- 1International Institute of Biomechanics and Occupational Ergonomics, Hyères, France
- 2Université de Toulon, Toulon, France
The objective of this systematic review and meta-analysis was to provide an overview of kinematic parameters associated with key points of interest in the tennis serve. The research was conducted according to the PRISMA guideline without date restriction. Google scholar, Science Direct, PubMed/Medline, Mendeley, and Science.gov databases were scanned to find relevant studies. Only English peer-review original article focused on joint body angles at trophy position, racket low point and ball impact were retained. The review, quality appraisal, and data extraction from selected studies were performed independently by two reviewers. A meta-analysis was carried out on the most studied joint parameters. Among the 2,844 records identified, 27 articles were included. The wide variety of methods used required data homogenization for comparison purposes. Trunk inclination (25.0 ± 7.1°) and front knee flexion (64.5 ± 9.7°) were the most studied parameters for trophy position. Shoulder lateral rotation (130.1 ± 26.5°) was systematically evaluated for racket low point. At ball impact, shoulder elevation (110.7 ± 16.9°) and elbow flexion (30.1 ± 15.9°) were the most considered joint angles. The systematic review revealed that many kinematic parameters were not quantified at the various key points of interest. Knowledge of the kinematics is essential for understanding the gesture, implementing training methods, and improving the performance.
1 Introduction
The serve is considered the most important stroke in tennis. It allows the player to start a point by controlling the speed and direction of the ball to take the advantage over the opponent. Many studies have divided the tennis serve into phases based on key points. Early studies focused on ball impact, quantifying the biomechanical parameters of the upper limb (1). Other authors have assessed the posture at the instant of maximal knee flexion (2) or maximal shoulder external rotation (3, 4). In 2011, Kovacs and Ellenbecker (5) described the service through 3 phases with 8 stages: preparation phase (start, release, loading, and cocking), acceleration phase (acceleration and contact) and follow through phase (deceleration and finish). The postures identified in previous work have been summarized in 4 key points: (1) ball release (BR) which corresponds to the moment when the ball leaves the player's non-serving hand (5); (2) trophy position (TP) defined as the moment when the racket reaches its first peak of vertical movement (6) and corresponding to a fully loaded lower body position, i.e., elbow lowest vertical position and maximum knee flexion (5); (3) racket low point (RLP), corresponding to the moment of maximum shoulder rotation with tip of the racket head pointing toward the ground (5) coinciding with the lowest vertical position of the racket when it is behind the back (6); (4) ball impact (BI), corresponding to contact between the racket's sieve and the ball (5). Most of these key points have been studied using kinematic data over the last 30 years. All these works have studied the tennis serve by including a large number of parameters such as age, level, serve type, serve velocity, foot or finishing technique, etc., while considering only a few kinematic data. Elliott and Wood (7) studied the effect of foot technique, i.e., foot-up vs. foot-back, on shoulder, elbow, wrist, and hip and knee flexion in the sagittal plane at BI (7). Reid et al. (8) addressed the effect of age (3 groups) during a flat serve from trunk inclination (TP), shoulder flexion and rotation (RLP and BI) and elbow flexion (BI). Tubez et al. (9) also studied the effect of age (3 groups) from trunk and shoulder rotation as well as elbow, wrist, and knee flexion but only for TP. Liang et al. (10) compared 3 levels of middle-school female players by considering lower limb joint angles at jump take-off and landing. In 2023, Touzard et al. (11) studied the effect of racket size during a flat serve only through knee and ankle flexion (TP). Reid and Giblin (12) compare a normal and an arabesque flat serve during the follow-through phase. The authors only considered trunk inclination at BI but studied peak values for the shoulder, hip and knee in during the preparation phase and in the final position of the serve. Mourtzios et al. (13) studied the effect of the serve type (flat, slice and topspin) on knee and ankle flexion for TP and BI. This effect of serve, flat, vs. kick, vs. slice, was also studied by Sheet et al. 2011 on back, shoulder, elbow, and wrist trajectories over the entire serve (14). Tanabe and Ito (15) compared the contribution of different angular velocities of the upper limb during a slow vs. fast serve. A few studies have investigated a more exhaustive list of parameters to qualify the serve. In 2003, Fleisig et al. (3) considered 6 joint angles (trunk, elbow, wrist and knee flexion, trunk inclination and shoulder elevation) for RLP and 5 joint angles (trunk, elbow, wrist and knee flexion, and trunk inclination) for BI. Fett et al. (24) reported 13 kinematic parameters for TP (trunk axial rotation), RLP (trunk, elbow and knee flexion, trunk and shoulder rotation and trunk tilt) and BI (trunk, elbow, wrist and knee flexion, trunk tilt and shoulder elevation).
Despite all this work, there is no consensus on the kinematic values at the key points of interest in the serve, and yet they are essential for understanding the gesture, optimization, and performance or training. To our knowledge, only the work of Brito et al. has assessed the “biophysics” of the tennis serve throughout a systematic scoping review (16). The authors proposed a mapping of relevant information (age, sex, level, measurement tools, playing surface, type of serve) to highlight what is already known and to identify gaps in the literature. However, no synthesis of the kinematic parameters that describe the tennis serve has been proposed. Currently, the numerous works available in the literature present a great heterogeneity of data, calculation methods, definition and location, making it difficult to compare data. One of the main challenges will be to propose a range of valid kinematic data for the different key points of the tennis serve. Trainers and coaches are interesting in using this data to optimize player performance, education and training. In this context, this review would provide a global view of kinematic data for tennis service worldwide.
The objective was to propose a detailed systematic review of kinematic data for all joint angles at each key point of interest, i.e., trophy position, racket low point, and ball impact. To achieve this, a precise study of all the work was necessary, in order to homogenize the data in the same reference frame, in accordance with the convention of the International Society of Biomechanics. A meta-analysis was carried out on the most studied parameters in order to propose a mean value with its standard deviation.
2 Materials and methods
This study was conducted according to PRISMA guidelines for reporting systematic reviews and meta-analyses (17). The search has been performed between September and December, 2023.
2.1 Search strategy and eligibility criteria
The search strategy included five databases: PubMed/Medline, Science Direct, Mendeley, Science.gov, and Google Scholar. The following set of keywords was used in each database: “Tennis” AND “Serve” AND “Kinematics”. The search was limited to English-language, full-text, peer-reviewed tennis serve studies. No date restrictions have been set. Studies were excluded if: (1) the study design is reviews, systematic reviews, conference proceedings, books or book chapters, commentaries, case studies, and case series; (2) no numerical kinematics data is described; (3) kinematics data is not related to a tennis serve key point of interest; (4) kinematic data do not refer to the anatomical angles of the tennis player (racket, ball, etc.); (5) tennis players are beginners or suffer from an injury. No restrictions regarding age, sex, level (above beginner), and type of measurement tool to quantify kinematic data were applied.
Results were imported from all databases and compiled into a table. An automatic function was used to remove duplicates. All publications were separately screened by two reviewers (PG and JJB) for eligibility according to the inclusion and exclusion criteria. Each reviewer excluded studies that did not meet the criteria. All differences were resolved by consensus after a further reading of the articles. The search strategy, the selection process, and reasons for exclusion are presented in the PRISMA flow chart (Figure 1).
2.2 Methodological quality appraisal and risk of bias
Two reviewers independently assessed the quality of the 27 included studies using the modified CONSORT 2010 checklist (17). The evaluations were compared and discrepancies were discussed to provide the final decision after rereading the article. The classification of McFarland and Fischer (18) was used to provide the quality appraisal: (1) checklist items over 85% corresponded to high quality; (2) checklist items less than 50% meant low quality; (3) studies with checklist items between 50% and 85% were considered as medium quality.
2.3 Data extraction
The following data were extracted from the included articles: country, number of participants, participant characteristics (sex, age, height, weight, level of practice), type of tennis serve (flat, kick, slice, and topspin), foot position, equipment used for kinematic data acquisition, all available body kinematic angles per key point of interest during the tennis serve (trophy position, racket low point and ball impact).
3 Results
3.1 Search results
The search of the 5 databases collected 2,844 articles. After removing the 356 duplicates, 2,488 articles were checked. From title/abstract screening, 2,431 were excluded. Among the 57 remaining articles, 30 were excluded after full reading because they did not meet the inclusion criteria. Finally, 27 articles were included in the present literature review. The search process is shown in Figure 1.
3.2 Quality appraisal
Table 1 shows the quality appraisal of the 27 included studies. Twenty four articles were assessed with medium quality (50%–85% of items present in each study) and three were assessed as low quality.
3.3 Study characteristics
The 27 articles selected cover 4 of the 5 continents (America, Asia, Europe, and Oceania) in 12 different countries. Seven studies were conducted solely on male tennis players (19, 21, 23, 24, 26, 30, 33), three studies included only female players (6, 8, 35), nine studies had a mixed population (1, 3, 11, 13, 20, 22, 25, 31, 36) and height did not provide this information (4, 9, 12, 27–29, 32, 34). Different age categories were studied. Data were reported using the following designations: children, teenagers, prepubescent, pubescent, adults (ranging from 9.3 (25) to 34.4 (31) years). Several levels of expertise were also studied. Information was reported using the terms: professional, national, international, International Tennis number ranking (ITN), International Tennis Federation ranking (ITF), Women's Tennis Association ranking (WTA), and Collegiates.
The characteristics of the tennis serve were studied through the type (flat, kick, slice, and topspin), foot position (foot up, foot back), and side (ad or deuce). Reid and Giblin also studied the effect of the final position of the lower limb during the follow-through phase [normal vs. arabesque (12)]. Four other studies investigated the effect of a specific condition: difference between two laboratories (32), racket size (11), wearing a postural shirt vs. a normal shirt (36), and with or without opponent (31).
All kinematic data reported in the studies follow the 3 key points of interest proposed by Whiteside et al. (6, 35): Trophy Phase (TP) position, Racket Low Point (RLP) and Ball Impact (BI). The kinematic parameters considered for the neck and trunk are flexion/extension, axial rotation and relative inclination. Absolute inclination (Tilt) relative to the service line for the trunk has been added, as it has been taken into account in some works. For the shoulder, flexion, abduction, axial rotation and elevation have been reported. Only flexion was studied for the elbow, wrist, knee and ankle. Finally, pronation/supination of the forearm and axial rotation of the pelvis were reported in one and two studies respectively. Table 2 summarizes the characteristics of the 27 studies and the kinematic data by key point.
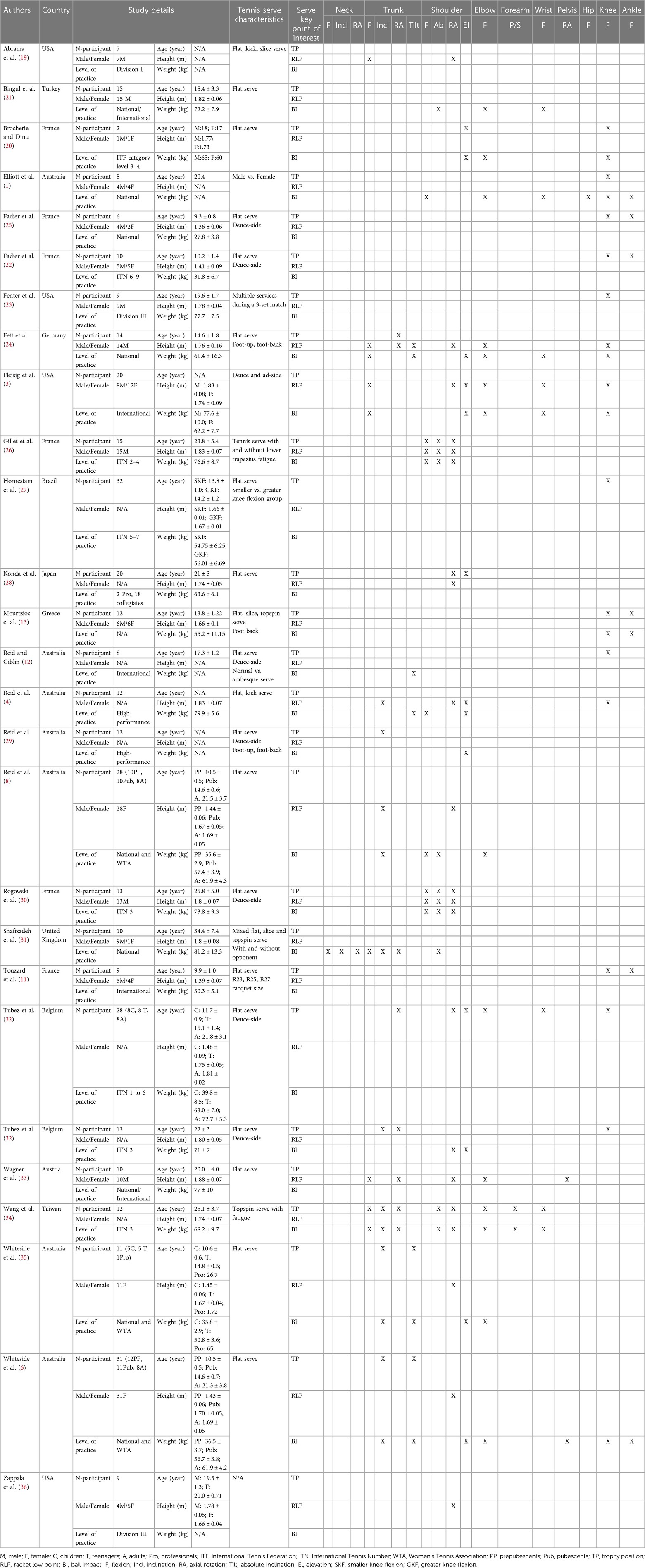
Table 2 Study characteristics and available kinematic data by key point of interest during tennis service for the 27 included studies.
Numerical kinematic values from the 27 included studies are presented by tennis serve key point of interest in Tables 3–5. Particular attention was paid to the data proposed in the studies, ensuring that they were defined in the same framework, following the International Society of Biomechanics' (ISB) conventions (37, 38). If this was not the case, a modification was applied to the data to make them homogeneous and therefore comparable with those of other studies. Two main cases were encountered: (1) when the authors reported intersegmental angular values, the complementary or supplementary angle was recomputed to correspond to the ISB definition, i.e., in the anatomical reference position (aligned segments) the joint angles are zero; (2) when the axes of rotation did not correspond to those of the ISB (notably for the shoulder and trunk), the movement was redecomposed to make the proposed values correspond to the ISB conventions.
3.4 Trophy position
Table 3 reports the kinematic data on TP extracted from 18 studies. Compared with Table 2, two columns have been added for knees and ankles, as many authors distinguish between the front and back lower limb. They considered the knee and ankle flexion of the front lower limb (closest to the service line), respectively called front knee and front ankle flexion, and those of the rear limb (respectively called back knee and back ankle flexion). The most studied joint areas were trunk inclination and knees flexion (front and back), with 15 and 22 sets of data respectively extracted from 6 to 11 studies. Neck joint angles, hip flexion, and pelvis axial rotation were not studied. Other joints were less studied, with only a few data sets available (between 2 and 9).
Figures 2–4 display the distribution of values for the three most studied joint angles: trunk inclination, front knee and back knee flexion. A mean value (± standard deviation) was computed from the data of all studies: 25.0 ± 7.1° for trunk inclination, 64.5 ± 9.7° and 67.4 ± 16.4° for front and back knee respectively. The plots show a wide dispersion of data between subjects within a same study. On the other hand, some studies report less dispersion.
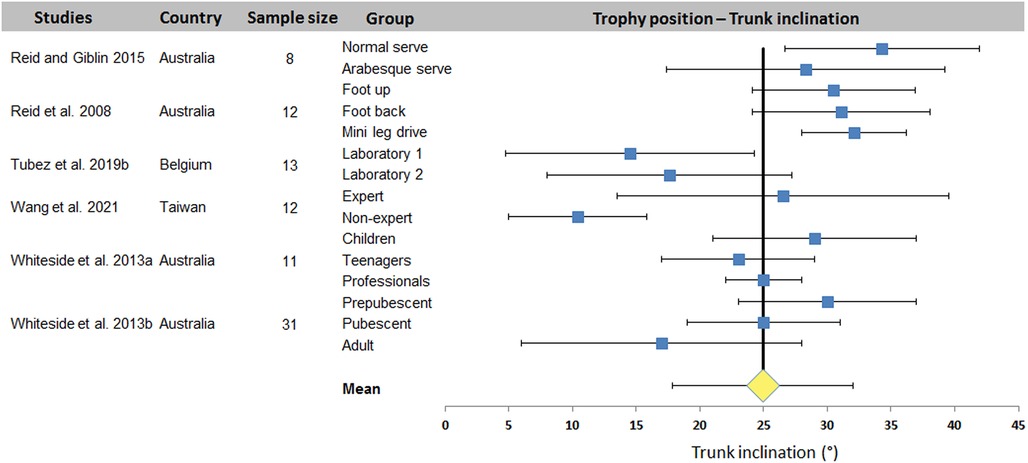
Figure 2 Distribution of trunk inclination values across studies. The square represents the mean value reported in each study. The diamond represents the mean trunk inclination computed over all studies. Horizontal bars represent standard deviation.
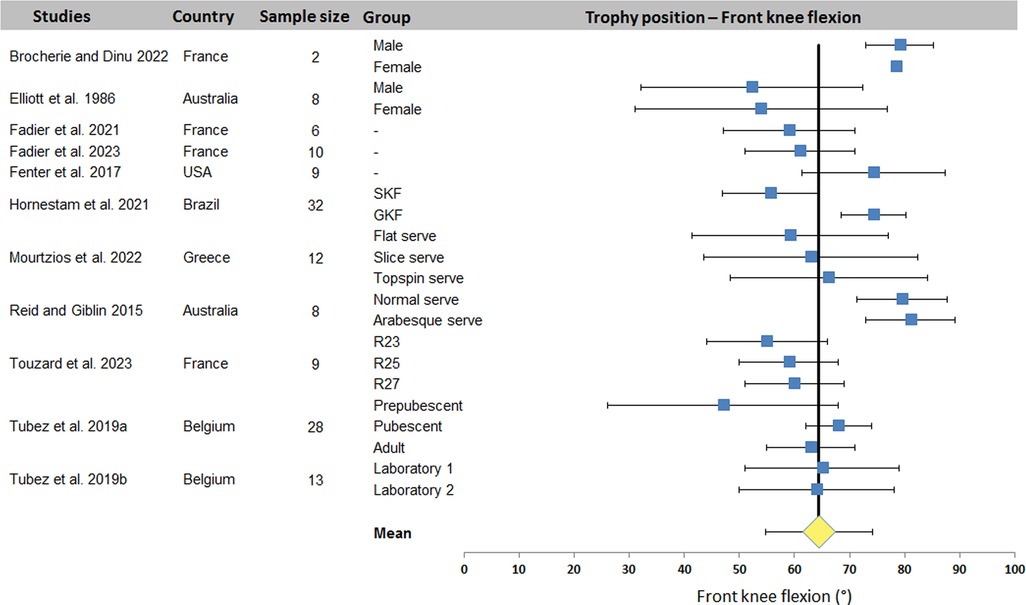
Figure 3 Distribution of front knee flexion values across studies. The square represents the mean value reported in each study. The diamond represents the mean front knee flexion computed over all studies. Horizontal bars represent standard deviation. R23-R25-R27, tennis racket size: scaled 23-inches, scaled 25-inches and full-size 27-inches; SKF, smaller knee flexion; GKF, greater knee flexion.
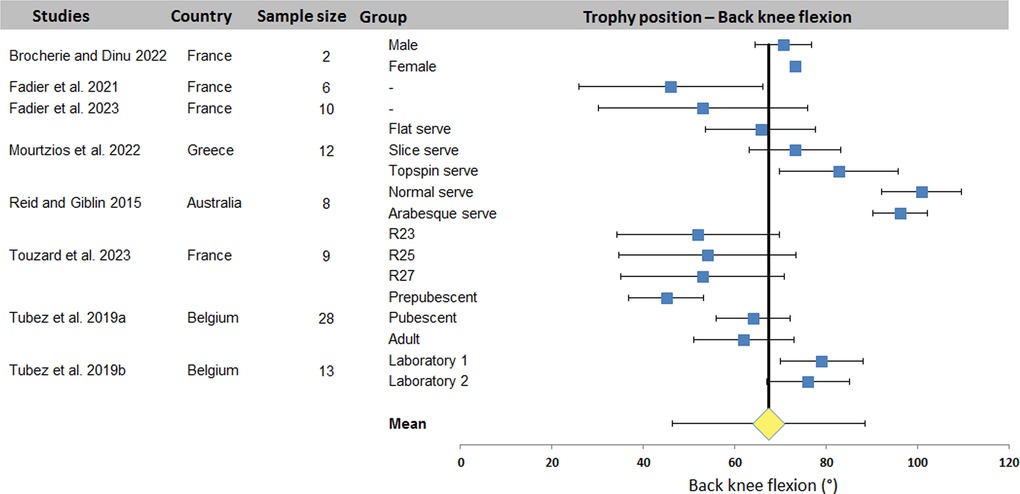
Figure 4 Distribution of back knee flexion values across studies. The square represents the mean value reported in each study. The diamond represents the mean back knee flexion computed over all studies. Horizontal bars represent standard deviation. R23-R25-R27, tennis racket size: scaled 23-inches, scaled 25-inches and full-size 27-inches.
3.5 Racket low point
Table 4 summarizes values of kinematic data on RLP extracted from 12 studies. Shoulder axial rotation was systematically measured (24 sets of data from the 12 studies). Neck joint angle, forearm pronation/supination, and hip and ankle flexions were not studied. Other joints were addressed in 1 or 4 studies (1–7 data sets).
Figure 5 presents the distribution of values for the shoulder lateral rotation. A mean value of 130.1 ± 26.5° was found across studies. The dispersion is smaller than that observed for the angles studied during the TP. Only Wagner et al. study (33) and the Whiteside et al. (6) studies have significant dispersion. It should be noted that Abrams et al. (19) work did not propose standard deviation values.
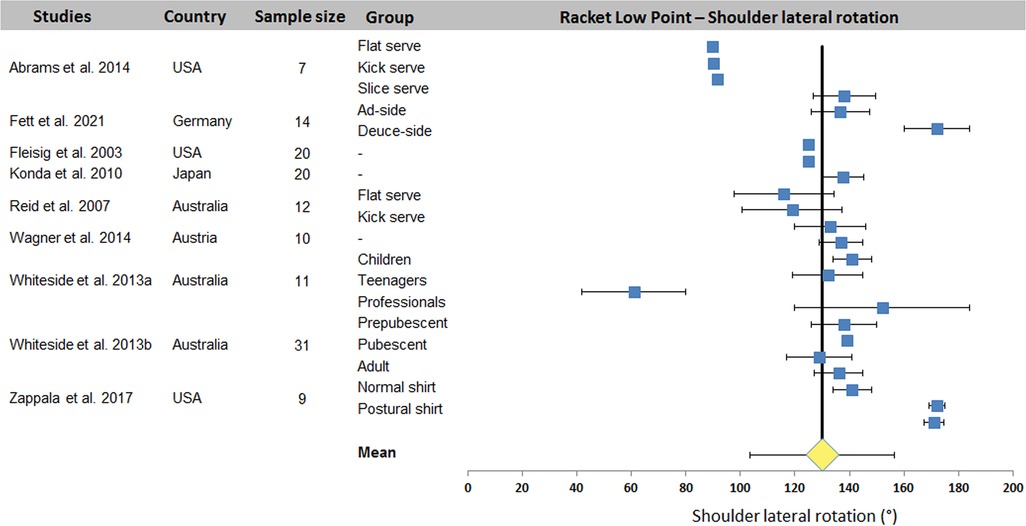
Figure 5 Distribution of shoulder lateral rotation values across studies. The square represents the mean value reported in each study. The diamond represents the mean shoulder external rotation computed over all studies. Horizontal bars represent standard deviation.
3.6 Ball impact
Table 5 diplays kinematic data from the 16 ball impact studies. Shoulder elevation and elbow flexion were the most studied joint angles (8 and 9 studies with 19 and 20 data sets respectively). Other joint angles were reported by 1–6 studies (2–13 data sets).
Figure 6 shows the 22 series of shoulder elevation data. Except the data from Brocherie's study (20), the observed values show relatively low variability, with acceptable homogeneity between studies despite the very different experimental conditions. A mean value of 110.7 ± 16.9° was computed for all 9 studies. Elbow flexion, on the other hand, showed greater extra- and intra-study dispersion (Figure 7). A mean value of 30.1 ± 15.9° was computed across the 10 studies.
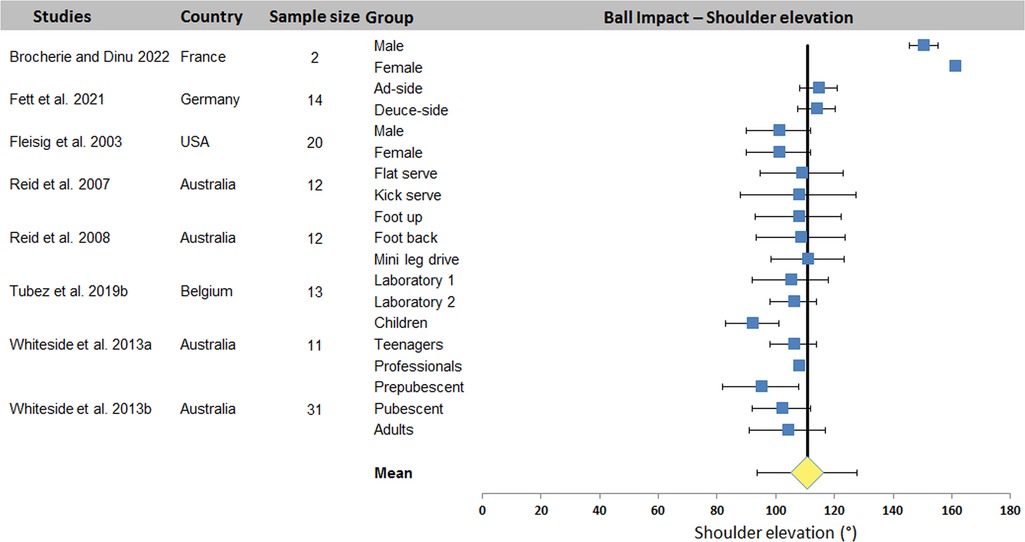
Figure 6 Distribution of shoulder elevation values across studies. The square represents the mean value reported in each study. The diamond represents the mean shoulder elevation computed over all studies. Horizontal bars represent standard deviation.
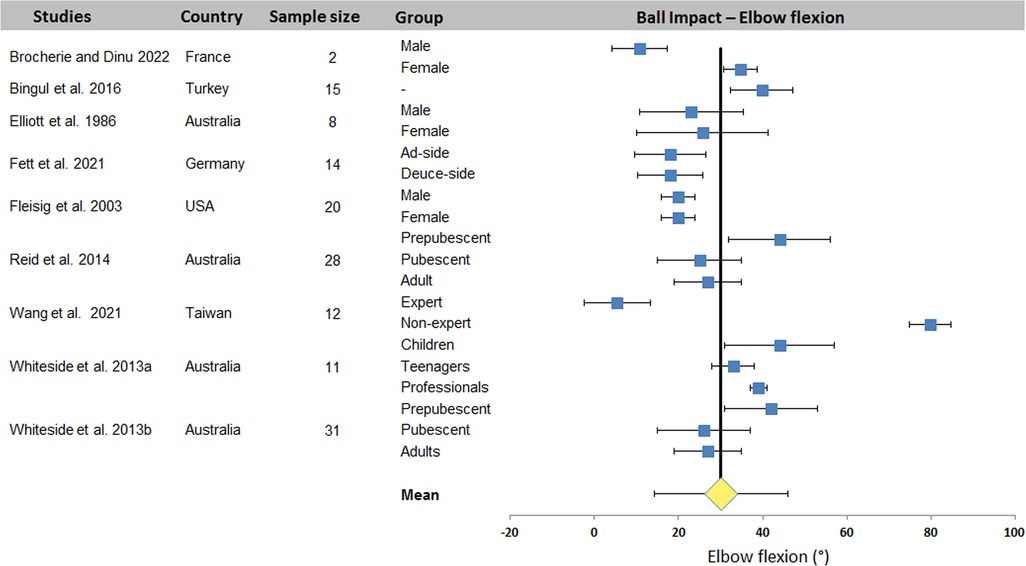
Figure 7 Distribution of elbow flexion across studies. The square represents the mean value reported in each study. The diamond represents the mean elbow flexion computed over all studies. Horizontal bars represent standard deviation.
4 Discussion
The aim of this study was to carry out a systematic review and meta-analysis of kinematic data on the tennis serve. One of the underlying questions is which joint angles are studied in relation to which key points, regardless of player characteristics (age, sex, level), methods of gesture analysis (video cameras, inertial measurement units, optoelectronic cameras, etc.) and the conditions under which the serve is studied (type of serve, foot technique, side of serve, etc.).
One general result that emerges clearly from this review is that not all the kinematic parameters that help define the player's body movement have been determined during the serve, i.e., all the joint angles. Summary tables were used to identify the joints studied in the selected studies (Table 2) and to quantify them according to each key point in each anatomical plane (Tables 3–5). The meta-analysis proposed mean values according to key points for 7 joint angles: trunk inclination (6 studies), front knee flexion (11 studies) and back knee flexion (8 studies) for TP, shoulder lateral rotation (9 studies) for RLP, and shoulder elevation (8 studies) and elbow flexion (9 studies) for BI. The data set presented is the result of data homogenization (system of common homogenous references in compliance with ISB convention).
The key point approach is often used in the literature. This could be explained by the complexity of the movement and the need for highly sophisticated measuring equipment to quantify whole-body motion during the full serve. Very few studies have proposed the temporal evolution of joint angles for all phases of the tennis serve (5). Reid et al. (4) and more recently Fett et al. (24) equipped all player segments (62 and 86 reflexive markers) and recorded movement using optoelectronic cameras. However, only a few parameters have been studied and temporal evolutions have hardly ever been exploited. Shafizadeh et al. (31) proposed a temporal profile of neck, back and shoulder abduction angles. The majority of studies have therefore used postures at various key points to study the tennis serve and some differences have been identified.
4.1 Characterization of key points during tennis serve
The analysis showed that there was a widespread consensus regarding the definition of serve key points, i.e., ball release, trophy position, racket low point, and ball impact. Trophy position was defined by Whiteside et al. (6) as the “first peak vertical displacement of the racket”. Kovacs and Ellenbecker (5) defines this moment as the “fully loaded lower body position, i.e., elbow lowest vertical position and maximum knee flexion”. Reid et al. (4) specifies that this position is characterized only by the knee flexion. Racket low point has been defined as the lowest vertical position of the racket when it is behind the back (6). Kovacs completes this definition by adding that this instant corresponds to maximal shoulder external rotation coinciding with the tip of the racket head pointing toward the ground (5). Several authors have therefore equated this key point with the moment of maximum external shoulder rotation (4, 19). The authors all agree that Ball Impact is the instant of contact between the ball and the racket. These key points have been used to define the different phases of the serve. There are two approaches. One considers that a phase is defined between two key points. Three phases are then described: preparation, propulsion and forwardswing (6, 24). The second approach is also divided into 3 phases with 8 stages: preparation phase (4 phases: start, release, loading, and cocking), acceleration phase (2 phases: acceleration and contact) and follow through phase (2 phase: deceleration and finish) (5, 20). In this framework, the definition of the three key points presented in this review, i.e., TP, RLP and BI, has taken into account the great variability in the names and parameters associated with these key points to construct summary Tables 2–5.
4.2 Different methods for defining and measuring joint angles between studies
Completing Tables 2–5 required particular care in determining the kinematic values proposed. These are directly linked to the measurement methods and conventions used to define the various computational references. This is despite the existence of an international convention published by the International Society of Biomechanics (37, 38) to define all anatomical landmarks. Several difficulties were encountered. Firstly, several authors used different vocabulary to define the same joint angle. The most common example is trunk inclination.
Several terms have been used: “lateral flexion” (31), “trunk tilt” (6), and “lateral flexion shoulder-pelvis alignment separation angle” (4). A wide disparity was also observed in the definition of shoulder angles. Terms such as horizontal flexion (8), horizontal shoulder extension (9), upper arm-thorax elevation angle (4), shoulder horizontal adduction (3), or simply “shoulder angle” (21) make it difficult to interpret values. Only medio-lateral rotation has been correctly defined using the terms external and internal shoulder rotation. Another difficulty was encountered with the shoulder. In the landmark-based computation method, the choice of rotation sequence has a direct influence on the rotation angle values obtained (39). As a result, several angles cannot be compared due to this difference in computation technique.
On the other hand, some angle definitions are defined in relation to different references, which make it impossible to compare specific angles with others. For example, we find trunk tilt for the rotation of the trunk in relation to the pelvis, and upper torso position for the angle between the trunk and the baseline, measured in the absolute reference (24). There were also variations in angle measurements. According to the ISB convention, all angles are defined as zero in the anatomical reference position. However, some authors considered the direct value separating two consecutive segments, resulting in a value of 180° when the two segments are aligned. This problem has been encountered for elbows (21), knees (13, 20) and ankles (35), requiring data transformation in order to make comparisons with other articles.
Finally, when a rotation was expressed in a non-conventional frame of reference or by the absence of a direction of rotation, modifications were made to homogenize the data.
4.3 Data heterogeneity
The systematic review identified 27 articles in which a total of 18 kinematic parameters were quantified at different key point of interest in the tennis serve. Results (Table 2) showed that Wang et al. (34) reported the highest number of data for one phase (8 joint angles) and also the highest number of parameters in one study with 16 values (8 for TP and 8 for BI). Fett et al. (24) and Fleisig et al. (3) rank second and third respectively, with 13 and 11 kinematic parameters studied, including the maximum for RLP (6 joint angles). Table 2 also shows that trunk inclination, shoulder rotation and elevation, elbow flexion and knee flexion were the most studied joints, regardless of experimental conditions. These 5 joint angles were retained for a meta-analysis. The results were presented in the form of forest plots, showing the mean and standard deviation of each condition in each study, as well as the mean over all the included studies. For TP, a mean of 25.0 ± 7.1° was found for trunk inclination, 64.5 ± 9.7° for front knee and 67.4 ± 16.4° for back knee flexion. For RLP, a mean of 130.1 ± 26.5° was obtained for shoulder external rotation. Finally, a mean of 110.7 ± 16.9° and 30.1 ± 15.9° were computed for shoulder elevation and elbow flexion respectively for BI.
For TP, the results show a low overall variability for trunk and front knee flexion. Other variability ranges from 20° to 50°. These higher variabilities are the consequence of one or two studies that have a significant impact on dispersion. This is true of the studies by Brocherie and Dinu (20) for shoulder elevation and Wang et al. (34) for elbow flexion during BI. The relative dispersion of each study shows variability (between 2 and 60° dispersion). Under these conditions, the smaller the number of studies included in the meta-analysis, the greater the effect of the dispersion of each study on the overall variability of each kinematic parameter studied. In our case, the parameter with the most data, i.e., shoulder axial rotation, only provided 22 quantified data sets for 16 studies.
The wide variability observed between studies is due to the wide range of populations evaluated and the experimental conditions under which the tennis serve was carried out. Indeed, a large variability in age [children aged 9 (11) vs. pubescent (4), vs. adult (31)] and level [national (24) vs. international (3)] was observed between the included studies (for both sexes). On the other hand, the type of serve, the foot technique, the side of the serve, the conditions of execution (match or laboratory, with a target or not, with an opponent or not, state of fatigue) are all parameters that enhance the heterogeneity of the results. Despite this heterogeneity, the analysis has enabled us to propose an average value for trunk inclination and front knee flexion, with a dispersion of less than 20°, which can be used in training, education and optimization. To these two parameters, we could add shoulder elevation and elbow flexion, which would have an equivalent dispersion without the studies that present very different results without any particular justification. The mean values would change from 110.7 ± 16.9° and 30.1 ± 15.9° to 104.6 ± 6.1° and 29.2 ± 9.9° respectively, making them more relevant.
4.4 Application of key findings
The meta-analysis enabled to propose, for each key point of the serve, the joint angles encountered in the greatest number of studies to quantify a sufficiently relevant and homogeneous mean value. The results were: 25.0 ± 7.1° for trunk inclination, 64.5 ± 9.7° for front knee flexion, and 67.4 ± 16.4° for back knee flexion during TP; 130.1 ± 26.5° for shoulder lateral rotation during RLP; and 110.7 ± 16.9° for shoulder elevation and 30.1 ± 15.9° for elbow flexion during BI. Hornestam et al. (27) showed that knee flexion had an impact on serve performance. Indeed, the group with the lowest knee flexion, 10° less than that presented in the present study (55.6 ± 8.47°), had a racket velocity reduction of 3.33 km.h−1. A recent study also showed that knee flexion was correlated with racket velocity, indicating that greater flexion results in greater racket velocity (40). It would therefore be advisable to look for significant knee flexion during TP in order to optimize racket velocity during the serve. The search for significant lateral shoulder rotation also seems to be important at RLP (8, 24). In fact, this rotation is directly correlated to the racket velocity (40). In addition, a high lateral rotation generates a very high medial rotation velocity, which contributes 40%–50% to the racket linear velocity (15, 41). At BI, shoulder elevation and low elbow flexion contribute to ball impact at high altitudes above 2.5 m (6, 24). Many authors have reported elbow flexion close to 30° at BI (1, 35). A slightly flexed elbow seems to have a mechanical advantage for the medial rotation of the shoulder and therefore the racket velocity (42).
4.5 Limitations
Some limitations should be addressed. The first limitation concerns data acquisition. Very different measurement tools were used to quantify the kinematic parameters. This implies a different level of precision which could have an incidence on the values obtained. A second limitation concerns the small sample size (2–32) and the low number of repetitions (<5), which are not statistically representative of the population. Another limitation concerns the selection of studies through inclusion criteria (limited to “original article” written in English). This could have led to exclude or omit interesting works that could have completed and extended the results of the present review and meta-analysis.
5 Conclusion
The present systematic review and meta-analysis identified trunk inclination, shoulder elevation and lateral rotation, elbow flexion and knee flexion as the most studied kinematic parameters at the various key points of interest in tennis serve. A mean value with a standard deviation has been proposed for each of them. More work needs to be carried out in the future, taking into account as many joint angles as possible, in order to obtain more data on the complete kinematics of the various key tennis serve postures. The full set of kinematic parameters is essential for a precise understanding of the tennis serve motion, and for their use in training, coaching and performance optimization.
Author contributions
JJ-B: Conceptualization, Data curation, Formal Analysis, Investigation, Methodology, Resources, Software, Validation, Visualization, Writing – original draft, Writing – review & editing. PG: Conceptualization, Data curation, Formal Analysis, Funding acquisition, Investigation, Methodology, Project administration, Resources, Software, Supervision, Validation, Visualization, Writing – original draft, Writing – review & editing.
Funding
The author(s) declare that no financial support was received for the research, authorship, and/or publication of this article.
Conflict of interest
The authors declare that the research was conducted in the absence of any commercial or financial relationships that could be construed as a potential conflict of interest.
Publisher's note
All claims expressed in this article are solely those of the authors and do not necessarily represent those of their affiliated organizations, or those of the publisher, the editors and the reviewers. Any product that may be evaluated in this article, or claim that may be made by its manufacturer, is not guaranteed or endorsed by the publisher.
References
1. Elliott B, Marsh T, Blanksby B. A three-dimensional cinematographic analysis of the tennis serve. Int J Sport Biomech. (1986) 2:260–71. doi: 10.1123/ijsb.2.4.260
2. Elliott B, Fleisig G, Nicholls R, Escamilia R. Technique effects on upper limb loading in the tennis serve. J Sci Med Sport. (2003) 6:76–87. doi: 10.1016/S1440-2440(03)80011-7
3. Fleisig G, Nicholls R, Elliott B, Escamilla R. Kinematics used by world class tennis players to produce high-velocity serves. Sports Biomech. (2003) 2:51–64. doi: 10.1080/14763140308522807
4. Reid M, Elliott B, Alderson J. Shoulder joint loading in the high performance flat and kick tennis serves. Br J Sports Med. (2007) 41:884–9. doi: 10.1136/bjsm.2007.036657
5. Kovacs M, Ellenbecker T. An 8-stage model for evaluating the tennis serve:implications for performance enhancement and injury prevention. Sports Health. (2011) 3:504–13. doi: 10.1177/1941738111414175
6. Whiteside D, Elliott B, Lay B, Reid M. A kinematic comparison of successful and unsuccessful tennis serves across the elite development pathway. Hum Mov Sci. (2013) 32:822–35. doi: 10.1016/j.humov.2013.06.003
7. Elliott B, Wood G. The biomechanics of the foot-up and foot-back tennis service techniques. Australian Journal of Sports Sciences. (1983) 3:3–6.
8. Reid M, Giblin G, Whiteside D. A kinematic comparison of the overhand throw and tennis serve in tennis players: how similar are they really? J Sports Sci. (2014) 33:713–23. doi: 10.1080/02640414.2014.962572
9. Tubez F, Schwartz C, Croisier J-L, Brüls O, Denoël V, Paulus J, et al. Evolution of the trophy position along the tennis serve player’s development. Sports Biomech. (2021) 20:431–43. doi: 10.1080/14763141.2018.1560493
10. Liang Z, Wu J, Yu J, Ying S, Liu Z, Zhang Y, et al. Comparison and analysis of the biomechanics of the lower limbs of female tennis players of different levels in foot-up serve. Front Physiol. (2023) 14:1125240. doi: 10.3389/fphys.2023.1125240
11. Touzard P, Lecomte C, Bideau B, Kulpa R, Fourel L, Fadier M, et al. There is no rush to upgrade the tennis racket in young intermediate competitive players: the effects of scaling racket on serve biomechanics and performance. Front Psychol. (2023) 14:1104146. doi: 10.3389/fpsyg.2023.1104146
12. Reid M, Giblin G. Another day, another tennis coaching intervention, but does this one do what coaches purport? Sports Biomech. (2015) 14:180–9. doi: 10.1080/14763141.2015.1045549
13. Mourtzios C, Athanailidis I, Arvanitidou V, Kellis E. Ankle and knee joint kinematics differ between flat, slice and topspin serves in young tennis players. Eur J Sport Sci. (2022) 1:16–22. doi: 10.24018/ejsport.2022.1.2.13
14. Sheets AL, Abrams GD, Corazza S, Safran MR, Andriacchi TP. Kinematics differences between the flat, kick, and slice serves measured using a markerless motion capture method. Ann Biomed Eng. (2011) 39:3011–20. doi: 10.1007/s10439-011-0418-y
15. Tanabe S, Ito A. A three-dimensional analysis of the contributions of upper limb joint movements to horizontal racket head velocity at ball impact during tennis serving. Sports Biomech. (2007) 6:418–33. doi: 10.1080/14763140701491500
16. Brito AV, Afonso J, Silva G, Fernandez-Fernandez J, Fernandes RJ. Biophysical characterization of the tennis serve: a systematic scoping review with evidence gap map. J Sci Med Sport. (2024) 27:125–40. doi: 10.1016/j.jsams.2023.10.018
17. Schulz KF, Altman DG, Moher D, The CG. CONSORT 2010 Statement: updated guidelines for reporting parallel group randomised trials. BMC Med. (2010) 8:18. doi: 10.1186/1741-7015-8-18
18. Mcfarland T, Fischer S. Considerations for industrial use: a systematic review of the impact of active and passive upper limb exoskeletons on physical exposures. IISE Trans Occup Ergon Hum Factors. (2019) 7:322–47. doi: 10.1080/24725838.2019.1684399
19. Abrams GD, Harris AH, Andriacchi TP, Safran MR. Biomechanical analysis of three tennis serve types using a markerless system. Br J Sports Med. (2014) 48:339–42. doi: 10.1136/bjsports-2012-091371
20. Brocherie F, Dinu D. Biomechanical estimation of tennis serve using inertial sensors: a case study. Front Sports Act Living. (2022) 4:962941. doi: 10.3389/fspor.2022.962941
21. Bingül BM, Aydin M, Bulgan Ç, Gelen E, Özbek A. Upper extremity kinematics of flat serve in tennis. S Afr J Res Sport Phys Educ. (2016) 38:17–25. doi: 10.4314/SAJRS.V38I2
22. Fadier M, Touzard P, Lecomte C, Bideau B, Cantin N, Martin C. Do serve distance and net height modify serve biomechanics in young tennis players? Int J Sports Sci Coach. (2023) 18:1513–20. doi: 10.1177/17479541221114106
23. Fenter B, Marzilli TS, Wang YT, Dong XN. Effects of a three-set tennis match on knee kinematics and leg muscle activation during the tennis serve. Percept Mot Skills. (2017) 124:214–32. doi: 10.1177/0031512516672773
24. Fett J, Oberschelp N, Vuong JL, Wiewelhove T, Ferrauti A. Kinematic characteristics of the tennis serve from the ad and deuce court service positions in elite junior players. PLOS ONE. (2021) 16:e0252650. doi: 10.1371/journal.pone.0252650
25. Fadier M, Touzard P, Martin C. Preliminary kinematic analysis of the serve in 10 and under players. Coach Sport Sci Rev. (2021) 29(85):12–4. doi: 10.52383/itfcoaching.v29i85.275
26. Gillet B, Rogowski I, Monga-Dubreuil E, Begon M. Lower trapezius weakness and shoulder Complex biomechanics during the tennis serve. Med Sci Sports Exerc. (2019) 51:2531–9. doi: 10.1249/MSS.0000000000002079
27. Hornestam JF, Souza TR, Magalhaes FA, Begon M, Santos TRT, Fonseca ST. The effects of knee flexion on tennis serve performance of intermediate level tennis players. Sensors. (2021) 21. doi: 10.3390/s21165254
28. Konda S, Yanai T, Sakurai S. Scapular rotation to attain the peak shoulder external rotation in tennis serve. Med Sci Sports Exerc. (2010) 42:1745–53. doi: 10.1249/MSS.0b013e3181d64103
29. Reid M, Elliott B, Alderson J. Lower-limb coordination and shoulder joint mechanics in the tennis serve. Med Sci Sports Exerc. (2008) 40:308–15. doi: 10.1249/mss.0b013e31815c6d61
30. Rogowski I, Creveaux T, Sevrez V, Cheze L, Dumas R. How does the scapula move during the tennis serve? Med Sci Sports Exerc. (2015) 47:1444–9. doi: 10.1249/MSS.0000000000000543
31. Shafizadeh M, Bonner S, Barnes A, Fraser J. Effects of task and environmental constraints on axial kinematic synergies during the tennis service in expert players. Eur J Sport Sci. (2019) 20:1178–86. doi: 10.1080/17461391.2019.1701093
32. Tubez F, Forthomme B, Croisier JL, Bruls O, Denoel V, Paulus J, et al. Inter-session reliability of the tennis serve and influence of the laboratory context. J Hum Kinet. (2019) 66:57–67. doi: 10.2478/hukin-2018-0064
33. Wagner H, Pfusterschmied J, Tilp M, Landlinger J, Von Duvillard SP, Muller E. Upper-body kinematics in team-handball throw, tennis serve, and volleyball spike. Scand J Med Sci Sports. (2014) 24:345–54. doi: 10.1111/j.1600-0838.2012.01503.x
34. Wang LH, Lo KC, Su FC. Skill level and forearm muscle fatigue effects on ball speed in tennis serve. Sports Biomech. (2021) 20:419–30. doi: 10.1080/14763141.2018.1560492
35. Whiteside D, Elliott B, Lay B, Reid M. The effect of age on discrete kinematics of the elite female tennis serve. J Appl Biomech. (2013) 29:573–82. doi: 10.1123/jab.29.5.573
36. Zappala J, Orrego C, Boe E, Fechner H, Salminen D, Cipriani DJ. Influence of posture-cuing shirt on tennis serve kinematics in division III tennis players. J Chiropr Med. (2017) 16:49–53. doi: 10.1016/j.jcm.2016.05.003
37. Wu G, Siegler S, Allard P, Kirtley C, Leardini A, Rosenbaum D, et al. ISB Recommendation on definitions of joint coordinate system of various joints for the reporting of human joint motion—part I: ankle, hip, and spine. J Biomech. (2002) 35:543–8. doi: 10.1016/S0021-9290(01)00222-6
38. Wu G, Van Der Helm FCT, Veeger HEJ, Makhsous M, Van Roy P, Anglin C, et al. ISB Recommendation on definitions of joint coordinate systems of various joints for the reporting of human joint motion—part II: shoulder, elbow, wrist and hand. J Biomech. (2005) 38:981–92. doi: 10.1016/j.jbiomech.2004.05.042
39. Bonnefoy-Mazure A, Slawinski J, Riquet A, Leveque JM, Miller C, Cheze L. Rotation sequence is an important factor in shoulder kinematics. Application to the elite players’ flat serves. J Biomech. (2010) 43:2022–5. doi: 10.1016/j.jbiomech.2010.03.028
40. Jacquier-Bret J, Gorce P. Kinematics of the tennis serve using an optoelectronic motion capture system: are there correlations between joint angles and racket velocity? Sensors. (2024) 24:3292. doi: 10.3390/s24113292
41. Elliott B, Marshall R, Noffal G. Contributions of upper limb segment rotations during the power serve in tennis. J Appl Biomech. (1995) 11:433–42. doi: 10.1123/jab.11.4.433
Keywords: tennis player, tennis serve, kinematics, biomechanics, trophy position, racket low point, ball impact, worldwide analysis
Citation: Jacquier-Bret J and Gorce P (2024) Kinematics characteristics of key point of interest during tennis serve among tennis players: a systematic review and meta-analysis. Front. Sports Act. Living 6:1432030. doi: 10.3389/fspor.2024.1432030
Received: 13 May 2024; Accepted: 13 June 2024;
Published: 8 July 2024.
Edited by:
Laura E. Diamond, Griffith University, AustraliaReviewed by:
Sam Hamner, Wu Tsai Human Performance Alliance, United StatesYuqi He, University of Pannonia, Hungary
Dusan Radivoje Mitic, University of Belgrade, Serbia
© 2024 Jacquier-Bret and Gorce. This is an open-access article distributed under the terms of the Creative Commons Attribution License (CC BY). The use, distribution or reproduction in other forums is permitted, provided the original author(s) and the copyright owner(s) are credited and that the original publication in this journal is cited, in accordance with accepted academic practice. No use, distribution or reproduction is permitted which does not comply with these terms.
*Correspondence: Julien Jacquier-Bret, amFjcXVpZXJAdW5pdi10bG4uZnI=