) system were used to capture the force-time characteristics of the CMJ. VALD ForceDecks' CMJ metrics of Take Off Peak Force (N), Positive Take-off Impulse (N • s), and Jump Height (Imp-Mom) (cm) have been found to have good to excellent concurrent validity with force plates embedded in the floor (AMTI, MA, United States); only showing relative differences of 0%, 1%, and 5%, respectively (30). This system is comprised of a bilateral, one-dimensional set of force plates, with a sampling frequency of 1,000 Hz. Members of the research team calibrated the force plates according to manufacturer specifications, by zeroing them out, ensuring no external mass was touching them, prior to the participant stepped onto them to complete CMJ testing (31). Participants' body mass was auto calculated by the force plates prior to their first jump. Test data was stored on the VALD Hub, a cloud-based analytics software.
Prior to CMJ testing, participants completed a familiarization of the jump protocol consisting of 3–5 jumps, with coaching instruction from the researchers. Participants completed three CMJs with each CMJ separated by 10–15 s. Participants executed the CMJ as described in the introduction and were encouraged to jump as high and as fast as possible. To increase study participation, rowers in this study had the opportunity to complete the CMJ at their convenience during the 2023 USACINC, including pre or post competition. The six phases of a CMJ are the weighing phase (32), start of movement, eccentric phase, concentric phase, take-off phase, and landing phase (33). The CMJ variables of interest were concentric mean force (N), concentric mean power (W), concentric peak force (N), jump height (impulse-momentum) (cm), peak power (W), countermovement depth (cm), and positive take-off impulse (N • s). Positive take-off impulse as defined here is the same as the vertical jump impulse that is used for the impulse-momentum calculation for jump height. Additionally, the relative temporal variables of concentric mean force/BM (N/kg), concentric mean power/BM (W/kg), and eccentric mean deceleration force (N) were evaluated. The time of the stretch-shortening cycle (TSSC) was calculated by summing the concentric and eccentric durations as described elsewhere (34). Table 1 provides definitions of CMJ phases and variables.
Statistical analyses were performed in Microsoft Excel (Redmond, WA) and Jamovi (Sydney, Australia) (35). Data visualizations were created in R Studio (Version 2024.12.0 + 467) (36) using the following packages: “ggplot2”, “corrplot”, and “cowplot”. Categorical variables were reported as frequencies and percentages. Continuous variables were evaluated descriptively using means and standard deviations or median [interquartile range (IQR)] for non-normally distributed data. All variables of interest were tested for normality using the Shapiro–Wilk test. Values were rounded up to the nearest hundredth. A Bonferroni correction was applied, setting statistical significance to p ≤ 0.007 (37); this was implemented to avoid a potential Type I Error and to account for the seven CMJ variables being analyzed from the same data set (38). The strength of the Pearson r correlation was defined as follows as 0 = zero, ±0.1–0.3 = weak, ±0.4–0.6 = moderate, ±0.7–0.9 = strong, and 1 = perfect (39).
Our analysis focused on the 2k erg time trial. The finish times for each participant (2k erg time) was converted to watts using the Concept2 pace calculator available on their website (40). The conversion of the participant's finish time to watts allowed a direct comparison of the mean power produced over the 2k erg time trial with the power and force-time curve characteristics performed during the CMJ. A correlation matrix was run between 2k watts (2kwatts) and the CMJ variables of interest. The selected CMJ variables were related to force, power, and impulse as these are some of the most common force-time variables used in rowing stroke analysis (14, 41–44). Partial correlations were conducted, controlling for previous and current self-reported rowing-related low back pain. 95% Confidence Intervals (95% CI) are provided for all correlational analyses.
Table 2 provides a breakdown of the characteristics of participants. A total of 30 rowers (male = 27, female = 3) participated in this study; a single male participant competed in two events. Unfortunately, due to the small sample of female participants, we were unable to control for sex in our partial correlation analysis and excluded all female participants from our final data analyses, thus our final total was 27 male rowers. The rowers' competing at the 2023 USACINC had 2k erg times ranging from 6:07.3 to 7:58.2 and their ages were between 18 and 46 years old. The rowers' on-water and indoor rowing experience ranged from 0 to 22 years indicating various training ages specific to rowing and reflective of their 2k erg performances.
Positive Take-off Impulse (N • s), was the only CMJ variable that demonstrated a strong positive relationship, and a statistically significant correlation across 2kwatts (r = 0.71, p < 0.001). The CMJ variables concentric mean force (N; r = 0.63, p < 0.001), concentric mean power (W; r = 0.52, p = 0.006), concentric peak force (N; r = 0.57, p = 0.002), and peak power (W; r = 0.55, p = 0.003) all displayed moderate positive, statistically significant correlations with 2kwatts. This suggests that higher levels of force produced over a long period of time occur during the CMJ and potentially may be important for rowing performance. Jump height (impulse momentum) (cm; r = −0.13, p = 0.518) and countermovement depth (cm; r = −0.28, p = 0.156) had weak, negative relationships to 2kwatts. A heatmap correlation matrix (Figure 1) is provided to visualize the strength of relationships between CMJ variables and 2kwatts. The complete summary of correlations between CMJ variables and 2kwatts, are found in Table 3 and presented in Figure 1.
When controlling for self-reported rowing-related LBP, positive take-off impulse was the only variable that displayed a strong, positive and significant correlation for both previous and current LBP (N • s; r = 0.75, p < 0.001; r = 0.76, p < 0.001, respectively) and 2kwatts. Concentric mean force was the only other variable that showed a strong, positive relationship (N; r = 0.74, p < 0.001) to current rowing-related LBP. This suggests that self-reported rowing-related LBP regardless of status (previous or current) impacts performance over the 2k race distance. The complete summary of the partial correlations between rowing-related LBP for 2kwatts are found in Table 4.
Table 4. Partial Correlations for rowing ergometer performance (watts) after controlling for previous and current rowing-related LBP.
The primary aim of this study was to evaluate the relationship between 2k erg and CMJ performance and its associated force-time curve characteristics related to force, power, and impulse. This is the first study to identify that the CMJ variable of positive take-off impulse was related to 2k erg performance. This is noteworthy because impulse is a direct reflection of an athlete's power, or work, achieved during the propulsive phase of the rowing stroke (42, 45). Force-time curve analysis of the rowing stroke is often used to assess several physical and biomechanical variables. This includes training intensity, timing, and rhythm between rowers in team boats, time to peak force, power, and impulse (14, 46). Impulse, defined as the area under the force-time curve (F x t), provides insight into the propulsive force generation of the rowing athlete (47). International level rowers have been shown to produce a larger impulse than national level rowers (48). Effective strength training focusing on Fmax and power characteristics to support rowing performance may allow endurance athletes to utilize a lower percentage of their Fmax capacity when training at low-intensity and to increase their velocity or power at maximal intensity (19). When evaluating rowing performance, power, defined as force multiplied by velocity (49), becomes an even more important physical quality and highlights the underlying need for high levels of strength and Fmax development. Recent on-water research continues to support that early peak force and RFD translate to faster boat velocities as well as a larger impulse in the force-time curve (14, 15). However, it still needs to be determined if rowers with the greatest impulse during a CMJ also produce the greatest impulse during on-water or ergometer rowing. This is an area that needs to be explored in future research as this can have value purely beyond performance enhancement but also potentially utilized for talent identification in developing rowing athletes.
Second, positive take-off impulse demonstrated the strongest relationship to 2kwatts compared to concentric impulse (N • s; 2kwatts r = 0.57, p = 0.002). This indicates that the sum of the eccentric and concentric impulse phases of the CMJ have a stronger relationship to rowing ergometer performance than concentric impulse alone. This is insightful because the drive (propulsive) phase of the rowing stroke has been considered a concentric dominant movement. However, recent research by Held and colleagues investigating on-water rowing, CMJ, and drop jumps has provided evidence that rowing involves a slow stretch-shortening cycle (SSC) of the vastus lateralis, vastus medialis, and gastrocnemius muscles (34) and that the SSC contributes mainly to high-intensity rowing (50). The SSC involves a pre-activation of the muscle before the eccentric phase, a short and fast eccentric phase, and finally a short delay before the concentric phase (51). The SSC increases the rate and magnitude of the stretch on muscles resulting in a higher force output (51) and has been classified as short (fast) (<0.250 ms) or long (slow) (>0.250 ms) (52). Held et al. found the removal of the eccentric portion of the flexion-extension cycle (FEC) within the rowing stroke, through the use of micro-pausing rowing, significantly reduced the SSC and reduced the onset and amplitude of EMG activity when compared to traditional FEC rowing (50). In a separate study, Held et al. found that 10 elite male rowers' time of the SSC (TSSC) was 731 ± 217 ms during the CMJ (34). The male rowers in our study displayed a mean TSSC of 1,049 ± 215.4 ms. Taken together, these findings suggest additional evidence of a slow SSC occurring during the CMJ in this cohort of rowing athletes. The differences in our TSSC may be attributed to the comparison of elite rowers who were all World Championship medalists against university, masters, and recreational rowers (34). Additionally, all of the elite rowers in Held's study had an average age of 22.8 ± 3.1 whereas our cohorts were not normally distributed (20.0 ± 4.5) including six participants over the age of 25 (ages 27–46). Future research should investigate long SSC exercises within the strength and conditioning program to determine if this outcome-based approach results in an improvement in rowing performance. For example, potential exercises may include the squat jump (27) or a weighted countermovement jump and/or modified plyometric exercises that focus on long and not short SSC. Figure 2 shows a comparison of CMJ and rowing force-time curves.
There are several noteworthy takeaways when evaluating our findings compared to research currently available in the literature. First, we found that jump height did not show a relationship to 2k erg performance which contradicts the findings of Podstawski et al. (28). This may be due to the fact that our jump height variable was calculated based on the impulse-momentum relationship which has been found to be the most reliable method (53), whereas Podstawski et al. directly calculated jump height from ground reaction forces without explicitly integrating force over time to determine take off velocity (28), which may account for the differences in results.
Additionally, the majority of Podstawski's cohort were junior male and female rowers at ages 15–16 (36 female, 55 male), 17–18 (26 female, 52 male), and 18+ (8 female, 23 male) which may affect the interpretation of results (28). In a separate study by Alfoldi, junior male rowers showed a lower jump height, peak power (W), and relative peak power (RPP W/kg) compared to their older peers (54). This indicates that simply the differences in chronological and training age may contribute to the differences in results between our studies. However, what is significantly different is our results for RPP compared to Alfoldi and colleagues. In our study, we found RPP to be 44.93 ± 5.3 W/kg compared to 3.76 W/kg (age 15–16), 4.42 W/kg (ages 17–18), and 4.59 W/kg (ages 19–22) (54).
Metikos et al. sought to determine the relationship between relative peak power (W/kg) from a CMJ and peak output during a 6-stroke peak power test on a Concept II rowing ergometer instrumented with a strain gauge, at three different drag factors: 90, 125, and 200 (55). A subset of their rowers (male = 15, female = 9) showed correlations of 0.76, 0.76, and 0.78 for each resistance level, respectively (55). This is additional evidence of the relationship between the CMJ force-time characteristics and rowing ergometer power output at a shorter time/distance. The 6-stroke peak power test would primarily be utilizing the phosphagen creatine energy system, whereas our study showed strong relationships in the CMJ between the aerobic (2k) energy system.
Rowers with previous and current rowing-related LBP showed a nearly identical strength of relationship to 2kwatts (r = 0.75, p < 0.001 vs. r = 0.76, p < 0.001). Interestingly, concentric mean force (N) had a change in the strength of relationship with previous LBP having a moderate positive relationship (r = 0.66, p < 0.001), while current LBP had a strong, positive relationship (r = 0.74, p < 0.001). This indicates that rowers who currently had LBP may have their rowing performance more negatively impacted than those with previous LBP. In fact, recent research by Martinez-Valdez et al. has shown that rowers who recently had rowing-related LBP exhibited altered muscle activation in the erector spinae during incremental rowing in a 7 × 4′ graded exercise test (56). Both the magnitude and distribution of erector spinae muscle activity was higher in rowers with rowing-related LBP. This alteration in muscle recruitment, firing, and activity may alter other motor patterns such as the rate, timing and muscle contraction in the CMJ. Thus, future research should be conducted to determine how it may impact training and athletic movements beyond rowing and jumping.
The CMJ has successfully been used to monitor neuromuscular fatigue in individuals (57, 58), team sport athletes (59, 60), and the military (61, 62), future research should investigate if changes in the force-time curve for both the CMJ and rowing stroke (on-water or rowing ergometer) can be used to determine acute and chronic neuromuscular fatigue, as well as to monitor rowers' performance across training cycles. Recent research by Everett et al. evaluated a loaded countermovement jump in 20 elite male rowers within and between pre-competition and competition mesocycles (63). The rowers were categorized into two groups: ones that had attained benchmarks of >1.7× bodyweight back squat, >1.1× bodyweight power clean, and >1.3× bodyweight bench press and those that did not. Rowers who did not achieve these strength standards had a 3.2% decrease in mean power in the loaded countermovement jump vs. 2.3% in the group that achieved those benchmarks (63). However, the loaded countermovement jumps were only completed in the first and last week of each mesocycle. Therefore, future research should aim to monitor changes in the CMJ on a weekly basis to identify more subtle changes in neuromuscular status. Additionally, the CMJ variables we identified in our study that had the strongest relationship to 2kerg performance, positive take-off impulse, concentric mean force, and peak power should be monitored to determine if they are more sensitive to these changes in neuromuscular status.
Finally, the relationship between 2k performance and body mass is well established. As body mass increases rowing performance improves (6, 64). This is supported in our study as well where we found that body mass was positively, moderately (r = 0.65, p < 0.001) to 2k erg performance. Furthermore, rowers who have higher levels of fat free mass (65) and muscle mass (66) have better 2k erg performance whereas increased body fat percentage negatively impacts 2k erg performance (6). However, when we look at the relative temporal CMJ variables in our study we found weak and non-significant relationships. For example, concentric mean force/BM (N/kg) (r = −0.0094, p = 0.963) and concentric mean power/BM (W/kg) (r = −0.05, p = 0.808) had a nearly zero relationship to 2k erg performance. This is a critical insight because this indicates absolute values of force, power, and impulse are potentially more important than relative values. Second, when we look at eccentric mean deceleration force (N) (r = 0.69, p < 0.001) this had a positive, nearly strong relationship to 2k erg performance. This indicates that a rowers' ability to turn around and apply concentric force is highly related to 2k erg performance—which is exactly what a rower needs to execute during every rowing stroke as they complete the recovery and begin the drive phase of the rowing stroke. Therefore, rowers who can control their body mass and change directions while applying high levels of force is an important characteristic in the CMJ and may be a distinguishing performance factor in rowing performance.
The participants in this study had the opportunity to complete the CMJ at their convenience during the 2023 USACINC. Unfortunately, this did not allow the research team to control for timing of the participants' CMJ repetitions in relation to their indoor rowing competition, potentially influencing their performance through prior exposure to high-intensity exercise. As a result, some participants' CMJ performance may not be reflective of their true capabilities, especially if they performed the test in the first hour after their indoor rowing event. Additionally, familiarization of the testing with participants was limited. Finally, due to a small sample of female rowers their data was excluded. Future research should focus on exploring CMJ force-time characteristics in female rowers to see if these same relationships are present. Furthermore, when investigating neuromuscular fatigue CMJ testing should include baselines testing as well as testing during periods of high volume and/or intensity, and following this period to more clearly identify any potential changes (67).
This study is the first to identify key CMJ variables with the strongest relationship to 2k erg performance. The findings highlight the importance of impulse in both the CMJ and rowing stroke. Future research should examine if these CMJ variables can be used as specific measurements of neuromuscular status in rowing athletes and to determine if this could be used as a way of talent identification. Furthermore, strength and conditioning coaches and rowers can use this information to help individualize training programs and exercise selection to improve neuromuscular function as a contributing factor to anaerobic performance, including the targeting of long SSC movements of the lower body. Finally, these relationships became stronger when controlling for previous or current rowing-related LBP.
The raw data supporting the conclusions of this article will be made available by the authors, without undue reservation.
The studies involving humans were approved by University of Kansas Institutional Review Board (STUDY00149616). The studies were conducted in accordance with the local legislation and institutional requirements. As we have outlined in the methods section, informed consent was provided electronically through digital signature.
JD: Conceptualization, Data curation, Formal Analysis, Investigation, Methodology, Project administration, Visualization, Writing – original draft, Writing – review & editing. AW: Conceptualization, Data curation, Formal Analysis, Investigation, Methodology, Project administration, Writing – original draft, Writing – review & editing. NP: Data curation, Investigation, Writing – review & editing. KA: Methodology, Supervision, Writing – review & editing. AF: Formal Analysis, Methodology, Supervision, Writing – review & editing.
The author(s) declare that financial support was received for the research and/or publication of this article. This study was conducted with the approval of the IRB at the University of Kansas Lawrence (STUDY00149616).
The authors would like to thank the Joe and Clara Tsai Foundation for their support and funding of this study as part of the Wu Tsai Human Performance Alliance. We would also like to thank VALD for providing the force plates for this data collection, Dan Garbutt for facilitating the study, and Daniel Cohen for his assistance regarding the discussion of temporal variables. We thank community partner USRowing for their cooperation with this study during their event.
JD owns and operates Science of Rowing, LLC, a digital publication that publishes reviews of rowing research. AW is a consultant for Science of Rowing, LLC and owner of Strength & Conditioning Academy Ltd.
The remaining authors declare that the research was conducted in the absence of any commercial or financial relationships that could be construed as a potential conflict of interest.
The author(s) declare that no Generative AI was used in the creation of this manuscript.
All claims expressed in this article are solely those of the authors and do not necessarily represent those of their affiliated organizations, or those of the publisher, the editors and the reviewers. Any product that may be evaluated in this article, or claim that may be made by its manufacturer, is not guaranteed or endorsed by the publisher.
1. Nugent FJ, Flanagan EP, Wilson F, Warrington GD. Strength and conditioning for competitive rowers. Strength Cond J. (2020) 42(3):6–21. doi: 10.1519/ssc.0000000000000531
2. Maestu J, Jurimae J, Jurimae T. Monitoring of performance and training in rowing. Sports Med. (2005) 35(7):597–617. doi: 10.2165/00007256-200535070-00005
3. Rowing W. World Best Times. (2024). Available at: https://worldrowing.com/athletes/best-times/ (Accessed December 03, 2024).
4. Hagerman FC. Applied physiology of rowing. Sports Med. (1984) 1(4):303–26. doi: 10.2165/00007256-198401040-00005
5. Martin SA, Tomescu V. Energy systems efficiency influences the results of 2,000 m race simulation among elite rowers. Clujul Med. (2017) 90(1):60. doi: 10.15386/cjmed-675
6. Ingham SA, Whyte GP, Jones K, Nevill AM. Determinants of 2,000 m rowing ergometer performance in elite rowers. Eur J Appl Physiol. (2002) 88(3):243–6. doi: 10.1007/s00421-002-0699-9
9. Suchomel TJ, Nimphius S, Stone MH. The importance of muscular strength in athletic performance. Sports Med. (2016) 46(10):1419–49. doi: 10.1007/s40279-016-0486-0
10. Cormie P, McGuigan MR, Newton RU. Developing maximal neuromuscular power: part 2—training considerations for improving maximal power production. Sports Med. (2011) 41(2):125–46. doi: 10.2165/11538500-000000000-00000
11. Gillen ZM, Wyatt FB, Winchester JB, Smith DA, Ghetia V. The relationship between aerobic and anaerobic performance in recreational runners. Int J Exerc Sci. (2016) 9(5):625–34. doi: 10.70252/KFOP9214
12. Connolly S, Peeling P, Binnie MJ, Goods PSR, Latella C, Taylor JL, et al. Sprint cycling rate of torque development associates with strength measurement in trained cyclists. Eur J Appl Physiol. (2023) 123(6):1215–27. doi: 10.1007/s00421-023-05143-1
13. Stone MH, Sands WA, Carlock J, Callan S, Dickie D, Daigle K, et al. The importance of isometric maximum strength and peak rate-of-force development in sprint cycling. J Strength Cond Res. (2004) 18(4):878–84. doi: 10.1519/14874.1
14. Holt AC, Aughey RJ, Ball K, Hopkins WG, Siegel R. Technical determinants of on-water rowing performance. Front Sports Act Living. (2020) 2:589013. doi: 10.3389/fspor.2020.589013
15. Warmenhoven J, Cobley S, Draper C, Harrison AJ, Bargary N, Smith R. Assessment of propulsive pin force and oar angle time-series using functional data analysis in on-water rowing. Scand J Med Sci Sports. (2017) 27(12):1688–96. doi: 10.1111/sms.12871
16. USRowing. USRowing Announces Olympic Games Paris 2024. Roster (2024). Available at: https://usrowing.org/news/usrowing-announces-olympic-games-paris-2024-roster (Accessed February 11, 2025).
17. Rowing B. Team GB Rowing Squad Selected for Paris 2024. (2024). Available at: https://www.britishrowing.org/2024/06/team-gb-rowing-squad-selected-for-paris-2024/ (Accessed February 11, 2025).
18. Ledergerber R, Jacobs MW, Roth R, Schumann M. Contribution of different strength determinants on distinct phases of Olympic rowing performance in adolescent athletes. Eur J Sport Sci. (2023) 23(12):2311–20. doi: 10.1080/17461391.2023.2230937
19. Beattie K, Kenny IC, Lyons M, Carson BP. The effect of strength training on performance in endurance athletes. Sports Med. (2014) 44(6):845–65. doi: 10.1007/s40279-014-0157-y
20. Steinacker JM. Physiological aspects of training in rowing. Int J Sports Med. (1993) 14(Suppl 1):S3–10.8262704
21. Bobbert MF, Gerritsen KG, Litjens MC, Van Soest AJ. Why is countermovement jump height greater than squat jump height? Med Sci Sports Exerc. (1996) 28(11):1402–12. doi: 10.1097/00005768-199611000-00009
22. Hoffman JR, Tenenbaum G, Maresh CM, Kraemer WJ. Relationship between athletic performance tests and playing time in elite college basketball players. J Strength Cond Res. (1996) 10(2):67–71.
23. Nuzzo JL, McBride JM, Cormie P, McCaulley GO. Relationship between countermovement jump performance and multijoint isometric and dynamic tests of strength. J Strength Cond Res. (2008) 22(3):699–707. doi: 10.1519/JSC.0b013e31816d5eda
24. Dos’Santos T, Thomas C, Comfort P, McMahon JJ, Jones PA. Relationships between isometric force-time characteristics and dynamic performance. Sports. (2017) 5(3):68. doi: 10.3390/sports5030068
25. Boullosa D, Abreu L, de Conceição FA, Cordero Rodríguez Y, Jimenez-Reyes P. The influence of training background on different rate of force development calculations during countermovement jump. Kinesiology. (2018) 50(1):90–5.
26. Thompson P, Wolf A. Training for the Complete Rower: A Guide to Improving Performance. Marlborough: Crowood (2016).
27. Rawlley-Singh I, Wolf A. A philosophical approach to aligning strength and conditioning support to a coaches’ performance model: a case study from a national rowing performance programme. Int J Sports Sci Coach. (2023) 18(1):278–91. doi: 10.1177/17479541221105454
28. Podstawski R, Boryslawski K, Alfoldi Z, Ferenc I, Wasik J. The effect of confounding variables on the relationship between anthropometric and physiological features in 2000-m rowing ergometer performance. Front Physiol. (2023) 14:1195641. doi: 10.3389/fphys.2023.1195641
29. USRowing. Rules of Rowing. (2024). Available at: https://usrowing.org/resources/rules-of-rowing (Accessed January 31, 2025).
30. Collings TJ, Lima YL, Dutaillis B, Bourne MN. Concurrent validity and test–retest reliability of VALD ForceDecks’ strength, balance, and movement assessment tests. J Sci Med Sport. (2024) 27(8):572–80. doi: 10.1016/j.jsams.2024.04.014
31. VALD. ForceDecks Test: Countermovement Jump Protocol. (2025). Available at: https://support.vald.com/hc/en-au/articles/4999886017817-ForceDecks-Test-Countermovement-Jump-Protocol (Accessed January 31, 2025).
32. McMahon JJ, Suchomel TJ, Lake JP, Comfort P. Understanding the key phases of the countermovement jump force-time curve. Strength Cond J. (2018) 40(4):96–106. doi: 10.1519/ssc.0000000000000375
33. Performance V. VALD ForceDecks Technical Glossary. (2024). Available at: https://support.vald.com/hc/en-au/articles/31552969607321-ForceDecks-Technical-Metric-Glossary (Accessed December 03, 2024).
34. Held S, Siebert T, Donath L. Electromyographic activity of the vastus medialis and gastrocnemius implicates a slow stretch-shortening cycle during rowing in the field. Sci Rep. (2020) 10(1):9451. doi: 10.1038/s41598-020-66124-4
35. Jamovi. The Jamovi Project 2022. Jamovi (2022). Jamovi (Version 2.3) [Computer Software]. Available at: https://www.jamovi.org (Accessed July 27, 2023).
36. Team RC. R: A Language and Environment for Statistical Computing. Vienna, Austria: R Foundation for Statistical Computing (2024). Available at: https://www.R-project.org/
37. Curtin F, Schulz P. Multiple correlations and Bonferroni’s correction. Biol Psychiatry. (1998) 44(8):775–7. doi: 10.1016/S0006-3223(98)00043-2
38. Armstrong RA. When to use the Bonferroni correction. Ophthalmic Physiol Opt. (2014) 34(5):502–8. doi: 10.1111/opo.12131
39. Akoglu H. User’s guide to correlation coefficients. Turk J Emerg Med. (2018) 18(3):91–3. doi: 10.1016/j.tjem.2018.08.001
40. Concept2. Pace Calculator. Concep2 (2024). Available at: https://www.concept2.com/indoor-rowers/training/calculators/pace-calculator (Accessed July 27, 2023).
41. Legge N, Draper C, Slattery K, O’Meara D, Watsford M. On-water rowing biomechanical assessment: a systematic scoping review. Sports Med Open. (2024) 10(1):101. doi: 10.1186/s40798-024-00760-2
42. Warmenhoven J, Cobley S, Draper C, Smith R. Over 50 years of researching force profiles in rowing: what do we know? Sports Med. (2018) 48(12):2703–14. doi: 10.1007/s40279-018-0992-3
43. Hume P. Movement analysis of scull and oar rowing. Handbook Hum Motion. (2018) 10:978–3. doi: 10.1007/978-3-319-14418-4_133
44. Soper C, Hume PA. Towards an ideal rowing technique for performance. Sports Med. (2004) 34(12):825–48. doi: 10.2165/00007256-200434120-00003
46. Draper C. Optimising Rowing Performance in Women’s Single Sculling. Lidcombe: University of Sydney (2005).
47. Hill H. Dynamics of coordination within elite rowing crews: evidence from force pattern analysis. J Sports Sci. (2002) 20(2):101–17. doi: 10.1080/026404102317200819
48. Warmenhoven J, Cobley S, Draper C, Harrison A, Bargary N, Smith R. Bivariate functional principal components analysis: considerations for use with multivariate movement signatures in sports biomechanics. Sports Biomech. (2019) 18(1):10–27. doi: 10.1080/14763141.2017.1384050
49. Komi PV. Units of measurement and terminology. In: Komi PV, editor. Strength and Power in Sport. 2nd ed. Oxford: Wiley Blackwell (2003). p. 13–4.
50. Held S, Raiteri B, Rappelt L, Hahn D, Donath L. Ultrasound and surface electromyography analyses reveal an intensity dependent active stretch-shortening cycle of the vastus lateralis muscle during ergometer rowing. Eur J Sport Sci. (2023) 23(9):1940–9. doi: 10.1080/17461391.2022.2119434
51. Komi P, Nicol C. Stretch-shortening cycle of muscle function. In: Zatsiorsky V, editor. The Encyclopaedia of Sports Medicine: Biomechanics in Sport. Oxford: Blackwell Sciences Ltd. (2000). p. 87–102. doi: 10.1002/9780470693797
52. Schmidtbleicher D. Training for power events. In: Komi PV, editor. Strength and Power in Sport. Oxford: Blackwell Scientific Publications (1992) 381–95.
53. Xu J, Turner A, Comfort P, Harry JR, McMahon JJ, Chavda S, et al. A systematic review of the different calculation methods for measuring jump height during the countermovement and drop jump tests. Sports Med. (2023) 53(5):1055–72. doi: 10.1007/s40279-023-01828-x
54. Alfőldi Z, Borysławski K, Ihasz F, Soós I, Podstawski R. Differences in the anthropometric and physiological profiles of Hungarian male rowers of Various age categories, rankings and career lengths: selection problems. Front Physiol. (2021) 12. doi: 10.3389/fphys.2021.747781
55. Metikos B, Mikulic P, Sarabon N, Markovic G. Peak power output test on a rowing ergometer: a methodological study. J Strength Cond Res. (2015) 29(10):2919–25. doi: 10.1519/jsc.0000000000000944
56. Martinez-Valdes E, Wilson F, Fleming N, McDonnell S-J, Horgan A, Falla D. Rowers with a recent history of low back pain engage different regions of the lumbar erector spinae during rowing. J Sci Med Sport. (2019) 22(11):1206–12. doi: 10.1016/j.jsams.2019.07.007
57. Balsalobre-Fernandez C, Tejero-Gonzalez CM, del Campo-Vecino J. Relationships between training load, salivary cortisol responses and performance during season training in middle and long distance runners. PLoS One. (2014) 9(8):e106066. doi: 10.1371/journal.pone.0106066
58. Balsalobre-Fernandez C, Tejero-Gonzalez CM, del Campo-Vecino J. Hormonal and neuromuscular responses to high-level middle- and long-distance competition. Int J Sports Physiol Perform. (2014) 9(5):839–44. doi: 10.1123/ijspp.2013-0539
59. Gathercole RJ, Sporer BC, Stellingwerff T, Sleivert GG. Comparison of the capacity of different jump and sprint field tests to detect neuromuscular fatigue. J Strength Cond Res. (2015) 29(9):2522–31. doi: 10.1519/jsc.0000000000000912
60. Philipp NM, Nijem RM, Cabarkapa D, Hollwedel CM, Fry AC. Investigating the stretch-shortening cycle fatigue response to a high-intensity stressful phase of training in collegiate men’s basketball. Front Sports Act Living. (2024) 6:1377528. doi: 10.3389/fspor.2024.1377528
61. Welsh TT, Alemany JA, Montain SJ, Frykman PN, Tuckow AP, Young AJ, et al. Effects of intensified military field training on jumping performance. Int J Sports Med. (2008) 29(01):45–52. doi: 10.1055/s-2007-964970
62. Fortes MB, Diment BC, Greeves JP, Casey A, Izard R, Walsh NP. Effects of a daily mixed nutritional supplement on physical performance, body composition, and circulating anabolic hormones during 8 weeks of arduous military training. Applied physiology. Nutr Metab. (2011) 36(6):967–75. doi: 10.1139/h11-124
63. Everett KL, Chapman DW, Mitchell JA, Ball N. Changes in loaded countermovement jumps during precompetition and competition training mesocycles in elite rowers. J Strength Cond Res. (2022):2622–7. doi: 10.1519/JSC.0000000000003843
64. Fiskerstrand Å, Seiler K. Training and performance characteristics among Norwegian international rowers 1970–2001. Scand J Med Sci Sports. (2004) 14(5):303–10. doi: 10.1046/j.1600-0838.2003.370.x
65. Yoshiga CC, Higuchi M. Rowing performance of female and male rowers. Scand J Med Sci Sports. (2003) 13(5):317–21. doi: 10.1034/j.1600-0838.2003.00321.x
66. Penichet-Tomas A, Pueo B, Selles-Perez S, Jimenez-Olmedo JM. Analysis of anthropometric and body composition profile in male and female traditional rowers. Int J Environ Res Public Health. (2021) 18(15):7826. doi: 10.3390/ijerph18157826
Keywords: countermovement jump (CMJ), rowing, force-time characteristics, impulse, 2,000-m
Citation: DeLeo JM, Wolf A, Philipp NM, Ackerman KE and Fry AC (2025) The relationship between countermovement jump force-time characteristics and 2,000-m rowing ergometer performance. Front. Sports Act. Living 7:1549763. doi: 10.3389/fspor.2025.1549763
Received: 21 December 2024; Accepted: 10 March 2025;
Published: 31 March 2025.
Edited by:
Hossein Rouhani, University of Alberta, CanadaReviewed by:
Alfonso Penichet-Tomas, University of Alicante, SpainCopyright: © 2025 DeLeo, Wolf, Philipp, Ackerman and Fry. This is an open-access article distributed under the terms of the Creative Commons Attribution License (CC BY). The use, distribution or reproduction in other forums is permitted, provided the original author(s) and the copyright owner(s) are credited and that the original publication in this journal is cited, in accordance with accepted academic practice. No use, distribution or reproduction is permitted which does not comply with these terms.
*Correspondence: Joseph M. DeLeo, am9zZXBoLmRlbGVvQGt1LmVkdQ==
†ORCID:
Joseph M. DeLeo
orcid.org/0000-0002-5502-6131
Alex Wolf
orcid.org/0000-0003-0228-2841
Nicolas M. Philipp
orcid.org/0000-0002-7413-0700
Kathryn E. Ackerman
orcid.org/0000-0003-2626-7785
Andrew C. Fry
orcid.org/0000-0001-8171-7684
Disclaimer: All claims expressed in this article are solely those of the authors and do not necessarily represent those of their affiliated organizations, or those of the publisher, the editors and the reviewers. Any product that may be evaluated in this article or claim that may be made by its manufacturer is not guaranteed or endorsed by the publisher.
Research integrity at Frontiers
Learn more about the work of our research integrity team to safeguard the quality of each article we publish.