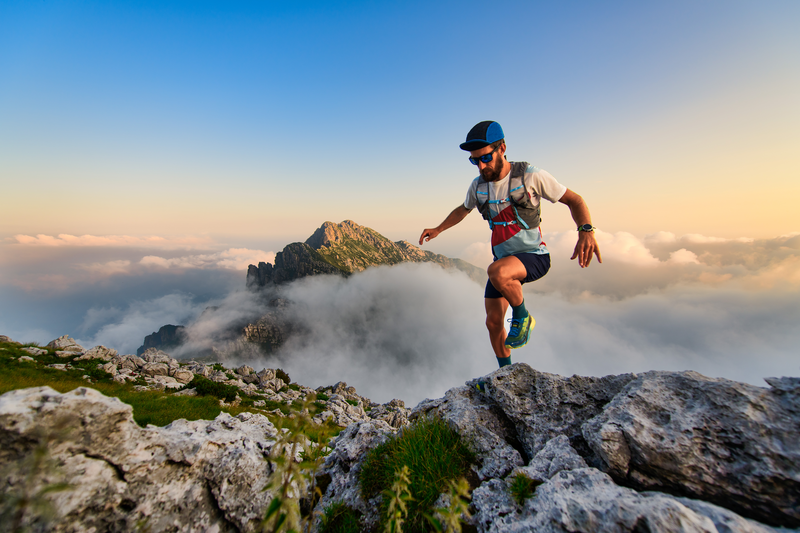
94% of researchers rate our articles as excellent or good
Learn more about the work of our research integrity team to safeguard the quality of each article we publish.
Find out more
PERSPECTIVE article
Front. Sports Act. Living , 18 March 2024
Sec. Elite Sports and Performance Enhancement
Volume 6 - 2024 | https://doi.org/10.3389/fspor.2024.1363730
Since swimming performance depends on both physical conditioning and technical proficiency, training zones should be built based on physiology and biomechanics inputs to dispose of structured and effective training programs. This paper presents a zone-based swimming training, supported by the oxygen uptake (O2) kinetics at low, moderate, heavy, severe and extreme intensities concurrently with lactate and heart rate values. Since technique is vital for efficiently moving through the water, upper limbs frequency and length should also be targeted during the workouts. The index of coordination was also added to our proposal since upper limbs synchronization is a key technical factor. To better establish and characterize a wide range of swimming intensities, the training methods and corresponding contents that better fit each training zone will be suggested. It will be shown that when under/at the anaerobic threshold (at low-to-moderate intensities), swimmers are at homeostasis and can maintain stable internal and external load indicators. However, above that boundary (at heavy and severe intensities), the physiological stable state is no longer observed and the anaerobic metabolism starts contributing significantly, with a technical degradation being more evident when performing near/at the O2max intensity. Then, when performing above aerobic power, on typical anaerobic intensities, O2 kinetics presents a very evident fast rise, ending abruptly due to exhaustion caused by muscle acidosis. This overall knowledge allows advancing toward more objective training programs and highlights the importance of systematic training control and swimmers' evaluation and advice.
Swimming is a sport where participants attempt to cover a specific distance in the shortest possible time and, due to its characteristics, has a strong influence from both physiological and biomechanical variables (1). Since competitive swimming races last from ∼20 s to 15 min (the 50 and 1,500 m events, respectively), it becomes evident that swimmers’ exertions rely on well-developed aerobic and anaerobic energy pathways (2–6). Thus, the importance of monitoring the training process to increase the available quantity and quality of information is unquestionable (7–10). The aerobic and anaerobic energy sources have been traditionally evaluated using O2 and blood lactate concentrations [La−], and heart rate (HR) has been a complementary indicator mainly used in practical settings where expensive instruments and complex procedures are challenging. The assessment of the ATP-CP contribution in swimming has not been a priority because the phosphagen stores have a small capacity, present low relevance in most of the competitive events (11) and muscular biopsy is an invasive procedure very hard to be conducted (12–14).
Swimming technique is also a critical performance determinant (15–18), as displayed in the well-known biophysical expression that exhibit that the maximum velocity attained in a particular context equals the product of the energy input by the ratio between the propulsive efficiency of the biomechanical system and the hydrodynamic drag opposed to the swimmer displacement (19, 20). Swimming can be seen as a thermodynamical process, where energy is processed in each instant of time until a mechanical work is performed with a given energy efficiency (17, 21). However, since the human body has a very low propelling efficiency, slight technical changes positively contributes to performance (8, 11, 22). As swimming technique evaluation is complex, researchers have been assessing stroke frequency (SF) and length (SL), and stroke index (SI), for a long time to observe if a measurable change in O2, [La−] or HR may well reflect technical variability (23, 24). More recently, measuring the lag time between propulsive phases of upper limbs (the Index of Coordination—IdC) became popular for characterizing the coordination patterns during swimming (25). In the current perspective, we present a zone-based swimming training supported by the O2 behaviour at low, moderate, heavy, severe and extreme intensities, concurrently with [La−]and HR values. Also, SF, SL and IdC will be described for each zone due to their biomechanical relevance.
The establishment of accurate training zones for increasing performance in individual, cyclic and continuous sports like swimming has been conducted for many years and it seems evident that the training process should reflect the demands of the races (1, 2, 22). For that purpose, swimmers' monitoring has become more frequent, growing in importance with the appearance of portable O2 apparatus, [La−] analysers, HR monitors and waterproof cameras (10, 11, 26). However, only a single value of one (or more) variable(s) has been used to define a training zone or characterize a swimming event. In addition, studies usually present O2, [La−] or HR maximal values and the mean SF and SL scores in a single bout or incremental protocols, not considering their behaviour along the entire exercise duration. This could be due to the difficulties in assessing internal and external load variables while swimming in standard pools and to the low number of available swimming flumes. Furthermore, even if the general kinematical variables are more easily obtainable by counting the number of cycles per lap or using a chronofrequencemeter, the digitalization of anatomical body parts is more rigorous but very time-demanding, leading to the analysis of only one swimming cycle in a single lap as representative of the entire exercise [e.g., (17)].
The detailed behaviour changes of relevant physiological and biomechanical variables with the swimming intensity variation would allow a more accurate definition of the training zones. O2 assessment in swimming has been implemented regularly only since the 1970s due to previous methodological difficulties (e.g., the incapacity to follow the swimmer along the pool, the difficulty in transporting equipment and the added drag imposed by the respiratory snorkel (27). With the development of O2 assessment portable and automated systems at the beginning of the current century, it became possible to obtain real-time values during swimming, not only during the recovery phase after exercise (26, 28, 29). Even if the specific O2 dynamics at the low, moderate, heavy, severe and extreme intensity domains have been mainly centred on running and cycling in laboratory settings (2, 30, 31), some studies conducted a breath-by-breath analysis of well-trained swimmers performing in competition-like conditions. As displayed in Figure 1, at low and moderate swimming intensities, O2 is characterized by a fast rise (after a non-expressive cardiodynamic phase) that will be prolonged until a steady-state is achieved (9, 32, 33). Since exercise is conducted at paces below or at the anaerobic threshold (AnT), the aerobic energy system supports (almost all) the energy requirements (13), the [La−] are low and the effort can be maintained for 30 min or longer (33, 34), with swimmers communicating a very light/light and moderate perception of effort (35–37).
Figure 1. Diagram of the oxygen uptake kinetics at different intensities, with the anaerobic threshold (AnT) and the maximum oxygen uptake (O2max) being identified. Values were obtained in front crawl swimming.
When the swimming intensity exceeds the physiological steady state and the swimmer cannot maintain body homeostasis, O2 fast component speeds up and the O2 plateau is no longer observed (32, 33, 38). This is a poorly known training zone that corresponds to the heavy intensity domain, displaying power outputs above the AnT and starting to cause a significant accumulation of [La−] over time (34, 39, 40). Here, a notable O2 slow component leads to an elevated O2 response (27, 32, 41) and exercise is expressed as hard to accomplish (28, 35). The appearance of this phenomenon, superimposed upon the O2 fast component, is due to the progressive recruitment and activation of fast twitch glycolic fibres (38, 42, 43), but this needs to be further studied particularly in swimming. The limit separating the heavy from the severe intensity domains is poorly defined in swimming, but the critical power is suggested for other cyclic sports [e.g., (44, 45)]. This has been debated since, by definition, critical power represents the exercise intensity that can be sustained without fatigue, with exhaustion occurring after 30–60 min of exercise (46).
In the severe intensity domain, the exercise intensity is significantly higher than at the AnT, and neither O2 nor [La−] can be stabilized (32, 40, 47), showing a pronounced magnitude compared with heavy intensity efforts (30, 32, 48). During constant pace exercises at this intensity, after an exuberant O2 fast component caused by the body's need for oxygen as the exercise proceeds, it continues moderately to increase until the point of exhaustion (11, 30, 45). In fact, the O2 slow component has been commonly reported for heavy (28, 38, 43) but mainly for severe swimming intensities (14, 41, 49) where its magnitude can exceed 1 L.min−1 and represent ≥25% of the total O2 increase above the pre-exercise baseline (50). At this exercise intensity domain, O2max is reached, reason why it corresponds to the aerobic power training zone (7), which is perceived as a very hard effort (35, 51). So, similarly to the AnT, the O2max is also a critical factor for establishing boundaries between intensity domains.
When swimming above the O2max intensity, i.e., at the extreme intensity domain (also known as the anaerobic capacity training zone), despite the O2-related studies conducted in field conditions are scarce, it was observed that O2 kinetics only present a fast component, rising in a very fast way and ending abruptly due to exhaustion (29, 41). This domain was the last to be proposed (52) and accounts for short duration and maximal intensity efforts at power outputs where exhaustion occurs before O2max is attained (41, 52). Here, there is not enough time to reach O2max, although the O2 obtained is of relevant magnitude for such short exercise durations (6, 29). This intensity domain is very scarcely studied in swimming, which does not make sense because the 50 m–200 m events (efforts in-between ∼20 s and 2 min duration) involve the use of different metabolic pathways compared to those involved in longer swimming events (5, 17, 53). [La−] are much more expressive than in previous intensity domains (41), with values of ∼16 mmol.L−1 at the 100 and 200 m exertions in anaerobically trained swimmers (53, 54). This expresses the use of the glycolysis at its maximal rate, with swimmers struggling until the end of the training set or competitive event with an extremely hard perceived exertion (35, 36).
HR assessment in swimming has been used to control the intensity of the training sets by the neck or wrist palpation during the first seconds of recovery (3). Since the carotid and radial arteries are not easily found, it is hard to count accurately and some rest periods are very short, swimming HR began to be frequently assessed using the easy-to-wear and low-cost telemetric HR monitors. Research-related data shows that HR remains stable at low and moderate swimming intensities within the first few min after the onset of exercise, similarly to O2 and [La−] behaviour (39, 55). In prolonged exercise, HR could progressively rise, particularly if at moderate intensities (34, 39). HR displays a fast rise at the beginning of heavy swimming intensities events, followed by a slight increase or progressive stabilization towards the end of exercise (55, 56). At severe exertions, after a first fast HR rise in the first min of exercise, a progressive levelling off seems to occur but with values significantly higher than in the previous intensity domain (18, 34, 43). At extreme swimming efforts, HR increases rapidly (∼90% of the maximum) during the initial stages of the bouts and climbs progressively toward the maximum as the efforts proceed (56, 57).
A key consideration when using O2, [La−] and HR data is that physiological responses should be related to swimming mechanics (16–18, 58). Since velocity is the product of SF and SL, researchers have always been interested in its assessment to observe if a measurable change in physiological stress-related variables may well reflect technical changes (11, 20). Early investigations on the topic in competitive swimming overestimated SL due to the assumption that it equalled the ratio between velocity and SF, calculating the former based on distance divided by time [e.g., (23, 24)] not accounting for the dive start, variations in mid-pool velocity and turning times. Later, SF and SL were calculated for every 25/50 m race partial in the clean swimming section using a chronofrequencemeter or video/computer analysis (59–61). It is well described that both variables change with intensity, with an increase of SF and a decrease of SL being particularly evident after the AnT intensity (8, 40, 61, 62). SF and SL changes in a non-linearly way (61–63), impacting on how to coordinate contralateral upper limb actions (64, 65). SI was defined as the product of the average swimming velocity and SL, and is considered a valid indicator of swimming efficiency (66). Due to text length constraints this variable will not be further explored but values are available elsewhere [e.g., (3)].
The upper limbs coordination is a fundamental aspect of swimmers' expertise, revealing catch-up, opposition and superposition distinctive coordination modes (<0, = 0 and >0, respectively) in front crawl (25). There is an IdC increase with the intensity rise (18, 65), progressing from catch-up to opposition and superposition coordination modes (18, 64). This evolution mirrors swimmers' adaptive responses to task-specific constraints, reflecting the intensity at which the task is executed (65, 67). There is a relationship between the general kinematical variables and the inter-arm coordination (67, 68), with data suggesting that energy requirements influence the biomechanical characteristics at the mentioned intensities (16, 58, 62, 65). Achieving optimal coordination needs synchronized movements of both upper and lower limbs to enhance velocities and efficiency, with the goal of attaining a 1:3 ratio between the number of upper and lower limb actions, regardless of velocity (69, 70). Consistency in limb coordination is paramount across successive cycles to maintain propulsion and body balance effects, being essential that the lower limb actions occur at the same relative time in each cycle (ensuring the consistent production of desired effects). The widely acknowledged optimal 1:3 frequency ratio emphasizes the importance of synchronized and coordinated actions, and signifies a smooth force-to-time profile, ultimately minimizing intra-cycle velocity variation (70).
Based on the dynamics of the physiological and biomechanical variables described in the above-referred literature, the following paragraphs, and our own knowledge and experience, different training zones are displayed in Table 1. It is possible to observe that the AnT (assessed metabolically using [La−] and the O2max (evaluated through ventilatory data) are critical factors for establishing boundaries between intensity domains. In this field, SF might serve to delineate the boundary between moderate and heavy intensity domains when [La−] is inaccessible (8, 40, 65) and HR combined with the rating of perceived exertion (RPE) can establish a boundary between heavy and severe intensity domains (35, 51, 62). When not disposing of ventilatory data, the 8 mmol.L−1 of [La−] might be used for limiting the upper boundary of the severe intensity domain since it is an average value commonly used for characterizing aerobic power swimming efforts (34, 57).
Table 1. Competitive swimming training zones (and corresponding methods and contents) based on commonly assessed physiologic and biomechanical variables (to be used, preferably, in populations of front crawl high-level swimmers).
When the aim is to target a specific intensity domain or training zone, certain considerations regarding training methods and contents should be recognized to better structure the swimming sets. For low and moderate intensities, the most effective approach is to employ continuous exercise with extended durations [or extensive interval training with short rests (66, 71, 72)], emphasizing the importance of maintaining high technical quality when maintaining a steady pace (14, 15, 22) or even with slight changes of intensity [e.g., the fartleck (3, 13)]. The development of aerobic capacity 1 and 2 are more relevant in early stages of the season and in younger/less experienced swimmers (58), aiming to increase the systemic oxidation capacity of pyruvic acid, lactic acid and lipids (7, 11). The aerobic capacity 1 training zone obtain high percentages of the total training volume since it is used for warming up and cooling down in each training session, as well as for promoting recovery between intense bouts (13, 22, 71). Aerobic capacity 3 implies increasing the intensity of the training sets, using the intensive interval training method (3) and, in the competitive period, breaking the so-called long-distance events in laps by employing the fractioned race pace training strategy (e.g., 15 × 100 m at 1,500 m pace with 10 s intervals).
The aerobic power training zone focuses on the traditionally called medium-distance races like the 400 m (26, 49) and aims to increase the transport, diffusion and peripheric perfusion of O2, as well as the mitochondrial capacity (73). The depletion of the muscle glycogen stores will not allow the training series to go over 10 min of duration if conducted at O2max pace (as it should). In the competitive period, the 400 m pace can be trained by making 4 × 100 m (10 s rest) at race pace (or even faster). Last but not least, the anaerobic power and capacity zones, as a fundamental focus of attention of competitive swimming (10, 11, 15), aims to activate the activity of glycolytic enzymes and reduce their sensitivity to metabolic acidosis by increasing buffering capacity (7, 71, 74). Since long and slow distance training can compromise sprint performance (11, 22), the swimmers’ engagement at extreme exertions needs to be maximal. Moreover, a wise definition of the rest period duration is the key to success since central and peripheral fatigue is a limiting factor of the training series continuity (3, 15). Early in the program, workouts should propose higher intensities for swimmers already fatigued and, later on, move on to conduct training sets in a fresh state to facilitate higher speeds (8, 58).
In the large spectrum of swimming intensities, the most well-known and manageable are those supported by the aerobic energy system, i.e., the low and moderate efforts (typically the 3–10 km open water events). However, above the AnT, exercise cannot be maintained for long due to the significative contribution of the anaerobic metabolism, with fast twitch fibres being progressively recruited (3, 13). Thus, fatigue will appear sooner or later when swimming at heavy intensities, with this being considered a “grey training zone” (where the 800 and 1,500 m races are included). The severe intensity domain is reached at exertions close to O2max paces, with the 400 m events being accepted as typical efforts. Exertions are very strenuous at the 50, 100, and 200 m competitive swimming events that are situated in the extreme intensity domain. Thus, anaerobic capacities are decisive to perform well and should be fully developed in addition to aerobic qualities (8, 10, 15). The evidence reported suggests that the behavior of relevant physiological variables should be well-considered when establishing training zones but always concurrently with upper limbs' general kinematics and coordination (1, 20). In fact, to succeed well, swimmers should not only focus on physical conditioning but also on developing technical skills (11, 22, 75). By combining training intensities, it is possible to optimize performance better, reduce injury risk and prevent overtraining, justifying the proposal of a new paradigm concerning the traditionally used training zones.
The original contributions presented in the study are included in the article/Supplementary Material, further inquiries can be directed to the corresponding author.
RJF: Writing – original draft, Writing – review & editing. DDC: Writing – original draft, Writing – review & editing. PF: Writing – original draft, Writing – review & editing.
The author(s) declare that no financial support was received for the research, authorship, and/or publication of this article.
RJF acknowledges Professor João Paulo Vilas-Boas for support and guidance during his academic career.
The authors declare that the research was conducted in the absence of any commercial or financial relationships that could be construed as a potential conflict of interest.
RJF and PF declared that they were an editorial board member of Frontiers, at the time of submission. This had no impact on the peer review process and the final decision.
All claims expressed in this article are solely those of the authors and do not necessarily represent those of their affiliated organizations, or those of the publisher, the editors and the reviewers. Any product that may be evaluated in this article, or claim that may be made by its manufacturer, is not guaranteed or endorsed by the publisher.
1. Nugent F, Comyns T, Kearney P, Warrington G. Ultra-short race-pace training (USRPT) in swimming: current perspectives. Open Access J Sports Med. (2019) 10:133–44. doi: 10.2147/OAJSM.S180598
2. Ogita F, Hara M, Tabata I. Anaerobic capacity and maximal oxygen uptake during arm stroke, leg kicking and whole body swimming. Acta Phvsiologica Scandinavia. (1996) 157:435–41. doi: 10.1046/j.1365-201X.1996.490237000.x
4. Zamparo P, Capelli C, Pendergast D. Energetics of swimming: a historical perspective. Eur J Appl Physiol. (2011) 111:367–78. doi: 10.1007/s00421-010-1433-7
5. Peyrebrune M, Toubekis A, Lakomy H, Nevill M. Estimating the energy contribution during single and repeated sprint swimming. Scand J Med Sci Sports. (2014) 24(2):369–76. doi: 10.1111/j.1600-0838.2012.01517.x
6. Massini DA, Almeida TAF, Macedo AG, Espada MC, Reis JF, Alves FJB, et al. Sex-specific accumulated oxygen deficit during short- and middle-distance swimming performance in competitive youth athletes. Sports Med Open. (2023) 9(1):49. doi: 10.1186/s40798-023-00546-8
7. Green HJ. Overview of the energy delivery systems. In: MacDougall JD, Wenger HA, editors. Physiological Testing of the Elite Athlete. New York, USA: Mouvement Publications Inc (1982). p. 3–6.
8. Sweetenham B. Maximizing a swimmer’s talent development. In: Hannula D, Thornton N, editors. The Swim Coaching Bible. USA: Human Kinetics (2001). p. 71–95.
9. Reis JF, Millet GP, Bruno PM, Vleck V, Alves FB. Sex and exercise intensity do not influence oxygen uptake kinetics in submaximal swimming. Front Physiol. (2017) 8:72. doi: 10.3389/fphys.2017.00072
10. Kabasakalis A, Mougios V. Biochemical monitoring of swimming training: old and new concepts. In: Fernandes RJ, editors. The Science of Swimming and Aquatic Activities. USA: Nova Science Publishers (2018). p. 79–93.
11. Costill DL, Maglischo EW, Richardson AB. Swimming. Oxford, UK: Blackwell Scientific Publications (1992).
12. Capelli C, Pendergast D, Termin B. Energetics of swimming at maximal speeds in humans. Eur J Appl Physiol. (1998) 78(5):385–93. doi: 10.1007/s004210050435
14. Sousa A. C., Vilas-Boas J. P., Fernandes R. J. VO2 Kinetics and metabolic contributions whilst swimming at 95, 100, and 105% of the velocity at VO2max. BioMed Res Int. (2014) 2014:675363. doi: 10.1155/2014/675363
15. Bottom M. Freestyle sprint training. In: Hannula D, Thornton N, editors. The Swim Coaching Bible. USA: Human Kinetics (2001). p. 209–19.
16. Barbosa TM, Bragada JA, Reis VM, Marinho DA, Carvalho C, Silva AJ. Energetics and biomechanics as determining factors of swimming performance: updating the state of the art. J Sci Med Sport. (2010) 13(2):262–9. doi: 10.1016/j.jsams.2009.01.003
17. Figueiredo P, Pendergast D, Vilas-Boas JP, Fernandes RJ. Interplay of biomechanical, energetic, coordinative and muscular factors in a 200 m front crawl swim. BioMed Res Int. (2013) 2013:897232. doi: 10.1155/2013/897232
18. Ribeiro J, Toubekis AG, Figueiredo P, de Jesus K, Toussaint HM, Alves F, et al. Biophysical determinants of front-crawl swimming at moderate and severe intensities. Int J Sports Physiol Perform. (2017) 12(2):241–6. doi: 10.1123/ijspp.2015-0766
19. di Prampero PE, Pendergast D, Wilson DW, Rennie DW. Energetics of swimming in man. J Appl Physiol. (1974) 37(1):1–5. doi: 10.1152/jappl.1974.37.1.1
20. Termin B, Pendergast DR. Training using the stroke-velocity relationship to combine biomechanical and metabolic paradigms. J Swim Res. (2000) 14:9–17.
21. Toussaint HM, Beek PJ. Biomechanics of competitive front crawl swimming. Sports Med. (1992) 13(1):8–24. doi: 10.2165/00007256-199213010-00002
23. East DJ. Swimming: an analysis of stroke frequency, stroke length, and performance. New Zealand J Health, Phys Educ Recreat. (1970) 3:16–7.
24. Craig A, Pendergast D. Relationships of stroke rate, distance per stroke, and velocity in competitive swimming. Med Sci Sports. (1979) 11:278–83.522640
25. Chollet D, Chalies S, Chatard JC. A new index of coordination for the crawl: description and usefulness. Int J Sports Med. (2000) 21(1):54–9. doi: 10.1055/s-2000-8855
26. Rodríguez F, Mader A. Energy metabolism during 400 m and 100 m crawl swimming: computer simulation based on free swimming measurement. In: Chatard JC, editors. Biomechanics and Medicine in Swimming IX. Saint-Etienne, France: Jean Monnet University (2003). p. 373–8.
27. Sousa A, Figueiredo P, Pendergast D, Kjendlie PL, Vilas-Boas JP, Fernandes RJ. Critical evaluation of oxygen uptake assessment in swimming. Int J Sports Physiol Perform. (2014b) 9(2):190–202. doi: 10.1123/ijspp.2013-0374
28. Reis VM, Marinho DA, Barbosa FP, Reis AM, Guidetti L, Silva AJ. Examining the accumulated oxygen deficit method in breaststroke swimming. Eur J Appl Physiol. (2010) 109:1129–35. doi: 10.1007/s00421-010-1460-4
29. Ribeiro J, Figueiredo P, Sousa A, Monteiro J, Pelarigo J, Vilas-Boas JP, et al. VO2 Kinetics and metabolic contributions during full and upper body extreme swimming intensity. Eur J Appl Physiol. (2015) 115:1117–24. doi: 10.1007/s00421-014-3093-5
30. Faina M, Billat V, Squadrone R, De Angelis M, Koralsztein JP, Dal Monte A. Anaerobic contribution to the time to exhaustion at the minimal exercise intensity at which maximal oxygen uptake occurs in elite cyclists, kayakists and swimmers. Eur J Appl Physiol. (1997) 76(1):13–20. doi: 10.1007/s004210050207
31. Carter H, Jones AM, Barstow TJ, Burnley M, Williams CA, Doust JH. Oxygen uptake kinetics in treadmill running and cycle ergometry: a comparison. J Appl Physiol. (2000) 89(3):899–907. doi: 10.1152/jappl.2000.89.3.899
32. de Jesus K, Sousa A, de Jesus K, Ribeiro J, Machado L, Rodríguez F, et al. The effects of intensity on v˙O2 kinetics during incremental free swimming. Appl Physiol Nutr Metabol. (2015) 40:918–23. doi: 10.1139/apnm-2015-0029
33. Pelarigo JG, Machado L, Fernandes RJ, Grecco CC, Vilas-Boas JP. Oxygen uptake kinetics and energy system’s contribution around maximal lactate steady state swimming intensity. PLoS One. (2017) 12(2):e0167263. doi: 10.1371/journal.pone.0167263
34. Fernandes RJ, Sousa M, Machado L, Vilas-Boas JP. Step length and individual anaerobic threshold assessment in swimming. Int J Sports Med. (2011) 32:940–6. doi: 10.1055/s-0031-1283189
35. Psycharakis SG. A longitudinal analysis on the validity and reliability of ratings of perceived exertion for elite swimmers. J Strength Condition Res. (2011) 25(2):420–6. doi: 10.1519/JSC.0b013e3181bff58c
36. Invernizzi PL, Longo S, Scurati R, Maggioni MA, Michielon G, Bosio A. Interpretation and perception of slow, moderate, and fast swimming paces in distance and sprint swimmers. Percept Mot Skills. (2014) 118(3):833–49. doi: 10.2466/27.29.PMS.118k23w0
37. Baldassarre R, Ieno C, Bonifazi M, Piacentini MF. Pacing and hazard score of elite open water swimmers during a 5-km indoor pool race. Int J Sports Physiol Perform. (2021) 16(6):796–801. doi: 10.1123/ijspp.2020-0197
38. Demarie S, Sardella F, Billat VL, Magini W, Faina M. TheVO2 slow component in swimming. Eur J Appl Physiol. (2001) 84:95–9. doi: 10.1007/s004210000348
39. Pelarigo J, Grecco CC, Denadai BS, Fernandes RJ, Vilas-Boas JP, Pendergast DR. Do 5% changes around maximal lactate steady state lead to swimming biophysical modifications? Hum Mov Sci. (2016) 49:258–66. doi: 10.1016/j.humov.2016.07.009
40. Carvalho DD, Soares S, Zacca R, Sousa J, Marinho DA, Silva AJ, et al. Anaerobic threshold biophysical characterisation of the four swimming techniques. Int J Sports Med. (2020) 41(5):318–27. doi: 10.1055/a-0975-9532
41. Monteiro AS, Magalhães JF, Knechtle B, Buzzachera CF, Vilas-Boas JP, Fernandes RJ. Acute ventilatory responses to swimming at increasing intensities. PeerJ. (2023) 11:e15042. doi: 10.7717/peerj.15042
42. Zoladz JA, Korzeniewski B. Physiological background of the change point in VO2 and the slow component of oxygen uptake kinetics. J Physiol Pharmacol. (2001) 52(2):167–84.11453098
43. Reis JF, Alves FB, Bruno PM, Vleck V, Millet GP. Effects of aerobic fitness on oxygen uptake kinetics in heavy swimming intensity. Eur J Appl Physiol. (2012) 112(5):1689–7. doi: 10.1007/s00421-011-2126-6
44. Jones AM, Vanhatalo A, Burnley M, Morton RH, Poole DC. Critical power: implications for determination of O2max and exercise tolerance. Med Sci Sports Exercise. (2010) 42(10):1876–90. doi: 10.1249/MSS.0b013e3181d9cf7f
45. Pessoa Filho DM, Alves FB, Reis JF, Grecco CC, Denadai BS. VO2 Kinetics during heavy and severe exercise in swimming. Int J Sports Med. (2012) 33(9):744–8. doi: 10.1055/s-0031-1299753
46. Hill DW. The critical power concept: a review. Sports Medicine. (1993) 16(4):237–54. doi: 10.2165/00007256-199316040-00003
47. Gaesser GA, Poole D. The slow component of oxygen uptake kinetics in humans. Exerc Sport Sci Rev. (1996) 24:35–71. doi: 10.1249/00003677-199600240-00004
48. Pringle J, Doust J, Carter H, Tolfrey K, Campbell I, Jones A. Oxygen uptake kinetics during moderate, heavy, and severe intensity “submaximal” exercise in humans: the influence of muscle fiber type and capillarisation. Eur J Appl Physiol. (2003) 89:289–300. doi: 10.1007/s00421-003-0799-1
49. Fernandes RJ, Keskinen KL, Colaço P, Querido AJ, Machado LJ, Morais PA, et al. Time limit at O2max velocity in elite crawl swimmers. Int J Sports Med. (2008) 29(02):145–50. doi: 10.1055/s-2007-965113
50. Poole DC, Barstow TJ, Gaesser GA, Willis WT, Whipp BJ. O2 slow component: physiological and functional significance. Med Sci Sports Exercise. (1994) 26(11):1354–8.
51. Ueda T, Kurokawa T. Relationships between perceived exertion and physiological variables during swimming. Int J Sports Med. (1995) 16(6):385–9. doi: 10.1055/s-2007-973025
52. Hill DW, Poole DC, Smith JC. The relationship between power and the time to achieve O2max. Med Sci Sports Exercise. (2002) 34:709–14. doi: 10.1097/00005768-200204000-00023
53. Olbrecht J. The science of winning: Planning, periodizing and optimizing swim training. Luton, England: Helen Layton Swimshop (2000).
54. Vescovi JD, Falenchuk O, Wells G. Blood lactate concentration and clearance in elite swimmers during competition. Int J Sports Physiol Perform. (2011) 6:106–17. doi: 10.1123/ijspp.6.1.106
55. Treffene RJ. Heart rate measurement technique in swimming performance prediction. In: Hollander AP, Huijing PA, de Groot G, editors. Biomechanics and Medicine in Swimming. USA: Human Kinetics (1983). p. 339–44.
56. Magel JR, McArdle WD, Glaser RM. Telemetered heart rate response to selected competitive swimming events. J Appl Physiol. (1969) 26(6):764–70. doi: 10.1152/jappl.1969.26.6.764
57. Zacca R, Fernandes RJ, Pyne DB, Castro FA. Swimming training assessment: the critical velocity and the 400-m test for age-group swimmers. J Strength Condition Res. (2016) 30(5):1365–72. doi: 10.1519/JSC.0000000000001239
58. Pyne DB, Goldsmith WM. Training and testing of competitive swimmers. In: Stager JM, Tanner DA, editors. Handbook of Sports Medicine And Science: Swimming, 2nd edn. Oxford, UK: Blackwell Publishing Ltd (2005). p. 128–43.
59. Keskinen KL, Komi PV. Stroking characteristics of front crawl swimming during exercise. J Appl Biomech. (1993) 9:219–26. doi: 10.1123/jab.9.3.219
60. Arellano R, Brown P, Cappaert J, Nelson R. Analysis of 50-, 100- and 200-m freestyle swimmers at the 1992 Olympic games. J Appl Biomech. (1994) 10:189–99. doi: 10.1123/jab.10.2.189
61. Psycharakis SG, Cooke CB, Paradisis GP, O'Hara J, Phillips G. Analysis of selected kinematic and physiological performance determinants during incremental testing in elite swimmers. J Strength Condition Research. (2008) 22(3):951–7. doi: 10.1519/JSC.0b013e31816a6364
62. Monteiro AS, Carvalho DD, Elói A, Silva F, Vilas-Boas JP, Buzzachera CF, et al. Repeatability of ventilatory, metabolic and biomechanical responses to an intermittent incremental swimming protocol. Physiol Meas. (2022) 43(7):10. doi: 10.1088/1361-6579/ac7c51
63. Dekerle J, Nesi X, Lefevre T, Depretz S, Sidney M, Marchand H, et al. Stroking parameters in front crawl swimming and maximal lactate steady state speed. Int J Sports Med. (2005) 26:53–8. doi: 10.1055/s-2004-817854
64. Seifert L, Boulesteix L, Chollet D. Effect of gender on the adaptation of arm coordination in front crawl. Int J Sports Med. (2004) 25(3):217–23. doi: 10.1055/s-2003-45253
65. Figueiredo P, Morais P, Vilas-Boas JP, Fernandes RJ. Changes in arm coordination and stroke parameters on transition through the lactate threshold. Eur J Appl Physiol. (2013b) 113:1957–64. doi: 10.1007/s00421-013-2617-8
66. Costill DL, Kovaleski J, Porter D, Kirwan J, Fielding R, King D. Energy expenditure during front crawl swimming: predicting success in middle-distance events. Int J Sports Med. (1985) 6(05):266–70. doi: 10.1055/s-2008-1025849
67. Seifert L, Chollet D, Rouard A. Swimming constraints and arm coordination. Hum Mov Sci. (2007) 26(1):68–86. doi: 10.1016/j.humov.2006.09.003
68. Alberty M, Potdevin F, Dekerle J, Pelayo P, Gorce P, Sidney M. Changes in swimming technique during time to exhaustion at freely chosen and controlled stroke rates. J Sports Sci. (2008) 26(11):1191–200. doi: 10.1080/02640410801974984
69. Guignard B, Rouard A, Chollet D, Bonifazi M, Dalla Vedova D, Hart J, et al. Upper to lower limb coordination dynamics in swimming depending on swimming speed and aquatic environment manipulations. Motor Control. (2019) 23(3):418–42. doi: 10.1123/mc.2018-0026
70. Mezêncio B, Pinho JP, Huebner R, Vilas-Boas JP, Amadio AC, Serrão JC. Overall indexes of coordination in front crawl swimming. J Sports Sci. (2020) 38(8):910–7. doi: 10.1080/02640414.2020.1737349
71. Harre D. Principles of Sports Training: Introduction to the Theory and Methods of Training. Germany: Sportverlag (1982).
72. Gastin PB. Energy system interaction and relative contribution during maximal exercise. Sports Medicine. (2001) 31(10):725–41. doi: 10.2165/00007256-200131100-00003
73. Stager JM, Coyle MA. Energy systems. In: Stager JM, Tanner DA, editors. Handbook of Sports Medicine and Science: Swimming, 2nd edn. Oxford, UK: Blackwell Publishing Ltd (2005). p. 1–19.
74. Rohrs DM, Stager JM. Evaluation of anaerobic power and capacity in competitive swimmers. J Swim Res. (1991) 7(3):12–6.
Keywords: physiology, biomechanics, intensity, swimmer, training areas
Citation: Fernandes RJ, Carvalho DD and Figueiredo P (2024) Training zones in competitive swimming: a biophysical approach. Front. Sports Act. Living 6:1363730. doi: 10.3389/fspor.2024.1363730
Received: 31 December 2023; Accepted: 4 March 2024;
Published: 18 March 2024.
Edited by:
Rafael Oliveira, Polytechnic Institute of Santarém, PortugalReviewed by:
Rui Miguel Silva, Instituto Politécnico de Viana do Castelo, Portugal© 2024 Fernandes, Carvalho and Figueiredo. This is an open-access article distributed under the terms of the Creative Commons Attribution License (CC BY). The use, distribution or reproduction in other forums is permitted, provided the original author(s) and the copyright owner(s) are credited and that the original publication in this journal is cited, in accordance with accepted academic practice. No use, distribution or reproduction is permitted which does not comply with these terms.
*Correspondence: Pedro Figueiredo cGZpZ3VlaXJlZG9AdWFldS5hYy5hZQ==
Disclaimer: All claims expressed in this article are solely those of the authors and do not necessarily represent those of their affiliated organizations, or those of the publisher, the editors and the reviewers. Any product that may be evaluated in this article or claim that may be made by its manufacturer is not guaranteed or endorsed by the publisher.
Research integrity at Frontiers
Learn more about the work of our research integrity team to safeguard the quality of each article we publish.