- 1School of Kinesiology, Western University, London ON, Canada
- 2Department of Kinesiology, California State University, Bakersfield, CA, United States
- 3Department of Kinesiology, California State University, Fullerton, CA, United States
Introduction: Running related injuries (RRI) are common, but factors contributing to running performance and RRIs are not commonly compared between different types of runners.
Methods: We compared running biomechanics previously linked to RRIs and performance between 27 recreational and 35 collegiate runners. Participants completed 5 overground running trials with their dominant limb striking a force plate, while outfitted with standardised footwear and 3-dimensional motion capture markers.
Results: Post hoc comparisons revealed recreational runners had a larger vertical loading rate (194.5 vs. 111.5 BW/s, p < 0.001) and shank angle (6.80 vs. 2.09, p < 0.001) compared with the collegiate runners who demonstrated greater vertical impulse (0.349 vs. 0.233 BWs, p < 0.001), negative impulse (−0.022 vs. −0.013 BWs, p < 0.001), positive impulse (0.024 vs. 0.014 BWs, p < 0.001), and propulsive force (0.390 vs. 0.333 BW, p = 0.002). Adjusted for speed, collegiate runners demonstrated greater total support moment (TSM), plantar flexor moment, knee extensor moment, hip extensor moment, and had greater proportional plantar flexor moment contribution and less knee extensor moment contribution to the TSM compared with recreational runners. Unadjusted for speed, collegiate runners compared with recreational had greater TSM and plantar flexor moment but similar joint contributions to the TSM.
Discussion: Greater ankle joint contribution may be more efficient and allow for greater capacity to increase speed. Improving plantarflexor function during running provides a strategy to improve running speed among recreational runners. Moreover, differences in joint kinetics and ground reaction force characteristics suggests that recreational and collegiate runners may experience different types of RRI.
Introduction
Running is a popular form of physical activity with global participation (1). For some, running serves as a recreational activity, and others participate as competitive athletes. Various health benefits result from running including a reduced risk of cardiovascular disease, and overall healthier lifestyle (2). Despite the health benefits from participation, running has a high injury incidence rate (3, 4). For example, 37%–63% of those who participate in running suffer from some form of running-related injury (RRI) annually (3, 4). Some RRIs persist and could have lasting outcomes that impact runners' ability to participate, their health, and quality of life (5).
Most RRIs result from overuse and repetitive loading on the lower body (6, 7). Running is repetitive and involves cyclical mechanical loading applied to the lower extremity. Overuse of the lower limb structures in combination with potential predisposing risk factors contribute to the likelihood of injury incidence (7). Behavioural differences between recreational and competitive runners (e.g., quantity, frequency, intensity) also contribute to an increased risk of an RRI (8–12). For instance, a recreational runner has been defined as someone who runs a minimum of 3 times per week and totals 16 kilometers of running during the week (11). Comparatively, competitive runners run more frequently, and accumulate more than 100 km within a week (8, 10). The difference in exposure between different types of runners may contribute to injury susceptibility.
Running biomechanics also influence injury risk and performance. For instance, high performance runners have a longer flight phase compared with recreational runners due to faster velocity, and larger propulsive force during terminal stance (13, 14). Moreover, a lower step rate increases the risk of bone stress injury in collegiate cross-country runners (15), which may differ between different types of runners. Furthermore, lower limb position at foot contact has been investigated for its influence on injury and performance (16). A more perpendicular shank relative to the ground and closer contacts to the body's center of mass allows for greater running economy by decreasing the braking force at ground contact (16–18). A higher braking force decreases running performance and also increases risk of RRI (16, 18).
Additionally, other factors such as lower limb stiffness, ground reaction forces (GRF), and propulsion forces differ between runners and contribute to RRI and performance indicators (e.g., running speed and efficiency) (16, 17). The summed action of the ankle, knee, and hip extensors [i.e., total support moment (TSM)] contribute to propulsion during running (19). Moreover, the ankle plantarflexors contribute the largest component of positive work compared with the knee and hip extensors (20). However, these findings were in controls at relatively slower speeds, and it is unclear how joint work distribution differs between groups running at more typical training paces. Deficiency in the ankle plantarflexor moment and reliance on the knee and hip extensors in recreational runners may contribute to performance deficits and slower self-selected speeds when participating in long distance running (20). Comparisons of recreational and competitive runners elucidates optimal biomechanical strategies for performance given the known differences in self-selected running speeds.
The purpose of this study was to compare running biomechanics that have previously been linked to RRI and performance between recreational and collegiate runners. We hypothesized that collegiate runners would have more perpendicular shank angles and larger GRFs than their recreational counterparts. We also hypothesized that all lower extremity extensor moments would be higher in the collegiate group with the proportion of ankle moment as a percent of the TSM also higher in the collegiate group. A secondary purpose was to evaluate the association between GRFs and shank angle during running in recreational and collegiate runners. We hypothesized that a larger shank angle would be associated with a larger braking force in both groups.
Materials and methods
Data used for this study were collected as part a larger study examining the association between running kinetics and femoral cartilage characteristics (21). All methods were approved by the university's institutional review board and participants provided informed written consent, before a single data collection session that lasted approximately 2-hours.
Participants
Twenty-seven recreational and 35 collegiate runners were recruited from the university cross country team, student population, and local running groups. The criterion for being defined as a collegiate runner was determined to be currently running or running in the preceding year for an intercollegiate team (22). A recreational runner was considered to be a person running up to 3 times a week for a minimum of 10 miles (16 km) within that week (11, 21). All participants were between the ages of 18 and 35 and required to be free from lower body injuries for 6 months before participation. Further exclusion from participation included a history of lower body intra articular injections, surgery, and a body mass index (BMI) greater than 25.0 kg/m2.
Running biomechanics
All participants were instructed to wear compression shorts for bottoms and either a tank top or sports bra for women and a compression shirt or shirtless for men. Standardized footwear (Nike Pegasus 32, Beaverton, OR) were provided by the laboratory to mitigate the influence of footwear being a confounding variable on running kinetics. Single retroreflective markers were placed on the posterior superior iliac spine, iliac crest, anterior superior iliac crest, greater trochanter, medial and lateral femoral epicondyles, medial and lateral malleoli, heel counter, and 1st and 5th metatarsals, while rigid clusters of 4 markers were placed on the thigh, shank, and foot. All markers were placed solely on the dominant limb. Three-dimensional running biomechanics were collected using a 20-m runway equipped with a 9-camera motion capture system recording at 240 Hz (Qualisys, Gothenburg, Sweden) and a force plate recording at 2,400 Hz (Advanced Mechanical Technology, Inc, Watertown, MA) positioned in the middle of the runway.
Upon completing a self-selected 5-min running warm up on a treadmill, participants completed 5 overground familiarization trials. Familiarization was used to ensure that participants could strike the force plate without noticeably altering their stride, and that a consistent running pace was achieved. Self-selected running speed was monitored with infrared timing gates (model TF100; TracTronix, Belton, MO) 2-m apart. Participants completed 5 trials with their dominant limb contacting the force plate and within ±5% of the self-selected speed obtained from familiarization trials.
Data reduction
Visual 3D (C-Motion, Inc, Germantown, MD) was used for model construction. Marker trajectories and GRFs were low pass filtered at 20 Hz (23). One fourth of the intertrochanteric distance was used to estimate the hip joint center. The midpoints between the femoral epicondyles and lateral and medial malleoli were used to determine centers for the knee and ankle, respectively. Ground contact and toe-off were identified when the vertical GRF exceeded and fell below 20N, respectively, and used to determine stance phase. The strike angle was calculated as the absolute angle of a modified virtual foot segment relative to the global coordinate system to describe the footstrike pattern of the sample (24). The long axis of the foot was offset from the heel counter and distal foot markers so that the virtual foot segment was parallel to the floor during the calibration trial (24). A rearfoot strike was categorized as >8°, a midfoot strike was categorized as between −1.6° and 8°, and a forefoot strike was categorized as <−1.6° (25). The shank angle was determined relative to the global coordinate system where 0° was considered perpendicular to the ground and was extracted at the time of ground contact.
The vertical loading rate was defined as the peak derivative during the first 13% of the stance phase (22, 26), and normalized to body weight. This method allowed for comparison of loading rates between runners with various footstrike patterns who do not have an impact peak, which is common in runners with forefoot strike pattern. The peak posterior and anterior GRFs were extracted from the first and second halves of the stance phases, respectively. Impulse from the vertical and anterior-posterior GRF components was extracted using trapezoidal integration. The positive to negative ratio was determined by dividing the positive impulse by the negative impulse. Vertical loading rate and peak GRF and impulse characteristics were normalized to body weight.
Inverse dynamics procedures were used to derive internal hip, knee, and ankle joint moments resolved in the proximal segment coordinate system. The TSM peak values and total contributions were expressed as percentages taken at the time of peak TSM. TSM is calculated using the algebraic sum of the sagittal plane hip, knee, and ankle extensor moments (19). All extensor moments were reported as normalized to participants' height and body weight, and as percentage of TSM. Extensor moments and GRFs were time-normalized to 101 data points and plotted as ensemble average with 95% confidence interval for visualization purposes (Figure 1).
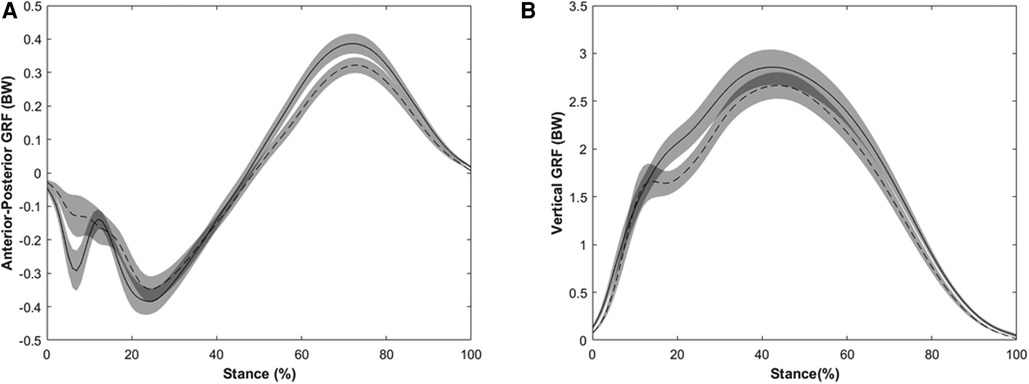
Figure 1. Ensemble average and 95% confidence interval for (A) vertical ground reaction force and (B) anterior-posterior ground reaction force. Solid indicates collegiate and dashed indicates recreational runners. Collegiate runners had a larger vertical impulse, propulsive force, negative impulse, positive impulse compared with recreational runners. However, recreational runners had a larger vertical LR and shank angle compared with collegiate runners.
Statistical analysis
All statistical analyses were completed using SPSS version 28.0 (IBM Corp., Armonk NY). Box plots were used to assess for outliers (>1.5× Interquartile Range) and extreme outliers (3× Interquartile Range) and normality was determined using the Shapiro-Wilk test separately for each group. The proportion of males and females, and proportion of rearfoot/midfoot/forefoot strikers were compared between groups using χ2 tests, and all other demographic characteristics were compared using independent samples t-tests. Ground reaction force characteristics and extensor moments were compared between groups using separate one-way multivariate analyses of variance, and post hoc comparisons were conducted using independent samples t-tests with Bonferroni correction (Family-wise α = 0.05). To further understand joint-level contributions to the TSM and propulsion between groups, we also compared extensor moments adjusted for self-selected speed as a co-variate. Pearson correlation was used to assess the relationship between shank angle and GRF characteristics. Correlation coefficients (r) were interpreted as poor (<0.3), fair (0.3–0.5), moderately strong (0.6–0.8) and very strong (>0.8) (27, 28).
Results
Descriptive statistics of participants demographic information can be found in Table 1. The recreational runners were older than the collegiate runners. The collegiate group reported greater amount of running and ran at a greater self-selected running speed compared with the recreational group. The collegiate group also had a greater proportion of midfoot and smaller proportion of rearfoot strike runners compared with the recreational group.
Ground reaction force comparison
One-way multivariate analysis of variance revealed that there was a significant difference in GRF and shank outcomes between groups [Pillai's trace = 0.739, F(51,10) = 14.415, p < 0.001, Figure 1]. Post hoc comparisons demonstrated recreational runners had a larger vertical loading rate, and shank angle compared with the collegiate runners who demonstrated greater vertical impulse, negative impulse, positive impulse, and propulsive force (Table 2).
Joint moment comparison
When unadjusted for speed, normalized extensor moments differed between groups [Pillai's trace = 0.204, F(57,4) = 3.648, p = 0.01, Figures 2, 3]. Post hoc comparisons showed the collegiate group had a greater TSM (p = 0.002) and plantarflexor moment (p = 0.029) compared with recreational runners but no difference in the knee extensor moment (p = 0.984) and hip extensor moment (p = 0.064) (Table 4).
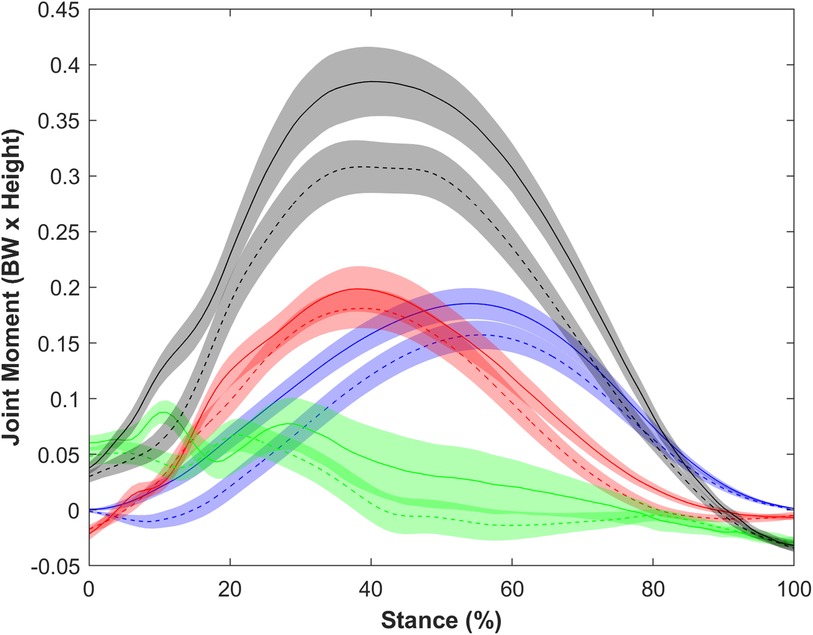
Figure 2. Ensemble average and 95% confidence interval for all extensor moments. Black indicates total support moment, green indicates hip extensor moment, red indicates knee extensor moment, and blue indicates plantarflexor moment. Solid indicates collegiate runners, and dashed indicates recreational runners. All joint moments were higher in collegiate compared with recreational runners.
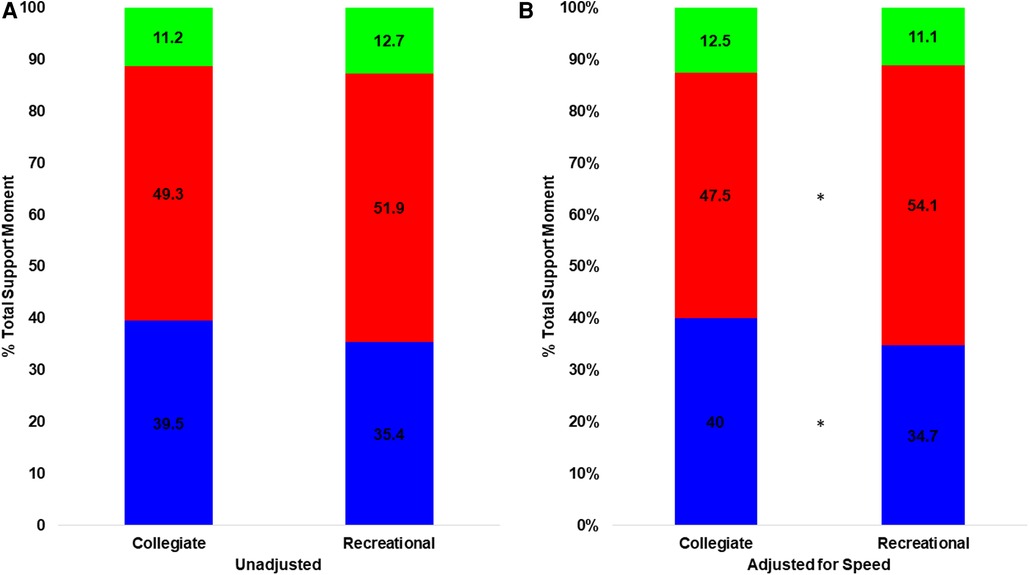
Figure 3. Joint moment distribution when (A) unadjusted and (B) adjusted for speed (blue is plantarflexor moment, red is knee extensor moment, green is hip extensor moment). *Indicates significant difference between groups. The plantarflexor and knee extensor moments differed between groups only when adjusted for speed.
When adjusted for speed, the normalized extensor moments differed between groups [Pillai's trace = 0.342, F(58,4) = 7.5421, p < 0.001, Figure 3]. Post hoc comparisons between the recreational and collegiate group indicated that the TSM (p < 0.001), plantar flexor moment (p = 0.004), knee extensor moment (p = 0.033), and hip extensor moment (p = 0.040) were greater in collegiate runners (Table 4).
When unadjusted for speed, the individual joint contributions to the TSM did not differ between groups [Pillai's trace = 0.073, F(60,2) = 2.349, p = 0.104].
When adjusted for speed, the contributions to TSM differed between groups [Pillai's trace = 0.106, F(59,2) = 3.503, p = 0.037], and the collegiate group had a greater plantar flexor moment (p = 0.037) and lower knee extensor moment (p = 0.018) compared with the recreational group (Table 4).
Correlational analyses
Significant and fair correlations (Figure 4, Table 3) were found between a less perpendicular shank angle relative to the ground and greater positive impulse (r = −0.403, p = 0.016), a smaller negative impulse (r = −0.362, p = 0.033), and greater positive-to-negative impulse ratio (r = −0.424, p = 0.011) in the collegiate group. A greater positive-to-negative impulse ratio was associated with a less perpendicular shank angle relative to the ground (r = −0.400, p = 0.038) in the recreational group. No other significant correlations were identified between shank angle and GRF characteristics in either group (all p > 0.05, Table 3).
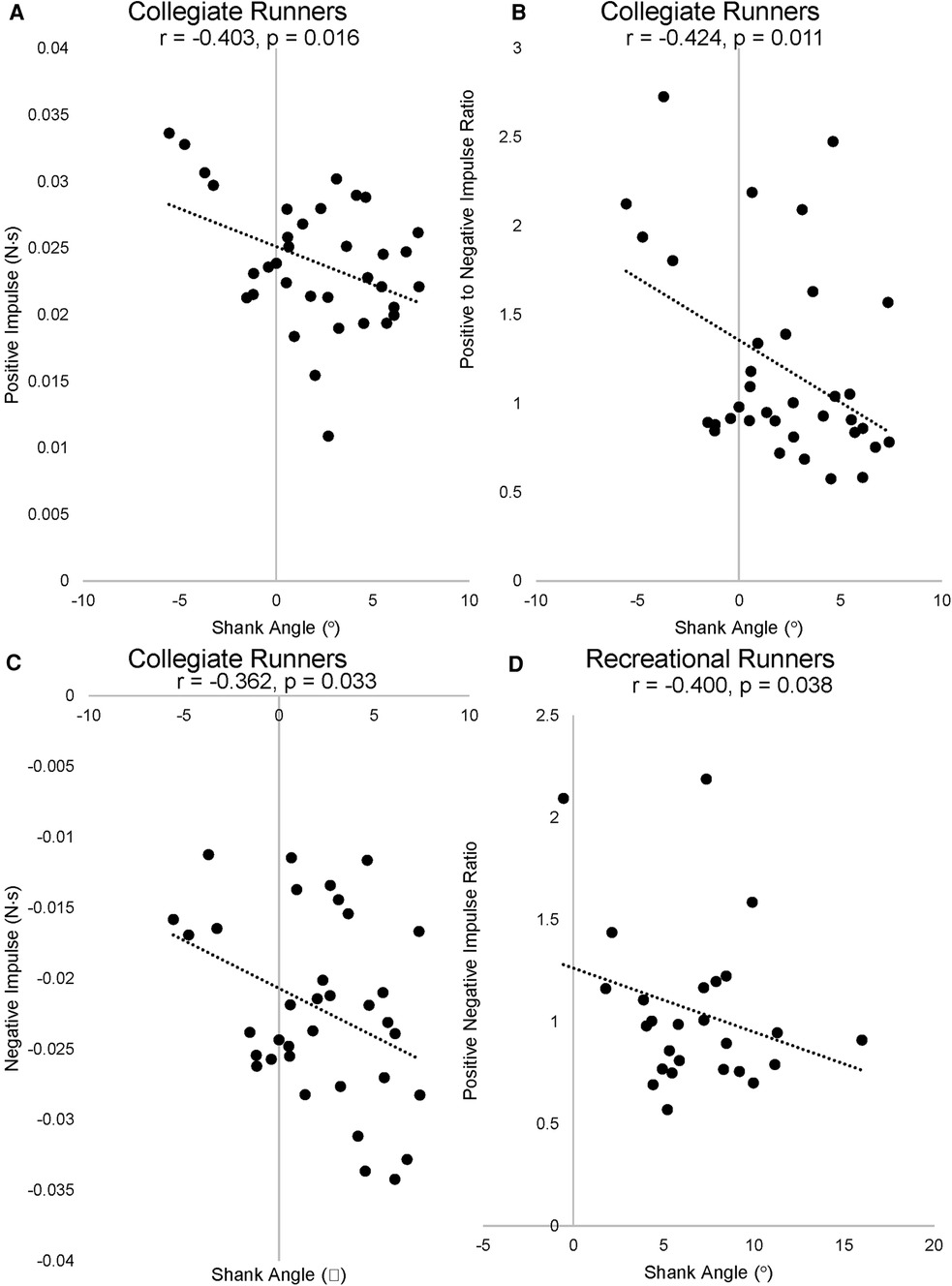
Figure 4. Scatterplots for significant associations between (A) shank angle and positive impulse in collegiate runners, (B) shank angle and positive to negative impulse ratio in collegiate runners, (C) shank angle and negative impulse in collegiate runners, and (D) positive to negative impulse ratio in recreational runners.

Table 3. Correlations (r) between shank angle at ground contact and ground reaction force characteristics (*p < 0.05).
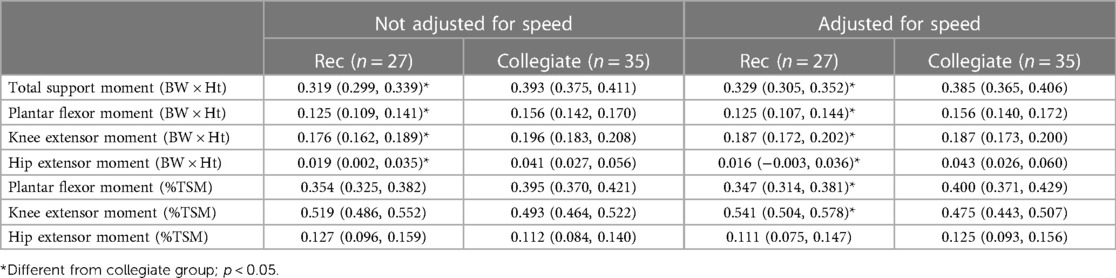
Table 4. Mean (95% confidence interval) of TSM, plantar flexor moment, knee extensor moment, and hip extensor moment controlled for body weight and height; and the plantar flexor moment, knee extensor moment, and hip extensor moment as a percentage of TSM for collegiate and recreational groups adjusted and unadjusted for speed.
Discussion
The primary purpose of this study was to compare injury- and performance- related running mechanics between recreational and collegiate runners. We further examined the association between ground contact and GRF characteristics within each group. The main findings indicated that collegiate runners used a larger proportion of the ankle extensors, but smaller proportion of the knee extensors compared with recreational runners. Moreover, collegiate runners had larger propulsive features (e.g., anterior force, vertical impulse) compared with recreational runners, and a more vertically oriented shank at ground contact (Figure 5). A more vertically oriented shank was associated with a larger propulsive impulse and smaller braking impulse.
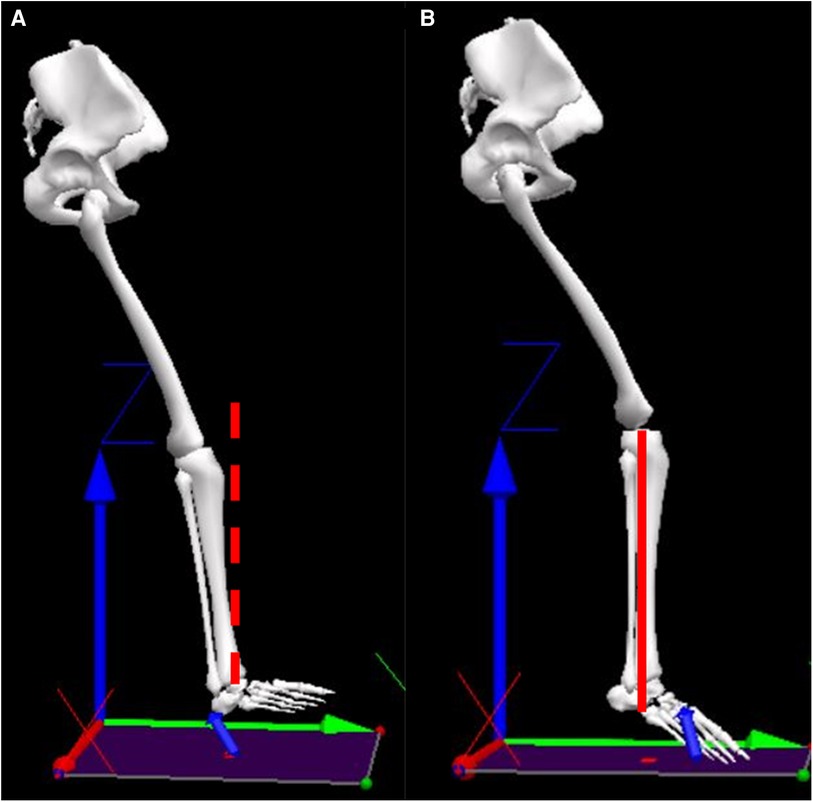
Figure 5. Representative figure of (A) recreational runner and (B) collegiate runner where the shank angle is larger versus upright, respectively.
We hypothesized that the joint moment distribution would differ between collegiate and recreational runners. Adjusting for speed, we found that the collegiate runners used a larger proportion of ankle extensor moment compared with recreational runners. The ankle plantar flexors are a large contributor to propulsion during running, and comprise the largest proportion of lower extremity joint work compared with the knee or hip extensors (20). Moreover, there is a distal to proximal shift in joint work during gait as speed increases from walking to sprinting (20, 29). As such, these findings suggest that collegiate runners use a more efficient strategy when speed is controlled that uses the ankle plantar flexors for propulsion. Moreover, the ability to utilize a larger proportion of the ankle plantar flexors when adjusting for speed likely increases their maximal running speed capacity relative to recreational runners and allow them to run at faster sub-maximal speeds for longer durations. Ankle power generation increases with faster running speeds and contributes to propulsion in recreational runners (14). Therefore, improving ankle plantar flexor function to reduce reliance on the hip and knee extensors during running may be a strategy to improve running performance in recreational runners and achieve similar self-selected speeds as collegiate runners during prolonged running.
The recreational runners had a larger knee extensor moment expressed as a percentage of TSM compared with collegiate runners when comparisons were adjusted for speed. A larger internal knee extensor moment contributes to greater patellofemoral contact stress (30, 31). As such, recreational runners utilize a running strategy that contributes to a disproportionate load on the patellofemoral joint that elevates risk for anterior knee pain (30, 32). Recreational runners may reduce their risk of injury from increasing the proportion of contribution from the ankle and hip joint extensor moments to the TSM. This contributes to reductions in knee extensor moment needed during running and decrease load to the patellofemoral joint. Conversely, collegiate runners may experience foot/ankle pathologies due to additional reliance on the ankle plantar flexors (33–35).
The collegiate runners in our sample had higher extensor moments but similar distribution of moments at every joint compared with recreational runners when comparisons were unadjusted for speed. These findings were expected and likely contributed to the overall difference in anterior force and self-selected running speed. Collegiate runners may have greater muscle capacity for joint extension from completing a larger training volume or supplementary resistance training (12, 36). These findings were supported by GRF comparisons, and collegiate runners who had greater propulsive force, and positive impulse compared with recreational runners. Supplementary forms of strength training with endurance training improves running economy, power, reactive strength, and running performance in collegiate runners (36). Therefore, improvements in running performance could be achieved in recreational runners by participating in supplementary resistance training similar to collegiate runners. Supplementary training may increase their muscle capacity at all joints for propulsive forces and positive impulse to the values that we observed in the collegiate group. Future research may aim to investigate if the additional of strength training to recreational runners could improve running speed.
We also found that recreational runners had higher vertical loading rates compared with collegiate runners when normalized to body weight. The vertical loading rate has been retrospectively (37), and prospectively (38), linked to tibial stress fracture (39, 40). However, these studies have largely been limited to recreational runners. In conjunction with our data, recreational runners may be at greater risk for stress fracture from elevated vertical loading rates. The vertical loading rate is strongly influenced by speed and foot strike pattern (41). As running speed increases, the vertical loading rate increases (41). Faster running speed also contribute to shift from rearfoot to a more anterior foot strike pattern for some runners (41). A more anterior foot strike pattern has been associated with reduced vertical loading rate compared with rearfoot contact at the same running speed (41). Collegiate runners in our sample ran faster and had a lower proportion of rearfoot strike runners. As such, faster speed and higher loading rates may have been mitigated by running with a more forefoot pattern (41). Collectively, these data suggest that collegiate and recreational runners have different biomechanics that contribute to unique injury patterns, and prospective data are needed to evaluate these hypotheses.
We also examined the association between shank kinematics at ground contact and GRF characteristics and found that a more vertically oriented shank was associated with less negative impulse and more positive impulse. The braking/posterior force has been prospectively linked to running-related injury (42), and a positive impulse is linked to faster running speeds (43). A prospective study on female recreational runners found that injury incidence was 8 times higher for runners with peak braking forces 0.27× body weight compared with 0.23× body weight (42). Moreover, a retrospective study found limbs with previous tibial stress fractures to demonstrate greater peak braking force values (44). Bone is stronger under compressive forces rather than shear force (45). Increased braking force could contribute to shear forces and greater bone-stress injury risk (44, 46). Furthermore, faster runners can apply 1.26 times greater average force per body weight in less time on the ground compared with slower runners, achieving 1.8 times faster top speeds (43). Elite runners have greater vertical forces 0.16 BW higher, and more vertical shank angle at ground contact compared with sub-elite runners (17). Therefore, our findings suggest that manipulating shank position presents a viable target for gait modification that may influence both injury risk and running performance. A more vertical shank angle at ground contact has been associated with better running performance and minimizes horizontal braking, which has been associated with better running economy and performance in distance runners (16). Furthermore, between laps one and two of an 800 m, the faster of the two laps presented a more vertical shank angle at ground contact in female distance runners at the world athletics championships (47). Our findings of a more vertically oriented shank in the collegiate group compared with recreational therefore, could be a biomechanical contributor to their faster running speeds. However, we note that our sample included some collegiate runners whose shank angles were slightly negative (i.e., leaned forward) rather than perpendicular, which may not be ideal. Moreover, correlation coefficients were only fair, and left a large portion of unexplained variance in GRF characteristics. Therefore, future studies should evaluate optimal shank angle during ground contact for both performance and injury prevention purposes.
Results of this study should be interpreted in the context of its limitations. Firstly, the cross-sectional study design negates the ability to evaluate if running mechanics are a result of different training magnitudes and behaviours of recreational and collegiate runners. Nonetheless, we still identified differences in running biomechanics that may contribute to the difference in ability of recreational and collegiate runners. Prospective data are needed to evaluate if running patterns change as a result of habitual training and can be manipulated via interventions (e.g., supplemental resistance training, biofeedback etc.). Secondly, we only examined self-selected running speeds to approximate habitual running. Our comparisons that statistically adjusted for running speed found differences in ankle and knee extensor contributions to the TSM that may influence propulsion. However, comparisons at matched speeds may further elucidate unique locomotive strategies that differentiate collegiate and recreational runners.
Conclusion
Collegiate runners compared with recreational runners demonstrated greater propulsion and a more vertically oriented shank that was associated with higher propulsive impulse and lower braking impulse. As such, runners could reduce braking forces that have previously shown association to tibial stress fractures by running with a more vertically oriented shank angle (42, 44, 46). A more vertical shank could also contribute to faster running through greater propulsion and less braking. Therefore, future studies may investigate the influence of altering shank angles on running performance and injury risk. Collegiate runners also used a greater proportion of the ankle extensors and smaller proportion of knee extensors than recreational runners when adjusted for speed. A greater contribution from the ankle joint compared with knee and hip at slower speeds may provide a more efficient propulsion strategy that allows for a larger capacity to increase running speed. Therefore, recreational runners may be able to achieve faster running speeds through greater proportional use of their ankle plantar flexors during propulsion.
Data availability statement
The raw data supporting the conclusions of this article will be made available by the authors, without undue reservation.
Ethics statement
The studies involving humans were approved by California State University, Fullerton Institutional Review Board. The studies were conducted in accordance with the local legislation and institutional requirements. The participants provided their written informed consent to participate in this study.
Author contributions
RJE: Conceptualization, Formal Analysis, Visualization, Writing – original draft, Writing – review & editing. TM: Conceptualization, Data curation, Methodology, Writing – review & editing. PM: Conceptualization, Data curation, Methodology, Writing – review & editing. DP: Conceptualization, Formal Analysis, Methodology, Resources, Software, Supervision, Visualization, Writing – review & editing.
Funding
The author(s) declare that no financial support was received for the research, authorship, and/or publication of this article.
DNP is supported by the Natural Science and Engineering Council of Canada (RGPIN-2022-04804).
Conflict of interest
The authors declare that the research was conducted in the absence of any commercial or financial relationships that could be construed as a potential conflict of interest.
Publisher's note
All claims expressed in this article are solely those of the authors and do not necessarily represent those of their affiliated organizations, or those of the publisher, the editors and the reviewers. Any product that may be evaluated in this article, or claim that may be made by its manufacturer, is not guaranteed or endorsed by the publisher.
References
1. Hulteen RM, Smith JJ, Morgan PJ, Barnett LM, Hallal PC, Colyvas K, et al. Global participation in sport and leisure-time physical activities: a systematic review and meta-analysis. Prev Med. (2017) 95:14–25. doi: 10.1016/j.ypmed.2016.11.027
2. Oja P, Titze S, Kokko S, Kujala UM, Heinonen A, Kelly P, et al. Health benefits of different sport disciplines for adults: systematic review of observational and intervention studies with meta-analysis. Br J Sports Med. (2015) 49:434–40. doi: 10.1136/bjsports-2014-093885
3. Dempster J, Dutheil F, Ugbolue UC. The prevalence of lower extremity injuries in running and associated risk factors: a systematic review. Phys Act Health. (2021) 5:133–45. doi: 10.5334/paah.109
4. Koech RC, Olivier B, Tawa N. A prevalence of running-related injuries among professional endurance runners in the Rift Valley, Kenya. S Afr J Sports Med. (2021) 33(1):1–4. doi: 10.17159/2078-516X/2021/v33i1a10690
5. Nielsen RO, Rønnow L, Rasmussen S, Lind M. A prospective study on time to recovery in 254 injured novice runners. PLoS One. (12) 9(6):1–6. doi: 10.1371/journal.pone.0099877
6. Hreljac A. Impact and overuse injuries in runners. Med Sci Sports Exerc. (2004) 36(5):845–9. doi: 10.1249/01.mss.0000126803.66636.dd
7. Dias Lopes A, Carlos L, Junior H, Yeung SS, Oliveira Pena Costa L. What are the main running-related musculoskeletal injuries? A systematic review. Sports Med. (2012) 42:891–905. doi: 10.1007/BF03262301
8. Clermont CA, Osis ST, Phinyomark A, Ferber R. Kinematic gait patterns in competitive and recreational runners. J Appl Biomech. (2017) 33(4):268–76. doi: 10.1123/jab.2016-0218
9. Kluitenberg B, van Middelkoop M, Diercks R, van der Worp H. What are the differences in injury proportions between different populations of runners? A systematic review and meta-analysis. Sports Med. (2015) 45(8):1143–61. doi: 10.1007/s40279-015-0331-x
10. Haugen T, Sandbakk Ø, Seiler S, Tønnessen E. The training characteristics of world-class distance runners: an integration of scientific literature and results-proven practice. Sports Med Open. (2022) 8(1):46. doi: 10.1186/s40798-022-00438-7
11. Luz BC, dos Santos AF, Serrão FV. Are hip and knee kinematics and training load characteristics relate to pain intensity and physical function level in runners with patellofemoral pain? Gait Posture. (2021) 84:162–8. doi: 10.1016/j.gaitpost.2020.11.027
12. Blagrove RC, Brown N, Howatson G, Hayes PR. Strength and conditioning habits of competitive distance runners. Strength Cond Res. (2017) 34(5):1392–9. doi: 10.1519/JSC.0000000000002261
13. Preece SJ, Bramah C, Mason D. The biomechanical characteristics of high-performance endurance running. Eur J Sport Sci. (2019) 19(6):784–92. doi: 10.1080/17461391.2018.1554707
14. Orendurff MS, Kobayashi T, Tulchin-Francis K, Tullock AMH, Villarosa C, Chan C, et al. A little bit faster: lower extremity joint kinematics and kinetics as recreational runners achieve faster speeds. J Biomech. (2018) 71:167–75. doi: 10.1016/j.jbiomech.2018.02.010
15. Kliethermes SA, Stiffler-Joachim MR, Wille CM, Sanfilippo JL, Zavala P, Heiderscheit BC. Lower step rate is associated with a higher risk of bone stress injury: a prospective study of collegiate cross country runners. Br J Sports Med. (2021) 55(15):851–6. doi: 10.1136/bjsports-2020-103833
16. Folland JP, Allen SJ, Black MI, Handsaker JC, Forrester SE. Running technique is an important component of running economy and performance. Med Sci Sports Exerc. (2017) 49(7):1412–23. doi: 10.1249/MSS.0000000000001245
17. Burns GT, Gonzalez R, Zendler JM, Zernicke RF. Bouncing behavior of sub-four minute milers. Sci Rep. (2021) 11(1):1–15. doi: 10.1038/s41598-021-89858-1
18. Napier C, Maclean CL, Maurer J, Taunton JE, Hunt MA. Real-time biofeedback of performance to reduce braking forces associated with running-related injury: an exploratory study. J Orthop Sports Phys Ther. (2019) 49(3):136–44. doi: 10.2519/jospt.2019.8587
19. Winter DA. Overall principle of lower limb support during stance phase of gait*. J Biomech. (1980) 13:923–7. doi: 10.1016/0021-9290(80)90162-1
20. Jin L, Hahn ME. Modulation of lower extremity joint stiffness, work and power at different walking and running speeds. Hum Mov Sci. (2018) 58:1–9. doi: 10.1016/j.humov.2018.01.004
21. Mitchell PK, Moffit TJ, Montgomery MM, Pamukoff DN. Running kinetics and femoral trochlea cartilage characteristics in recreational and collegiate distance runners. J Sports Sci. (2022) 40(1):89–95. doi: 10.1080/02640414.2021.1976492
22. Moffit TJ, Montgomery MM, Lockie RG, Pamukoff DN. Association between knee- and hip-extensor strength and running-related injury biomechanics in collegiate distance runners. J Athl Train. (2020) 55(12):1262–9. doi: 10.4085/1062-6050-0532.19
23. Mai P, Willwacher S. Effects of low-pass filter combinations on lower extremity joint moments in distance running. J Biomech. (2019) 95:1–10. doi: 10.1016/j.jbiomech.2019.08.005
24. Gonzales JM, Galpin AJ, Montgomery MM, Pamukoff DN. Comparison of lower limb muscle architecture and geometry in distance runners with rearfoot and forefoot strike pattern. J Sports Sci. (2019) 37(19):2184–90. doi: 10.1080/02640414.2019.1626050
25. Altman AR, Davis IS. A kinematic method for footstrike pattern detection in barefoot and shod runners. Gait Posture. (2012) 35(2):298–300. doi: 10.1016/j.gaitpost.2011.09.104
26. Rice HM, Jamison ST, Davis IS. Footwear matters: influence of footwear and foot strike on load rates during running. Med Sci Sports Exerc. (2016) 48(12):2462–8. doi: 10.1249/MSS.0000000000001030
27. Chan YH. Biostatistics 104: correlational analysis. Singapore Med J. (2003) 44(12):614–9.14770254
28. Thomas JR, Nelson JK. Research methods in physical activity. Champaign, IL: Human Kinetics (2001).
29. Schache AG, Brown NAT, Pandy MG. Modulation of work and power by the human lower-limb joints with increasing steady-state locomotion speed. J Exp Biol. (2015) 218(15):2472–81. doi: 10.1242/jeb.119156
30. Liao TC, Keyak JH, Powers CM. Runners with patellofemoral pain exhibit greater peak patella cartilage stress compared with pain-free runners. J Appl Biomech. (2018) 34(4):298–305. doi: 10.1123/jab.2017-0229
31. dos Santos AF, Nakagawa TH, Serrão FV, Ferber R. Patellofemoral joint stress measured across three different running techniques. Gait Posture. (2019) 68:37–43. doi: 10.1016/j.gaitpost.2018.11.002
32. Esculier JF, Maggs K, Maggs E, Dubois B. A contemporary approach to patellofemoral pain in runners. J Athl Train. (2020) 55(12):1206–14. doi: 10.4085/1062-6050-0535.19
33. Daoud AI, Geissler GJ, Wang F, Saretsky J, Daoud YA, Lieberman DE. Foot strike and injury rates in endurance runners: a retrospective study. Med Sci Sports Exerc. (2012) 44(7):1325–34. doi: 10.1249/MSS.0b013e3182465115
34. Starbuck C, Bramah C, Herrington L, Jones R. The effect of speed on achilles tendon forces and patellofemoral joint stresses in high-performing endurance runners. Scand J Med Sci Sports. (2021) 31(8):1657–65. doi: 10.1111/sms.13972
35. Ertman B, Klaeser M, Voie L, Gheidi N, Vannatta CN, Rutherford D, et al. Alterations in achilles tendon stress and strain across a range of running velocities. J Sports Sci. (2023) 41:1–7. doi: 10.1080/02640414.2023.2225015
36. Li F, Wang R, Newton RU, Sutton D, Shi Y, Ding H. Effects of complex training versus heavy resistance training on neuromuscular adaptation, running economy and 5-km performance in well-trained distance runners. PeerJ. (2019) 2019(4):1–21. doi: 10.7717/peerj.6787
37. Milner CE, Ferber R, Pollard CD, Hamill J, Davis IS. Biomechanical factors associated with tibial stress fracture in female runners. Med Sci Sports Exerc. (2006) 38(2):323–8. doi: 10.1249/01.mss.0000183477.75808.92
38. Davis IF, Milner CE, Hamill JF. Does increased loading during running lead to tibial stress fractures? A prospective study. Med Sci Sports Exerc. (2004) 36(5):S58. doi: 10.1249/00005768-200405001-00271
39. Warden SJ, Davis IS, Fredericson M. Management and prevention of bone stress injuries in long-distance runners. J Orthop Sports Phys Ther. (2014) 44:749–65. doi: 10.2519/jospt.2014.5334
40. Zadpoor AA, Nikooyan AA. The relationship between lower-extremity stress fractures and the ground reaction force: a systematic review. Clin Biomech. (2011) 26:23–8. doi: 10.1016/j.clinbiomech.2010.08.005
41. Breine B, Malcolm P, Galle S, Fiers P, Frederick EC, De Clercq D. Running speed-induced changes in foot contact pattern influence impact loading rate. Eur J Sport Sci. (2019) 19(6):774–83. doi: 10.1080/17461391.2018.1541256
42. Napier C, MacLean CL, Maurer J, Taunton JE, Hunt MA. Kinetic risk factors of running-related injuries in female recreational runners. Scand J Med Sci Sports. (2018) 28(10):2164–72. doi: 10.1111/sms.13228
43. Weyand PG, Sternlight DB, Bellizzi MJ, Wright S, Weyand PG. Faster top running speeds are achieved with greater ground forces not more rapid leg movements. J Appl Physiol. (2000) 89:1991–9. doi: 10.1152/japplphysiol.00010.2023
44. Zifchock RA, Davis I, Hamill J. Kinetic asymmetry in female runners with and without retrospective tibial stress fractures. J Biomech. (2006) 39(15):2792–7. doi: 10.1016/j.jbiomech.2005.10.003
45. Turner CH, Wang T, Burr DB. Shear strength and fatigue properties of human cortical bone determined from pure shear tests. Calcif Tissue Int. (2001) 69(6):373–8. doi: 10.1007/s00223-001-1006-1
46. Nordin AD, Dufek JS, Mercer JA. Three-dimensional impact kinetics with foot-strike manipulations during running. J Sport Health Sci. (2017) 6(4):489–97. doi: 10.1016/j.jshs.2015.11.003
Keywords: running, biomechanics, injury, performance, speed, gait, propulsion
Citation: Evans RJ, Moffit TJ, Mitchell PK and Pamukoff DN (2023) Injury and performance related biomechanical differences between recreational and collegiate runners. Front. Sports Act. Living 5:1268292. doi: 10.3389/fspor.2023.1268292
Received: 27 July 2023; Accepted: 4 September 2023;
Published: 14 September 2023.
Edited by:
Lei Qian, Southern Medical University, ChinaReviewed by:
Carlo M. Biancardi, Universidad de la República, UruguayEvelyn Muschter, Technical University Dresden, Germany
Brian Hanley, Leeds Beckett University, United Kingdom
© 2023 Evans, Moffit, Mitchell and Pamukoff. This is an open-access article distributed under the terms of the Creative Commons Attribution License (CC BY). The use, distribution or reproduction in other forums is permitted, provided the original author(s) and the copyright owner(s) are credited and that the original publication in this journal is cited, in accordance with accepted academic practice. No use, distribution or reproduction is permitted which does not comply with these terms.
*Correspondence: Derek N. Pamukoff ZHBhbXVrb2ZAdXdvLmNh