- 1Department of Human Kinetics, Université du Québec à Trois-Rivières, Trois-Rivières, QC, Canada
- 2Laboratoire de Recherche sur le Hockey, Université du Québec à Trois-Rivières, Trois-Rivières, QC, Canada
- 3Service de l'Activité Physique et Sportive, Université du Québec à Trois-Rivières, Trois-Rivières, QC, Canada
Introduction: Sprinting ability and anaerobic capacities are the determinant variables of the performance of ice hockey players. Recent research in sprinting showed the existence of distinct force–velocity (F–V) profiles, but the link between these profiles and anaerobic capacities remains unclear. This study explores the associations between F–V variables and anaerobic capacities among cohorts of highly trained adolescent ice hockey players.
Methods: Data from 36 men (age, 15.1 ± 0.2 years) and 34 women (age, 16.5 ± 0.7 years) were collected during off-season camps. All athletes completed a 30-m sprint test, a Wingate anaerobic test (WAnT), and a repeated-sprint anaerobic (RSA) test. F–V variables were calculated from the 30-m sprint test. Partial Pearson correlation coefficients for pooled data and Pearson correlation coefficients for individual male and female datasets were calculated.
Results: Among the F–V variables, maximal theoretical velocity and power were moderately to largely associated with WAnT and RSA performance (|r| = 0.30–0.70). Maximal theoretical force was moderately associated with the RSA (r = −0.32 to −0.33).
Discussion: The results indicate the importance for highly trained adolescent players to be able to apply force at high velocities to maximize anaerobic capacities. Important differences between male and female players suggest training priorities may differ according to sex.
Introduction
With approximately 18% of effective playing time spent in high-intensity skating activities, performance in ice hockey is a combination of short bouts of explosive and powerful movements cumulating in high energetic demands (1). The ability to skate and accelerate at higher speeds distinguishes the best young players (2). Improving sprinting ability off the ice during off-season is important as it may improve skating performance in-season (3). Developing the anaerobic system is also important as this metabolic system assumes 69% of energy costs during competition (4). To develop both of these capacities, strength and conditioning coaches turn to combinations of various off-ice methods, such as strength exercises, heavy resisted sprints, repeated sprints, and high-intensity training (5–9). However, the concurrent use of all these training methods during off-season presents periodization challenges that may hinder strength/power and anaerobic gains (10, 11).
In recent years, analysis of the sprint force–velocity (F–V) profile has gained popularity among researchers and practitioners who have turned toward this evaluation method to efficiently individualize training prescriptions (12, 13). The procedure gives an overall view of the strength/power/velocity capabilities of an athlete (defined in Table 1) during sprinting tasks by following the time–velocity curve of their center of mass (14). The mechanical capabilities evaluated using this method for a given athlete are compared with those of an optimal profile (12, 15). In fact, Morin and Samozino (15) have demonstrated that theoretical maximal force (F0) translates to shorter distance sprint performance (<20 m), whereas theoretical maximal velocity (V0) translates to longer distance sprint performance (>30 m).
Available research including ice hockey players focused mainly on associations between sprinting F–V, skating F–V, vertical jump performance, and skating performance. Perez et al. (16) explored the associations between both profiles and jumping, running, or skating performance with 17 French national team female players (mean age, 21.6 ± 3.4 years). They demonstrated that maximal theoretical power (Pmax) displayed the strongest associations with the three performance indicators (|r| ranging from 0.81 to 0.92; p < 0.001). Other work including ice hockey players was used to confirm the validity and reliability of the method on the ice, this time with French elite female players and Finnish elite male junior players (17–19). To our knowledge, F–V data for highly trained adolescent ice hockey players have yet to be published. As the physical and physiological performance does not linearly change during the critical stage of adolescence (20–22), studying these age groups is important. Understanding the links between sprinting F–V and a wider set of physical or physiological markers such as anaerobic capacity could guide the decisions of the practitioners during off-season.
In other sports, some work has linked sprint mechanical capabilities to anaerobic capacities. The results with rugby sevens players and soccer players consistently showed that the ability to efficiently generate force at high velocities (e.g., V0 and Drf) was impaired while the ability to efficiently generate force at low speeds (e.g., F0 and Rfmax) remained constant during repeated sprints (23, 24). In both cases, linear sprints were used during the repeated-sprint test even though team sports performance includes much shorter sprints and multiple changes of directions (25). In addition to such limitations, the links between mechanical capabilities during an independent maximal sprint test and repeated-sprint ability were not reported. Given the widespread use of anaerobic capacity tests during off-season testing of ice hockey players (25, 26), a better understanding of the associations between sprinting F–V and common anaerobic capacity tests could help practitioners narrow down the preferred and most effective training methods during off-season. Hence, this study aimed to explore associations between sprinting F–V mechanical capabilities and anaerobic capacities of 15- to 17-year-old male and female ice hockey players.
Two specific objectives arise from this study. First, this study explores how the mechanical capabilities of sprinting F–V are related to the variables from anaerobic tests used in ice hockey (e.g., the Wingate and a repeated-sprint ability test with a 180° turn). Second, this study explores the differences between male and female players. Based on the previous results by Perez et al. (16), it was hypothesized that Pmax shows the strongest associations with the different variables of both anaerobic tests. It was also believed that V0 shows stronger associations with the Wingate given the time needed to reach peak power (around 7 s) and F0 shows stronger associations with the repeated sprints due to the included change of direction in this study. Finally, given that female athletes tend to have more force-oriented profiles (27, 28), it was hypothesized that F0 plays a more important role in the anaerobic performance for this population.
Methods
Participants
Every spring, the Quebec Ice Hockey Federation invites the most promising players (men and women) to development camps. Invitations are based on the performance of players during the regular season. Among these invited players, the best will be part of the team competing at the national level. In the summer of 2022, 42 men and 42 women were invited to a summer camp launching off-season training for players. Various physical and physiological abilities were tested. Goaltenders were excluded from the study design given the unique nature and demands of their position (29). Injured players or players who were unable to complete the entire testing protocol were also excluded from the study. Finally, 36 men (age = 15.1 ± 0.2 years, stature = 177.5 ± 6.4, mass = 70.6 ± 8.4) and 34 women (age = 16.5 ± 0.7 years, stature = 167.3 ± 5.5, mass = 65.4 ± 7.0) participated in the study. Given their training status and preparation for national-level competitions, the participants are classified as highly trained athletes (30). Post hoc power analysis for between-groups comparisons and correlations was computed using G*Power v.3.1.9.7. Statistical power for the smallest observed effect size (0.67) at a significance level of α = 0.05 was 0.8 for both methods. This study was approved by the ethics board of the researchers’ institution (CER-21-278-07.29). Following the rules and regulations of our country and province, only written consent from players was obtained. Article 21 of the Civil Code of Quebec stipulates that “Consent to research that could interfere with the integrity of a minor may be given by the person having parental authority or the tutor. A minor 14 years of age or over, however, may give consent alone if, in the opinion of the competent research ethics committee, the research involves only minimal risk and the circumstances justify it.”
Study design
Male and female players were tested on two separate consecutive days, following similar procedures (e.g., Day 1 = men, Day 2 = women). The players were divided into two subgroups alternating between physical testing and team meetings. The testing protocol of the camp consisted of anthropometric measurements, strength, speed, agility, power, anaerobic capacity, and mobility. These physical parameters were collected via various tests: stature, mass, grip strength, chin-ups, bench press, standing long jump, countermovement jump, 30-s Wingate anaerobic test (WAnT), 30-m sprint, 5-10-5 shuttle run, and repeated anaerobic sprint test with a 180° change of direction [repeated-sprint anaerobic (RSA)]. The tests were divided into two sessions. For the morning session, the players were divided into five groups alternating between one agility and five muscular tests. The WAnT was done at the end of the session. The players then had 3 h before the afternoon session. During this session, they completed the 30-m sprint followed 15 min later by the RSA.
Horizontal F–V profile
To determine the individual F–V of each player, the players ran two 30-m sprints. The method has an “acceptable” intraday and inter-day reliability [intraclass correlation coefficient (ICC) ≥ 0.75 and coefficient of variation (CV) ≤ 10%] (14). Before the sprints, the players started in a feet supported crouched position and stood still until instructed to run by the research assistant. They were given 3- to 4-min rest between trials. Instant velocity over the sprinting distance was collected at 46.875 Hz using a Stalker ATS II (Stalker Sport, Richardson, TX, USA). The radar was placed 3 m behind the starting line and 1 m aboveground approximately at the height of the center of mass of the players. Following protocols and guidelines established by Samozino et al. (14), raw velocity data were first imported into R studio. Then, data prior to the start and after the maximal velocity plateau were discarded. The remaining data were fitted with a mono-exponential function, and mechanical variables were calculated (14, 18). An optimal profile at 10 m (Sfvopt) was computed following the proposed methods by Samozino et al. (31). To improve reliability, the average values of both trials were used for further analyses (32, 33).
Wingate
To evaluate anaerobic capacity, the WAnT was used. The WAnT is a popular test that measures the power production of a player throughout a 30-s bout. The validity and reliability of the test to evaluate the anaerobic capacity of ice hockey players were confirmed (34, 35). The test was completed on an air-braked cycle ergometer (Wattbike Pro/Trainer, Nottingham, UK, 2022), which was also validated in a previous study (33). The test started with a 5-min warm-up on a cycle ergometer with a resistance of 75 W and a cadence of 85 revolutions per minute (rpm). For the last 6 s of each minute, the players were asked to sprint. A 3-min rest period was given before the start of the test. After the WAnT, a 2-min cooldown was given. Peak power, relative peak power, average power (e.g., average power exerted during the test), relative average power, anaerobic capacity (e.g., the sum of average values recorded in each of six 5-s blocks), and anaerobic fatigue (e.g., drop-off in power from the highest to the lowest 5-s block represented as a percentage) were extracted directly from the Wattbike cycle ergometer console and used for analysis.
Repeated-sprint ability
To evaluate the anaerobic capacity of the players in a more practical approach, a RSA test with a 180° change of direction (RSA) was designed. In practice, the available space was limited and imposed constraints for the chosen test format in line with guidelines by Kyles et al. (25). The players ran 20 m and then changed direction to run back 20 m. The players completed six sprints and had 30 s to complete a sprint and start the next. This procedure was validated previously with cohorts of soccer players (36) and chosen to introduce a change of direction component, which is important for the performance in team sports such as ice hockey. Sprint times were collected using Swift (Swift Performance, Northbrook, IL, USA) single-beam laser timing gates placed on the starting line. The players started 30 cm behind the gates. The best time between the first two sprints, total time, and a fatigue index were used for analysis. The fatigue index was computed following the recommendations of Glaister et al. (37) by using the percentage decrement score [100 × (total sprint time ÷ ideal sprint time)] − 100, where the ideal sprint time = number of sprints × fastest sprint time. The results for two female players and seven male players were excluded from final analysis as they did not perform their best sprint in the first two trials, which could indicate the use of a pacing strategy (24).
Statistical analysis
Descriptive statistics for sprinting F–V, Wingate, and RSA are presented in Table 1. Normality assumptions for each variable were confirmed by verifying the skewness and kurtosis of each distribution. To compare male and female players, independent Student t-test were computed with Cohen's D to estimate effect sizes. The reliability of F–V mechanical capabilities with adolescent ice hockey players was confirmed by computing ICCs and CV for both trials. All variables had ICC values ≥ 0.90 and CV ≤ 10% (38). To explore relations between the F–V and anaerobic capacity of the players while controlling for sex differences, partial Pearson correlation coefficients for pooled data and Pearson correlation coefficients for individual sex (e.g., men and women) were calculated. As recommended by Hopkins et al. (38), the magnitude of Pearson's correlation coefficients (r) was considered trivial (r < 0.10), small (r = 0.10–0.29), moderate (r = 0.30–0.49), large (r = 0.50–0.69), very large (r = 0.70–0.89), nearly perfect (r = 0.90–0.99), and perfect (r = 1.00). Statistical analyses were conducted using SPSS version 28.0 (IBM Corporation, Armonk, NY, USA).
Results
Associations between F–V mechanical capabilities and anaerobic capacities
Partial Pearson's correlation coefficients suggest that F0 had a small association with the relative peak power (r = 0.26, p = 0.049) of the WAnT and was moderately associated with total time (r = −0.32, p = 0.012) and best time (r = −0.33, p = 0.10) of the RSA. V0 and Pmax were moderately associated with peak power (r = 0.43 and 0.35, p < 0.001 and p = 0.006), relative peak power (r = 0.49 and 0.44, p < 0.001), average power (r = 0.30, p = 0.019 and 0.018), and anaerobic capacity (r = 0.37 and 0.32, p = 0.003 and 0.012) of the WAnT and were largely associated with total time (r = −0.65 and –0.57, p < 0.001) and best time (r = −0.70 and −0.60, p < 0.001) of the RSA. Rfmax was moderately associated with the relative peak power (r = 0.33, p = 0.011) of the WAnT and had small associations with total time (r = −0.26, p = 0.049) and best time (r = −0.27, p = 0.035) of the RSA. Sfv and Drf were not associated with the WAnT or the RSA.
Differences between male and female players
As shown in Table 2, independent Student t-tests demonstrated that male players were taller and heavier and performed better than female players in all variables except for Sfv and Drf. F0, Sfv, and Rfmax of the male players were not associated with WAnT or RSA variables. V0 was moderately associated with peak power (r = 0.34, p = 0.037) and relative peak power (r = 0.34, p = 0.045) in the WAnT and was largely associated with total time (r = −0.59, p < 0.001) and best time (r = −0.64, p < 0.001) in the RSA. Pmax was not associated with variables from the WAnT, but was moderately associated with total time (r = −0.41, p = 0.028) and largely associated with the best time (r = −0.53, p = 0.003) in the RSA. Drf was moderately associated with total time (r = –0.39, p = 0.036) in the RSA.
Sfv and Drf of the female players were not associated with WAnT or RSA variables. F0 was moderately associated with relative peak power (r = 0.34, p = 0.047), relative average power (r = 0.34, p = 0.050) of the WAnT, and total time (r = −0.45, p = 0.010) and best time (r = −0.40, p = 0.022) of the RSA. V0 was largely associated to relative peak power (r = 0.62, p < 0.001), moderately associated with peak power (r = 0.47, p = 0.005) and anaerobic capacity (r = 0.39, p = 0.022) of the WAnT, and largely associated with total time (r = −0.75, p < 0.001) and best time (r = −0.78, p < 0.001) of the RSA. Pmax was largely associated with relative peak power (r = 0.53, p = 0.001), moderately with relative average power (r = 0.38, p = 0.026) of the WAnT, and largely associated with total time (r = −0.68, p < 0.001) and best time (r = −0.66, p < 0.001) of the RSA. Rfmax was moderately associated with relative peak power (r = 0.48, p = 0.004), relative average power (r = 0.38, p = 0.025) of the WAnT and with total time (r = −0.43, p = 0.013), and best time (r = −0.45, p = 0.011) of the RSA. Partial correlations and correlations for male and female players for F0 and V0 are illustrated in Figures 1, 2 to express the main differences between the sexes.
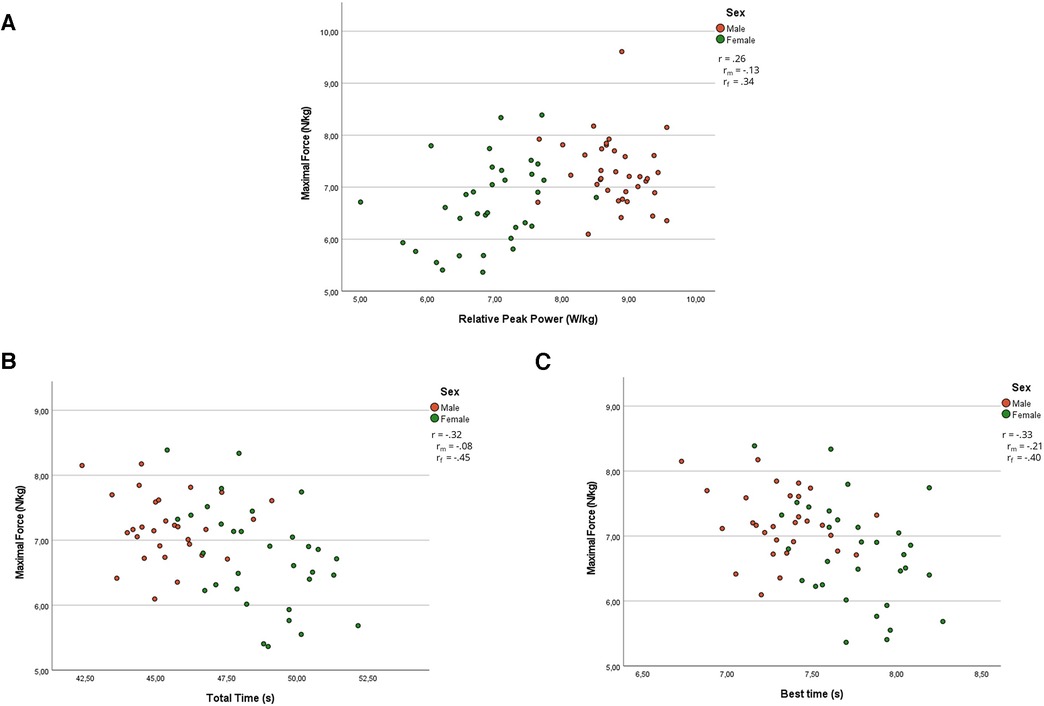
Figure 1. Partial correlation and correlations between maximal theoretical force (F0) and relative peak power (A), total time (B), and best time (C) for pooled data and male and female data, respectively. r, partial correlation; rm, correlation for men; rf, correlations for women.
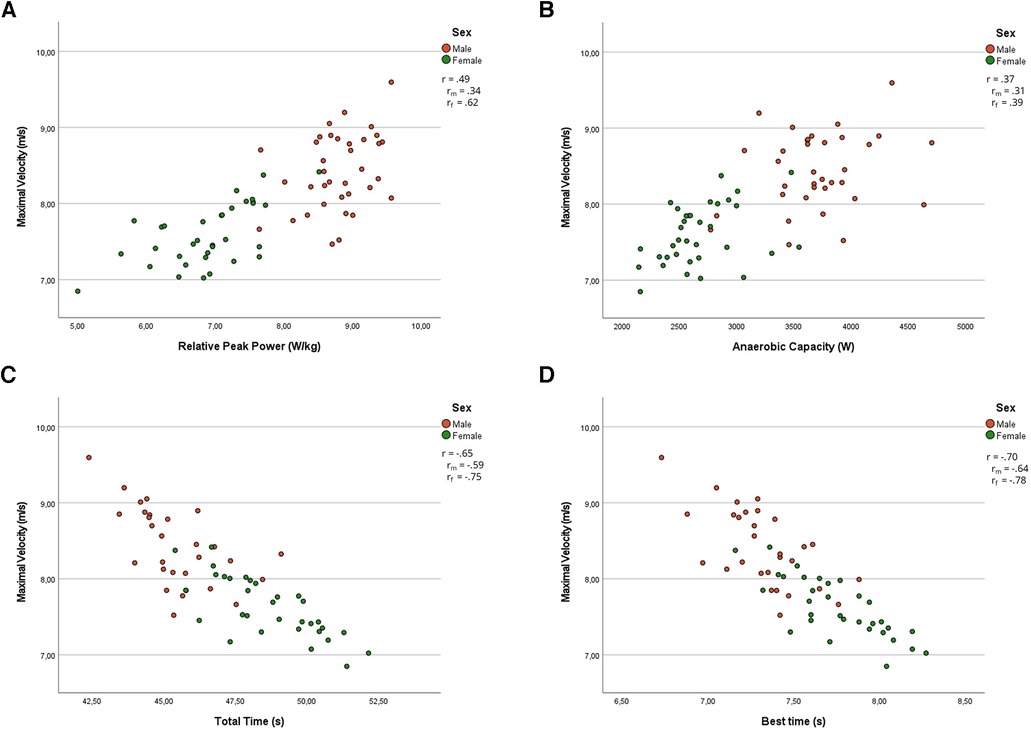
Figure 2. Partial correlation and correlations between maximal theoretical velocity (V0) and relative peak power (A), anaerobic capacity (B), total time (C), and best time (D) for pooled data and male and female data, respectively. r, partial correlation; rm, correlation for men; rf, correlations for women.
Discussion
The relevance of F–V athlete profiling has emerged over the last decade. However, little is known about its associations with physical attributes that require high-intensity efforts, such as anaerobic capacities. Considering the importance of such qualities to excel in ice hockey, a deeper understanding of these associations is helpful for strength and conditioning coaches to optimize performance. This paper was the first to explore the associations between sprinting F–V mechanical capabilities and anaerobic capacities of highly competitive adolescent male and female ice hockey players. The general aim of this study was to widen current knowledge around sprinting F–V and its links with anaerobic capacities measured threw common tests. Previous work analyzing the sprinting F–V of ice hockey players was fairly limited focusing more on the validity and reliability of the method with a limited number of subpopulations, that is, female French senior to junior national teams and male players from elite Finnish leagues (16–19). The first objective of this study was to explore how the mechanical capabilities of sprinting F–V were related to variables from common anaerobic tests such as the Wingate and repeated-sprint test. To provide a complete observation of these associations, three main hypotheses were proposed. The first hypothesis was that Pmax would show the strongest associations with the different variables of both anaerobic tests, V0 would show stronger associations with the cycling test, and F0 would show stronger associations with the repeated-sprint ability. When considering results for pooled data, Pmax was associated with anaerobic power and anaerobic capacity in both tests yet appeared stronger for the running test (moderate associations for peak power, relative peak power, and average power in the WAnT vs. large associations for total time and best time in the RSA). Unlike initially hypothesized, V0 showed the strongest associations with performance in both tests, correlating largely with the RSA and moderately with the WAnT. In contrast, F0 showed few associations to the Wingate with a small association to relative peak power and moderate associations with the RSA. The initial hypothesis was based on the proposed interpretations of Morin and Samozino (15) and only focused on an analysis of the tasks used in this study. However, these interpretations were majorly based on the observations with elite-level athletes. The different playing levels and ages of participants from this study probably influenced the associations observed in this study.
The second objective of this study was to explore differences between male and female players. The hypothesis was that F0 would exhibit stronger associations with performance in female athletes as they tend to have more force-oriented F–V profiles (27, 28). Our results confirmed this hypothesis as associations between F0 and performance in both anaerobic tests were only present for the female players (see Figures 1, 2). The cycling performance and repeated-sprint performance of male players in this study were mainly determined by V0. Given their young age, F0 may not have been fully developed at the moment of testing (22) and could explain the observed differences with female players. Previous work by Perez et al. (16) highlighted the absence of associations between the actual F–V and performance of an athlete as observed in this study. To our knowledge, this study was the first to include the computation of Sfvopt and FVimb. in the evaluation of the sprinting F–V with ice hockey players. Looking at Figure 3, both groups show a force deficit which may explain the large associations between V0 and RSA performance. The appearance of associations with F0 for female players could be attributed to the portion of players with a velocity deficit (bottom quartile of female box plot). Nonetheless, practitioners training sprint ability and anaerobic capacities concurrently should choose training methods according to the sex of players. Highly trained female players may benefit from methods including explosive exercises at low and high velocities while highly trained male players in the age range of this study could focus more on developing maximal velocity. Jiménez-Reyes et al. (12) categorized exercises according to the desired training focus for enhanced jumping performance. Similarly, practitioners could use resisted sprints for an emphasis on low velocities (5) and flying sprints to train at higher velocities to enhance sprint performance.
Although this study adds to available knowledge regarding sprinting F–V profiling in ice hockey, certain limitations persist. First, while the reliability of the computation method by Samozino et al. (14) has been reported with youth athletes (22), no validation of the method has been published. The sprinting mechanics of teenage ice hockey players are different from elite adult sprinters. However, participants in this study have exhibited lower mechanical characteristics than higher-level athletes in previous studies (17–19). Given velocity follows the same mono-exponential function in youth (22) and aerodynamics included in the method adjusts to the stature and mass of each individual (14), there are a few reasons that the method should not be valid with this study population. Second, the observational design of this study does not confirm the causal effects of the different sprinting F–V variables on anaerobic performance. The type and strength of associations could be explained by the theoretical basis presented by Morin and Samozino (15) and previous observations. However, further research should focus on interventions demonstrating the advantages of individualized training based on the F–V as this would yield the best practical applications (12, 39). Third, this study only considered off-ice data that were collected during off-season. Prediction of skating abilities from off-ice activities or the transfer of such activities to skating performance and anaerobic capacities on the ice remains unclear. For example, Perez et al. (16) demonstrated that sprinting and skating F–V were not correlated. Ultimately, the goal of ice hockey training is to maximize performance on the ice. In this regard, developing research designs that allow the evaluation of the transferability of off-ice attributes to on-ice performance is a key concern for stakeholders overseeing the development of highly competitive ice hockey players. Comparing the skating F–V and anaerobic capacities of players on the ice would allow for a better understanding of determinant indicators in ice hockey and designing efficient training programs.
Conclusion
This research provides a deeper understanding of underlying associations between the sprinting F–V and anaerobic capacities of highly trained adolescent male and female ice hockey players. Pooled data demonstrated that the ability to apply force at low and high velocities are determinant for anaerobic performance on a cycle ergometer and repeated-sprint ability including changes of direction. A separate analysis of male and female players demonstrated that determinant mechanical capabilities of sprinting vary according to sex. Specifically, the cycling and repeated-sprint performance of younger male players could be mainly determined by the ability to apply force at high velocities, whereas the ability to generate force at slow speeds may be of added importance for female players. Practitioners must understand that determinant mechanical capabilities of sprinting for anaerobic performance may be different for male and female athletes and training priorities may change accordingly.
Data availability statement
The raw data supporting the conclusions of this article will be made available by the authors, without undue reservation.
Ethics statement
The study involving humans was approved by Comité d'Éthique de la recherche avec des Êtres Humains UQTR. The study was conducted in accordance with the local legislation and institutional requirements. Written informed consent for participation was obtained from participants but was not required from the participants' legal guardians/next of kin because the project was approved by the ethics committee, which follows the rules and regulations of our country and province. Article 21 of the Civil Code of Quebec stipulates that “Consent to research that could interfere with the integrity of a minor may be given by the person having parental authority or the tutor. A minor 14 years of age or over, however, may give consent alone if, in the opinion of the competent research ethics committee, the research involves only minimal risk and the circumstances justify it.”
Author contributions
JG: Conceptualization, Formal analysis, Methodology, Software, Writing – original draft, Writing – review & editing. PP: Data curation, Investigation, Writing – original draft. JB: Conceptualization, Data curation, Methodology, Project administration, Resources, Writing – original draft. JL: Conceptualization, Formal analysis, Funding acquisition, Investigation, Methodology, Project administration, Supervision, Writing – original draft, Writing – review & editing.
Funding
The authors declare financial support was received for the research, authorship, and/or publication of this article.
JL received a governmental grant from Ministère de l’Éducation du Québec (Initiative Projets Synergiques). The project was also funded by the MITACS-Elevation (IT31707) between 2021 and 2023.
Acknowledgments
The authors wish to thank the players who participated in this study and the staff of Hockey Quebec for their collaboration during the planning and execution of testing.
Conflict of interest
The authors declare that the research was conducted in the absence of any commercial or financial relationships that could be construed as a potential conflict of interest.
Publisher's note
All claims expressed in this article are solely those of the authors and do not necessarily represent those of their affiliated organizations, or those of the publisher, the editors and the reviewers. Any product that may be evaluated in this article, or claim that may be made by its manufacturer, is not guaranteed or endorsed by the publisher.
References
1. Brocherie F, Girard O, Millet GP. Updated analysis of changes in locomotor activities across periods in an international ice hockey game. Biol Sport. (2018) 35(3):261–7. doi: 10.5114/biolsport.2018.77826
2. Douglas AS, Rotondi MA, Baker J, Jamnik VK, Macpherson AK. A comparison of on-ice external load measures between subelite and elite female ice hockey players. J Strength Cond Res. (2022) 36(7):1978–83. doi: 10.1519/JSC.0000000000003771
3. Haukali E, Tjelta LI. Relationship between off-season changes in power and in-season changes in skating speed in young ice hockey players. Int J Appl Sports Sci. (2016) 28(2):111–22. doi: 10.24985/ijass.2016.28.2.111
4. Burr JF, Jamnik RK, Baker J, Macpherson A, Gledhill N, McGuire E. Relationship of physical fitness test results and hockey playing potential in elite-level ice hockey players. J Strength Cond Res. (2008) 22(5):1535–43. doi: 10.1519/JSC.0b013e318181ac20
5. Matthews MJ, Comfort P, Crebin R. Complex training in ice hockey: the effects of a heavy resisted sprint on subsequent ice-hockey sprint performance. J Strength Cond Res. (2010) 24(11):2883–7. doi: 10.1519/JSC.0b013e3181e7253c
6. Dæhlin TE, Haugen OC, Haugerud S, Hollan I, Raastad T, Rønnestad BR. Improvement of ice hockey players’ on-ice sprint with combined plyometric and strength training. Int J Sports Physiol Perform. (2017) 12(7):893–900. doi: 10.1123/ijspp.2016-0262
7. Lagrange S, Ferland P-M, Leone M, Comtois AS. Contrast training generates post-activation potentiation and improves repeated-sprint ability in elite ice hockey players. Int J Exerc Sci. (2020) 13(6):183.32148640
8. Naimo M, De Souza E, Wilson J, Carpenter A, Gilchrist P, Lowery R, et al. High-intensity interval training has positive effects on performance in ice hockey players. Int J Sports Med. (2015) 36(01):61–6. doi: 10.1055/s-0034-1382054
9. Brocherie F, Perez J, Guilhem G. Effects of a 14-day high-intensity shock microcycle in high-level ice hockey players’ fitness. J Strength Cond Res. (2022) 36(8):2247–52. doi: 10.1519/JSC.0000000000003769
10. Rønnestad BR, Øfsteng SJ, Ellefsen S. Block periodization of strength and endurance training is superior to traditional periodization in ice hockey players. Scand J Med Sci Sports. (2019) 29(2):180–8. doi: 10.1111/sms.13326
11. Wilson JM, Marin PJ, Rhea MR, Wilson SM, Loenneke JP, Anderson JC. Concurrent training: a meta-analysis examining interference of aerobic and resistance exercises. J Strength Cond Res. (2012) 26(8):2293–307. doi: 10.1519/JSC.0b013e31823a3e2d
12. Jiménez-Reyes P, Samozino P, Brughelli M, Morin J-B. Effectiveness of an individualized training based on force-velocity profiling during jumping. Front Physiol. (2017) 7:677. doi: 10.3389/fphys.2016.00677
13. Lahti J, Jiménez-Reyes P, Cross MR, Samozino P, Chassaing P, Simond-Cote B, et al. Individual sprint force-velocity profile adaptations to in-season assisted and resisted velocity-based training in professional rugby. Sports. (2020) 8(5):74. doi: 10.3390/sports8050074
14. Samozino P, Rabita G, Dorel S, Slawinski J, Peyrot N, Saez de Villarreal E, et al. A simple method for measuring power, force, velocity properties, and mechanical effectiveness in sprint running. Scand J Med Sci Sports. (2016) 26(6):648–58. doi: 10.1111/sms.12490
15. Morin J-B, Samozino P. Interpreting power-force-velocity profiles for individualized and specific training. Int J Sports Physiol Perform. (2016) 11(2):267–72. doi: 10.1123/ijspp.2015-0638
16. Perez J, Guilhem G, Hager R, Brocherie F. Mechanical determinants of forward skating sprint inferred from off- and on-ice force-velocity evaluations in elite female ice hockey players. Eur J Sport Sci. (2021) 21(2):192–203. doi: 10.1080/17461391.2020.1751304
17. Perez J, Guilhem G, Brocherie F. Ice hockey forward skating force-velocity profiling using single unloaded vs. multiple loaded methods. J Strength Cond Res. (2022) 36(11):3229–33. doi: 10.1519/JSC.0000000000004078
18. Perez J, Guilhem G, Brocherie F. Reliability of the force-velocity-power variables during ice hockey sprint acceleration. Sports Biomech (2022) 21(1):56–70. doi: 10.1080/14763141.2019.1648541
19. Stenroth L, Vartiainen P, Karjalainen PA. Force-velocity profiling in ice hockey skating: reliability and validity of a simple, low-cost field method. Sports Biomech (2023) 22(7):874–89. doi: 10.1080/14763141.2020.1770321
20. Leiter JR, Cordingley DM, MacDonald PB. Development of anaerobic fitness in top-level competitive youth ice hockey players. J Strength Cond Res. (2018) 32(9):2612–5. doi: 10.1519/JSC.0000000000002403
21. Cordingley DM, Sirant L, MacDonald PB, Leiter JR. Three-year longitudinal fitness tracking in top-level competitive youth ice hockey players. J Strength Cond Res. (2019) 33(11):2909–12. doi: 10.1519/JSC.0000000000003379
22. Fernández-Galván LM, Jiménez-Reyes P, Cuadrado-Peñafiel V, Casado A. Sprint performance and mechanical force-velocity profile among different maturational stages in young soccer players. Int J Environ Res Public Health. (2022) 19(3):1412. doi: 10.3390/ijerph19031412
23. Jiménez-Reyes P, Cross M, Ross A, Samozino P, Brughelli M, Gill N, et al. Changes in mechanical properties of sprinting during repeated sprint in elite rugby sevens athletes. Eur J Sport Sci. (2019) 19(5):585–94. doi: 10.1080/17461391.2018.1542032
24. Hermosilla-Palma F, Loro-Ferrer JF, Merino-Muñoz P, Gómez-Álvarez N, Bustamante-Garrido A, Cerda-Kohler H, et al. Changes in the mechanical properties of the horizontal force-velocity profile during a repeated sprint test in professional soccer players. Int J Environ Res Public Health. (2022) 20(1):704. doi: 10.3390/ijerph20010704
25. Kyles A, Oliver JL, Cahill MJ, Lloyd RS, Pedley J. Linear and change of direction repeated sprint ability tests: a systematic review. J Strength Cond Res. (2023) 37(8):1703–17. doi: 10.1519/JSC.0000000000004447
26. Nightingale SC, Miller S, Turner A. The usefulness and reliability of fitness testing protocols for ice hockey players: a literature review. J Strength Cond Res. (2013) 27(6):1742–8. doi: 10.1519/JSC.0b013e3182736948
27. Devismes M, Aeles J, Philips J, Vanwanseele B. Sprint force-velocity profiles in soccer players: impact of sex and playing level. Sports Biomech (2021) 20(8):947–57. doi: 10.1080/14763141.2019.1618900
28. Haugen TA, Breitschädel F, Seiler S. Sprint mechanical properties in soccer players according to playing standard, position, age and sex. J Sports Sci. (2020) 38(9):1070–6. doi: 10.1080/02640414.2020.1741955
29. Marcotte-L’Heureux V, Charron J, Panenic R, Comtois AS. Ice hockey goaltender physiology profile and physical testing: a systematic review and meta-analysis. Int J Exerc Sci. (2021) 14(6):855.
30. McKay AK, Stellingwerff T, Smith ES, Martin DT, Mujika I, Goosey-Tolfrey VL, et al. Defining training and performance caliber: a participant classification framework. Int J Sports Physiol Perform. (2021) 17(2):317–31. doi: 10.1123/ijspp.2021-0451
31. Samozino P, Peyrot N, Edouard P, Nagahara R, Jimenez-Reyes P, Vanwanseele B, et al. Optimal mechanical force-velocity profile for sprint acceleration performance. Scand J Med Sci Sports. (2022) 32(3):559–75. doi: 10.1111/sms.14097
32. Simperingham KD, Cronin JB, Pearson SN, Ross A. Reliability of horizontal force–velocity–power profiling during short sprint-running accelerations using radar technology. Sports Biomech (2019) 18(1):88–99. doi: 10.1080/14763141.2017.1386707
33. Edwards T, Banyard HG, Piggott B, Haff GG, Joyce C. Reliability and minimal detectable change of sprint times and force-velocity-power characteristics. J Strength Cond Res. (2022) 36(1):268–72. doi: 10.1519/JSC.0000000000004161
34. Bringhurst RF, Wagner DR, Schwartz S. Wingate anaerobic test reliability on the Velotron with ice hockey players. J Strength Cond Res. (2020) 34(6):1716–22. doi: 10.1519/JSC.0000000000002458
35. Driller MW, Argus CK, Shing CM. The reliability of a 30-s sprint test on the Wattbike cycle ergometer. Int J Sports Physiol Perform. (2013) 8(4):379–83. doi: 10.1123/ijspp.8.4.379
36. Charron J, Garcia JEV, Roy P, Ferland PM, Comtois AS. Physiological responses to repeated running sprint ability tests: a systematic review. Int J Exerc Sci. (2020) 13(4):1190.33042370
37. Glaister M, Howatson G, Pattison JR, McInnes G. The reliability and validity of fatigue measures during multiple-sprint work: an issue revisited. J Strength Cond Res. (2008) 22(5):1597–601. doi: 10.1519/JSC.0b013e318181ab80
38. Hopkins W, Marshall S, Batterham A, Hanin J. Progressive statistics for studies in sports medicine and exercise science. Med Sci Sports Exerc. (2009) 41:3. doi: 10.1249/MSS.0b013e31818cb278
Keywords: anaerobic capacity, repeated-sprint ability, speed, power, force-velocity profile, performance
Citation: Glaude-Roy J, Pharand P, Brunelle J-F and Lemoyne J (2023) Exploring associations between sprinting mechanical capabilities, anaerobic capacity, and repeated-sprint ability of adolescent ice hockey players. Front. Sports Act. Living 5:1258497. doi: 10.3389/fspor.2023.1258497
Received: 14 July 2023; Accepted: 20 November 2023;
Published: 12 December 2023.
Edited by:
Jeppe Foged Vigh-Larsen, University of Southern Denmark, DenmarkReviewed by:
Jean Romain Riviere, Université Savoie Mont Blanc, FranceChristopher Kirk, Sheffield Hallam University, United Kingdom
© 2023 Glaude-Roy, Pharand, Brunelle and Lemoyne. This is an open-access article distributed under the terms of the Creative Commons Attribution License (CC BY). The use, distribution or reproduction in other forums is permitted, provided the original author(s) and the copyright owner(s) are credited and that the original publication in this journal is cited, in accordance with accepted academic practice. No use, distribution or reproduction is permitted which does not comply with these terms.
*Correspondence: Julien Glaude-Roy julien.glaude-roy@uqtr.ca