- 1School of Strength and Conditioning Training, Beijing Sport University, Beijing, China
- 2Sport Training Center, China Institute of Sport Science, Beijing, China
- 3Sports Coaching College, Beijing Sport University, Beijing, China
- 4Competitive Sports Department, Beijing Research Institute of Sports Science, Beijing, China
Objective: The purpose of this systematic review and meta-analysis was to investigates whether transcranial direct current stimulation applied during physical training increases muscle strength in comparison with sham tDCS combined with physical training.
Methods: Randomized controlled trials of the effects of tDCS combined physical training intervention on muscle strength and cortical excitability were collected by searching Web of Science, Pubmed, EBSCO, CNKI. The retrieval date ends in April 2024. 11 randomized controlled trials are finally included. The total sample size of the study is 338. The experimental group was subjected to tDCS combined with physical training intervention, and the control group was physical training combined with sham tDCS intervention.
Results: There is a significant increase in maximal strength (SMD = 0.38; 95% CI: 0.09, 0.67; p = 0.01) and lower limb explosive strength (MD = 2.90; 95% CI: 1.06, 4.74; p = 0.002) when physical training was performed with tDCS, but not following physical training combined with sham tDCS. Subgroup analysis of the subject population showed an increase in muscle strength in those with training experience following tDCS combined with physical training (SMD = 0.39; 95% CI: 0.08, 0.70; p = 0.01), but not for those without training experience (SMD = 0.29; 95% CI: −0.06, 0.63; p = 0.10). Motor evoked potential (MEP) wave amplitude increased significantly following physical training with tDCS (SMD = 0.71; 95% CI: 0.18, 1.24; p = 0.008), but was not different between groups (SMD = 0.16; 95% CI: −0.33, 0.65; p = 0.52).
Conclusions: tDCS combined with physical training intervention can improve muscle strength, lower limb explosive strength and cerebral cortex excitability. Compared to tDCS combined with training of small muscle groups, tDCS combined with training of large muscle groups was more effective in improving muscle strength. Muscle strength was more likely to improve after tDCS combined with physical training in people with physical training experience compared with people without physical training experience. The combination of tDCS with physical training intervention and the sham-tDCS with physical training intervention both increased cortical excitability.
Systematic Review Registration: https://www.crd.york.ac.uk/, PROSPERO, identifier (CRD42024550454).
1 Introduction
Muscle strength represents a critical determinant of athletic performance, with a complex interplay between myogenic and neurogenic factors influencing its development (1). In daily training sessions, coaches mostly start from the myogenic factors, and produce adaptive changes in the skeletal muscle system through appropriate training means (2, 3). From a neurological perspective, cortical excitability is also one of the most important factors affecting muscle strength and plays a pivotal role in the generation of muscle power (4). Neuropriming techniques use neurons as an entry point to increase cortical excitability through stimulation of the brain nerves, strengthening the connection between the brain and the muscles, and thus improving muscle strength (5, 6). Transcranial direct current stimulation (tDCS), as one of the Neuropriming techniques, is a non-invasive brain stimulation technique that generates weak direct current through electrodes placed in the skull (7). It was initially applied in the medical field to treat diseases such as Parkinson's and stroke (8, 9). Given that tDCS has been demonstrated to enhance patients’ motor ability (10–12), as well as the characteristics of portability, noninvasiveness, and easy manipulation (13), it has been used by many researches to explore the stimulation effects on health people and athletes’ performance.
With long-term physical training, the skeletal muscle system may be slow to improve strength due to a ceiling-like effect, so some researchers, in order to explore more effective ways to improve muscle strength, have applied tDCS to physical training, thus exploring whether physical training combined with tDCS can effectively promote muscle strength improvement. However, the effect of brain stimulation combined with physical training as a novel approach to enhancing performance is contingent upon the dosage of tDCS, individual differences, and the training protocol employed. Therefore, findings on the effectiveness of tDCS in combination with physical training have been inconsistent. Hendy et al. (14) indicated that maximum strength of the untrained biceps brachii and cerebral cortex excitability were increased following 2 weeks of physical training combined with 2 mA tDCS. Other studies have also shown that knee extension (15), wrist extension (16), and knee flexion (17) strengths were improved after a period of tDCS combined with physical training intervention compared to the physical training with sham tDCS. However, some studies have shown that the tDCS combined physical training intervention did not improve muscle strength (18), and cortical excitability (19, 20). In conclusion, there is still some controversy regarding the effects of tDCS combined with physical training intervention on muscle strength and cortical excitability, so it is necessary to integrate and analyse this type of research.
The published studies reviews on tDCS have focused exclusively on the effects of single tDCS session on muscle strength in healthy populations. There is, however, a notable absence of reviews and evaluations of the effects of tDCS combined with physical training as concurrent tDCS and training on physical performance (21, 22). Therefore, the purpose of this systematic review and meta-analysis was to investigates transcranial direct current stimulation applied during physical training increases muscle strength in comparison with sham tDCS combined with physical training.
2 Methods
2.1 Search strategy
Keywords used in the search were as follows: (“Transcranial Direct Current Stimulation” OR “tDCS” OR “Transcranial Electrical Stimulations” OR “Transcranial Electrical Stimulation”) AND (“Muscle Strength” OR “Strength Training” OR “physical training” OR “resistance training” OR “strength exercise”). Databases searched included PubMed, EBSCO, CNKI, and Web of Science. The deadline for searching was 18 April 2024. Figure 1 shows the screening process for studies. Taking the web of science database as an example, the specific search strategy is as follows:
#1 “transcranial direct current stimulation"[MeSH Terms] OR (“transcranial"[All Fields] AND “direct"[All Fields] AND “current"[All Fields] AND “stimulation"[All Fields]) OR “transcranial direct current stimulation"[All Fields] OR (“transcranial direct current stimulation"[MeSH Terms] OR (“transcranial"[All Fields] AND “direct"[All Fields] AND “current"[All Fields] AND “stimulation"[All Fields]) OR “transcranial direct current stimulation"[All Fields] OR “tdcs"[All Fields]) OR (“transcranial direct current stimulation"[MeSH Terms] OR (“transcranial"[All Fields] AND “direct"[All Fields] AND “current"[All Fields] AND “stimulation"[All Fields]) OR “transcranial direct current stimulation"[All Fields] OR (“transcranial"[All Fields] AND “electrical"[All Fields] AND “stimulations"[All Fields]) OR “transcranial electrical stimulations"[All Fields]) OR (“transcranial direct current stimulation"[MeSH Terms] OR (“transcranial"[All Fields] AND “direct"[All Fields] AND “current"[All Fields] AND “stimulation"[All Fields]) OR “transcranial direct current stimulation"[All Fields] OR (“transcranial"[All Fields] AND “electrical"[All Fields] AND “stimulation"[All Fields]) OR “transcranial electrical stimulation"[All Fields])
#2 “muscle strength"[MeSH Terms] OR (“muscle"[All Fields] AND “strength"[All Fields]) OR “muscle strength"[All Fields] OR (“resistance training"[MeSH Terms] OR (“resistance"[All Fields] AND “training"[All Fields]) OR “resistance training"[All Fields] OR (“strength"[All Fields] AND “training"[All Fields]) OR “strength training"[All Fields]) OR ((“physical examination"[MeSH Terms] OR (“physical"[All Fields] AND “examination"[All Fields]) OR “physical examination"[All Fields] OR “physical"[All Fields] OR “physically"[All Fields] OR “physicals"[All Fields]) AND (“education"[MeSH Subheading] OR “education"[All Fields] OR “training"[All Fields] OR “education"[MeSH Terms] OR “train"[All Fields] OR “train s"[All Fields] OR “trained"[All Fields] OR “training s"[All Fields] OR “trainings"[All Fields] OR “trains"[All Fields])) OR (“resistance training"[MeSH Terms] OR (“resistance"[All Fields] AND “training"[All Fields]) OR “resistance training"[All Fields]) OR ((“strength"[All Fields] OR “strengths"[All Fields]) AND (“exercise"[MeSH Terms] OR “exercise"[All Fields] OR “exercises"[All Fields] OR “exercise therapy"[MeSH Terms] OR (“exercise"[All Fields] AND “therapy"[All Fields]) OR “exercise therapy"[All Fields] OR “exercising"[All Fields] OR “exercise s"[All Fields] OR “exercised"[All Fields] OR “exerciser"[All Fields] OR “exercisers"[All Fields]))
#3 (#1 AND #2)
2.2 Inclusion and exclusion criteria
Determination of study inclusion criteria based on the PICOS principle (23). Studies were included for review if they fulfilled the following selection criteria: (a) Intervention and control measures: experimental group received tDCS stimulation and training intervention, control group received sham tDCS plus training intervention; (b) Outcome measures: metrics related to maximal strength and explosive strength, measures related to cortical excitability (motor-evoked potentials); (c) Study design: the included studies were randomized controlled trials or self-controlled experiments.
The exclusion criteria were as follows: (a) Participants were patients, the elderly, or athletes; (b) No experimental data; (c) Repeatedly published literature; and (d) animal experiments.
2.3 Data extraction
The following data, presented as Microsoft Word files (Microsoft Corp.), were extracted from the included studies: (a) leader author, year of publication; (b) descriptive information of the sample including sample size and age; (c) tDCS protocols: electrode positioning and dosage; (d) training protocols: duration, intensity and frequency; (d) the methodology of strength and MEP assessment. Data extraction was carried out by 3 authors (Liu Jintong, Zhao Fan and Li Chunlei) and was cross-checked between them, with any discrepancies resolved by discussion and consensus.
2.4 Quality assessment
The Cochrane Risk Assessment Tool for assessing risk of bias was used to assess the risk of bias of the included randomized clinical trials. Assessment with this tool includes six domains: selection bias, performance bias, detection bias, attrition bias, reporting bias, and other bias. Each domain can be scored as: low risk of bias, some concerns, or high risk of bias. The study search, data extraction and quality assessment were carried out by two independent reviewers (Liu and Li), with a third reviewer involved in case of disagreement (Zhao).
2.5 Statistical analyses
This meta-analysis compared the effects of sham tDCS combined with physical training vs. tDCS in combination with physical training on maximal muscular strength, vertical jump and cortical excitability. Since the muscle strength and cortical excitability performance data in this study were continuous variables with inconsistent testing units, standardized mean difference (SMD) was used, whereas the vertical jump performance data were consistent with the testing units and were continuous variables, so they were expressed by mean difference (MD). Effect sizes were defined by the Cochrane Handbook, with SMD(MD) < 0.5 as a small effect size, 0.5 ≤ SMD(MD) < 0.8 as a medium effect size, and SMD(MD) ≥ 0.8 as a large effect size (24). Heterogeneity was explored using the I2 statistic. When I2 = 0, it indicated that there was no heterogeneity between studies, when I2 < 50%, it indicated that there was a low degree of heterogeneity between studies, and at this time, a fixed effect model was selected for analysis, when I2 ≥ 50%, a random effect model needed to be selected for analysis. Publication bias was visually assessed by creating funnel plots using Review Manager version 5.4 and by conducting Egger's regression tests on the results of studies that included 10 or more studies using Stata version 16.0. Statistical significance was determined at p < 0.05. Review Manager 5.4 was used for all analyses.
3 Results
3.1 Search results
758 studies were found after searching and 598 relevant studies were obtained after removing duplicate studies in EndNote X9.3.3 software. 62 studies were selected after reading the titles and abstracts, which were rescreened by reading the full text. Only 11 studies were included in this systematic review and meta-analysis after rereading the full texts (Figure 1).
3.2 Study characteristics
The included studies were published between 2013 and 2024; the participants included in the study were all healthy (age: 23.55 ± 2.54), with a total sample size of 338, totaling 166 in the experimental group and 172 in the control group. The control group was physical training intervention only and the experimental group was physical training combined with tDCS intervention. Information on the training program, the dosage of the tDCS were also included in the study. Table 1 shows the characteristics of the included studies.
3.3 Methodological quality and publication bias
A total of 11 papers were included, of which 11 described the randomisation methodology, 3 described the hidden allocation scheme, 7 described the specific blinding methodology, and 2 described the blinding methodology for outcome assessment. The data results were complete for all the included literature. In summary, 7 articles were of high quality, 2 articles were rated as medium risk, and 2 articles were rated as high risk. The results were presented in a traffic light diagram (Figure 2).
Considering that there must be at least ten studies to assess publication bias, publication bias could not be assessed for lower limb explosive strength and cortical excitability due to the small number of studies (≤10). In addition, no evidence of publication bias was observed through visual evaluation of funnel plots and Egger's test results for maximal strength (p = 0.362) (Figure 3).
3.4 Meta-analysis results
3.4.1 Maximal strength
Eight studies from the included studies reported the effects of tDCS in combination with physical training intervention on muscle strength. As the test for heterogeneity showed low heterogeneity (p = 0.14, I2 = 32%), and I2 < 50%, we used the fixed-effects model. Based on the meta-analysis, tDCS combined with physical training intervention was significantly more effective than physical training intervention alone in improving muscle strength, and the improvement of muscle strength reached the level of small effect size (SMD = 0.38, 95% CI: 0.09, 0.67, p = 0.01) (Figure 4).
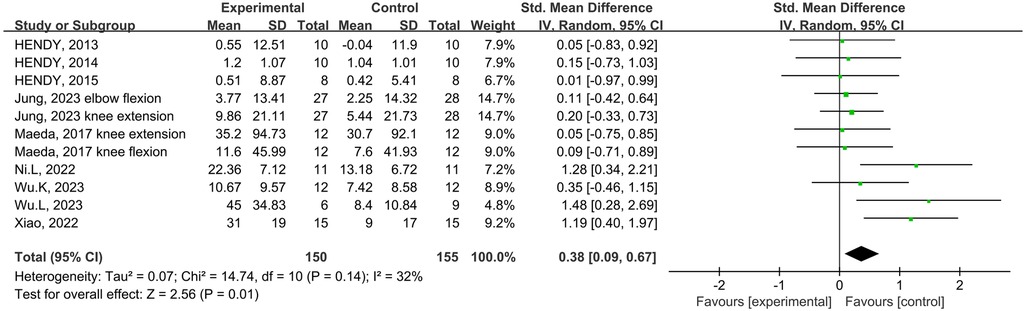
Figure 4. Meta-analyses of the effects of tDCS combined with physical training interventions on muscular strength.
Subgroup analysis of the subject population showed an increase in muscle strength in those with training experience following tDCS combined physical training (SMD = 0.39; 95% CI: 0.08, 0.70; p = 0.01), but not for those without training experience (SMD = 0.29; 95% CI: −0.06, 0.63; p = 0.10) (Figure 5). Subgroup analysis for muscle group showed muscle strength enhancement in large muscle group following tDCS combined physical training (SMD = 0.39; 95% CI: 0.08, 0.71; p = 0.01), but not for small muscle group (SMD = 0.29; 95% CI: −0.04, 0.63; p = 0.09) (Figure 6).
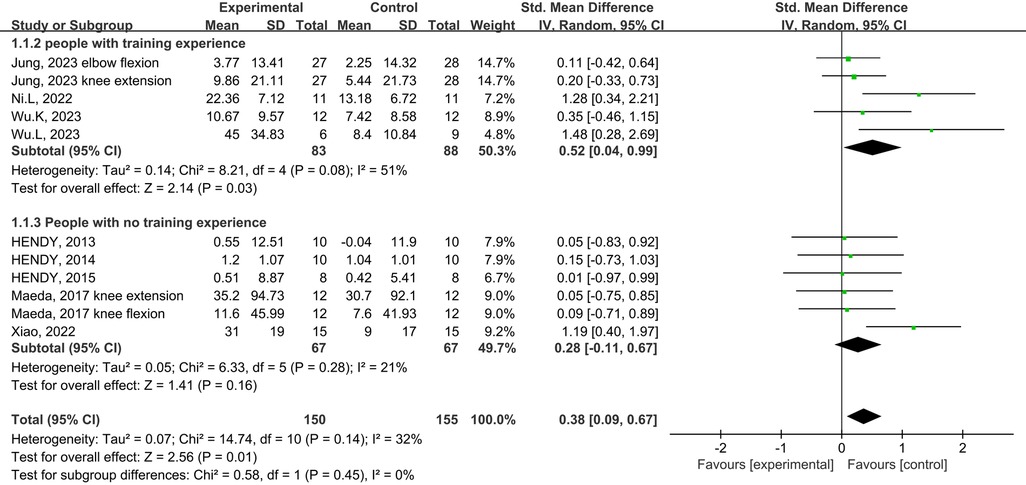
Figure 5. Subgroup analysis of the effects of tDCS combined with physical training intervention on muscle strength in people with and without training experience.
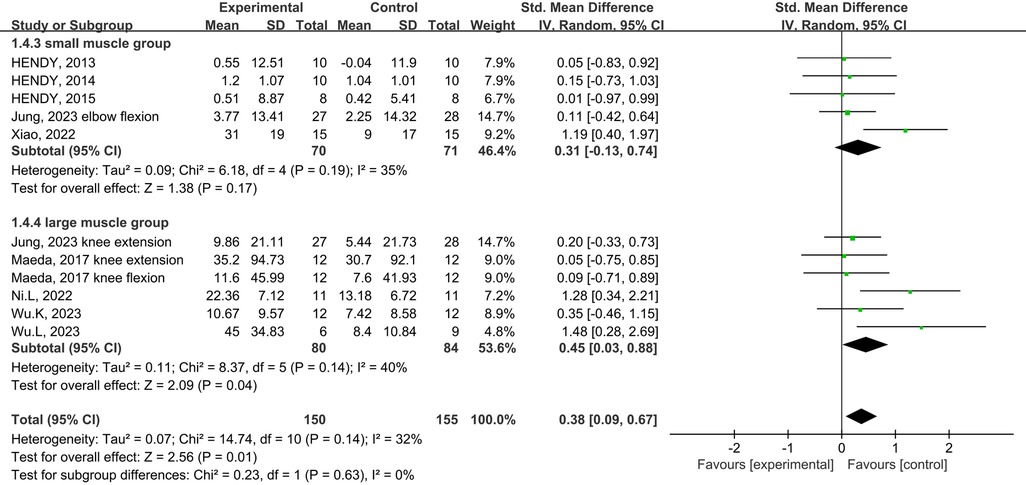
Figure 6. Subgroup analysis of the effects of tDCS combined with physical training intervention for different muscle groups on muscle strength.
3.4.2 Lower limb explosive strength
A total of four studies from the included studies reported the effects of tDCS combined with physical training intervention on lower limb explosive strength. As the test for heterogeneity showed no heterogeneity (p = 0.40, I2 = 0%), and I2 ≥ 0%, we used the fixed-effects model for the effect size test. Based on the meta-analysis, tDCS combined with the physical training intervention was significantly more effective than physical training intervention alone in improving lower limb explosive strength, and the improvement of explosive strength reached the level of large effect size (MD = 2.90; 95% CI: 1.06, 4.74; p = 0.002) (Figure 7).
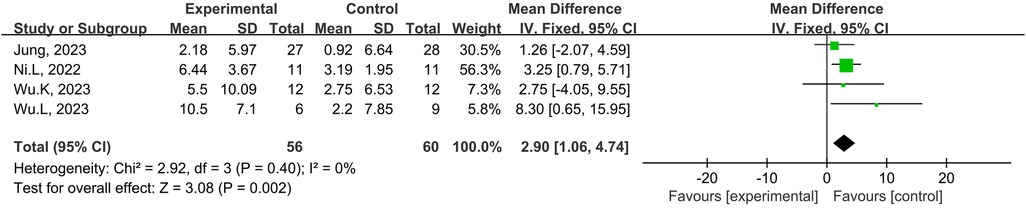
Figure 7. Meta-analyses of the effects of tDCS combined with physical training intervention on lower limb power.
3.4.3 Cortical excitability
A total of four studies from the included studies reported the effects of tDCS in combination with physical training intervention on cortical excitability. Firstly, effect sizes were combined for within-group MEP wave amplitude, and the test for heterogeneity resulted in a low degree of heterogeneity (p = 0.003, I2 = 32%), with I2 ≤ 50%, so we used the fixed-effects model for the effect size test. Secondly, effect sizes were combined for MEP wave amplitude between groups, and the heterogeneity test results were low degree of heterogeneity (p = 0.12, I2 = 49%), and I2 ≤ 50%, so effect sizes were tested using the fixed effect model. Meta-analysis results showed that tDCS combined with physical training intervention, although it could increase cortical excitability (SMD = 0.71; 95% CI: 0.18, 1.24; p = 0.008) (Figure 8), but there was no statistical difference between the two groups compared to the control group (SMD = 0.16; 95% CI: −0.33, 0.65; p = 0.52) (Figure 9).

Figure 8. Meta-analyses of the effects of within-group tDCS combined with physical training intervention on cortical excitability.

Figure 9. Meta-analyses of the effects of inter-group tDCS combined with physical training intervention on cortical excitability.
4 Discussion
This is the first systematic review explored the effects of physical training combined with tDCS on measures of muscle strength and cortical excitability. Results indicate that when physical training combined with tDCS, maximal strength and lower limb explosive strength could be significantly improved. Moreover, our results showed that there was a significant increase in the amplitude of MEP following the stimulation of the primary motor area of cerebral cortex with either tDCS combined with physical training or sham tDCS combined with physical training.
Muscle strength is influenced not only by myogenic factors but also by neural factors. The highest level structure controlling human movement is the motor area of the cerebral cortex, including the primary motor cortex, premotor cortex and supplementary motor cortex. Among them, the primary motor cortex is the most important part of the motor system, and the motor control areas are arranged in reverse order, with those controlling the lower limbs at the top of the cerebral hemispheres and those controlling the face located near the central sulcus, whose motor commands are directed to the motor neurons and interneurons in the spinal cord via the corticospinal tracts or the cortical brainstem tracts, which in turn control the muscular activities of the limbs and trunk (32). The central nervous system regulates muscle strength by elevating motor unit discharge frequency and motor unit synchronization (33). Firstly, the higher the central nervous system excitability, the higher the neuron firing frequency, which causes the muscle to start the next contraction before the previous contraction has finished, and the muscle single contractions are superimposed, resulting in an increase in muscle contraction tension (34). Secondly, the stronger the excitability of the central nervous system, the higher the degree of synchronization of motor unit initiation, so that more neurons produce excitation at the same time, sending out higher frequency and more concentrated nerve impulses, so that some less excitable motor units are involved in muscle contraction, thus increasing muscle strength (35).
tDCS is used as a brain modulation technique to alter the excitability of the cerebral cortex by applying lower electrical currents to specific areas of the brain for a period of time (36). It is important to note that tDCS does not produce potentiation changes, but rather depolarizes cell membranes by current stimulation. From a molecular perspective, tDCS regulates the opening of ion channels through voltage. In the resting state, the extramembrane Na+ concentration is significantly higher than the intramembrane. Upon stimulation, the Na+ channels are opened, allowing the ions to enter the intracellular membrane along the concentration gradient. Once the concentration reaches a certain threshold, a transient positive potential is generated (37). Meanwhile, it has also been shown that tDCS can change neuronal non-synaptic excitability by altering the intracellular Ca+ concentration in the brain, which in turn improves central nerve impulses (38). Some researchers have monitored changes in cortical excitability, and the results showed an increase in MEP wave amplitude and cortical excitation following tDCS (39). Increased cortical excitability allows more motor neurons to be involved in excitation, which increases muscle recruitment. In addition, tDCS can also indirectly improve brain function by increasing cerebral blood flow (40), modulating synaptic plasticity (41), and neural network connectivity (42), which in turn alters the excitability of spinal motor neurons and improves muscle strength.
In terms of improving muscle strength, it has been shown in many studies a single tDCS can result in an acute improvement in muscle strength. Kenville et al. (43) showed a significant increase in maximal isometric squat strength after a single tDCS in 25 healthy adults. Anoushiravani et al. (44) came to the same conclusions. However, these studies only explored the effect of a single acute tDCS on muscle strength and did not include combined tDCS physical training as an intervention. It is important to note that strength training can also alter brain function. It has been shown that after training at a certain intensity, cortical MEP amplitude increases (45), accompanied by a decrease in short-interval intracortical inhibition (SICI) (46). tDCS has a similar effect on changes in brain function, and when the two are combined, it is unclear whether the effects on the brain and even motor performance are enhanced or counteracted when the two are combined. Based on this some researchers have combined tDCS stimulation with physical training to investigate its effects on muscle strength, however the results have been mixed. In the present study, relevant literature was pooled and meta-analysed, and according to the results, tDCS in combination with physical training intervention improved muscle strength more than physical training intervention alone. Wu Li (29) showed that tDCS combined with weighted semi-squat jump training was effective in improving muscle strength in healthy college students. Xiao et al. (26) demonstrated that after four weeks of tDCS in combination with foot core strength training, metatarsal flexor strength as well as improvement of proprioceptive function of the ankle in healthy populations could be significantly improved. It is thought that this phenomenon is related to alterations in synaptic plasticity (47, 48). Generally, tDCS induces immediate effects by changing neurons’ resting membrane potentials, which usually dissipate after an hour (49). In this study, which is indexed by the power of selection, the duration of tDCS stimulation was more than two weeks. tDCS repetitive stimulation provides a cumulative and more lasting effect (25), which is closely related to the rise in the expression of N-methyl-D-aspartate (NMDA) and α-amino-3-hydroxy-5-methyl-4-isoxazolepropionic acid (AMPA) at glutamate-sensitive receptors in the postsynaptic membrane relationship, with elevated NMDA and AMPA expression increasing sensitivity to glutamate thereby triggering postsynaptic potentials (50). In addition, synaptic efficacy may also be affected by changes in astrocytic calcium ion concentrations (51).
However, muscle strength after tDCS stimulation in combination with physical training interventions did not differ significantly between groups, but pre- and post-tests within the groups changed significantly. A study by Jung et al. (15) found that the maximum isometric strength of the elbow flexors and knee extensors in the experimental group changed significantly after tDCS combined with physical training interventions as compared to the baseline level, while at the same time, the muscle strength in the control group also increased. So the increase in muscle strength between the groups was not statistically significant. Maeda et al. (17) performed centrifugal contraction training of knee extension and knee flexion with combined tDCS stimulation on 24 healthy adults for 3 weeks, and results showed that both experimental and control groups increased muscle strength in knee extension and knee flexion, but that there was no significant difference between groups in muscle strength. It has been explained that the slightly longer interval between tDCS stimulation three times per week was not enough to affect synaptic plasticity in the motor cortex, and that increasing the number of tDCS interventions per week might have a better effect (15, 52). Liu et al. (53) administered tDCS intervention five times per week to professional rowers, and the athletic performance significantly improved after two weeks of the intervention. Similarly it has been demonstrated that daily tDCS stimulation is more likely to alter cortical excitability than spaced stimulation (52). This may be attributed to a direct enhancement of protein synthesis by tDCS during the training period, or alternatively, to a downstream interaction of its excitatory effects with exercise-related protein synthesis during and after the training period (54).
Subgroup analyses found that tDCS in combination with large muscle group training improved muscle strength more than small muscle groups. Hendy and Kidgell (25) performed tDCS stimulation combined with weighted dumbbell wrist extension training on 30 participants over a three-week period, and the results showed that neither experimental nor control groups showed significantly different changes in muscle strength. Similar results have also been obtained by combining tDCS stimulation with biceps dumbbell curl training. However, when tDCS stimulation was combined with large muscle group training, the training effect of the combined intervention was significantly better than that of the training group alone (16, 17, 30). These results suggest that training of different muscle groups may influence the stimulation effect of tDCS. It is possible that compared to small muscles, large muscles possess more potential to improve muscle strength (55). Specifically, Larger muscles possess a larger cross-sectional area and contain a greater number of motor neurons than smaller muscles. However, not all motor neurons are recruited during muscle contraction; rather, only a subset of them is engaged. Furthermore, the number of unrecruited neurons is greater in larger muscle groups than in smaller ones, suggesting that the potential for increased muscle strength is greater in the former (56). tDCS engages a greater number of unrecruited motor units in muscle contraction, resulting in a greater force output (37).
The results of another subgroup analysis showed that muscle strength was more likely to be improved after the tDCS combined physical training intervention in people with training experience compared to people without training experience. This may be due to the fact that people with training experience have better movement stability and high stability of the test movements when performing the pre and post-tests of the experiment due to regular training, i.e., there are fewer additional variables in the experiment and more net intervention efficacy induced by the experiment (57). However, it has also been shown that after tDCS training alone, there is a higher potential for improvement in muscle strength in diseased populations (58, 59) compared to healthy populations (60, 61) due to the fact that diseased populations such as stroke patients may have a greater potential for strength enhancement due to factors such as decreased cortical excitability, and blocked neural pathway conduction. Therefore, future research on tDCS could focus more on athletes or people with good athletic experiences to investigate whether the “ceiling” effect may affect performance enhancement.
The vertical jump is one of the most common indicators of lower limb explosive strength (62). In this study, the relevant literature was pooled and Meta-analyzed, and the results showed that tDCS in combination with physical training improved lower limb explosive strength in a healthy population more than physical training intervention alone. Ni (30) found a significant increase in vertical jump height after six-week tDCS stimulation in combination with squat training intervention in 33 healthy male college students (30). Many foreign studies have confirmed the significant effect of tDCS on lower limb explosive strength enhancement (44, 63, 64). tDCS combined with physical training intervention to improve the physiological mechanism of explosive strength is currently unknown. It has been shown that an increase in the frequency of spinal motor neuron impulse delivery at the beginning of muscle contraction is the main reason for the elevated rate of training-induced contraction force generation (RFD) (65). tDCS stimulation increases cortical excitability, which in turn increases the downward nerve impulses from cortical spinal tract fibers to control the activity of spinal motor neurons innervating skeletal muscles. Therefore, increasing the frequency of spinal motor neuron impulse delivery through the combined effect of tDCS stimulation as well as training may be one reason for inducing increased explosive power. Another reason could be due to the decrease in SICI that promotes muscle strength. Weier et al. (46) found that after several weeks of intense strength training, muscle strength increased while SICI continued to decrease. This is consistent with changes in SICI after tDCS stimulation (66). Additionally, a correlation between enhanced motor performance and increased corticospinal excitability and decreased SICI following tDCS intervention has been demonstrated (67). Hendy and Kidgell (16) concluded that SICI was significantly reduced only when strength exercise was combined with the tDCS condition. In addition to exploring the starting point from a neural perspective, it has also been hypothesised that tDCS increases explosive power output per second by improving the integration efficiency of the energy supply system (68).
Motion-evoked potential (MEP) is the potential recorded on the surface of the corresponding muscles or nerves when the excitation generated by the application of electrical or magnetic stimulation to cortical motor areas depolarizes the anterior horn cells of the spinal cord or peripheral neuromotor fibres through downward conduction pathways (69), and it is one of the most important indexes used to reflect the changes in the excitability of the corticospinal nerve bundles. Meta-analysis of the present study showed that there was no between-group difference in the effect of tDCS combined with physical training intervention on MEP amplitude, but MEP amplitude was significantly increased within the group.
After tDCS in combination with biceps curl training, Hendy et al. (28) reported the same findings, but the differences between groups were not statistically significant when compared to the physical training intervention group. This suggests that unilateral strength training-induced cortical plasticity combined with tDCS-induced plasticity has complementary effects on neuromodulation of motor pathways controlling the inactive limb. Kim et al. (27) found that not only was MEP wave amplitude significantly increased after tDCS combined with an physical training intervention in participants, but there was also a statistically significant between-group difference when compared to the control group. However, Summers et al. (19) study came up with the opposite result, after performing tDCS stimulation combined with finger tracking training on 14 participants, MEP amplitude decreased in the tDCS combined physical training intervention group while MEP amplitude increased in the physical training only intervention, probably because tDCS intervention along with strength training causes a disruptive effect on cortical excitability. As a result, fewer studies have examined tDCS's effects on cortical excitability in combination with physical training, and the results can differ considerably depending on the area stimulated and the training intervention. It would therefore be beneficial for future studies to examine the effects of tDCS in combination with physical training on the excitability of the cortex.
5 Limitations and prospects
Research on tDCS combined with physical training intervention has focused on healthy people, and few studies have focused on athletes. tDCS stimulation frequency and duration, as well as the program of the physical training will affect the final performance of the physical training, and there is almost no research in this area. Different tDCS stimulation devices have different effects on the results of the study. tDCS stimulation devices available on the market include the Halo sport, HD-tDCS, and sponge tDCS. It is suggested that future research on tDCS could be conducted from the perspectives of the study population, the program of the training, and the stimulation device.
(1) Study population: The selection of participants is not only limited to the healthy people, but also can be spread to athletes, to further explore whether the tDCS combined physical training intervention will have a “ceiling” effect due to prolonged training adaptations. (2) Training protocal: Different training contents and different load choices will have different effects on neural stimulation, and different weekly training frequencies will have different effects on the cerebral cortex, therefore, the content of the physical training interventions, the frequency of the training, and the selection of the load intensity are all issues that deserve to be further explored in depth. (3) Selection of the type of tDCS stimulation instrument: Different types of instruments have different strengths and weaknesses. Sponge electrode tDCS stimulation is less accurate and may induce changes in the excitability of neighboring areas of the cerebral cortex, making the results inaccurate. High-definition tDCS (HD-tDCS) can accurately localise the corresponding areas of the cerebral cortex, and the stimulation range is more precise. Halo sport is more convenient to wear than the former two, but the stimulation accuracy may be lower. Therefore, the choice of tDCS stimulation devices can be diversified in the future.
6 Conclusions
Compared with conventional physical training, tDCS combined with physical training intervention can more effectively improve muscle strength, lower limb explosive power. More specifically, compared to tDCS combined with training of small muscle groups, tDCS combined with training of large muscle groups was more effective in improving muscle strength. People with training experience are more likely to improve muscle strength after tDCS than those without training experience. In addition, the combination of tDCS with physical training and sham tDCS with physical training both resulted in increased cortical excitability.
Data availability statement
The original contributions presented in the study are included in the article/Supplementary Material, further inquiries can be directed to the corresponding author.
Author contributions
JL: Conceptualization, Data curation, Formal Analysis, Funding acquisition, Investigation, Methodology, Project administration, Resources, Software, Supervision, Validation, Visualization, Writing – original draft, Writing – review & editing. CL: Conceptualization, Data curation, Formal Analysis, Investigation, Methodology, Project administration, Software, Supervision, Writing – review & editing. JF: Conceptualization, Data curation, Funding acquisition, Investigation, Project administration, Software, Validation, Writing – review & editing. HX: Formal Analysis, Investigation, Methodology, Project administration, Writing – review & editing. XZ: Methodology, Project administration, Supervision, Validation, Writing – review & editing. FZ: Conceptualization, Formal Analysis, Supervision, Writing – review & editing.
Funding
The author(s) declare that no financial support was received for the research, authorship, and/or publication of this article.
Conflict of interest
The authors declare that the research was conducted in the absence of any commercial or financial relationships that could be construed as a potential conflict of interest.
Publisher's note
All claims expressed in this article are solely those of the authors and do not necessarily represent those of their affiliated organizations, or those of the publisher, the editors and the reviewers. Any product that may be evaluated in this article, or claim that may be made by its manufacturer, is not guaranteed or endorsed by the publisher.
Supplementary material
The Supplementary Material for this article can be found online at: https://www.frontiersin.org/articles/10.3389/fspor.2024.1446588/full#supplementary-material
References
1. Suchomel TJ, Nimphius S, Bellon CR, Stone MH. The importance of muscular strength: training considerations. Sports Med. (2018) 48(4):765–85. doi: 10.1007/s40279-018-0862-z
2. Luckin KM, Badenhorst CE, Cripps AJ, Landers GJ, Merrells RJ, Bulsara MK, et al. Strength training in long-distance triathletes: barriers and characteristics. J Strength Cond Res. (2021) 35(2):495–502. doi: 10.1519/JSC.0000000000002716
3. Ronnestad BR, Hansen EA, Raastad T. High volume of endurance training impairs adaptations to 12 weeks of strength training in well-trained endurance athletes. Eur J Appl Physiol. (2012) 112(4):1457–66. doi: 10.1007/s00421-011-2112-z
4. Gomez-Feria J, Martin-Rodriguez JF, Mir P. Corticospinal adaptations following resistance training and its relationship with strength: a systematic review and multivariate meta-analysis. Neurosci Biobehav Rev. (2023) 152(4):367–491. doi: 10.1016/j.neubiorev.2023.105289
5. Bortoletto M, Pellicciari MC, Rodella C, Miniussi C. The interaction with task-induced activity is more important than polarization: a tDCS study. Brain Stimul. (2015) 8(2):269–76. doi: 10.1016/j.brs.2014.11.006
6. Stagg CJ, Nitsche MA. Physiological basis of transcranial direct current stimulation. Neuroscientist. (2011) 17(1):37–53. doi: 10.1177/1073858410386614
7. Edwards DJ, Cortes M, Wortman-Jutt S, Putrino D, Bikson M, Thickbroom G, et al. Transcranial direct current stimulation and sports performance. Front Hum Neurosci. (2017) 11(2):351–92. doi: 10.3389/fnhum.2017.00243
8. Beretta VS, Conceicao NR, Nobrega-Sousa P, Orcioli-Silva D, Braz Fonseca Dantas LK, Bucken Gobbi LT, et al. Transcranial direct current stimulation combined with physical or cognitive training in people with Parkinson’s disease: a systematic review. J Neuroeng Rehabil. (2020) 17(1)46–61. doi: 10.1186/s12984-020-00701-6
9. Biundo R, Weis L, Antonini A. tDCS effect on cognitive performance in Parkinson’s disease. Mov Disord. (2016) 31(8):1253. doi: 10.1002/mds.26685
10. Bruce AS, Howard JS, Van Werkhoven H, McBride JM, Needle AR. The effects of transcranial direct current stimulation on chronic ankle instability. Med Sci Sports Exercise. (2020) 52(2):335–44. doi: 10.1249/MSS.0000000000002129
11. Baroni A, Magro G, Martinuzzi C, Brondi L, Masiero S, Milani G, et al. Combined effects of cerebellar tDCS and task-oriented circuit training in people with multiple sclerosis: a pilot randomized control trial. Restor Neurol Neurosci. (2022) 40(2):85–95. doi: 10.3233/RNN-211245
12. Lefaucheur J-P, Antal A, Ayache SS, Benninger DH, Brunelin J, Cogiamanian F, et al. Evidence-based guidelines on the therapeutic use of transcranial direct current stimulation (tDCS). Clin Neurophysiol. (2017) 128(1):56–92. doi: 10.1016/j.clinph.2016.10.087
13. Brunoni AR, Nitsche MA, Bolognini N, Bikson M, Wagner T, Merabet L, et al. Clinical research with transcranial direct current stimulation (tDCS): challenges and future directions. Brain Stimul. (2012) 5(3):175–95. doi: 10.1016/j.brs.2011.03.002
14. Hendy AM, Teo WP, Kidgell DJ. Anodal tDCS prolongs the cross-education of strength and corticospinal plasticity. Int J Stroke. (2015) 10:63. doi: 10.1111/ijs.12634_27
15. Jung J, Salazar Fajardo JC, Kim S, Kim B, Oh S, Yoon B. Effect of tDCS combined with physical training on physical performance in a healthy population. Res Q Exerc Sport. (2024) 95(1):149–56. doi: 10.1080/02701367.2023.2166894
16. Hendy AM, Kidgell DJ. Anodal-tDCS applied during unilateral strength training increases strength and corticospinal excitability in the untrained homologous muscle. Exp Brain Res. (2014) 232(10):3243–52. doi: 10.1007/s00221-014-4016-8
17. Maeda K, Yamaguchi T, Tatemoto T, Kondo K, Otaka Y, Tanaka S. Transcranial direct current stimulation does not affect lower extremity muscle strength training in healthy individuals: a triple-blind, sham-controlled study. Front Neurosci. (2017) 11:179. doi: 10.3389/fnins.2017.00179
18. Frazer AK, Howatson G, Ahtiainen JP, Avela J, Rantalainen T, Kidgell DJ. Priming the motor cortex with anodal transcranial direct current stimulation affects the acute inhibitory corticospinal responses to strength training. J Strength Cond Res. (2019) 33(2):307–17. doi: 10.1519/JSC.0000000000002959
19. Summers RLS, Chen M, Hatch A, Kimberley TJ. Cerebellar transcranial direct current stimulation modulates corticospinal excitability during motor training. Front Hum Neurosci. (2018) 12:118. doi: 10.3389/fnhum.2018.00118
20. Williams JA, Pascual-Leone A, Fregni F. Interhemispheric modulation induced by cortical stimulation and motor training. Phys Ther. (2010) 90(3):398–410. doi: 10.2522/ptj.20090075
21. Machado D, Unal G, Andrade SM, Moreira A, Altimari LR, Brunoni AR, et al. Effect of transcranial direct current stimulation on exercise performance: a systematic review and meta-analysis. Brain Stimul. (2019) 12(3):593–605. doi: 10.1016/j.brs.2018.12.227
22. Bornheim S, Croisier JL, Leclercq V, Baude C, Kaux JF. The effects of tDCS on physical performances: a systematic literature review. Sci Sports. (2020) 35(5):255–70. doi: 10.1016/j.scispo.2020.05.002
23. Li H-J, He L-Y, Liu Z-S, Sun Y-N, Yan S-Y, Liu J, et al. On-site quality control of acupuncture randomized controlled trial: design of content and checklist of quality control based on PICOST. Zhongguo Zhen Jiu. (2014) 34(2):183–5.24796063
24. Cumpston MS, McKenzie JE, Welch VA, Brennan SE. Strengthening systematic reviews in public health: guidance in the Cochrane Handbook for Systematic Reviews of Interventions, 2nd edition. J Public Health. (2022) 44(4):E588–E92. doi: 10.1093/pubmed/fdac036
25. Hendy AM, Kidgell DJ. Anodal tDCS applied during strength training enhances motor cortical plasticity. Med Sci Sports Exercise. (2013) 45(9):1721–9. doi: 10.1249/MSS.0b013e31828d2923
26. Xiao S, Wang B, Yu C, Shen B, Zhang X, Ye D, et al. Effects of intervention combining transcranial direct current stimulation and foot core exercise on sensorimotor function in foot and static balance. J Neuroeng Rehabil. (2022) 19(1):34–89. doi: 10.1186/s12984-022-01077-5
27. Kim G-W, Ko M-H. Facilitation of corticospinal tract excitability by transcranial direct current stimulation combined with voluntary grip exercise. Neurosci Lett. (2013) 548:181–4. doi: 10.1016/j.neulet.2013.05.037
28. Hendy AM, Teo W-P, Kidgell DJ. Anodal transcranial direct current stimulation prolongs the cross-education of strength and corticomotor plasticity. Med Sci Sports Exercise. (2015) 47(9):1788–97. doi: 10.1249/MSS.0000000000000600
29. Wu L. Effects of Transcranial Direct Current Stimulation Combined with Blood Flow Restriction Training on Skeletal Muscle Strength and Anaerobic Exercise Capacity. Wuhan: Jianghan University (2023).
30. Ni L. Effect of Anodal Transcranial Direct Current Stimulation on Lower Limb Explosive Force of Male in Sports Colleges. Wuhan: Wuhan Sports University (2022).
31. Gua WG. Effects of Anodised Transcranial Direct Current Stimulation Combined with Unilateral Training on Lower Limb Explosive Strength of Male College Students in Sports Colleges. Wuhan: Wuhan Sports University (2023).
32. Nicholls JGRMA, Fuchs PA, Brown DA, Diamond ME, Weisblat DA. From Neuron to Brain(FIFTH EDITION). England: Sinauer Associates (2012).
33. Moritani T. Neuromuscular adaptations during the acquisition of muscle strength, power and motor tasks. J Biomech. (1993) 26(1):95–107. doi: 10.1016/0021-9290(93)90082-P
34. Del Vecchio A. Neuromechanics of the rate of force development. Exerc Sport Sci Rev. (2023) 51(1):34–42. doi: 10.1249/JES.0000000000000306
36. Nitsche MA, Paulus W. Sustained excitability elevations induced by transcranial DC motor cortex stimulation in humans. Neurology. (2001) 57(10):1899–901. doi: 10.1212/WNL.57.10.1899
37. Chase HW, Boudewyn MA, Carter CS, Phillips ML. Transcranial direct current stimulation: a roadmap for research, from mechanism of action to clinical implementation. Mol Psychiatry. (2020) 25(2):397–407. doi: 10.1038/s41380-019-0499-9
38. Ardolino G, Bossi B, Barbieri S, Priori A. Non-synaptic mechanisms underlie the after-effects of cathodal transcutaneous direct current stimulation of the human brain. J Physiol. (2005) 568(Pt 2):653–63. doi: 10.1113/jphysiol.2005.088310
39. Feurra M, Bianco G, Polizzotto NR, Innocenti I, Rossi A, Rossi S. Corticocortical connectivity between right parietal and bilateral primary motor cortices during imagined and observed actions: a combined TMS/tDCS study. Front Neural Circuits. (2011) 5(1):301–80. doi: 10.3389/fncir.2011.00010
40. Lang N, Siebner HR, Ward NS, Lee L, Nitsche MA, Paulus W, et al. How does transcranial DC stimulation of the primary motor cortex alter regional neuronal activity in the human brain? Eur J Neurosci. (2005) 22(2):495–504. doi: 10.1111/j.1460-9568.2005.04233.x
41. Reis J, Fritsch B. Modulation of motor performance and motor learning by transcranial direct current stimulation. Curr Opin Neurol. (2011) 24(6):590–6. doi: 10.1097/WCO.0b013e32834c3db0
42. Polania R, Nitsche MA, Paulus W. Modulating functional connectivity patterns and topological functional organization of the human brain with transcranial direct current stimulation. Hum Brain Mapp. (2011) 32(8):1236–49. doi: 10.1002/hbm.21104
43. Kenville R, Maudrich T, Maudrich D, Villringer A, Ragert P. Cerebellar transcranial direct current stimulation improves maximum isometric force production during isometric barbell squats. Brain Sci. (2020) 10(4):37–63. doi: 10.3390/brainsci10040235
44. Anoushiravani S, Alizadehgoradel J, Iranpour A, Bilehsavar OY, Pouresmali A, Nitsche MA, et al. The impact of bilateral anodal transcranial direct current stimulation of the premotor and cerebellar cortices on physiological and performance parameters of gymnastic athletes: a randomized, cross-over, shamcontrolled study. Sci Rep. (2023) 13(1):231–367. doi: 10.1038/s41598-023-37843-1
45. Goodwill AM, Pearce AJ, Kidgell DJ. Corticomotor plasticity following unilateral strength training. Muscle Nerve. (2012) 46(3):384–93. doi: 10.1002/mus.23316
46. Weier AT, Pearce AJ, Kidgell DJ. Strength training reduces intracortical inhibition. Acta Physiol. (2012) 206(2):109–19. doi: 10.1111/j.1748-1716.2012.02454.x
47. Boroda E, Sponheim SR, Fiecas M, Lim KO. Transcranial direct current stimulation (tDCS) elicits stimulus-specific enhancement of cortical plasticity. Neuroimage. (2020) 211:116598. doi: 10.1016/j.neuroimage.2020.116598
48. Strube W, Bunse T, Malchow B, Hasan A. Efficacy and interindividual variability in motor-cortex plasticity following anodal tDCS and paired-associative stimulation. Neural Plast. (2015) 2015:530423. doi: 10.1155/2015/530423
49. Bastani A, Jaberzadeh S. Does anodal transcranial direct current stimulation enhance excitability of the motor cortex and motor function in healthy individuals and subjects with stroke: a systematic review and meta-analysis. Clin Neurophysiol. (2012) 123(4):644–57. doi: 10.1016/j.clinph.2011.08.029
50. Monte-Silva K, Kuo M-F, Hessenthaler S, Fresnoza S, Liebetanz D, Paulus W, et al. Induction of late LTP-like plasticity in the human motor cortex by repeated non-invasive brain stimulation. Brain Stimul. (2013) 6(3):424–32. doi: 10.1016/j.brs.2012.04.011
51. Monai H, Ohkura M, Tanaka M, Oe Y, Konno A, Hirai H, et al. Calcium imaging reveals glial involvement in transcranial direct current stimulation-induced plasticity in mouse brain. Nat Commun. (2016) 7(1):11100. doi: 10.1038/ncomms11100
52. Alonzo A, Brassil J, Taylor JL, Martin D, Loo CK. Daily transcranial direct current stimulation (tDCS) leads to greater increases in cortical excitability than second daily transcranial direct current stimulation. Brain Stimul. (2012) 5(3):208–13. doi: 10.1016/j.brs.2011.04.006
53. Liu X, Yang X, Hou Z, Ma M, Jiang W, Wang C, et al. Increased interhemispheric synchrony underlying the improved athletic performance of rowing athletes by transcranial direct current stimulation. Brain Imaging Behav. (2019) 13(5):1324–32. doi: 10.1007/s11682-018-9948-3
54. Reis J, Schambra HM, Cohen LG, Buch ER, Fritsch B, Zarahn E, et al. Noninvasive cortical stimulation enhances motor skill acquisition over multiple days through an effect on consolidation. Proc Natl Acad Sci U S A. (2009) 106(5):1590–5. doi: 10.1073/pnas.0805413106
55. Zamparo P, Minetti AE, di Prampero PE. Interplay among the changes of muscle strength, cross-sectional area and maximal explosive power: theory and facts. Eur J Appl Physiol. (2002) 88(3):193–202. doi: 10.1007/s00421-002-0691-4
56. Stone MH, O'Bryant H, Garhammer J. A hypothetical model for strength training. J Sports Med Phys Fitness. (1981) 21(4):342–51.7339218
57. Lattari E, Campos C, Lamego MK, Legey S, Neto GM, Rocha NB, et al. Can transcranial direct current stimulation improve muscle power in individuals with advanced weight-training experience? J Strength Cond Res. (2020) 34(1):97–103. doi: 10.1519/JSC.0000000000001956
58. Chang W-J, Bennell KL, Hodges PW, Hinman RS, Young CL, Buscemi V, et al. Addition of transcranial direct current stimulation to quadriceps strengthening exercise in knee osteoarthritis: a pilot randomised controlled trial. PLoS One. (2017) 12(6):28–61. doi: 10.1371/journal.pone.0180328
59. Tanaka S, Takeda K, Otaka Y, Kita K, Osu R, Honda M, et al. Single session of transcranial direct current stimulation transiently increases knee extensor force in patients with hemiparetic stroke. Neurorehabil Neural Repair. (2011) 25(6):565–9. doi: 10.1177/1545968311402091
60. Abdelmoula A, Baudry S, Duchateau J. Anodal transcranial direct current stimulation enhances time to task failure of a submaximal contraction of elbow flexors without changing corticospinal excitability. Neuroscience. (2016) 322:94–103. doi: 10.1016/j.neuroscience.2016.02.025
61. Vargas VZ, Baptista AF, Pereira GOC, Pochini AC, Ejnisman B, Santos MB, et al. Modulation of isometric quadriceps strength in soccer players with transcranial direct current stimulation: a crossover study. J Strength Cond Res. (2018) 32(5):1336–41. doi: 10.1519/JSC.0000000000001985
62. Markovic G, Dizdar D, Jukic I, Cardinale M. Reliability and factorial validity of squat and countermovement jump tests. J Strength Cond Res. (2004) 18(3):551–5. doi: 10.1519/1533-4287(2004)18%3C551:RAFVOS%3E2.0.CO;2
63. Chen C-H, Chen Y-C, Jiang R-S, Lo L-Y, Wang IL, Chiu C-H. Transcranial direct current stimulation decreases the decline of speed during repeated sprinting in basketball athletes. Int J Environ Res Public Health. (2021) 18(13). doi: 10.3390/ijerph18136967
64. Kamali A-M, Ijadi M, Keshtkarhesamabadi B, Kazemiha M, Mahmoudi R, Roozbehi A, et al. A dual-mode neurostimulation approach to enhance athletic performance outcome in experienced taekwondo practitioners. Sci Rep. (2023) 13(1):31–46. doi: 10.1038/s41598-022-26610-3
65. Aagaard P, Simonsen EB, Andersen JL, Magnusson P, Dyhre-Poulsen P. Increased rate of force development and neural drive of human skeletal muscle following resistance training. J Appl Physiol (1985). (2002) 93(4):1318–26. doi: 10.1152/japplphysiol.00283.2002
66. Edwards DJ, Krebs HI, Rykman A, Zipse J, Thickbroom GW, Mastaglia FL, et al. Raised corticomotor excitability of M1 forearm area following anodal tDCS is sustained during robotic wrist therapy in chronic stroke. Restor Neurol Neurosci. (2009) 27(3):199–207. doi: 10.3233/RNN-2009-0470
67. Hummel F, Celnik P, Giraux P, Floel A, Wu W-H, Gerloff C, et al. Effects of non-invasive cortical stimulation on skilled motor function in chronic stroke. Brain J Neurol. (2005) 128(Pt 3):490–9. doi: 10.1093/brain/awh369
68. Tang W, Danyang L, Huili H, Fei X, Weifeng G, Lixu T, et al. Transcranial direct current stimulation intervention for motor performance: effects and applied strategies. China Sport Sci. (2020) 40(08):74–87.
Keywords: transcranial direct current stimulation, explosive strength, muscle strength, physical training, cerebral cortex excitability
Citation: Liu J, Li C, Fang J, Xu H, Zhang X and Zhao F (2024) Effects of physical training combined with transcranial direct current stimulation on maximal strength and lower limb explosive strength in healthy adults. Front. Sports Act. Living 6:1446588. doi: 10.3389/fspor.2024.1446588
Received: 10 June 2024; Accepted: 20 August 2024;
Published: 20 September 2024.
Edited by:
Paul J. Byrne, South East Technological University, IrelandReviewed by:
Anderson Souza Oliveira, Aalborg University, DenmarkVirginia López-Alonso, University of A Coruña, Spain
Copyright: © 2024 Liu, Li, Fang, Xu, Zhang and Zhao. This is an open-access article distributed under the terms of the Creative Commons Attribution License (CC BY). The use, distribution or reproduction in other forums is permitted, provided the original author(s) and the copyright owner(s) are credited and that the original publication in this journal is cited, in accordance with accepted academic practice. No use, distribution or reproduction is permitted which does not comply with these terms.
*Correspondence: Fan Zhao, Wmhhb2ZhbjgzQDEyNi5jb20=