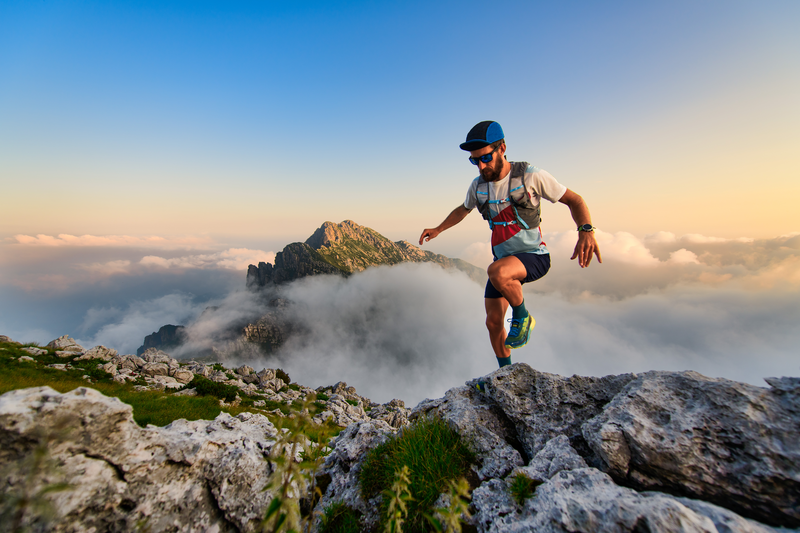
95% of researchers rate our articles as excellent or good
Learn more about the work of our research integrity team to safeguard the quality of each article we publish.
Find out more
ORIGINAL RESEARCH article
Front. Sports Act. Living , 01 April 2025
Sec. Biomechanics and Control of Human Movement
Volume 7 - 2025 | https://doi.org/10.3389/fspor.2025.1524489
This article is part of the Research Topic Human movement coordination in healthy and pathological conditions: From neuromuscular and kinetic principles to muscle-tendon function View all 11 articles
Background: Trunk posture and lumbo-pelvic coordination can influence spinal loading and are commonly used as clinical measures in the diagnosis and management of low-back pain and injury risk. However, sex and pain specific characteristics have rarely been investigated in a large cohort of both healthy individuals and low-back pain patients. It has also been suggested that the motor control of trunk stability and trunk movement variability is altered in individuals with low-back pain, with possible implications for pain progression. Nonetheless, clear links to low-back pain are currently lacking.
Objective: To investigate trunk posture, lumbo-pelvic coordination, trunk dynamic stability and trunk movement variability in an adequately large cohort of individuals with low-back pain and asymptomatic controls and to explore specific effects of sex, pain intensity and pain chronicity.
Methods: We measured lumbo-pelvic kinematics during trunk flexion and trunk dynamic stability and movement variability during a cyclic pointing task in 306 adults (156 females) aged between 18 and 64 years, reporting either no low-back pain or pain in the lumbar area of the trunk. Participants were grouped based on their characteristic pain intensity as asymptomatic (ASY, N = 53), low to medium pain (LMP, N = 185) or medium to high pain (MHP, N = 68). Participants with low-back pain that persisted for 12 weeks or longer were categorized as chronic (N = 104). Data were analyzed using linear mixed models in the style of a two way anova.
Results: Female participants showed a higher range of motion in both the trunk and pelvis during trunk flexion, as well as an increased lumbar lordosis in standing attributed to a higher pelvic angle that persisted throughout the entire trunk flexion movement, resulting in a longer duration of lumbar lordosis. The intensity and chronicity of the pain had a negligible effect on trunk posture and the lumbo-pelvic coordination. Pain chronicity had an effect on trunk dynamic stability (i.e., increased trunk instability), while no effects of sex and pain intensity were detected in trunk dynamic stability and movement variability.
Conclusions: Low-back pain intensity and chronicity was not associated with lumbo-pelvic posture and kinematics, indicating that lumbo-pelvic posture and kinematics during a trunk flexion movement have limited practicality in the clinical diagnosis and management of low-back pain. On the other hand, the increased local instability of the trunk during the cyclic coordination task studied indicates control errors in the regulation of trunk movement in participants with chronic low-back pain and could be considered a useful diagnostic tool in chronic low-back pain.
Low-back pain (LBP) is a leading cause of years lived with disability for 568 million people world wide (1) and is the leading health condition contributing to the need for rehabilitation services globally (2), with immense health care costs and loss of productivity regardless of the treatment indicating the need for nuanced interventions (3). Range of motion (RoM), posture and alignment of the spine during trunk flexion have often been used as key components in the clinical diagnosis of people with low-back pain (4, 5). Trunk flexion in the sagittal plane is a frequently performed movement especially in work-related activities (6) and earlier studies have associated trunk flexion movements with an increased load on the spine by measuring intradiscal pressures in vivo (7–9) and using musculoskeletal models (10–12). Trunk flexion involves both lumbar and pelvic tilt, and quantifying lumbo-pelvic coordination has potential applications in load and injury-risk assessment (13–16) and low-back pain therapy (17, 18). Although the lumbo-pelvic coordination during trunk flexion and extension movements has been extensively studied (19–22), determinants such as sex- or pain-specific characteristics have rarely been examined. Some studies (19) found no differences in lumbo-pelvic coordination between female and male participants with and without low-back pain, whereas others (23) reported sex-specific differences in lumbo-pelvic coordination in asymptomatic participants. A recent systematic review (24) found an effect of sex on lumbar lordosis and lumbar RoM, but noted that the studies available for synthesis were limited and more evidence was needed. Considering the importance of lumbar lordosis in spinal loading (11, 16, 25) and its possible association with low-back pain and spondylolysis (26, 27) an assessment of lumbo-pelvic kinematics in a large number of healthy participants and patients with low-back pain may be of clinical relevance.
It is generally accepted that the risk of developing low-back pain is multifactorial (28–31) and that besides psychosocial (32–34) and personal factors (35–37), a deterioration of the motor control of spinal stability may be related to the onset and progression of low-back pain (13, 38–40). Non-linear analyses can be used to characterize the motor control of trunk stability during repetitive dynamic trunk movements (41). The local dynamic stability of the trunk can be assessed from kinematic data using the maximum finite-time Lyapunov exponent (41). Positive values of this exponent show a divergence of the nearest neighbors in state space over time, while larger values reveal a faster divergence (42), thus indicating a greater effect of small perturbations in trunk kinematics. While there are indications of an association between pathological conditions and trunk movement variability (43, 44) it should be noted that movement variability may also be of functional relevance and indicate a skilled repertoire of a healthy motor system (45) that is needed to cope with perturbations (46). Investigations that incorporate the temporal structure of the movement, such as non-linear time series analyses, can provide insight into the motor control of a systems stability (39, 47) and help to understand the effect of low-back pain on movement (48). However, the effect of low-back pain on trunk dynamic stability and trunk movement variability is currently not well understood (49). Previous studies found no clear effect of low-back pain on trunk dynamic stability (50) and movement variability (51). Current reviews find insufficient evidence (51) and report inconsistent results (52) probably due to differences in methods, task demands and inclusion criteria. It has been suggested that subgroups according to pain characteristics (52, 53), analyses of possible confounders and a large number of participants included (51) may help to detect low-back specific adjustments in spinal motion, thereby increasing the clinical utility of certain measurement variables.
The purpose of the current study was to investigate trunk posture, lumbo-pelvic coordination, trunk dynamic stability and trunk movement variability in an adequately large cohort of participants with low-back pain and asymptomatic controls and to explore specific effects of sex, pain intensity and pain chronicity. Based on literature reports of sex-specific characteristics in lumbar lordosis, sacrum orientation and lumbo-pelvic rhythm (23, 54) we hypothesized that females would have higher lumbar lordosis, higher pelvic RoM and lower lumbo-pelvic ratio than males. Furthermore, based on the reported inconsistent findings of low-back pain on spinal posture and movement (55), we hypothesized that pain intensity would not affect the investigated variables, but that chronic low-back pain would, due to possible control errors in the regulation of trunk movement.
To determine an appropriate sample size for six groups (i.e., three levels of pain intensity, two levels of sex), we conducted an a priori power analysis (G*Power 3.1.9.7) using the outcomes in measures of lumbo-pelvic coordination from an earlier study by our group (56), where we observed medium effect sizes from 0.5 to 0.7 (Cohen's d) between male and female participants. Assuming a more conservative medium effect size of f = 0.20, an alpha error of 0.05 and a statistical power of 0.8 for a balanced group design, a total of 244 participants should suffice to detect specific differences between pain groups or interaction effects (df = 2) in measures of lumbo-pelvic coordination. To detect differences between male and female participants (df = 1), a total of 199 participants would be sufficient. Based on this power analysis and an assumed data loss ratio of at least 20% due to drop out or data quality, we recruited 306 adults for this study (156 females, 150 males, Table 1). We included participants aged between 18 and 64 years, reporting either no low-back pain or pain in the lumbar area of the trunk. Exclusion criteria were as follows: body mass index (BMI) >28, central or peripheral neurological impairments, prior spine surgery, malposition or aberration of lower extremities, pregnancy, medication with opioids or muscle relaxants, rheumatism, osteoporosis or acute infection, cardiac diseases. Study participants were recruited within the ongoing “Berlin Back Study”—a prospective cross-sectional investigation registered with the German Clinical Trial Register (DRKS-ID: DRKS00027907, DRKS00029361). The recruitment of participants was conducted through multiple channels, including local promotion at Charité-Universitätsmedizin Berlin (via mailed flyers, notice boards, online platforms, and social media), public outreach (including newspapers, magazines, podcasts, and television), collaborations with local businesses and administrative bodies, and word-of-mouth referrals. The study protocol adheres to the ethical principles outlined in the Helsinki Declaration (57). The study follows the STROBE guidelines (58) and was approved by the Ethics Committee of the Humboldt-Universität zu Berlin (HU-KSBF-EK_2021_0006). Written informed consent was obtained from all participants.
Pain intensity was assessed using the validated German version (59) of the Graded Chronic Pain Scale (60). The characteristic pain intensity (CPI) (61) was calculated as the average of 0–10 ratings of pain right now, average pain and worst pain in the last three months multiplied by 10 resulting in a score between 0 and 100. CPI scores were then used to classify all participants as either (1) asymptomatic (ASY, CPI = 0) (2), participants with low to medium pain (LMP, CPI = 1–49), or (3) participants with medium to high pain (MHP, CPI = 50–100) (60). The chronicity of low-back pain was assessed for all participants during the clinical examination conducted by an orthopedic and trauma surgery specialist. Low-back pain that persisted for 12 weeks or longer was defined as chronic (62).
All participants performed three consecutive trunk forward bending movements. They were instructed to perform the movement in a slow but comfortable pace without bending their knees and until the individual maximal trunk flexion position. The lumbo-pelvic alignment and range of motion of both the trunk and pelvis were measured using two 3-dimensional accelerometers (Biovision, Berlin; size 1 × 1 × 1 cm, 500 Hz). The sensors were attached to the skin at the level of thoracic vertebrae 12 (T12) and sacral vertebrae 1 (S1) using double-sided adhesive tape (Figure 1A).
Figure 1. (A) Two 3-dimensional accelerometers positioned at sacral vertebrae 1 (S1) and thoracic vertebrae 12 (T12) were used to measure the pelvic (pelvicangle) and trunk (trunkangle) angles during three trunk flexion movements. (B) Positive values (+) indicate a forward-rotated orientation with respect to the vertical, while negative values (−) indicate a backward-rotated orientation of the pelvis or trunk. The lumbar angle (lumbarangle) was calculated as the difference between trunkangle and pelvicangle. (C) Angles of trunk, pelvis and lumbar spine during the three trunk flexion movements. The range of motion of the pelvis (pelvicRoM), the trunk (trunkRoM) and the lumbar spine (lumbarRoM) was calculated as the difference of the respective maximum angle during the movement and the respective angle in the upright standing position. Values of the lumbar angle less than zero indicate the phase of lumbar lordosis while values of the lumbar angle above zero indicate the phase of lumbar kyphosis.
The obtained acceleration data were low pass filtered at 5 Hz using a 2nd order IIR Butterworth zero-phase filter and processed using a moving average in a time window of 500 ms. The orientation of the local coordinate system of each sensor in reference to the global space coordinate system was determined using the gravitational field. To minimize the influence of accelerations additional to the gravitational acceleration, participants were encouraged to execute the movement at a low, controlled velocity and only trials in which the norm of the gravitational vector was 1 ± 0.10 g were included in further calculations. During the trunk forward bending movement the angles of the pelvis and the trunk were measured in the sagittal plane using the orientation of the local coordinate systems of the sensors at S1 and T12 with respect to the global coordinate system. Positive values of the two angles indicate a forward-rotated orientation (with respect to the vertical), while negative values indicate a backward-rotated orientation of the pelvis or the trunk (Figure 1B). The difference between the trunk angle and the pelvic angle was used to calculate the lumbar angle with negative values indicating the magnitude of lumbar lordosis. Finally, a moving average filter was applied to the calculated angle values.
To identify start and end points of each cycle, we calculated secant slopes of the trunk angle in time increments of 100 ms. The start of a cycle was determined by the secant slope value falling below 0.1 and the end of a cycle was defined by the maximum angle value. Each cycle was time normalized to 1,000 data points and from the three performed cycles a mean trial was calculated. The range of motion of the pelvis (pelvicRoM), the trunk (trunkRoM) and the lumbar spine (lumbarRoM) was calculated as the difference of the respective maximum angle value during the trunk forward bending movement and the respective angle value in the upright standing position (Figure 1C). During the forward bending movement the duration of the lumbar lordosis and the duration of the lumbar lordosis normalized to the movement time was quantified from movement start to the first occurrence of a positive value in the lumbar angle. Further, we quantified the changes of the pelvic angle during the lumbar lordosis (pelvicRoM−Lordosis), during the lumbar kyphosis (pelvicRoM−Kyphosis) and the pelvic angle at the end of the lordotic phase to identify the orientation of the pelvis during the transition from lordosis to kyphosis. Finally, the lumbo-pelvic ratio (LPR) was calculated as the ratio of the changes in lumbar spine orientation to the changes in pelvic orientation for the whole movement (LPRfull) and for the lumbar lordosis (LPRLordosis) and kyphosis (LPRKyphosis) phases of the movement.
The participants performed a cyclic movement that comprised trunk rotation and flexion by reaching targets positioned on the left and right side of the participant (Figure 2A). The targets were mounted on metal frames and adjusted to match the participants height at eye level and the proximal border of the patella. The frames were adjusted in width to the arm span width of the respective participant. The movement was explained and demonstrated to the participants and the movement frequency of 10 cycles per minute (0.17 Hz) was introduced by a digital metronome. After a short familiarization phase participants continuously performed a total of 32 movement cycles.
Figure 2. Movement task and assessment of the local dynamic stability of the trunk. (A) Participants repeatedly executed a pointing task from position 1 over 2 with the right arm and 3–4 with the left arm with a frequency of 10 cycles per minute (0.17 Hz); (B) one-dimensional time-series x(t) derived from the norm of the 3-dimensional accelerations of the trunk (accelerometer at the level of the second thoracic vertebrae); (C) reconstructed state space of the trunk movement using dimension m = 3 and time delay τ = 75; (D) diverging Euclidean distances of a nearest neighbor pair in the reconstructed state space; (E) average logarithmic rate (ln) of divergence of all nearest neighbor pairs over time and the maximum Lyapunov exponent (λmax) as slope of the linear fit to the resulting ln(divergence) curve for 0–40 samples.
The local trunk dynamic stability was examined using the maximum finite-time Lyapunov exponent (λmax). Kinematic data were collected using a 3-dimensional accelerometer (Biovision, Berlin; size 1 × 1 × 1 cm, 500 Hz) attached to the skin at the level of the second thoracic vertebrae (T2). The obtained acceleration data were low pass filtered at 20 Hz using a 2nd order IIR Butterworth zero-phase filter. We calculated the norm of the measured 3-dimensional accelerations after subtracting the respective minima. The resulting one-dimensional time-series was demeaned and a total of 30 cycles were used for subsequent analysis (Figure 2B). The time-series was time normalized to 18,000 data points (600 data points per cycle) and the reconstruction of the trunk motion in state space (Figures 2C,D) was performed using the method of delay embedding by choosing an appropriate time delay τ and embedding dimension m as follows (Equation 1):
with S(t) representing the m-dimensional reconstructed state vector, x(t) the one-dimensional Euclidean norm series, τ the time delay, and m the embedding dimension. For each time series a constant time delay of τ = 75 was appropriate based on average mutual information analysis (63). Global false nearest neighbor's analysis (64) revealed dimension m = 3 to be sufficient for the reconstruction of the current data. Finally, the maximum Lyapunov exponent was calculated as the slope of the logarithmic average divergence curve using the algorithm of Kantz (65) for the first 40 values (Figure 2E). This parameter describes the average logarithmic divergence between initially neighboring trajectories in state space. Thus, the higher the maximum Lyapunov exponent, the more unstable the system responds locally to external mechanical induced perturbations (66) or to internal motor control perturbations (67). To quantify the variability of the trunk movement, the time series of the norm of the measured 3-dimensional accelerations during all 30 cycles was used. Each cycle was time normalized to 101 data points (0%–100%) and standard deviations were calculated across all cycles at every time point. Finally, the mean variability (MeanSD) was calculated over all values for each trial (68).
To account for possible effects of sex, pain intensity and pain chronicity on lumbo-pelvic coordination, trunk dynamic stability and trunk movement variability, we analyzed the data using linear mixed models in the style of a two way anova with interaction term with the factors sex and pain intensity and the covariate pain chronicity for the anthropometric characteristics (i.e., age, height, mass, BMI) and the kinematic parameters (i.e., movement time, trunkRoM, pelvicRoM, lumbarRoM, lumbar angle at beginning and end of trunk flexion, pelvic angle at beginning and end of trunk flexion and lumbar lordosis, pelvicRoM−Lordosis, pelvicRoM−Kyphosis, duration of lumbar lordosis, normalized duration of lumbar lordosis, LPRfull, LPRLordosis, LPRKyphosis, variability, maximum Lyapunov Exponent) by using the generalized least squares (gls) approach (69) implemented in the package “nlme” (70). In the case of a significant main or interaction effect we performed a post-hoc analysis with controlling the false discovery rate using the approach by Benjamini and Hochberg (71). All the significance levels were set to α = 0.05 and analyses were conducted in R (72).
Participants showed no significant differences in age (p = 0.103) or body height (p = 0.698) between the different pain groups (Table 1). However, male participants were significantly taller (p < 0.001), heavier (p < 0.001) and had a higher BMI (p < 0.001) than females. In the body mass (p = 0.040) and BMI (p = 0.022) of the participants we identified a significant main effect of pain intensity. Participants with medium to high pain had a higher BMI than asymptomatic participants (p = 0.027) and participants with low to medium pain (p = 0.027). No effect of pain chronicity was detected in age (p = 0.582), body height (p = 0.350), body mass (p = 0.525), and BMI (p = 0.144) of the participants.
In the time of the forward trunk bending movement no effect of sex (p = 0.132), pain intensity (p = 0.052), pain chronicity (p = 0.274) or a sex-by-pain interaction (p = 0.105) was detected (Table 2). In the trunkRoM we identified a significant main effect of sex (p < 0.001) and pain intensity (p = 0.009) but no effect of pain chronicity (p = 0.440) or a sex-by-pain interaction (p = 0.479). Females demonstrated a higher trunkRoM compared to males (p < 0.001). In participants with medium to high pain trunkRoM was significantly reduced when compared to asymptomatic participants (p = 0.006). We did not detect statistically significant differences in trunkRoM between participants with low to medium pain and participants with medium to high pain (p = 0.087). In the pelvicRoM we detected a significant main effect of sex (p < 0.001) but no effect of pain intensity (p = 0.069), pain chronicity (p = 0.998) or a sex-by-pain interaction (p = 0.223). Females demonstrated a higher pelvicRoM compared to males (p < 0.001). In the lumbarRoM we did not find any significant main effect of sex (p = 0.594), pain intensity (p = 0.052), pain chronicity (p = 0.346) or a sex-by-pain interaction (p = 0.675). The lumbar angle in the upright standing position demonstrated a significant main effect of sex (p < 0.001) and pain intensity (p = 0.002), but no effect of pain chronicity (p = 0.921) or a sex-by-pain interaction (p = 0.437; Table 3). Female participants had a significantly greater lumbar angle in the upright standing position (i.e., higher lumbar lordosis; p < 0.001). Participants with low to medium pain showed a significantly reduced lumbar angle in the upright standing position (i.e., smaller lumbar lordosis) when compared to asymptomatic participants (p = 0.049) and participants with medium to high pain (p = 0.049). We did not detect statistically significant differences in the lumbar angle during upright standing between participants with medium to high pain and asymptomatic participants (p = 0.929). In the lumbar angle at the end of the movement we detected a significant main effect of sex (p < 0.001) but no effect of pain intensity (p = 0.203), pain chronicity (p = 0.217) or a or a sex-by-pain interaction (p = 0.654). Females showed a significantly smaller lumbar angle at the end of the movement (i.e., less kyphosis) than males (p < 0.001).
Table 2. Movement time (timemov), range of motion of the trunk (trunkRoM), pelvis (pelvicRoM) and lumbar spine (lumbarRoM) of participants with different pain levels.
Table 3. Angle of the lumbar spine in the upright standing position and at the end of the movement, pelvic angle during standing, at the end of the lumbar lordosis and at the end of the movement, trunk angle during standing and at the end of the movement, changes in pelvic angle during the phases of lumbar lordosis (pelvicROM−lordosis) and lumbar kyphosis (pelvicROM−kyphosis), mean duration of the lumbar lordosis (LORdur), mean duration of the lumbar lordosis relative to the movement time (LORdur−norm) for participants with different pain levels.
In the pelvic angle during the upright standing position we identified a significant main effect of sex (p < 0.001) and pain intensity (p = 0.008), but no effect of pain chronicity (p = 0.157) or a sex-by-pain interaction (p = 0.364). Female participants had a significantly higher pelvic angle in the upright standing position (p < 0.001). In female participants with low to medium pain the pelvic angle in the upright standing position was significantly smaller than in asymptomatic female participants (p = 0.013) and female participants with medium to high pain (p = 0.047). In the pelvic angle at the end of the lordotic phase we identified a significant main effect of sex (p < 0.001) but no effect of pain intensity (p = 0.201), pain chronicity (p = 0.931) or a sex-by-pain interaction (p = 0.945). Female participants showed significantly higher pelvic angles at the end of the lordotic phase than male participants (p < 0.001). In the pelvic angle at the end of the movement we identified a significant main effect of sex (p < 0.001) and pain intensity (p = 0.036), but no effect of pain chronicity (p = 0.340) or a sex-by-pain interaction (p = 0.654). Female participants showed significantly higher pelvic angles at the end of the movement than male participants (p < 0.001). Participants with medium to high pain showed a significantly reduced pelvic angles at the end of the movement when compared to asymptomatic participants (p = 0.031).
In the trunk angle during the upright standing position we identified a significant effect of pain chronicity (p = 0.042), but no effect of sex (p = 0.292), pain intensity (p = 0.252) or a sex-by-pain interaction (p = 0.888). Participants with chronic pain had a significantly lower trunk angle in the upright standing position (p = 0.042). In the trunk angle at the end of the movement we identified a significant main effect of sex (p < 0.001) and pain intensity (p = 0.003), but no effect of pain chronicity (p = 0.947) or a sex-by-pain interaction (p = 0.400). Female participants showed significantly higher trunk angles at the end of the movement than male participants (p < 0.001). Participants with medium to high pain showed a significantly reduced trunk angle at the end of the movement when compared to asymptomatic participants (p = 0.011).
In pelvicRoM during the phase of lumbar lordosis we found a significant main effect of sex (p < 0.001), but no effect of pain intensity (p = 0.068), pain chronicity (p = 0.339) or a sex-by-pain interaction (p = 0.840). Females demonstrated significantly higher values in pelvicRoM during the phase of lumbar lordosis than males (p < 0.001). In pelvicRoM during the phase of lumbar kyphosis we detected a significant main effect of pain intensity (p = 0.027), but no effects of sex (p = 0.391), pain chronicity (p = 0.322) or a sex-by-pain interaction (p = 0.733). post-hoc analysis revealed no significant differences between the different pain groups. In the duration of lumbar lordosis and the duration of lumbar lordosis normalized to the movement time we detected a significant main effect of sex (p < 0.001), but no effect of pain intensity (p = 0.299 and p = 0.103), pain chronicity (p = 0.234 and p = 0.837) or a sex-by-pain interaction (p = 0.616 and p = 0.590). Females were significantly longer in a lordotic position when compared to males (p < 0.001).
In the LPR we detected a significant main effect of sex (p < 0.001) during the phase of the lumbar kyphosis, with females demonstrating a lower LPR during this phase of the movement (Table 4, Figure 3). No effect of pain intensity (p > 0.05), pain chronicity (p > 0.05) or a sex-by-pain interaction (p > 0.05) was evident in LPRfull, LPRLordosis and LPRKyphosis. Finally, in movement variability and the maximum Lyapunov Exponent during the cyclic pointing task no effect of sex (p = 0.822 and p = 0.543), pain intensity (p = 0.214 and p = 0.719) or a sex-by-pain interaction (p = 0.812 and p = 0.661) was detected. However, in the maximum Lyapunov Exponent we found a significant effect of pain chronicity (p = 0.008), with higher values in participants with chronic pain. In movement variability no effect of pain chronicity was detected (p = 0.247).
Table 4. Lumbo-pelvic ratio of the full movement (LPRfull), during the phase of lumbar lordosis (LPRLordosis) and lumbar kyphosis (LPRKyphosis), variability of the trunk (VAR) and maximum lyapunov exponent (MLE) of the participants with different pain levels.
Figure 3. Individual lumbar angle as a function of pelvic angle for participants with different low-back pain intensities during trunk flexion. Crosses represent means ± standard deviations at upright standing, end of lumbar lordosis and end of movement, respectively. The average slope of each segment represents the corresponding lumbo-pelvic ratio.
In this study we investigated lumbo-pelvic coordination, dynamic stability and movement variability of the trunk in a large sample of participants with low-back pain and asymptomatic controls. We identified sex-specific characteristics in measures of lumbo-pelvic coordination while pain intensity and pain chronicity only had a minor impact. Pain chronicity had an effect on trunk dynamic stability, while variability and dynamic stability of the trunk were not affected by sex and pain intensity.
During the trunk forward bending movement, female participants had a higher lumbar angle (i.e., a higher lumbar lordosis) in the upright standing position due to a higher pelvic angle. The higher pelvic angle in females was maintained throughout the entire movement, resulting in a longer duration of the lumbar lordosis. The fact that the lumbo-pelvic ratio did not differ between females and males during the lordotic phase suggests that the relative position of the pelvis and trunk is maintained in both females and males during this phase. Low-back pain had no effect on pelvic angle or lumbar lordosis, which indicates that the identified lumbo-pelvic posture and kinematics were sex-specific. A similar sex-specific behavior of the lumbo-pelvic coordination during trunk forward bending has been reported for healthy adults (23) and adolescent athletes (56). The lower lumbo-pelvic ratio found in females during the phase of lumbar kyphosis was the reason for a tendency towards a lower lumbo-pelvic ratio in females during the full range of motion (p = 0.051). When comparing asymptomatic males and females during a full trunk flexion, females generally displayed a smaller lumbo-pelvic ratio than males (23, 54, 73), mainly due to a greater pelvic range of motion and a greater angle of sacrum orientation in standing and full flexion. Sex-specific differences in sacral shape and orientation (74) or hamstring flexibility (75) might affect the lumbo-pelvic kinematics resulting in a higher pelvic angle in females. It has been argued that in female participants lower muscular strength capacities (76–79) and sex-specific characteristics in muscle morphology (80, 81) may also affect lumbar lordosis and pelvic inclination (26). However, several studies failed to support a relationship between trunk muscle strength, lumbar lordosis and pelvic inclination (56, 82, 83) suggesting that muscle strength may not be the reason for the sex-specific higher lumbar lordosis. The transmission of muscular forces and the resulting stability of the pelvis is dependent on the ligamentous network (84–86) and there is evidence that the pre-tension of the sacrotuberous ligament differ between males and females (87). Hence, sex-specific variations in the pre-tension of the sacrotuberous ligament may have the potential to influence the posture and kinematics of the lumbo-pelvic region (88–90).
A lordotic posture and higher pelvic rotation is associated with an increased loading of the spine (11, 16, 25). Arjmand and Shirazi-Adl (25) reported that lordotic postures increased pelvic rotation and trunk extensor muscle forces with concomitant increases in spine loading. The found effects of sex on lumbar lordosis and pelvic motion may increase trunk loading and can be interpreted as a possible risk factor for a low-back injury in females. Epidemiological studies reporting a higher prevalence of low-back pain in females (91–93) support the higher risk of a low-back injury in females. However, in our results, low-back pain intensity was not associated with lumbo-pelvic posture and kinematics (i.e., no effects of pain on lumbar lordosis, pelvic RoM, duration of lumbar lordosis, lumbo-pelvic ratio), indicating that lumbo-pelvic posture and kinematics may not be suitable measures to differentiate low-back pain patients and may not be a clear risk factor for a low-back injury. Participants with medium to high pain showed a reduced trunk RoM compared to asymptomatic controls, due to a lower trunk angle at the end of the forward flexion, but without differences in lumbar lordosis or pelvic rotation. Similarly, other studies (53, 55) observed no differences in lumbar lordosis and pelvic angle between participants with and without low-back pain. Some previous studies have reported an effect of low-back pain on the lumbo-pelvic ratio, with a relatively greater lumbar contribution during full trunk flexion in participants with low-back pain (19, 22, 94). When classifying patients with low-back pain into specific subgroups, Kim et al. (21) found differences in the lumbo-pelvic ratio between healthy participants and specific subgroups of low-back pain. It has therefore been suggested, that the relative contributions of the lumbar spine and the pelvis to a flexion movement may be of clinical relevance (55) with applications in diagnostics (19, 21, 22, 95), load and injury-risk estimation (13–16) and therapy of low-back pain (17, 18). When pooling data from previous experimental studies in a meta-analytic approach, Laird et al. (55), similar to our study with the large number of participants, found no significant differences in lumbo-pelvic coordination for participants with and without low-back pain. In our study, participants with low to medium pain intensity had a reduced lumbar spine angle when standing upright compared to both asymptomatic controls and participants with medium to high pain intensity. However, the participants with medium to high pain did not differ from the asymptomatic controls, so the results did not show a consistent effect of pain intensity on lumbar spine alignment. We argue that, besides the influence of lumbo-pelvic posture and kinematics on spinal loading (11), it remains unclear whether these variables can influence the prevalence of low-back pain.
We did not detect any significant main effect of sex and pain intensity on trunk dynamic stability and trunk movement variability during the used movement task. There are indications of differences in the variability of trunk movements between people with low-back pain and asymptomatic controls (43, 44). However, the characterization of these modifications is currently not well understood (49). Heterogeneous metrics and inclusion criteria limit the quality of evidence, making it difficult to draw conclusive inferences. Several previous studies also reported no main effect of low-back pain on the spatial variability and local dynamic stability of trunk kinematics during repetitive reaching tasks (39, 96). When reducing pain intensity by implementing an exercise therapy, Arampatzis et al. (50) reported unchanged local dynamic stability of trunk motion despite a significant reduction in low-back pain. Recent reviews, however, show inconsistent results (52) reporting differences (97) or no differences (51) in movement variability between participants with low-back pain and asymptomatic controls. It appears that both the variability and the local dynamic stability of trunk movement may not be sensitive enough to detect an association with low-back pain intensity. Although pain intensity did not affect local dynamic stability, we found higher values of the short-term Lyapunov exponent in chronic low-back-pain patients, indicating higher local trunk instability in these participants. It seems that chronic pain, in our case at least 12 weeks of continuous pain, may induce internal control errors in the regulation of trunk movement during cyclic coordinative tasks involving flexion-extension and rotation, worsening the local dynamic stability of the trunk. These findings indicate that non-linear analysis of trunk movement kinematics may be a useful tool in chronic low-back pain patients and could be considered in the clinical diagnosis and management of low-back-pain.
There are some limitations associated with the approach used in the current study. It is worth noting that with the accelerometers in our study we estimated the sacrum orientation as part of the pelvic girdle and not the pelvic tilt or the curvature of the lumbar spine directly. Still, our results are in agreement with those from previous studies that assessed lumbo-pelvic coordination using noninvasive methods (11, 20, 22, 56, 98) and it has been argued that sacral and pelvic ranges of motion can be assumed to be equal (23). Further, it has been demonstrated that the curvature measured on the back surface significantly correlates with angles measured from x-rays (99) and provides a reasonable accurate measurement of the total lumbar motion (100). To further account for possible differences between sensor position and subdermal anatomical structures, subjects with a BMI >28.0 kg/m2 were excluded, yet limiting our results to only a subgroup of the general population. Finally, it can be argued that the age of the participants may influence lumbo-pelvic kinematics. We further analyzed our data regarding participants age and detected an effect of age in measures of lumbo-pelvic kinematics such as the lumbar angle during upright standing (i.e., lumbar lordosis, p = 0.039), the lumbo-pelvic ratio (LPR) during the full movement (p < 0.001) and the phase of lumbar kyphosis (p = 0.006), range of motion of the lumbar spine (p < 0.001) and the pelvis during full movement (p = 0.004) and during the phase of lumbar lordosis (p = 0.032). Importantly, the previously detected main effects of sex, pain intensity and pain chronicity did remain. The findings show, as also reported earlier (23, 101), that age may influence lumbo-pelvic kinematics.
In summary this current study highlights the effects of sex and low-back pain intensity on lumbo-pelvic coordination and trunk kinematics in standing and during trunk flexion. The presented results emphasize sex-specific characteristics in measures of lumbo-pelvic coordination while pain intensity and pain chronicity appear to have a minor impact. Our results suggest that lumbo-pelvic posture and kinematics during a trunk flexion movement have limited practicality in the clinical diagnosis and management of low-back pain. The increased local instability of the trunk during the cyclic coordination task studied indicates control errors in the regulation of trunk movement in participants with chronic low-back pain and could be considered a useful diagnostic tool in chronic low-back pain.
The datasets presented in this study can be found in online repositories. The names of the repository/repositories and accession number(s) can be found below: https://zenodo.org/records/15044691.
The studies involving humans were approved by Ethics Committee of the Humboldt-Universität zu Berlin (HU-KSBF-EK_2021_0006). The studies were conducted in accordance with the local legislation and institutional requirements. The participants provided their written informed consent to participate in this study.
LF: Conceptualization, Methodology, Formal analysis, Investigation, Data curation, Writing – original draft, Writing – review & editing, Project administration. AS: Data curation, Formal analysis, Investigation, Methodology, Project administration, Software, Writing – review & editing. HS: Funding acquisition, Project administration, Writing – review & editing. AA: Conceptualization, Formal analysis, Funding acquisition, Methodology, Project administration, Resources, Supervision, Writing – original draft, Writing – review & editing.
The author(s) declare that financial support was received for the research and/or publication of this article. This study is part of the Research Unit 5177 and was financed by the German Research Foundation (DFG, AR 809/2-1, SCHM 2572/12-1, SCHM 2572/11-1). The funders had no role in the study design, data collection and analysis, decision to publish or preparation of the manuscript. Open Access funding was enabled by the Open Access Publication Fund of Humboldt-Universität zu Berlin.
We acknowledge the support by Theresa Ahnert and Barbara Schiller for the organization of the recruitment.
The authors declare that the research was conducted in the absence of any commercial or financial relationships that could be construed as a potential conflict of interest.
The author(s) declared that they were an editorial board member of Frontiers, at the time of submission. This had no impact on the peer review process and the final decision.
The author(s) declare that no Generative AI was used in the creation of this manuscript.
All claims expressed in this article are solely those of the authors and do not necessarily represent those of their affiliated organizations, or those of the publisher, the editors and the reviewers. Any product that may be evaluated in this article, or claim that may be made by its manufacturer, is not guaranteed or endorsed by the publisher.
1. GBD 2017 Disease and Injury Incidence and Prevalence Collaborators. Global, regional, and national incidence, prevalence, and years lived with disability for 354 diseases and injuries for 195 countries and territories, 1990–2017: a systematic analysis for the Global Burden of Disease Study 2017. Lancet. (2018) 392(10159):1789–858. doi: 10.1016/S0140-6736(18)32279-7
2. Cieza A, Causey K, Kamenov K, Hanson SW, Chatterji S, Vos T. Global estimates of the need for rehabilitation based on the Global Burden of Disease Study 2019: a systematic analysis for the Global Burden of Disease Study 2019. Lancet. (2020) 396(10267):2006–17. doi: 10.1016/S0140-6736(20)32340-0
3. Solumsmoen S, Poulsen G, Kjellberg J, Melbye M, Munch TN. The impact of specialised treatment of low back pain on health care costs and productivity in a nationwide cohort. EClinicalMedicine. (2022) 43:101247. doi: 10.1016/j.eclinm.2021.101247
4. Aksekili MAE. Low back pain. In: Longo UG, Denaro V, editors. Textbook of Musculoskeletal Disorders. Cham: Springer International Publishing (2023). p. 329–37. doi: 10.1007/978-3-031-20987-1_37
5. Al Ameri K, Al Memari S. Performing back physical examination. In: Lari S, Al Memari S, Al Marzooqi D, editors. Family Medicine OSCE: First Aid to Objective Structured Clinical Examination. Singapore: Springer Nature (2024). p. 299–300. doi: 10.1007/978-981-99-5530-5_78
6. Jansen JP, Burdorf A, Steyerberg E. A novel approach for evaluating level, frequency and duration of lumbar posture simultaneously during work. Scand J Work Environ Health. (2001) 27(6):373–80. doi: 10.5271/sjweh.629
7. Nachemson A. The effect of forward leaning on lumbar intradiscal pressure. Acta Orthop Scand. (1965) 35(1–4):314–28. doi: 10.3109/17453676508989362
8. Nachemson AL. Disc pressure measurements. Spine. (1981) 6(1):93–7. doi: 10.1097/00007632-198101000-00020
9. Wilke H, Neef P, Hinz B, Seidel H, Claes L. Intradiscal pressure together with anthropometric data–a data set for the validation of models. Clin Biomech. (2001) 16(Suppl 1):S111–126. doi: 10.1016/S0268-0033(00)00103-0
10. Takahashi I, Kikuchi S-i, Sato K, Sato N. Mechanical load of the lumbar spine during forward bending motion of the trunk-a biomechanical study. Spine. (2006) 31(1):18–23. doi: 10.1097/01.brs.0000192636.69129.fb
11. Tafazzol A, Arjmand N, Shirazi-Adl A, Parnianpour M. Lumbopelvic rhythm during forward and backward sagittal trunk rotations: combined in vivo measurement with inertial tracking device and biomechanical modeling. Clin Biomech. (2014) 29(1):7–13. doi: 10.1016/j.clinbiomech.2013.10.021
12. Liu T, Khalaf K, Adeeb S, El-Rich M. Effects of lumbo-pelvic rhythm on trunk muscle forces and disc loads during forward flexion: a combined musculoskeletal and finite element simulation study. J Biomech. (2019) 82:116–23. doi: 10.1016/j.jbiomech.2018.10.009
13. Cholewicki J, McGill SM. Mechanical stability of the in vivo lumbar spine: implications for injury and chronic low back pain. Clin Biomech. (1996) 11(1):1–15. doi: 10.1016/0268-0033(95)00035-6
14. Arjmand N, Plamondon A, Shirazi-Adl A, Larivière C, Parnianpour M. Predictive equations to estimate spinal loads in symmetric lifting tasks. J Biomech. (2011) 44(1):84–91. doi: 10.1016/j.jbiomech.2010.08.028
15. Firouzabadi A, Arjmand N, Zhang T, Pumberger M, Schmidt H. Effect of low back pain on the kinetics and kinematics of the lumbar spine—a combined in vivo and in silico investigation. J Biomech. (2024) 164:111954. doi: 10.1016/j.jbiomech.2024.111954
16. Ghezelbash F, Shirazi-Adl A, Arjmand N, El-Ouaaid Z, Plamondon A, Meakin JR. Effects of sex, age, body height and body weight on spinal loads: sensitivity analyses in a subject-specific trunk musculoskeletal model. J Biomech. (2016) 49(14):3492–501. doi: 10.1016/j.jbiomech.2016.09.026
17. Ikeda DM, McGill SM. Can altering motions, postures, and loads provide immediate low back pain relief: a study of 4 cases investigating spine load, posture, and stability. Spine. (2012) 37(23):E1469–1475. doi: 10.1097/BRS.0b013e31826c97e5
18. O’Sullivan P. Diagnosis and classification of chronic low back pain disorders: maladaptive movement and motor control impairments as underlying mechanism. Man Ther. (2005) 10(4):242–55. doi: 10.1016/j.math.2005.07.001
19. Esola MA, McClure PW, Fitzgerald GK, Siegler S. Analysis of lumbar spine and hip motion during forward bending in subjects with and without a history of low back pain. Spine. (1996) 21(1):71–8. doi: 10.1097/00007632-199601010-00017
20. Granata KP, Sanford AH. Lumbar-pelvic coordination is influenced by lifting task parameters. Spine. (2000) 25(11):1413–8. doi: 10.1097/00007632-200006010-00014
21. Kim MH, Yi CH, Kwon OY, Cho SH, Cynn HS, Kim YH, et al. Comparison of lumbopelvic rhythm and flexion-relaxation response between 2 different low back pain subtypes. Spine. (2013) 38(15):1260–7. doi: 10.1097/BRS.0b013e318291b502
22. Porter JL, Wilkinson A. Lumbar-hip flexion motion. A comparative study between asymptomatic and chronic low back pain in 18- to 36-year-old men. Spine. (1997) 22(13):1508–14. doi: 10.1097/00007632-199707010-00017
23. Pries E, Dreischarf M, Bashkuev M, Putzier M, Schmidt H. The effects of age and gender on the lumbopelvic rhythm in the sagittal plane in 309 subjects. J Biomech. (2015) 48(12):3080–7. doi: 10.1016/j.jbiomech.2015.07.030
24. Arshad R, Pan F, Reitmaier S, Schmidt H. Effect of age and sex on lumbar lordosis and the range of motion. A systematic review and meta-analysis. J Biomech. (2019) 82:1–19. doi: 10.1016/j.jbiomech.2018.11.022
25. Arjmand N, Shirazi-Adl A. Biomechanics of changes in lumbar posture in static lifting. Spine. (2005) 30(23):2637–48. doi: 10.1097/01.brs.0000187907.02910.4f
26. Been E, Kalichman L. Lumbar lordosis. Spine J. (2014) 14(1):87–97. doi: 10.1016/j.spinee.2013.07.464
27. Christie HJ, Kumar S, Warren SA. Postural aberrations in low back pain. Arch Phys Med Rehabil. (1995) 76(3):218–24. doi: 10.1016/S0003-9993(95)80604-0
28. Kerr MS, Frank JW, Shannon HS, Norman RW, Wells RP, Neumann WP, et al. Biomechanical and psychosocial risk factors for low back pain at work. Am J Public Health. (2001) 91(7):1069–75. doi: 10.2105/ajph.91.7.1069
29. Parreira P, Maher CG, Steffens D, Hancock MJ, Ferreira ML. Risk factors for low back pain and sciatica: an umbrella review. Spine J. (2018) 18(9):1715–21. doi: 10.1016/j.spinee.2018.05.018
30. Stevenson JM, Weber CL, Smith JT, Dumas GA, Albert WJ. A longitudinal study of the development of low back pain in an industrial population. Spine. (2001) 26(12):1370–7. doi: 10.1097/00007632-200106150-00022
31. Taylor JB, Goode AP, George SZ, Cook CE. Incidence and risk factors for first-time incident low back pain: a systematic review and meta-analysis. Spine J. (2014) 14(10):2299–319. doi: 10.1016/j.spinee.2014.01.026
32. Bigos SJ, Battie MC, Spengler DM, Fisher LD, Fordyce WE, Hansson T, et al. A longitudinal, prospective study of industrial back injury reporting. Clin Orthop Relat Res. (1992) 279:21–34. doi: 10.1097/00003086-199206000-00004
33. Carroll LJ, Cassidy JD, Côté P. Depression as a risk factor for onset of an episode of troublesome neck and low back pain. Pain. (2004) 107(1–2):134–9. doi: 10.1016/j.pain.2003.10.009
34. Hoogendoorn WE, Bongers PM, de Vet HC, Houtman IL, Ariëns GA, van Mechelen W, et al. Psychosocial work characteristics and psychological strain in relation to low-back pain. Scand J Work Environ Health. (2001) 27(4):258–67. doi: 10.5271/sjweh.613
35. Battié MC, Bigos SJ, Fisher LD, Spengler DM, Hansson TH, Nachemson AL, et al. Anthropometric and clinical measures as predictors of back pain complaints in industry: a prospective study. J Spinal Disord. (1990) 3(3):195–204.2151987
36. Battié MC, Bigos SJ, Fisher LD, Hansson TH, Nachemson AL, Spengler DM, et al. A prospective study of the role of cardiovascular risk factors and fitness in industrial back pain complaints. Spine. (1989) 14(2):141–7. doi: 10.1097/00007632-198902000-00001
37. Feldman DE, Rossignol M, Shrier I, Abenhaim L. Smoking. A risk factor for development of low back pain in adolescents. Spine. (1999) 24(23):2492–6. doi: 10.1097/00007632-199912010-00011
38. Cholewicki J, Silfies SP, Shah RA, Greene HS, Reeves NP, Alvi K, et al. Delayed trunk muscle reflex responses increase the risk of low back injuries. Spine. (2005) 30(23):2614–20. doi: 10.1097/01.brs.0000188273.27463.bc
39. Graham RB, Oikawa LY, Ross GB. Comparing the local dynamic stability of trunk movements between varsity athletes with and without non-specific low back pain. J Biomech. (2014) 47(6):1459–64. doi: 10.1016/j.jbiomech.2014.01.033
40. Willigenburg NW, Kingma I, Hoozemans MJ, van Dieën JH. Precision control of trunk movement in low back pain patients. Hum Mov Sci. (2013) 32(1):228–39. doi: 10.1016/j.humov.2012.12.007
41. Granata KP, England SA. Stability of dynamic trunk movement. Spine. (2006) 31(10):E271–6. doi: 10.1097/01.brs.0000216445.28943.d1
42. Rosenstein MT, Collins JJ, De Luca CJ. A practical method for calculating largest lyapunov exponents from small data sets. Phys D Nonlinear Phenom. (1993) 65(1):117–34. doi: 10.1016/0167-2789(93)90009-P
43. Dideriksen JL, Gizzi L, Petzke F, Falla D. Deterministic accessory spinal movement in functional tasks characterizes individuals with low back pain. Clin Neurophysiol. (2014) 125(8):1663–8. doi: 10.1016/j.clinph.2013.11.037
44. van den Hoorn W, Bruijn SM, Meijer OG, Hodges PW, van Dieën JH. Mechanical coupling between transverse plane pelvis and thorax rotations during gait is higher in people with low back pain. J Biomech. (2012) 45(2):342–7. doi: 10.1016/j.jbiomech.2011.10.024
45. Harbourne RT, Stergiou N. Movement variability and the use of nonlinear tools: principles to guide physical therapist practice. Phys Ther. (2009) 89(3):267–82. doi: 10.2522/ptj.20080130
46. Stergiou N, Decker LM. Human movement variability, nonlinear dynamics, and pathology: is there a connection? Hum Mov Sci. (2011) 30(5):869–88. doi: 10.1016/j.humov.2011.06.002
47. Dingwell JB, Cusumano JP, Cavanagh PR, Sternad D. Local dynamic stability versus kinematic variability of continuous overground and treadmill walking. J Biomech Eng. (2001) 123(1):27–32. doi: 10.1115/1.1336798
48. Lockhart T, Stergiou N. New perspectives in human movement variability. Ann Biomed Eng. (2013) 41(8):1593–4. doi: 10.1007/s10439-013-0852-0
49. van Dieën JH, Reeves NP, Kawchuk G, van Dillen LR, Hodges PW. Analysis of motor control in patients with low back pain: a key to personalized care? J Orthop Sports Phys Ther. (2019) 49(6):380–8. doi: 10.2519/jospt.2019.7916
50. Arampatzis A, Schroll A, Moreno Catala M, Laube G, Schuler S, Dreinhofer K. A random-perturbation therapy in chronic non-specific low-back pain patients: a randomised controlled trial. Eur J Appl Physiol. (2017) 117(12):2547–60. doi: 10.1007/s00421-017-3742-6
51. Bakar F A, Staal JB, van Cingel R, Saito H, Ostelo R, van Dieën JH. Not all movements are equal: differences in the variability of trunk motor behavior between people with and without low back pain-A systematic review with descriptive synthesis. PLoS One. (2023) 18(9):e0286895. doi: 10.1371/journal.pone.0286895
52. Saito H, Watanabe Y, Kutsuna T, Futohashi T, Kusumoto Y, Chiba H, et al. Spinal movement variability associated with low back pain: a scoping review. PLoS One. (2021) 16(5):e0252141. doi: 10.1371/journal.pone.0252141
53. Laird RA, Kent P, Keating JL. How consistent are lordosis, range of movement and lumbo-pelvic rhythm in people with and without back pain? BMC Musculoskelet Disord. (2016) 17(1):403. doi: 10.1186/s12891-016-1250-1
54. Pan F, Firouzabadi A, Zander T, Schmidt H. Sex-dependent differences in lumbo-pelvic coordination for different lifting tasks: a study on asymptomatic adults. J Biomech. (2020) 102:109505. doi: 10.1016/j.jbiomech.2019.109505
55. Laird RA, Gilbert J, Kent P, Keating JL. Comparing lumbo-pelvic kinematics in people with and without back pain: a systematic review and meta-analysis. BMC Musculoskelet Disord. (2014) 15:229. doi: 10.1186/1471-2474-15-229
56. Arampatzis A, Frank J, Laube G, Mersmann F. Trunk muscle strength and lumbo-pelvic kinematics in adolescent athletes: effects of age and sex. Scand J Med Sci Sports. (2019) 29(11):1691–8. doi: 10.1111/sms.13503
57. WMA—The World Medical Association. Declaration of Helsinki (2013). Verfügbar unter: https://www.wma.net/what-we-do/medical-ethics/declaration-of-helsinki/ (zitiert Juni 13, 2023).
58. von Elm E, Altman DG, Egger M, Pocock SJ, Gøtzsche PC, Vandenbroucke JP, et al. The strengthening the reporting of observational studies in epidemiology (STROBE) statement: guidelines for reporting observational studies. Lancet. (2007) 370(9596):1453–7. doi: 10.1016/S0140-6736(07)61602-X
59. Klasen BW, Hallner D, Schaub C, Willburger R, Hasenbring M. Validation and reliability of the German version of the chronic pain grade questionnaire in primary care back pain patients. Psychosoc Med. (2004) 1:Doc07.19742049
60. Von Korff M, Ormel J, Keefe FJ, Dworkin SF. Grading the severity of chronic pain. Pain. (1992) 50(2):133–49. doi: 10.1016/0304-3959(92)90154-4
61. Dworkin SF, Von Korff M, Whitney CW, Le Resche L, Dicker BG, Barlow W. Measurement of characteristic pain intensity in field research. Pain. (1990) 41:S290. doi: 10.1016/0304-3959(90)92696-N
62. Cohen SP, Vase L, Hooten WM. Chronic pain: an update on burden, best practices, and new advances. Lancet. (2021) 397(10289):2082–97. doi: 10.1016/S0140-6736(21)00393-7
63. Fraser AM, Swinney HL. Independent coordinates for strange attractors from mutual information. Phys Rev A Gen Phys. (1986) 33(2):1134–40. doi: 10.1103/PhysRevA.33.1134
64. Kennel MB, Brown R, Abarbanel HD. Determining embedding dimension for phase-space reconstruction using a geometrical construction. Phys Rev A. (1992) 45(6):3403–11. doi: 10.1103/PhysRevA.45.3403
65. Kantz H. A robust method to estimate the maximal lyapunov exponent of a time series. Phys Lett A. (1994) 185(1):77–87. doi: 10.1016/0375-9601(94)90991-1
66. Santuz A, Ekizos A, Eckardt N, Kibele A, Arampatzis A. Challenging human locomotion: stability and modular organisation in unsteady conditions. Sci Rep. (2018) 8(1):2740. doi: 10.1038/s41598-018-21018-4
67. Janshen L, Santuz A, Ekizos A, Arampatzis A. Fuzziness of muscle synergies in patients with multiple sclerosis indicates increased robustness of motor control during walking. Sci Rep. (2020) 10(1):7249. doi: 10.1038/s41598-020-63788-w
68. Dingwell JB, Marin LC. Kinematic variability and local dynamic stability of upper body motions when walking at different speeds. J Biomech. (2006) 39(3):444–52. doi: 10.1016/j.jbiomech.2004.12.014
69. Pinheiro J, Bates DM. Mixed-Effects Models in S and S-PLUS. New York: Springer-Verlag (2000). (Statistics and Computing). Verfügbar unter: http://link.springer.com/10.1007/b98882 (zitiert November 16, 2023).
70. Pinheiro J, Bates D. nlme: linear and nonlinear mixed effects models. Version 3.1-167 (2025). Available at: https://CRAN.R-project.org/package=nlme (Accessed March 01, 2025).
71. Benjamini Y, Hochberg Y. Controlling the false discovery rate: a practical and powerful approach to multiple testing. J R Stat Soc Ser B. (1995) 57(1):289–300. doi: 10.1111/j.2517-6161.1995.tb02031.x
72. R Core Team. R: A Language and Environment for Statistical Computing. Vienna, Austria: R Foundation for statistical Computing (2023). Verfügbar unter: https://www.R-project.org/.
73. Shojaei I, Vazirian M, Croft E, Nussbaum MA, Bazrgari B. Age related differences in mechanical demands imposed on the lower back by manual material handling tasks. J Biomech. (2016) 49(6):896–903. doi: 10.1016/j.jbiomech.2015.10.037
74. Vialle R, Levassor N, Rillardon L, Templier A, Skalli W, Guigui P. Radiographic analysis of the sagittal alignment and balance of the spine in asymptomatic subjects. J Bone Joint Surg Am. (2005) 87(2):260–7. doi: 10.2106/JBJS.D.02043
75. Blackburn JT, Bell DR, Norcross MF, Hudson JD, Kimsey MH. Sex comparison of hamstring structural and material properties. Clin Biomech. (2009) 24(1):65–70. doi: 10.1016/j.clinbiomech.2008.10.001
76. Gomez T, Beach G, Cooke C, Hrudey W, Goyert P. Normative database for trunk range of motion, strength, velocity, and endurance with the isostation B-200 lumbar dynamometer. Spine. (1991) 16(1):15–21. doi: 10.1097/00007632-199101000-00003
77. Kumar S, Dufresne RM, Van Schoor T. Human trunk strength profile in flexion and extension. Spine. (1995) 20(2):160–8. doi: 10.1097/00007632-199501150-00006
78. Kumar S, Chaffin DB, Redfern M. Isometric and isokinetic back and arm lifting strengths: device and measurement. J Biomech. (1988) 21(1):35–44. doi: 10.1016/0021-9290(88)90189-3
79. Langrana NA, Lee CK, Alexander H, Mayott CW. Quantitative assessment of back strength using isokinetic testing. Spine. (1984) 9(3):287–90. doi: 10.1097/00007632-198404000-00011
80. Jorgensen MJ, Marras WS, Granata KP, Wiand JW. MRI-derived moment-arms of the female and male spine loading muscles. Clin Biomech. (2001) 16(3):182–93. doi: 10.1016/S0268-0033(00)00087-5
81. Marras WS, Jorgensen MJ, Granata KP, Wiand B. Female and male trunk geometry: size and prediction of the spine loading trunk muscles derived from MRI. Clin Biomech. (2001) 16(1):38–46. doi: 10.1016/S0268-0033(00)00046-2
82. Arampatzis A, Laube G, Schroll A, Frank J, Bohm S, Mersmann F. Perturbation-based exercise for prevention of low-back pain in adolescent athletes. Transl Sports Med. (2021) 4:128–37. doi: 10.1002/tsm2.191
83. Youdas JW, Garrett TR, Egan KS, Therneau TM. Lumbar lordosis and pelvic inclination in adults with chronic low back pain. Phys Ther. (2000) 80(3):261–75. doi: 10.1093/ptj/80.3.261
84. Böhme J, Lingslebe U, Steinke H, Werner M, Slowik V, Josten C, et al. The extent of ligament injury and its influence on pelvic stability following type II anteroposterior compression pelvic injuries–A computer study to gain insight into open book trauma. J Orthop Res. (2014) 32(7):873–9. doi: 10.1002/jor.22618
85. Henyš P, Ramezani M, Schewitz D, Höch A, Möbius D, Ondruschka B, et al. Sacrospinous and sacrotuberous ligaments influence in pelvis kinematics. J Anat. (2022) 241(4):928–37. doi: 10.1111/joa.13739
86. Snijders CJ, Hermans PFG, Niesing R, Spoor CW, Stoeckart R. The influence of slouching and lumbar support on iliolumbar ligaments, intervertebral discs and sacroiliac joints. Clin Biomech. (2004) 19(4):323–29. doi: 10.1016/j.clinbiomech.2004.01.006
87. Steinke H, Kurz S, Fischer B, Schuberth S, Hobusch C, Heyde CE. The sacrotuberous ligament is preloaded in situ. J Mech Behav Biomed Mater. (2022) 134:105368. doi: 10.1016/j.jmbbm.2022.105368
88. Aldabe D, Hammer N, Flack NAMS, Woodley SJ. A systematic review of the morphology and function of the sacrotuberous ligament. Clin Anat. (2019) 32(3):396–407. doi: 10.1002/ca.23328
89. Hammer N, Scholze M, Kibsgård T, Klima S, Schleifenbaum S, Seidel T, et al. Physiological in vitro sacroiliac joint motion: a study on three-dimensional posterior pelvic ring kinematics. J Anat. (2019) 234(3):346–58. doi: 10.1111/joa.12924
90. Hammer N, Steinke H, Lingslebe U, Bechmann I, Josten C, Slowik V, et al. Ligamentous influence in pelvic load distribution. Spine J. (2013) 13(10):1321–30. doi: 10.1016/j.spinee.2013.03.050
91. Fett D, Trompeter K, Platen P. Back pain in elite sports: a cross-sectional study on 1,114 athletes. PLoS One. (2017) 12(6):e0180130. doi: 10.1371/journal.pone.0180130
92. Keogh E. Sex and gender differences in pain: past, present, and future. Pain. (2022) 163(Suppl 1):S108–16. doi: 10.1097/j.pain.0000000000002738
93. Kjaer P, Wedderkopp N, Korsholm L, Leboeuf-Yde C. Prevalence and tracking of back pain from childhood to adolescence. BMC Musculoskelet Disord. (2011) 12:98. doi: 10.1186/1471-2474-12-98
94. McClure PW, Esola M, Schreier R, Siegler S. Kinematic analysis of lumbar and hip motion while rising from a forward, flexed position in patients with and without a history of low back pain. Spine. (1997) 22(5):552–8. doi: 10.1097/00007632-199703010-00019
95. Larivière C, Gagnon D, Loisel P. The effect of load on the coordination of the trunk for subjects with and without chronic low back pain during flexion-extension and lateral bending tasks. Clin Biomech. (2000) 15(6):407–16. doi: 10.1016/S0268-0033(00)00006-1
96. Wildenbeest MH, Kiers H, Tuijt M, van Dieën JH. Associations of low-back pain and pain-related cognitions with lumbar movement patterns during repetitive seated reaching. Gait Posture. (2022) 91:216–22. doi: 10.1016/j.gaitpost.2021.10.032
97. Alsubaie AM, Mazaheri M, Martinez-Valdes E, Falla D. Is movement variability altered in people with chronic non-specific low back pain? A systematic review. PLoS One. (2023) 18(6):e0287029. doi: 10.1371/journal.pone.0287029
98. Nelson JM, Walmsley RP, Stevenson JM. Relative lumbar and pelvic motion during loaded spinal flexion/extension. Spine. (1995) 20(2):199–204. doi: 10.1097/00007632-199501150-00013
99. Adams MA, Dolan P, Marx C, Hutton WC. An electronic inclinometer technique for measuring lumbar curvature. Clin Biomech. (1986) 1(3):130–4. doi: 10.1016/0268-0033(86)90002-1
100. Stokes IA, Bevins TM, Lunn RA. Back surface curvature and measurement of lumbar spinal motion. Spine. (1987) 12(4):355–61. doi: 10.1097/00007632-198705000-00009
Keywords: lumbo-pelvic rhythm, spine alignment, trunk stability, trunk variability, lordosis
Citation: Fischer L, Schroll A, Schmidt H and Arampatzis A (2025) Sex-specific trunk movement coordination in participants with low-back pain and asymptomatic controls. Front. Sports Act. Living 7:1524489. doi: 10.3389/fspor.2025.1524489
Received: 7 November 2024; Accepted: 19 March 2025;
Published: 1 April 2025.
Edited by:
Huub Maas, VU Amsterdam, NetherlandsReviewed by:
Kuan Wang, Shanghai University of Medicine and Health Sciences, ChinaCopyright: © 2025 Fischer, Schroll, Schmidt and Arampatzis. This is an open-access article distributed under the terms of the Creative Commons Attribution License (CC BY). The use, distribution or reproduction in other forums is permitted, provided the original author(s) and the copyright owner(s) are credited and that the original publication in this journal is cited, in accordance with accepted academic practice. No use, distribution or reproduction is permitted which does not comply with these terms.
*Correspondence: Adamantios Arampatzis, YS5hcmFtcGF0emlzQGh1LWJlcmxpbi5kZQ==
†ORCID:
Lukas Fischer
orcid.org/0009-0001-5708-5346
Adamantios Arampatzis
orcid.org/0000-0002-4985-0335
Disclaimer: All claims expressed in this article are solely those of the authors and do not necessarily represent those of their affiliated organizations, or those of the publisher, the editors and the reviewers. Any product that may be evaluated in this article or claim that may be made by its manufacturer is not guaranteed or endorsed by the publisher.
Research integrity at Frontiers
Learn more about the work of our research integrity team to safeguard the quality of each article we publish.