- 1Geriatric Centre, Heidelberg University Hospital, Agaplesion Bethanien Hospital Heidelberg, Heidelberg, Germany
- 2Unit of Digital Geriatric Medicine, Heidelberg University Hospital, Heidelberg, Germany
- 3Optimization, Robotics, and Biomechanics, Institute of Computer Engineering, Heidelberg University, Heidelberg, Germany
- 4Translational and Clinical Research Institute (TCRI), Newcastle University, Newcastle, United Kingdom
- 5Department of Human Movement, Training and Active Aging, Institute of Sports and Sports Science, Heidelberg University, Heidelberg, Germany
Introduction: The ability to respond effectively to external perturbations is crucial for avoiding falls. The Stepping Threshold Test (STT) has been developed to assess this reactive balance, but its ability to discriminate between fallers and non-fallers is still unsubstantiated. This study aimed to evaluate the discriminant validity of the STT in distinguishing fallers and non-fallers and its convergent validity.
Methods: Thirty-six older adults (age = 80 ± 5 years), with 13 (36%) of them reporting a fall history in the past year, completed the STT on a perturbation treadmill. They received surface perturbations of progressively increasing magnitude while standing. Single- and multiple-step thresholds were assessed using an all-step count evaluation (STT-ACE), and a direction-sensitive evaluation strategy (STT-DSE). Receiver operating characteristics and area under the curves (AUC) were analyzed to evaluate the discriminative accuracy. Convergent validity was explored by 13 hypothesized associations with other mobility, psychological, and cognitive assessments.
Results: Fallers and non-fallers significantly differed in the STT-DSE (p = 0.033), but not in the STT-ACE or other commonly used mobility assessments. Acceptable discriminative accuracy was obtained for the STT-DSE (AUC = 0.72), but not for the STT-ACE and other mobility assessments (AUC = 0.53–0.68). Twelve (92%) associations were consistent with our hypotheses for the STT-DSE, and ten (77%) for the STT-ACE.
Conclusion: Our findings provide preliminary evidence that the STT, when using the STT-DSE, may discriminate between older adult fallers and non-fallers. The STT appears to be a valid tool for assessing reactive balance, with its STT-DSE being recommended due to its better discriminant and convergent validity compared to the STT-ACE.
1 Introduction
Falls among older people can be serious incidents that too often lead to personal and social consequences such as avoidance of physical activity, fear of falling, or financial burden for health care. Effective fall prevention involves early identification of individuals at risk of falling. According to the World Falls Guidelines, the assessment of gait and balance is strongly recommended for fall risk assessment (1). Commonly used assessment tools for evaluating gait and balance in relation to fall risk (1, 2) include gait speed tests (3), Timed Up and Go (TUG) (4), Berg Balance Scale (BBS) (5), 5-chair stand test (3), and Short Physical Performance Battery (SPPB) (3). However, previous studies have indicated that most of these tools are inconsistent in their ability to predict or differentiate between older adult fallers and non-fallers (1, 2, 6, 7).
Reactive balance, defined as the ability to respond effectively to external perturbations (e.g., slips, trips) in order to avoid a loss of balance (8), plays a crucial role in the multifactorial aetiology of falls. Notably, slipping and tripping are the most common circumstances in which falls occur among older adults (9–13). As the purpose of fall risk assessment is to address the mechanism of falls and contributing risk factors (1), reactive balance should be an integral part of evaluating an individual's risk of falling (14, 15). However, most frequently used mobility assessments that aim to evaluate fall risk such as gait speed tests, TUG, BBS or SPPB do not measure reactive balance abilities. In contrast, the Balance Evaluation Systems Test (BESTest) (16) or the Performance-Oriented Mobility Assessment (POMA) (17), two other established gait and balance assessment tools, contain single items on reactive balance. However, they have a limited ability to specifically assess reactive balance since the few reactive balance items in both tests have a low level of unpredictability concerning unexpected loss of balance, which is crucial for testing reactive balance (18). To date, specific tests on reactive balance are rare and usually consist of waist-pulls, tether-releases, or platform motions (15). These methods may, however, also be constrained by one-directional perturbations, low unpredictability, difficulty in adjusting the perturbation magnitude, and/or a narrow focus on only reactive stepping rather than overall reactive balance—all of which have been mentioned as important aspects for assessing reactive balance (15, 18–20). Recent developments in treadmill technologies enable the application of perturbations in various directions, with a precise adjustment of their magnitude and high level of unpredictability (21).
The Stepping Threshold Test (STT) (22) has recently been developed for specific reactive balance assessment on a perturbation treadmill that evaluates compensatory stepping responses to unexpected surface perturbations of increasing magnitude in anterior-posterior (AP) and medio-lateral (ML) directions. The convergent validity of the STT has been documented in fall-prone older adults by testing hypotheses on associations with measures of global and static balance (Brief-BESTest, 8-level balance scale), functional mobility (TUG), and fear of falling [Short Falls Efficacy Scale-International (Short FES-I)] (22). In addition, the STT evaluation strategy in classifying reactive balance responses has been proven to be inter-observer reliable in healthy adults and stroke patients, and convergently valid (BBS, 6-min walk test, FES-I) in stroke patients (23). However, there is still a lack of evidence on the discriminant validity of the STT in distinguishing between fallers and non-fallers, and its convergent validity has not yet been demonstrated through expected associations with other measures on reactive balance, gait capacity, muscle strength, and executive and cognitive functioning.
Thus, the aim of this study was to evaluate (1) the discriminant validity of the STT to distinguish between older adult fallers and non-fallers, and (2) the convergent validity of the STT by testing 13 hypotheses on associations with various fall risk factors. We hypothesized that older adults with a fall history would show significant differences in the STT compared to those without. Furthermore, we expected the STT, as a measure of reactive balance, to show moderate positive associations with global (22, 24, 25), static (25, 26), and dynamic (reactive) balance (27, 28), gait capacity (23, 24, 26), functional mobility (22, 25), global cognition (29), and executive functioning (30), as well as low to moderate positive associations with muscle strength (26, 30), and a moderate negative association with fear of falling (24, 26, 31).
2 Methods
2.1 Design
This is a secondary analysis of baseline data from the FEATURE study, a randomized, controlled pilot intervention trial that evaluates the effects of perturbation-based treadmill training on reactive balance in fall-prone older adults. Details about the design, intervention, and primary outcomes of this study have been described previously (32). The FEATURE study was approved by the Ethics Committee of the Medical Faculty Heidelberg (S-602/2022), conducted in accordance with the Helsinki Declaration, and prospectively registered at the German Clinical Trials Register (DRKS00030805). Written informed consent was obtained from all participants prior to study inclusion.
2.2 Participants
Participants were recruited between January and July 2023 from an ambulatory geriatric rehabilitation sports club (REGE e.V.) for older adults, that is associated with a German geriatric hospital. Inclusion criteria were age ≥65 years, increased risk of falling [TUG >12 s and/or usual gait speed <1.0 m/s, and/or fall(s) in past 12 months], and ability to walk ≥2 min without walking aid. Exclusion criteria were cognitive impairment [Mini-Mental State Examination (MMSE) <24 pt.] (33), or severe neurological, cardiovascular, metabolic, and psychiatric disorders.
2.3 Measurements
All measurements were consistently administered by a master’s student in sports science (C.L.), who had received extensive training in interview and test administration to ensure the highest possible standardization and data quality.
2.3.1 Descriptive measures
Age, gender, chronic disease, education, and fall history (≥1 fall in the past 12 months) were assessed by self-reporting. A fall was defined as any “unexpected event in which the participant comes to rest on the ground, floor, or lower level” (34), without considering whether the fall was avoidable or not. Nutritional status was assessed through the body mass index. Cognitive measures included the MMSE (33) for global cognition and the Trail Making Test (TMT) (35) for executive functioning. Subjective health status was assessed using the EQ-5D visual analogue scale (36). Psychological measures comprised the Short FES-I (37) and 5-item Geriatric Depression Scale (GDS-5) (38, 39). Physical frailty was determined according to the criteria of the Fried frailty phenotype (unintentional weight loss, exhaustion, low physical activity, slowness, weakness) (40).
2.3.2 Mobility assessments
Other balance measures included the Brief-BESTest (41) for global balance, the SPPB balance test (3) for static balance, the Four Square Step Test (FSST) (42) for dynamic balance, and the Dynamic Stepping Threshold Test (DSTT) for dynamic reactive balance, which is a modified version of the STT while walking (32). Muscle strength was assessed by a handgrip strength test (JAMAR dynamometer) (43) and the 5-chair stand test (3). Functional mobility was measured with the SPPB (3) and TUG (4), and gait capacity with the 4-m gait speed test (3) and 2-min walk test (2MWT) (44).
2.3.3 Stepping Threshold Test
The STT assesses reactive balance while standing and is executed on a perturbation treadmill that allows for surface translation perturbations in AP and ML directions with increasing magnitude (22). Participants are secured by a harness system and instructed to stand with both feet together, reacting to a maximum of 24 (+1) unannounced perturbations by as few compensatory steps as possible. The test consists of six levels with four perturbations each (forward, backward, left, right) in random order and with gradually increasing magnitudes over the six levels (Supplementary Material). Time intervals between perturbations range from 10 to 19.5 s and are also randomized. Level 4 contains an additional perturbation not considered for the evaluation to maintain the unpredictability of the perturbation direction. The STT total score (8–56 pt.) is the sum of eight single-step and multiple-step thresholds (2 thresholds×4 directions), each defined as the level (1–6) at which a participant requires one step or multiple steps (≥2) to regain balance. The stepping behavior can be evaluated using an all-step count evaluation (STT-ACE) and a direction-sensitive evaluation strategy. For the STT-ACE, each step—defined as an observable change in the bipedal base of support (BoS)—is counted until the participant reaches a static steady-state balance after the perturbation. For the STT-DSE, only steps that result in a sensible extension of the BoS in the opposite direction of the surface translation perturbation are considered (22). Thresholds have to be confirmed in two consecutive levels to be scored while the first of these levels is set as the threshold (45). The STT prematurely is terminated if participants fall or express excessive fear demanding the test to be stopped. If so, missing thresholds are set at one level above the last executed level (22). The STT was performed on the BalanceTutor™ (MediTouch, Netanya, Israel) and video recorded by two cameras (HERO9 Black, GoPro, San Mateo, CA, USA) positioned at about 35° fronto-lateral to the participant and recording at a frame rate of 60 Hz. The video-based assessment of step thresholds in response to surface perturbations during standing has been shown to be inter-observer reliable (Kappa coefficient = 0.89–0.99) (23). Step thresholds for STT scoring were determined from the video recordings by the same rater (N.H.) for consistency, who was not involved in the descriptive measures and mobility assessments.
2.4 Assessment of discriminant and convergent validity
Discriminant validity—defined as the ability of an instrument to differentiate between groups that are known to be different (46)—was evaluated by the discriminative accuracy of the STT-ACE and STT-DSE to distinguish between participants with fall history (fallers) and those without (non-fallers). The discriminative accuracy of other commonly used mobility assessments for fall risk was also examined to provide a preliminary basis for comparison.
Convergent validity—defined as the extent to whether the instrument under study correlates with other instruments to the degree one would expect (47)—was examined through testing 13 hypotheses on expected associations of the STT-ACE and STT-DSE, respectively, with other mobility, psychological and cognitive fall risk factors. The correlation hypotheses are based on existing literature (see introduction). As recommended in guidelines for evaluating psychometric properties (47, 48), convergent validity was considered to be established if ≥75% of the observed associations were consistent with our hypotheses.
2.5 Statistical analysis
Differences between fallers and non-fallers were analyzed using t-tests for independent samples, Mann-Whitney U-tests, and χ2-tests or Fisher's exact tests. Receiver operating characteristic (ROC) and area under the curve (AUC) analyses were performed to determine the accuracy and optimal cutoff points of each mobility assessment in discriminating fallers from non-fallers. AUC values were interpreted as follows: no (AUC ≤0.5), poor (0.5< AUC <0.7), acceptable (0.7≤ AUC <0.8), excellent (0.8≤ AUC <0.9), or outstanding discriminative (AUC ≥0.9) (49). Youden's index [(sensitivity + specificity)–1] was calculated to identify the optimal cutoff point from ROC analyses (50). Spearman rank correlation coefficients (r) were calculated for testing the correlation hypotheses of the STT-ACE and STT-DSE with other mobility, psychological, and cognitive assessments. Coefficients were interpreted as low (r < 0.3), moderate (r = 0.3–0.5), or high (r > 0.5) (51). Scatter plots with regression lines and 95%-confidence interval (CI) bands were also constructed to visualize correlations. Statistical significance was set at p < 0.05. All statistical analyses were performed using IBM SPSS version 29.0 (IBM Corporation, Armonk, NY, USA).
3 Results
3.1 Participant characteristics
Thirty-six community-dwelling older adults (age = 80.3 ± 5.4 years, females: n = 26, 72.2%) were included. One third (n = 13, 36%) reported experiencing at least one fall in the past 12 months (Table 1), with only 4 participants (11%) classified as recurrent fallers (≥2 falls). More than half (n = 19, 53%) were categorized as pre-frail or frail. Gait capacity was mildly impaired, with a mean gait speed of 0.84 ± 0.16 m/s (Table 2), and two-thirds reported at least moderate fear of falling (n = 24, 66.7%). No significant differences in sociodemographic, nutritional, medical, cognitive, psychological, and frailty characteristics were observed between fallers and non-fallers (p = 0.162–0.568; Table 1). Two participants, one with and one without fall history, declined to conduct the STT due to anxiety.
3.2 Discriminant validity
The STT-DSE score differed significantly between fallers and non-fallers (p = 0.033) with acceptable discriminative accuracy [AUC = 0.72, 95%-confidence interval (CI) 0.54–0.91]. The optimal cutoff point for the STT-DSE was 15.5 points, with 96% sensitivity and 42% specificity (Figure 1). No significant differences between fallers and non-fallers (p = 0.086–0.754) and poor discriminative accuracy (AUC = 0.53–0.68, 95%–CI 0.32–0.88) were found for the STT-ACE (AUC = 0.68, 95%–CI 0.49–0.88) and all other mobility assessments (AUC = 0.53–0.65, 95%–CI 0.32–0.85) (Table 2).
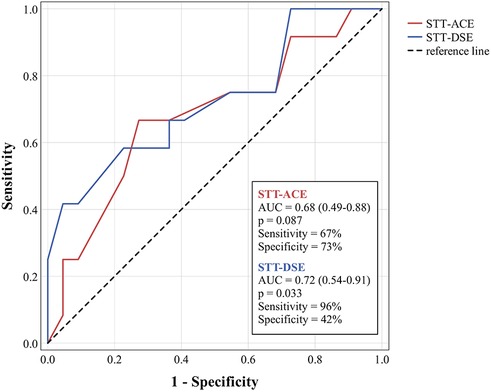
Figure 1. Receiver operating characteristic curves for discriminating fallers and non-fallers using the STT-ACE and STT-DSE.
3.3 Convergent validity
Twelve out of 13 observed associations (92%) with global, static, dynamic and reactive balance, gait capacity, muscle strength, functional mobility, global cognition and executive functioning were consistent with our hypotheses for the STT-DSE (Table 3). The only association for the STT-DSE that was not aligned with our hypotheses was fear of falling. Ten out of 13 observed associations (77%) were consistent for the STT-ACE, with fear of falling, global cognitive, and executive functioning not aligning as expected. High correlations were found for both STT evaluation strategies with global, static, and dynamic reactive balance (BriefBESTest, SPPB balance test, DSTT: r≥0.53), and moderate to high correlations with dynamic balance (FSST: r=–0.37;–0.52), gait capacity (4-m gait speed test, 2MWT: r = 0.38;0.62), and functional mobility (TUG, SPPB: r=|0.34;0.55|). Low correlations were observed for fear of falling (Short FES-I: r≤|0.28|). Correlations with cognitive measures were moderate for the STT-ACE (MMSE, TMT: r≥|0.39|), but low for the STT-DSE (MMSE, TMT: r≤|0.29|). Except for muscle strength (5-chair stand test), the STT-DSE (r=|0.23–0.69|) demonstrated higher correlations than the STT-ACE (r=|0.18–0.58|) with the other assessments. Scatter plots for all correlations are presented in the Supplementary Material.
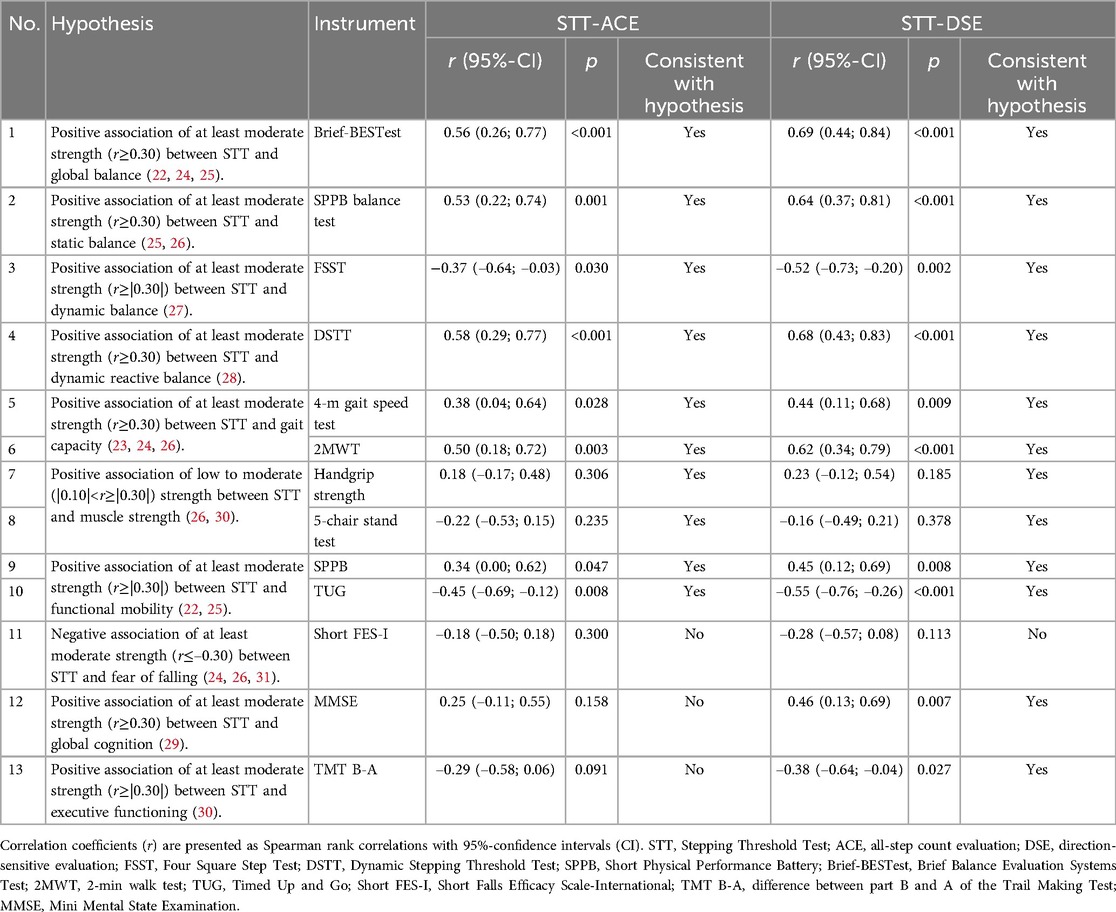
Table 3. Associations of the two evaluation strategies of the Stepping Threshold Test with other assessments of fall risk factors.
4 Discussion
This study evaluated the discriminant and convergent validity of the STT in community-dwelling, fall-prone older adults. To our knowledge, our findings are the first to show acceptable discriminative accuracy of the STT in distinguishing older adult fallers from non-fallers through its DSE strategy, which considers only those compensatory steps in the opposite direction of the perturbation. The ACE strategy of the STT and other commonly used mobility assessments for fall risk showed only poor discriminative accuracy in our sample. Convergent validity of the STT was suggested by 92% of the observed associations for the STT-DSE and 77% for the STT-ACE with various fall risk factors being consistent with our hypotheses, indicating that the STT-DSE might be more valid than the STT-ACE. The results extend previous findings (22) on the convergent validity of the STT in fall-prone older adults by investigating associations with measures of dynamic reactive balance, gait capacity, muscle strength, and cognitive and executive functioning.
4.1 Discriminant validity
The initial STT development and validation study by Adams et al. (22) also investigated its discriminant validity but found no significant differences between fallers and non-fallers using either STT evaluation strategy. In contrast, we found a significantly lower STT-DSE score for fallers, demonstrating acceptable discriminative accuracy (AUC = 0.72) for identifying participants’ fall history. The difference in the STT-ACE came close to the level of significance (p = 0.086) and to the threshold for acceptable discriminative accuracy (AUC = 0.68). The discrepancy to Adams et al. (22) might have resulted from the higher heterogeneity in STT scores [robust coefficient of variance (RCV) = 53%–66%] within our older sample (age = 80 ± 5 years) as opposed to their younger sample (age = 75 ± 6 years; RCV = 21%–33%). Our findings, however, align with those of a meta-analysis on stepping performance and falls in older adults that showed fallers performing worse in reactive step tests (e.g., waist-pulls, tether-releases, slip perturbations) and taking more recovery steps following a perturbation compared to non-fallers (15). These reactive step tests showed moderate sensitivity (73%, 95%–CI 57%–85%), low specificity (59%, 95%–CI 33%–81%), and an acceptable AUC (0.74, 95%–CI 0.47–0.90). Further, more recent original studies confirmed the lower reactive balance ability of older adult fallers (31, 52). Crenshaw et al. (52) performed AP surface perturbations while standing and found that the posterior single-step threshold was lower in fallers, with a significant, but poor discriminative accuracy between fallers and non-fallers (p = 0.049, AUC = 0.62). Batcir et al. (31), who applied surface perturbations in ML direction while standing, also showed that single-step and multiple-step thresholds were lower in fallers.
The STT-DSE seemed to be slightly more accurate compared to the STT-ACE in discriminating fallers from non-fallers in our study. These two evaluation strategies offer different perspectives on stepping behavior. Reactive steps in the opposite direction of the surface perturbation, as considered in the STT-DSE, extend the BoS toward the center of mass (COM) motion to keep the COM within the limits of stability. These (crossover) steps directly contribute to maintaining balance and are effective reactive stepping behaviors for preventing falling, whereas ineffective crossover stepping has been associated with unsuccessful balance recovery in older adults in response to lateral surface perturbations while standing (53). Other steps extending the BoS in different directions, as considered in the STT-ACE, may be rather ineffective and not directly relevant to balance control, or might merely serve to increase standing comfort (22). Thus, the STT-DSE may provide a more accurate mapping of reactive balance ability compared to the STT-ACE. The general assumption that fallers have poorer reactive balance than non-fallers supports our findings that the STT-DSE showed acceptable discriminant validity and might be suitable for evaluating an individual's reactive balance and fall risk. In addition, especially in more frail populations with limited overall physical capacity, the STT-DSE might be more appropriate, as these individuals may take more “ineffective” steps that are not relevant for directly compensating for balance disturbances but rather for improving standing comfort, which are nonetheless considered in the STT-ACE.
Fallers and non-fallers did not show significant differences in other mobility assessments for fall risk, which also consistently demonstrated only poor discriminative accuracy (AUC = 0.53–0.65). This could be attributed to the fact that these assessments do not specifically address common fall mechanisms in real life (e.g., unexpected trips or slips), which may result in lower ecological validity compared to reactive balance measures such as the STT. Notably, previous studies in larger samples of community-dwelling older adults have shown higher discriminative accuracy for some of these mobility assessments (e.g., Brief-BESTest: AUC = 0.76, gait speed test: AUC = 0.69, TUG: AUC = 0.80, FSST: AUC = 0.73) (2, 41, 42). Future studies specifically designed to assess differences in the discriminant validity of the STT compared to such assessments, with larger sample sizes, are needed to further explore our preliminary findings.
Our findings suggest that the STT-DSE can distinguish fallers from non-fallers with high sensitivity (96%) and low specificity (42%). Although having both high sensitivity and high specificity is ideal, a fall risk assessment with high sensitivity but low specificity ensures that those truly at risk of falling are accurately identified. This allows for timely fall prevention measures, even if some individuals with low fall risk are also targeted.
4.2 Convergent validity
Convergent validity of the STT and its two evaluation strategies was investigated by testing hypotheses on associations reported in previous studies between reactive balance or stepping tests and other mobility-related, psychological, and cognitive fall risk factors. More than 75% of these associations were consistent with our hypotheses for both evaluation strategies, suggesting the STT is convergently valid (47, 48).
Adams et al. (22) observed low to moderate correlations of the STT with measures of global balance (Brief-BESTest: STT-ACE: r = 0.41, STT-DSE: r = 0.39), static balance (8-level balance scale: STT-ACE: r = 0.17, STT-DSE: r = 0.25), functional mobility (TUG: STT-ACE: r = –0.38, STT-DSE: r = –0.44), and fear of falling (Short FES-I: STT-ACE: r = –0.11, STT-DSE: r = –0.10) in fall-prone older adults (age = 75 ± 6 years; TUG = 7.8 ± 1.3 s). Other studies including people at a supposedly higher risk of falling, such as stroke patients [mean BBS <45 pt. indicate high fall risk (54)] (23, 24), older women (age = 77 ± 8 years) (26) or older adults (age = 79 ± 5 years) (31), and assisted living residents (age = 82 ± 6 years, TUG = 13.8 ± 5.3 s) (25) reported higher correlations of reactive balance tests with global balance (BBS: r = 0.44–0.69), static balance (unipedal stance test: r = 0.40–0.57), functional mobility: (TUG: r = –0.45, POMA: r = 0.44), and fear of falling (FES-I: r = –0.30 to −0.58). As our population was older (80 ± 5 years) and physically more limited (TUG = 12.6 ± 6.3 s) compared to that of Adams et al. (22), we expected to find also higher correlations between the STT and these fall risk measures. Indeed, we found moderate to high correlations of the STT, irrespective of the evaluation strategy, with the Brief-BESTest, SPPB balance test, TUG, and SPPB (r = |0.34–0.69|), being consistent with our hypothesis that the STT is moderately to highly associated with global and static balance, and functional mobility.
The unexpected low correlation with the Short FES-I may be due to the lack of variance in our sample, with only two participants (6%) reporting high fear of falling and a relatively low RCV (33%) in the Short FES-I. In contrast, Handelzalts et al. (23), who correlated the FES-I with an STT-derived fall threshold (i.e., perturbation magnitude that led to unambiguous support by the harness system) reported high correlations (r = –0.58) in stroke patients. However, more than half of these patients had high fear of falling, and the RCV of the FES-I was three times as large (100%). Likewise, Batcir et al. (31) observed higher correlations between single-step (r = −0.40) and multiple-step (r = −0.30) thresholds to ML surface perturbations in older adults, with a high variance in the FES-I (CV >78%). Our findings are consistent with Adams et al. (22), showing low correlations of the Short FES-I with the STT-ACE (r = –0.11) and STT-DSE (r=–0.10) in fall-prone older adults with similar low variance in the Short FES-I (RCV = 28%).
Werth et al. (27) found a moderate to high correlation (r = 0.36–0.52) between reactive and voluntary stepping responses in a mixed sample of young, middle-aged, and older adults. Accordingly, we hypothesized at least moderate correlations between the STT and the FSST as measure of dynamic balance control during volitional stepping. Correlation coefficients of r=–0.37 for the STT-ACE and r=–0.52 for the STT-DSE with the FSST were consistent with our hypothesis.
Owings et al. (28) reported a moderate correlation (r = 0.36) between static reactive balance, measured by a tether-release test (maximum angle for single-step threshold), and dynamic reactive balance, measured by successful balance recovery during walking (not touching treadmill handles or falling into the harness system) in response to an anterior surface perturbation, in healthy older adults. Based on this finding, we hypothesized that the STT would be at least moderately correlated with the DSTT. Correlations for both the STT-ACE (r = 0.58) and STT-DSE (r = 0.68) aligned with this hypothesis, suggesting even stronger associations. This might be due to the DSTT being a modified version of the STT for measuring reactive balance while walking, with a very similar test protocol that includes perturbations of progressively increasing magnitude and assesses the ability to adapt and learn from repeated perturbations, which has been linked to a lower risk of falling (55, 56).
Higher correlations between the STT and DSTT, compared to the FSST, might be attributed to the fact that reactive balance tests, whether static or dynamic, share more similar control mechanisms with each other than with volitional stepping tests. While both reactive and volitional stepping require fast swing phase and appropriate foot placement, volitional stepping involves more deliberate initiation, unlike the reflexive nature of reactive stepping.
Associations between single-, multiple-step, and/or fall thresholds to surface perturbations while standing and gait capacity measures have been reported to be moderate in community-dwelling older women (7.5-m gait speed test: mean r = 0.30, range 0.24–0.37) (26), and moderate to high (10-m gait speed test: mean r = 0.34, range 0.12–0.55; 6-min walk test: r = 0.60) in stroke patients (23, 24). Thus, we expected at least moderate correlations between the STT and gait capacity also in our study, and the observed correlations for both the STT-ACE and STT-DSE were consistent with this expectation (r = 0.38–0.62).
Lower limb muscle strength has been identified as a predictor of step thresholds to anterior and ML waist pulls while standing in older adults (80 ± 4 years) with low to moderate fall risk (30). In addition, another study in older women reported low to moderate correlations of lower limb muscle strength (r = 0.15–0.36) and handgrip strength (r = 0.20–0.30) with single-step and multiple-step thresholds to AP surface perturbations while standing (26). As hypothesized from these findings, we observed that the STT has only a low correlation with handgrip strength and 5-chair stand test (r=|0.16–0.23|).
Few studies have investigated associations between reactive balance and cognitive functioning in older adults. Kannan and Bhatt (29) indicated that cognitively impaired older adults exhibit a deteriorated reactive standing balance control in response to posterior surface perturbations compared to cognitively intact counterparts. Additionally, Sturnieks et al. (30) identified executive functioning (TMT) as predictor of step thresholds to ML waist pulls while standing in older adults. Based on these findings, we hypothesized that the STT would be at least moderately correlated with measures of global cognition (MMSE) and executive functioning (TMT). Correlations observed for the STT-DSE (MMSE: r = 0.46, TMT: r=–0.39) align with this hypothesis, while those for the STT-ACE (MMSE: r = 0.25, TMT: r=–0.29) did not.
This study has some limitations. First, as this was a secondary analysis of the FEATURE study, the sample size was small and not specifically designed to evaluate the discriminant and convergent validity of the STT, and the hypotheses for testing its convergent validity were not formulated a priori. A sensitivity power analysis was conducted to determine the minimum effect sizes that could be reliably detected by each statistical test used in this study, based on the available sample sizes (Supplementary Material). This analysis revealed that most effect sizes did not meet these thresholds, indicating that some results may be underpowered and need to be interpreted with caution. Future studies with larger sample sizes are required to provide more precise effect size estimates and reduce the risk of type II errors (false negatives), particularly for smaller effect sizes that were consistent with hypotheses in direction and magnitude but did not reach statistical significance. Second, participants were recruited from a geriatric rehabilitation sports club, which may limit the generalizability to the broader older population who may not be regularly engaging in physical exercise. Third, fall history was gathered through self-reporting, which may be subject to recall bias, potentially leading to inaccuracies in the fall history (57). Fourth, as this study was based on a retrospective fall history, the predictive value of the STT cannot be assumed. Future prospective studies are necessary to evaluate the STT's ability to predict future falls.
5 Conclusion
The present study provides preliminary evidence for the discriminant and convergent validity of the STT for measuring reactive balance in fall-prone older adults. When using the STT-DSE strategy, the STT showed an acceptable ability to discriminate between older adult fallers and non-fallers. Convergent validity of the STT was suggested by various associations with other mobility-related, psychological, and cognitive fall risk factors that were consistent with our hypotheses, with the STT-DSE appearing to be more convergently valid than the STT-ACE. The STT seems to be an appropriate tool for assessing reactive balance and fall risk in older adults, with its STT-DSE being recommended due to its better discriminant and convergent validity compared to the STT-ACE. Given the exploratory nature of this secondary analysis study and its limited statistical power, the findings should be interpreted with caution. Future studies specifically designed to evaluate the psychometric properties of the STT, with larger sample sizes, are necessary to confirm these findings.
Data availability statement
The raw data supporting the conclusions of this article will be made available by the authors, without undue reservation.
Ethics statement
The studies involving humans were approved by the Ethics Committee of the Medical Faculty Heidelberg (S- 602/2022). The studies were conducted in accordance with the local legislation and institutional requirements. The participants provided their written informed consent to participate in this study. Written informed consent was obtained from the individual(s) for the publication of any potentially identifiable images or data included in this article.
Author contributions
NH: Methodology, Investigation, Visualization, Writing – original draft, Project administration, Formal Analysis, Data curation, Conceptualization. TB: Investigation, Writing – review & editing. CB: Writing – review & editing, Conceptualization. JMB: Conceptualization, Writing – review & editing, Resources. LHS: Conceptualization, Writing – review & editing. SS: Formal Analysis, Conceptualization, Writing – review & editing, Methodology. CW: Data curation, Writing – review & editing, Supervision, Methodology, Formal Analysis, Conceptualization.
Funding
The author(s) declare financial support was received for the research, authorship, and/or publication of this article. Open access funding was enabled and organized by the project DEAL.
Acknowledgments
We kindly thank Colin Ludwig (CL), Meret Nickel, Carolin Breitwieser, and Lara Daugs for their assistance with screening, recruitment, training, and assessment in the FEATURE study, and all the volunteers for their willingness to participate in the study.
Conflict of interest
The authors declare that the research was conducted in the absence of any commercial or financial relationships that could be construed as a potential conflict of interest.
The author(s) declared that they were an editorial board member of Frontiers, at the time of submission. This had no impact on the peer review process and the final decision.
Publisher's note
All claims expressed in this article are solely those of the authors and do not necessarily represent those of their affiliated organizations, or those of the publisher, the editors and the reviewers. Any product that may be evaluated in this article, or claim that may be made by its manufacturer, is not guaranteed or endorsed by the publisher.
Supplementary material
The Supplementary Material for this article can be found online at: https://www.frontiersin.org/articles/10.3389/fspor.2024.1462177/full#supplementary-material
References
1. Montero-Odasso M, Van Der Velde N, Martin FC, Petrovic M, Tan MP, Ryg J, et al. World guidelines for falls prevention and management for older adults: a global initiative. Age Ageing. (2022) 51(9):afac205. doi: 10.1093/ageing/afac205
2. Jepsen D B, Robinson K, Ogliari G, Montero-Odasso M, Kamkar N, Ryg J, et al. Predicting falls in older adults: an umbrella review of instruments assessing gait, balance, and functional mobility. BMC Geriatr. (2022) 22(1):615. doi: 10.1186/s12877-022-03271-5
3. Guralnik JM, Simonsick EM, Ferrucci L, Glynn RJ, Berkman LF, Blazer DG, et al. A short physical performance battery assessing lower extremity function: association with self-reported disability and prediction of mortality and nursing home admission. J Gerontol. (1994) 49(2):M85–94. doi: 10.1093/geronj/49.2.M85
4. Podsiadlo D, Richardson S. The timed “up & go": a test of basic functional mobility for frail elderly persons. J Am Geriatr Soc. (1991) 39(2):142–8. doi: 10.1111/j.1532-5415.1991.tb01616.x
5. Berg K, Wood-Dauphine S, Williams JI, Gayton D. Measuring balance in the elderly: preliminary development of an instrument. Physiother Can. (1989) 41(6):304–11. doi: 10.3138/ptc.41.6.304
6. Balasubramanian CK, Boyette A, Wludyka P. How well do functional assessments of mobility and balance discriminate fallers and recurrent fallers from non-fallers among ambulatory older adults in the community? Physiother Can. (2015) 67(2):184–93. doi: 10.3138/ptc.2014-19
7. Freiberger E, Fabbietti P, Corsonello A, Lattanzio F, Sieber C, Tap L, et al. Short physical performance battery is not associated with falls and injurious falls in older persons: longitudinal data of the SCOPE project. Eur Geriatr Med. (2024) 15(3):831–42. doi: 10.1007/s41999-024-00941-y
8. Woollacott MH, Shumway-Cook A. Postural dysfunction during standing and walking in children with cerebral palsy: what are the underlying problems and what new therapies might improve balance? Neural Plast. (2005) 12(2-3):211–9. discussion 63-72. doi: 10.1155/NP.2005.211
9. Berg WP, Alessio HM, Mills EM, Tong C. Circumstances and consequences of falls in independent community-dwelling older adults. Age Ageing. (1997) 26(4):261–8. doi: 10.1093/ageing/26.4.261
10. Blake AJ, Morgan K, Bendall MJ, Dallosso H, Ebrahim SB, Arie TH, et al. Falls by elderly people at home: prevalence and associated factors. Age Ageing. (1988) 17(6):365–72. doi: 10.1093/ageing/17.6.365
11. Crenshaw JR, Bernhardt KA, Achenbach SJ, Atkinson EJ, Khosla S, Kaufman KR, et al. The circumstances, orientations, and impact locations of falls in community-dwelling older women. Arch Gerontol Geriatr. (2017) 73:240–7. doi: 10.1016/j.archger.2017.07.011
12. Niino N, Tsuzuku S, Ando F, Shimokata H. Frequencies and circumstances of falls in the national institute for longevity sciences, longitudinal study of aging (NILS-LSA). J Epidemiol. (2000) 10(1 Suppl):S90–4. doi: 10.2188/jea.10.1sup_90
13. Campbell AJ, Borrie MJ, Spears GF, Jackson SL, Brown JS, Fitzgerald JL. Circumstances and consequences of falls experienced by a community population 70 years and over during a prospective study. Age Ageing. (1990) 19(2):136–41. doi: 10.1093/ageing/19.2.136
14. Granacher U, Muehlbauer T, Gruber M. A qualitative review of balance and strength performance in healthy older adults: impact for testing and training. J Aging Res. (2012) 2012:708905. doi: 10.1155/2012/708905
15. Okubo Y, Schoene D, Caetano MJ, Pliner EM, Osuka Y, Toson B, et al. Stepping impairment and falls in older adults: a systematic review and meta-analysis of volitional and reactive step tests. Ageing Res Rev. (2021) 66:101238. doi: 10.1016/j.arr.2020.101238
16. Horak FB, Wrisley DM, Frank J. The balance evaluation systems test (BESTest) to differentiate balance deficits. Phys Ther. (2009) 89(5):484–98. doi: 10.2522/ptj.20080071
17. Tinetti ME. Performance-oriented assessment of mobility problems in elderly patients. J Am Geriatr Soc. (1986) 34(2):119–26. doi: 10.1111/j.1532-5415.1986.tb05480.x
18. Maki BE, McIlroy WE. Control of rapid limb movements for balance recovery: age-related changes and implications for fall prevention. Age Ageing. (2006) 35(Suppl 2):ii12–ii8. doi: 10.1093/ageing/afl078
19. McCrum C, Bhatt TS, Gerards MHG, Karamanidis K, Rogers MW, Lord SR, et al. Perturbation-based balance training: principles, mechanisms and implementation in clinical practice. Front Sports Act Living. (2022) 4:1015394. doi: 10.3389/fspor.2022.1015394
20. Hilliard MJ, Martinez KM, Janssen I, Edwards B, Mille ML, Zhang Y, et al. Lateral balance factors predict future falls in community-living older adults. Arch Phys Med Rehabil. (2008) 89(9):1708–13. doi: 10.1016/j.apmr.2008.01.023
21. Shapiro A, Melzer I. Balance perturbation system to improve balance compensatory responses during walking in old persons. J Neuroeng Rehabil. (2010) 7:32. doi: 10.1186/1743-0003-7-32
22. Adams M, Brüll L, Lohkamp M, Schwenk M. The stepping threshold test for reactive balance: validation of two observer-based evaluation strategies to assess stepping behavior in fall-prone older adults. Front Sports Act Living. (2021) 3:715392. doi: 10.3389/fspor.2021.715392
23. Handelzalts S, Steinberg-Henn F, Soroker N, Schwenk M, Melzer I. Inter-observer reliability and concurrent validity of reactive balance strategies after stroke. Isr Med Assoc J. (2019) 21(12):773–8.31814338
24. Handelzalts S, Gray G, Steinberg-Henn F, Soroker N, Melzer I. Characteristics of proactive balance and gait performance in subacute stroke patients demonstrating varying reactive balance capacity: a research study. NeuroRehabilitation. (2020) 46(4):491–500. doi: 10.3233/NRE-203039
25. Madigan ML, Aviles J, Allin LJ, Nussbaum MA, Alexander NB. A reactive balance rating method that correlates with kinematics after trip-like perturbations on a treadmill and fall risk among residents of older adult congregate housing. J Gerontol A Biol Sci Med Sci. (2018) 73(9):1222–8. doi: 10.1093/gerona/gly077
26. Crenshaw JR, Bernhardt KA, Atkinson EJ, Khosla S, Kaufman KR, Amin S. The relationships between compensatory stepping thresholds and measures of gait, standing postural control, strength, and balance confidence in older women. Gait Posture. (2018) 65:74–80. doi: 10.1016/j.gaitpost.2018.06.117
27. Werth J, König M, Epro G, Seeley J, Potthast W, Karamanidis K. Volitional step execution is an ineffective predictor of recovery performance after sudden balance loss across the age range. Hum Mov Sci. (2021) 76:102769. doi: 10.1016/j.humov.2021.102769
28. Owings TM, Pavol MJ, Foley KT, Grabiner MD. Measures of postural stability are not predictors of recovery from large postural disturbances in healthy older adults. J Am Geriatr Soc. (2000) 48(1):42–50. doi: 10.1111/j.1532-5415.2000.tb03027.x
29. Kannan LN, Bhatt TS. Perturbation-based balance assessment: examining reactive balance control in older adults with mild cognitive impairments. Physiol Int. (2021) 108(3):353–70. doi: 10.1556/2060.2021.00181
30. Sturnieks DL, Menant J, Vanrenterghem J, Delbaere K, Fitzpatrick RC, Lord SR. Sensorimotor and neuropsychological correlates of force perturbations that induce stepping in older adults. Gait Posture. (2012) 36(3):356–60. doi: 10.1016/j.gaitpost.2012.03.007
31. Batcir S, Shani G, Shapiro A, Alexander N, Melzer I. The kinematics and strategies of recovery steps during lateral losses of balance in standing at different perturbation magnitudes in older adults with varying history of falls. BMC Geriatr. (2020) 20(1):249. doi: 10.1186/s12877-020-01650-4
32. Hezel N, Sloot LH, Wanner P, Becker C, Bauer JM, Steib S, et al. Feasibility, effectiveness and acceptability of two perturbation-based treadmill training protocols to improve reactive balance in fall-prone older adults (FEATURE): protocol for a pilot randomised controlled trial. BMJ Open. (2023) 13(9):e073135. doi: 10.1136/bmjopen-2023-073135
33. Folstein MF, Folstein SE, McHugh PR. Mini-mental state”. A practical method for grading the cognitive state of patients for the clinician. J Psychiatr Res. (1975) 12(3):189–98. doi: 10.1016/0022-3956(75)90026-6
34. Hauer K, Lamb SE, Jorstad EC, Todd C, Becker C. Systematic review of definitions and methods of measuring falls in randomised controlled fall prevention trials. Age Ageing. (2006) 35(1):5–10. doi: 10.1093/ageing/afi218
35. Reitan R. TMT, Trail Making Test a & B. South Tucson, AR: Reitan Neuropsychology Laboratory (1992).
36. Hinz A, Kohlmann T, Stöbel-Richter Y, Zenger M, Brähler E. The quality of life questionnaire EQ-5D-5l: psychometric properties and normative values for the general German population. Qual Life Res. (2014) 23(2):443–7. doi: 10.1007/s11136-013-0498-2
37. Hauer KA, Kempen GI, Schwenk M, Yardley L, Beyer N, Todd C, et al. Validity and sensitivity to change of the falls efficacy scales international to assess fear of falling in older adults with and without cognitive impairment. Gerontology. (2011) 57(5):462–72. doi: 10.1159/000320054
38. Gauggel S, Birkner B. Validität und Reliabilität einer deutschen Version der geriatrischen Depressionsskala (GDS). Z Klin Psychol. (1999) 28(1):19–27. doi: 10.1026//0084-5345.28.1.18
39. Hoyl MT, Alessi CA, Harker JO, Josephson KR, Pietruszka FM, Koelfgen M, et al. Development and testing of a five-item version of the geriatric depression scale. J Am Geriatr Soc. (1999) 47(7):873–8. doi: 10.1111/j.1532-5415.1999.tb03848.x
40. Fried LP, Tangen CM, Walston J, Newman AB, Hirsch C, Gottdiener J, et al. Frailty in older adults: evidence for a phenotype. J Gerontol A Biol Sci Med Sci. (2001) 56(3):M146–56. doi: 10.1093/gerona/56.3.M146
41. Marques A, Almeida S, Carvalho J, Cruz J, Oliveira A, Jácome C. Reliability, validity, and ability to identify fall status of the balance evaluation systems test, mini–balance evaluation systems test, and brief–balance evaluation systems test in older people living in the community. Arch Phys Med Rehabil. (2016) 97(12):2166–73.e1. doi: 10.1016/j.apmr.2016.07.011
42. Dite W, Temple VA. A clinical test of stepping and change of direction to identify multiple falling older adults. Arch Phys Med Rehabil. (2002) 83(11):1566–71. doi: 10.1053/apmr.2002.35469
43. Roberts HC, Denison HJ, Martin HJ, Patel HP, Syddall H, Cooper C, et al. A review of the measurement of grip strength in clinical and epidemiological studies: towards a standardised approach. Age Ageing. (2011) 40(4):423–9. doi: 10.1093/ageing/afr051
44. Brooks D, Davis AM, Naglie G. The feasibility of six-minute and two-minute walk tests in in-patient geriatric rehabilitation. Can J Aging. (2007) 26(2):159–62. doi: 10.3138/cja.26.2.009
45. Batcir S, Sharon H, Shani G, Levitsky N, Gimmon Y, Kurz I, et al. The inter-observer reliability and agreement of lateral balance recovery responses in older and younger adults. J Electromyogr Kinesiol. (2018) 40:39–47. doi: 10.1016/j.jelekin.2018.03.002
46. Hattie J, Cooksey RW. Procedures for assessing the validities of tests using the “known-groups” method. Appl Psychol Meas. (1984) 8(3):295–305. doi: 10.1177/014662168400800306
47. Abma IL, Rovers M, van der Wees PJ. Appraising convergent validity of patient-reported outcome measures in systematic reviews: constructing hypotheses and interpreting outcomes. BMC Res Notes. (2016) 9:226. doi: 10.1186/s13104-016-2034-2
48. Terwee CB, Bot SD, de Boer MR, van der Windt DA, Knol DL, Dekker J, et al. Quality criteria were proposed for measurement properties of health status questionnaires. J Clin Epidemiol. (2007) 60(1):34–42. doi: 10.1016/j.jclinepi.2006.03.012
49. Hosmer DW Jr, Lemeshow S, Sturdivant RX. Applied Logistic Regression. John Wiley & Sons (2013). doi: 10.1002/9781118548387
50. Fluss R, Faraggi D, Reiser B. Estimation of the youden Index and its associated cutoff point. Biom J. (2005) 47(4):458–72. doi: 10.1002/bimj.200410135
51. Cohen J. Statistical Power Analysis for the Behavioral Sciences. Routledge (2013). doi: 10.4324/9780203771587
52. Crenshaw JR, Bernhardt KA, Atkinson EJ, Achenbach SJ, Khosla S, Amin S, et al. Posterior single-stepping thresholds are prospectively related to falls in older women. Aging Clin Exp Res. (2020) 32:2507–15. doi: 10.1007/s40520-020-01480-9
53. Batcir S, Berdichevsky Y, Bachner YG, Lubovsky O, Debi R, Melzer I. Characteristics of unsuccessful balance reactive responses to lateral loss of balance in older adults. Gerontology. (2024) 70(7):689–700. doi: 10.1159/000535968
54. Alghadir AH, Al-Eisa ES, Anwer S, Sarkar B. Reliability, validity, and responsiveness of three scales for measuring balance in patients with chronic stroke. BMC Neurol. (2018) 18(1):141. doi: 10.1186/s12883-018-1146-9
55. Gerards MHG, Meijer K, Karamanidis K, Grevendonk L, Hoeks J, Lenssen AF, et al. Adaptability to balance perturbations during walking as a potential marker of falls history in older adults. Front Sports Act Living. (2021) 3:682861. doi: 10.3389/fspor.2021.682861
56. Pai YC, Wang E, Espy DD, Bhatt T. Adaptability to perturbation as a predictor of future falls: a preliminary prospective study. J Geriatr Phys Ther. (2010) 33(2):50–5.20718383
Keywords: reactive balance, postural control, perturbation, falls, risk assessment, older adults, validation study
Citation: Hezel N, Buchner T, Becker C, Bauer JM, Sloot LH, Steib S and Werner C (2024) The Stepping Threshold Test for assessing reactive balance discriminates between older adult fallers and non-fallers. Front. Sports Act. Living 6:1462177. doi: 10.3389/fspor.2024.1462177
Received: 9 July 2024; Accepted: 24 September 2024;
Published: 11 October 2024.
Edited by:
Yoshiro Okubo, Neuroscience Research Australia, AustraliaReviewed by:
Itshak Melzer, Ben-Gurion University of the Negev, IsraelChristopher McCrum, Maastricht University, Netherlands
Copyright: © 2024 Hezel, Buchner, Becker, Bauer, Sloot, Steib and Werner. This is an open-access article distributed under the terms of the Creative Commons Attribution License (CC BY). The use, distribution or reproduction in other forums is permitted, provided the original author(s) and the copyright owner(s) are credited and that the original publication in this journal is cited, in accordance with accepted academic practice. No use, distribution or reproduction is permitted which does not comply with these terms.
*Correspondence: Natalie Hezel, bmF0YWxpZS5oZXplbEBhZ2FwbGVzaW9uLmRl