- 1Movement and Training Science, Faculty of Sport Science, Leipzig University, Leipzig, Germany
- 2HELIOS Klinikum Wuppertal, University of Witten/Herdecke, Wuppertal, Germany
- 3Department of Movement and Training Science, Faculty of Humanities and Social Sciences, Institute of Sport Science, University of Wuppertal, Wuppertal, Germany
Introduction: It is known that maximum oxygen uptake depends on age, sex, endurance capacity, and chronic heart failure. However, due to the required invasive or often applied non-continuous approaches, less is known on underlying central and peripheral factors. Thus, this study aimed to investigate the effects of age, sex, endurance capacity, and chronic heart failure on non-invasively and continuously measured central and peripheral factors of oxygen uptake.
Methods: 15 male children (11 ± 1 years), 15 male (24 ± 3 years) and 14 female recreationally active adults (23 ± 2 years), 12 male highly trained endurance athletes (24 ± 3 years), and 10 male elders (59 ± 6 years) and 10 chronic heart failure patients (62 ± 7 years) were tested during a cardiopulmonary exercise test on a cycling ergometer until exhaustion for: blood pressure, heart rate, stroke volume, cardiac output, cardiac power output, vastus lateralis muscle oxygen saturation, and (calculated) arterio-venous oxygen difference. For the non-invasive and continuous measurement of stroke volume and muscle oxygen saturation, bioreactance analysis and near-infrared spectroscopy were used, respectively. A two-factor repeated measure ANOVA and partial eta-squared effect sizes () were applied for statistical analyses at rest, 80, and 100% of oxygen uptake.
Results: For the age effect, there were statistically significant group differences for all factors (p ≤ .033; ). Concerning sex, there were group differences for all factors (p ≤ .010; ), except diastolic blood pressure and heart rate (p ≥ .698; ). For the effect of endurance capacity, there were no group differences for any of the factors (p ≥ .065; ). Regarding chronic heart failure, there were group differences for the heart rate and arterio-venous oxygen difference (p ≤ .037; ).
Discussion: Age, sex, endurance capacity, and chronic heart failure affect central and peripheral factors of oxygen uptake measured by non-invasive and continuous technologies. Since most of our findings support pioneer work using invasive or non-continuous measures, the validity of our applied technologies is indirectly confirmed. Our outcomes allow direct comparison between different groups serving as reference data and framework for subsequent studies in sport science and medicine aiming to optimise diagnostics and interventions in athletes and patients.
1. Introduction
Exercise capacity reflects the maximum amount of physical exertion a person can achieve (1). Its assessment is established in different settings, ranging from the evaluation of physical performance in athletes (2) to cardiorespiratory diseases in patients (3). Thereby, the assessment of maximum oxygen uptake during cardiopulmonary exercise testing is considered as the gold-standard approach (4). The oxygen uptake is defined by the Fick's equation as the product of cardiac output and arterio-venous oxygen difference (5). Thus, oxygen uptake depends on several central and peripheral factors, including the stroke volume and heart rate as well as mixed arterio-venous oxygen content, respectively (5). It is well known that maximum oxygen uptake depends on age, sex, endurance capacity, and diseases clearly limiting exercise capacity such as chronic heart failure (6–8). However, more research accounting for the underlying physiological factors on a central and peripheral level is needed.
Maximum oxygen uptake must be understood as a surrogate parameter, reflecting the interaction of the respiratory, cardiovascular, and muscular system (6). As maximum oxygen uptake is influenced by several factors (6), its estimation alone does not allow any clear conclusions to be drawn about the efficiency of the cardiopulmonary system and muscle tissue (9) or underlying causes of exercise intolerance (10). Therefore, it is essential to take central and peripheral factors of oxygen uptake into account for optimising diagnostics and interventions in both athletes and patients (9, 11). Due to the invasive or often non-continuous character of established technologies such as pulmonary artery catheter thermodilution or transoesophageal echocardiography and rebreathing technique or transthoracic echocardiography (12), respectively, new non-invasive and continuous approaches are promising to apply in research and practice (11).
In this context, thoracic bioreactance analysis and near-infrared spectroscopy are such technologies to estimate central and peripheral factors of oxygen uptake in a non-invasive and continuous manner (13, 14). In fact, cardiac output reflects the haemodynamic response to physical exercise in healthy participants (15) and patients (16), and cannot be predicted from maximum oxygen uptake alone (17, 18). However, the stroke volume can be measured using thoracic bioreactance analysis (14). Then, cardiac output using stroke volume and heart rate as well as cardiac power output additionally using (mean arterial) blood pressure can be predicted (11). Furthermore, near-infrared spectroscopy, particularly the thereby measured saturation of oxygen uptake in the skeletal muscle tissue, gives insight into the local perfusion and metabolic status (19–21). To date, such additional non-invasive and continuous approaches are still less applied during cardiopulmonary exercise testing. However, first results using these approaches to investigate central and peripheral factors of oxygen uptake simultaneously are promising (11).
It is clear, that age (22, 23), sex (23, 24), endurance capacity (23, 25), and chronic heart failure (11, 26) have an impact on maximum oxygen uptake and consequently also to the underlying central and peripheral factors. Previous studies investigating different age groups showed that children (22, 27) and older adults (23, 28) had lower values in central factors compared to young adults. Moreover, contradictory results were found concerning differences between younger and older adults on peripheral factors (21, 28). Regarding the differences between sexes, studies showed that male adults had higher values in central and peripheral factors than female adults (23, 24). Prior studies on endurance capacity revealed that trained adults possessed higher values in central (23, 25) but similar values in peripheral factors (23, 25, 28) compared to sedentary adults. Concerning chronic heart failure, it was shown that corresponding patients had lower values in central but similar values in peripheral factors compared to healthy controls (26, 29). However, most of these previous studies used invasive or non-continuous technologies. Such approaches are limited by the fact that they are not suitable for routine measurements due to the possible risk of infections or other complications (30, 31) as well as loss of information on physiological functions (32). Although previous studies built pioneer work in this research area, further studies with standardised, non-invasive, and continuous measurement approaches are needed to better understand differences in maximum oxygen uptake according to the underlying factors.
This study aimed to investigate the effects of age, sex, endurance capacity, and chronic heart failure on non-invasively and continuously measured central and peripheral factors of oxygen uptake. It was expected that there would be differences between the investigated groups assigned to effects. Our findings will increase the understanding of underlying factors of maximum oxygen uptake and may help to optimise specific diagnostics and interventions in sport science and medicine. Additionally, our study may indirectly question the validity of the non-invasive and continuous technologies and serve as an experimental framework for future studies. It should be noted that the data concerning chronic heart failure has already been published elsewhere (11) and was re-analysed here to associate with further reasonable populations.
2. Materials and methods
2.1. Participants
In total, 15 male children (11 ± 1 years), 15 male recreationally active adults (24 ± 3 years), 14 female recreationally active adults (23 ± 2 years), 12 male highly trained endurance athletes (24 ± 3 years), 10 male elders (59 ± 6 years), and 10 male chronic heart failure patients (62 ± 7 years) participated. For the examination of the four different effects, the groups were assigned as follows: (i) age: male children, male recreationally active adults, and male elders; (ii) sex: male and female recreationally active adults; (iii) endurance capacity: male recreationally active adults and male highly trained endurance athletes; and (iv) chronic heart failure: male elders (serving as healthy controls) and male chronic heart failure patients. The activity level of the recreationally active adults and the highly trained endurance athletes was defined according to the Participant Classification Framework (33). The participants were recruited from the sports students of the local university, different sports clubs, a local practice for cardiology, and the investigators' circle of acquaintances. Excluding the chronic heart failure patients, 25 different team or racquet (34%) and individual sports (66%) were performed by the participants, ranging from soccer, handball, and swimming to general fitness training, dancing, and horseback riding. Regarding the children, male, and female recreationally active adults, they were included in the study when they participated in 6 or less hours of sports per week, and their primary sport was not an endurance sport. The highly trained endurance athletes were included if they participated in cycling or triathlon competitions at least at a national amateur level. Concerning the elders and chronic heart failure patients, 3 or less hours of sports per week were allowed to participate. In case of the chronic heart failure patients, only 3 did any sport at all in form of cardiac rehabilitation (n = 1) and prevention programs (n = 2). Additionally, chronic heart failure patients were included when an ejection fraction of ≤35% was present to reduce the typical heterogeneity of chronic heart failure. Also, patients were individually medicated, with all taking beta-blockers. All participants stated that they had no acute or chronic diseases of the musculoskeletal system and those that militate against maximum exhaustion testing. Further descriptive characteristics on anthropometric characteristics and variables measured under maximum load of all six groups are presented in Table 1. All participants or, in the case of the children, the legal guardian signed a written informed consent. The study was approved by the Ethics Committee of the local university (MS/JE 29.11.11; MS/BB 180321).
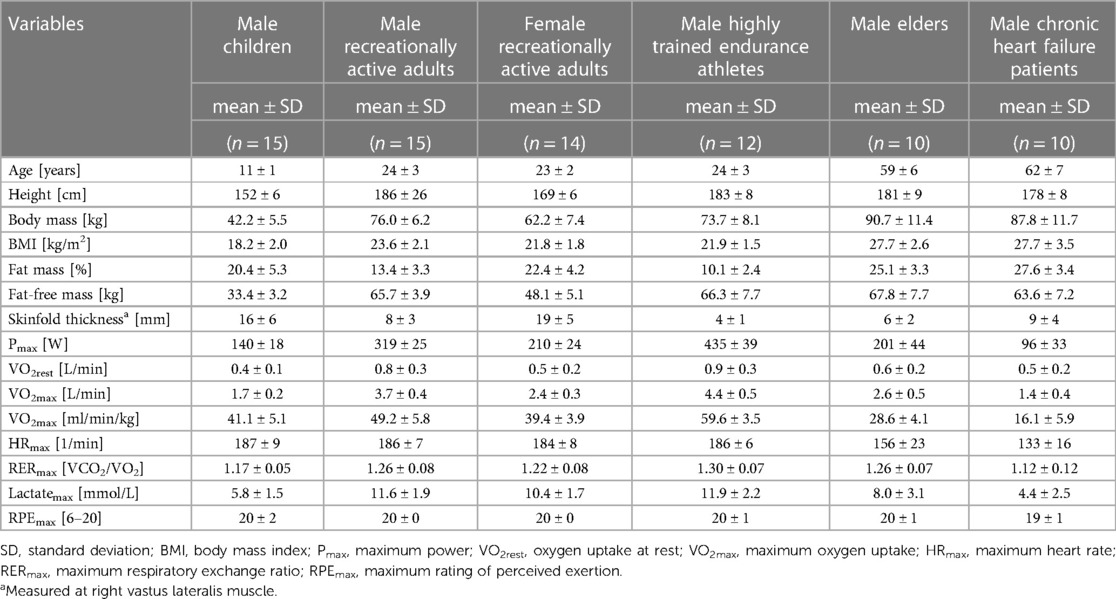
Table 1. Anthropometric characteristics and variables measured under maximum load of the participants from six groups recruited.
2.2. Study design
A cross-sectional design under laboratory conditions was applied. The measurements were carried out on a single day. The participants were instructed in advance not to eat any food and to only drink water for 2 h before testing. Furthermore, no strenuous exercises were allowed 24 h before the measurement. After anthropometric measures were taken, a cardiopulmonary exercise test until exhaustion on a cycling ergometer was performed. Here, the six aforementioned groups were the independent, while the dependent variables were as follows: oxygen uptake, systolic and diastolic blood pressure, mean arterial pressure, heart rate, stroke volume, cardiac output, cardiac power output, muscle oxygen saturation, and (calculated) arterio-venous oxygen difference. Importantly, in our study, the latter two dependent variables represented peripheral and the others central factors, as conducted before (11). During the measurements of the children and chronic heart failure patients a legal guardian and a cardiologist was present, respectively.
2.3. Anthropometric measurements
Using a 4-point bioelectric impedance analysis (Bodystat, Quadscan 4,000, Douglas, United Kingdom), body fat and fat-free mass were determined in supine position. Additionally, skinfold thickness of the right vastus lateralis muscle was measured using a calliper (Baseline® Medical Skinfold Caliper, Baseline® evaluation instruments, United States). The reason for determining the skinfold thickness was that interference with the near-infrared spectroscopy signal can occur due to the skin and subcutaneous tissue (34). The validity of the 4-point bioelectric impedance analyses is r = 0.98–0.99 (35).
2.4. Cardiopulmonary exercise test
The cardiopulmonary exercise test was performed on two different cycling ergometers. Generally, a stationary ergometer (Excalibur sport, Lode, Groningen, Netherlands) was used. To allow a more sport-specific testing for the highly trained endurance athletes, they brought their own bikes to be clamped in a mobile ergometer (Cyclus-2, RBM elektronik-automation, Leipzig, Germany). A ramp-like protocol was applied. For reaching comparable times to exhaustion, the initial as well as increasing load (every 60 s) was different between groups and conducted as follows: 5 W for chronic heart failure patients, 10 W for children and elders, 15 W for female recreationally active adults, 20 W for male recreationally active adults, and 30 W for highly trained endurance athletes. The load was aborted when the required pedalling frequency of 75–80 rpm could no longer be maintained (11). According to the initial loads of the corresponding test protocols, participants performed a 5-minute cool-down at 15, 30, 45, 60, and 90 W, respectively. To clarify exhaustion, three out of the following five criteria had to be reached, with only one being a subjective criterion: (i) heart rate ≥ 200 bpm-age; (ii) blood lactate ≥ 8 mmol/L; (iii) respiratory exchange ratio ≥1.1; (iv) rating of perceived exertion ≥19; and (v) visual analogue scale ≥60% of total exertion (36–38). However, there were adaptations for the children and chronic heart failure patients. Children had to reach two out of the following three criteria: (i) heart rate ≥95% of 200 bpm-age; (ii) blood lactate ≥ 4 mmol/L; and (iii) respiratory exchange ratio ≥1.0 (39, 40). Subjective criteria were not considered, as children tend to have a limited capacity for valid subjective assessment (41). For the chronic heart failure patients, clarification of exhaustion was solely based on subjective criteria, as conducted previously (11). During the cardiopulmonary exercise test, oxygen uptake was measured breath-by-breath using a gas analyser (Power-Cube Ergo, Ganshorn, Niederlauer, Germany). Prior to each testing, the system was calibrated according to the manufacturer's instructions. Additionally, every 60 s, systolic and diastolic blood pressure were measured manually on the left arm using a cuff and stethoscope (Boso Med-1, Bosch & Sohn, Jungingen, Germany). Equivalent mean arterial pressure was then calculated as described elsewhere (11). Immediately after reaching exhaustion, capillary blood was taken from the participant's earlobe to be analysed by an electro-enzymatic analyser (EKF-diagnostics, Biosen C_line Sport, Cardiff, United Kingdom) for the lactate concentration. The reliability of the stationary and mobile cycling ergometer, and gas and lactate analyser is CV = 8.2% (42), CV = 2.3% (43), ICC = 0.991–0.995 (44), and CV = 1.3% (45), respectively.
2.5. Bioreactance analysis
During the cardiopulmonary exercise test, non-invasively and continuously measured stroke volume and heart rate were recorded by a bioreactance analysis (Cheetah Nicom, Cheetah Medical, Vancouver, USA). The technology used is based on phase shifts, occurring when an alternating electric current with a frequency of 75 Hz is passed through the thorax. Then, stroke volume can be extrapolated from these phase shifts. Further details on the measurement principle can be found elsewhere (46). For measuring, eight electrodes were applied to the participant's back according to the manufacturer's instructions. Afterwards, cardiac output and cardiac power output, as the product of cardiac output and mean arterial pressure, were estimated as described elsewhere (11). All data were measured beat-by-beat. For the bioreactance analyses, validity and reliability are r = 0.82 (47) and ICC = 0.59–0.98 (48), respectively.
2.6. Near-infrared spectroscopy
Throughout the cardiopulmonary exercise test, muscle oxygen saturation of the right vastus lateralis muscle, as part of the main contribution to force production while cycling, was measured non-invasively and continuously by a near-infrared spectroscopy (Moxy Monitor, Fortiori Design LLC, Hutchinson, USA), as conducted previously (49). The technology is based on light waves ranging from 630 to 850 nm, which are sequentially being send from four light emitting diodes into the underlying tissue. The amount of returned scatted light is then recorded by two detectors. Further details on the measurement principle can be found elsewhere (49). According to the manufacturer's instructions, the near-infrared spectroscopy was surrounded by a light shield and then placed on the prominent part of the muscle belly of the vastus lateralis muscle. Data were measured at a frequency of 2 Hz. The reliability of the near-infrared spectroscopy is ICC = 0.77–0.99 (49). Additionally, as a peripheral factor, the arterio-venous oxygen difference was calculated from oxygen uptake and cardiac output using the Fick's principle.
2.7. Statistical analysis
For statistical analyses, all data collected during the cardiopulmonary exercise test were averaged over 60 s to fit its incremental stages. Then, they were considered at rest and interpolated at 80% and 100% of maximum oxygen uptake. For descriptive purposes, the relative oxygen uptake at all interpolated points was presented as well. The normal distributions of the data were checked by the Shapiro-Wilk test. A two-factor repeated measure ANOVA (group × time) was applied to examine the effects of age, sex, endurance training, and chronic heart failure. The assumption of sphericity was checked with the Mauchly's test and, if required, the degrees of freedom were adjusted using the Greenhouse–Geisser correction. Effect sizes were calculated using partial eta-squared (), with ≥.01 indicating small, ≥.059 medium, and ≥.138 large effects (50). Differences at rest, 80, and 100% of maximum oxygen uptake were calculated by post-hoc tests using Bonferroni correction. To increase the readability, the data are only presented in figures. The precise values can be calculated from the raw data provided in the Supplementary Material. For the discussion on central and peripheral factors of oxygen uptake, only the data provided by the figures and tested by the aforementioned statistical analyses were used.
3. Results
3.1. Age
Figure 1 shows the mean differences in central and peripheral factors of oxygen uptake between male children, recreationally active adults, and elders. There were statistically significant group differences (p ≤ .033) supported by large effect sizes () for all factors. Time differences were also statistically significant (p < .001) with large effect sizes () for all factors. Interaction differences were statistically significant for all factors (p ≤ .017) supported by large effect sizes (), with exception of the arterio-venous oxygen difference (p = .540; ).
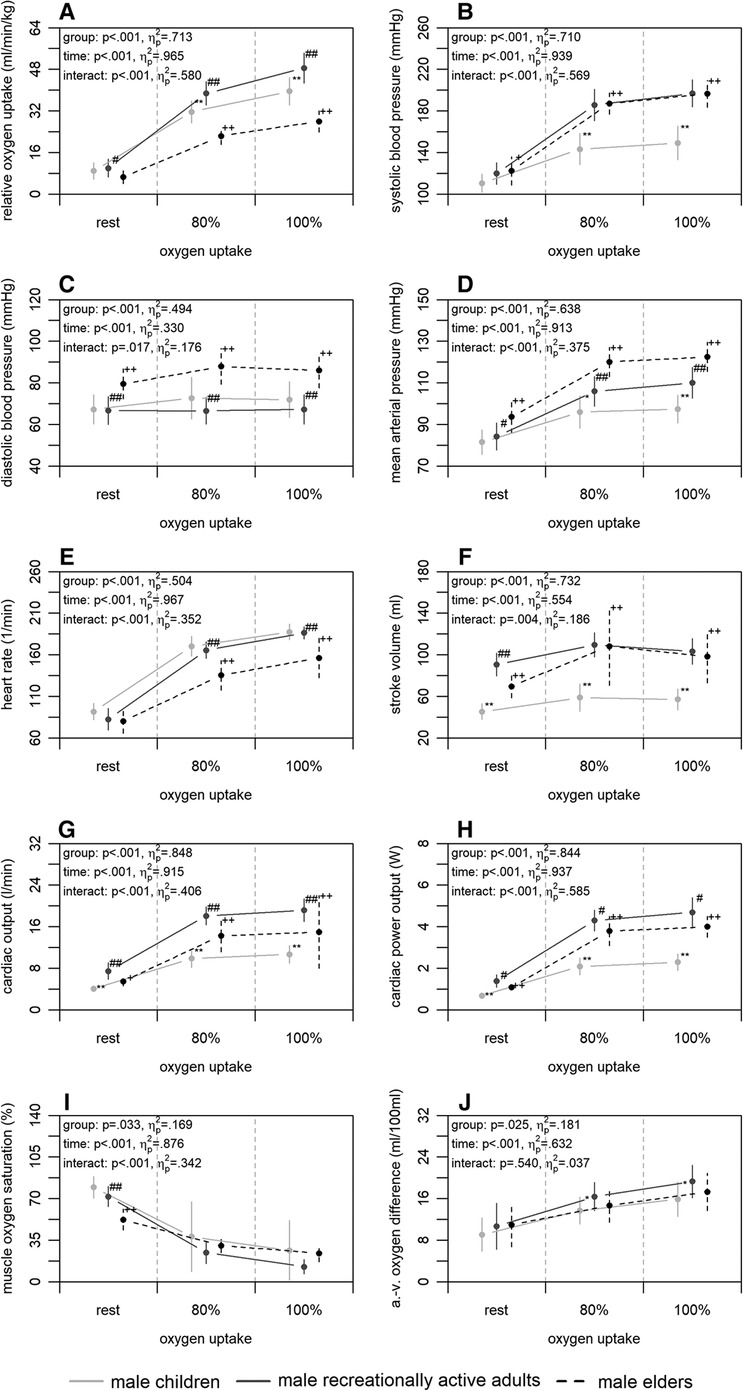
Figure 1. Relative oxygen uptake (A), central (B–H), and peripheral factors (I, J) of the male children, recreationally active adults, and elders at rest, 80, and 100% of maximum oxygen uptake. a.-v., arterio-venous. Means and standard deviations are shown. The asterisks * and ** indicate differences between children and adults at p < .05 and <.001, respectively. The hashes # and ## indicate differences between adults and elders at p < .05 and <.001, respectively. The plus signs + and ++ indicate differences between elders and children at p < .05 and <.001, respectively.
3.2. Sex
Figure 2 shows the mean differences in central and peripheral factors of oxygen uptake between male and female recreationally active adults. There were statistically significant group differences for all factors (p ≤ .010) with large effect sizes (), except for the diastolic blood pressure and heart rate (p ≥ .698; ). Time differences were statistically significant for all factors (p < .001) supported by large effect sizes (), with exception of the diastolic blood pressure (p = .133; ). Interaction differences were statistically significant for the systolic blood pressure, stroke volume, cardiac output, and cardiac power output (p ≤ .027) supported by large effect sizes ().
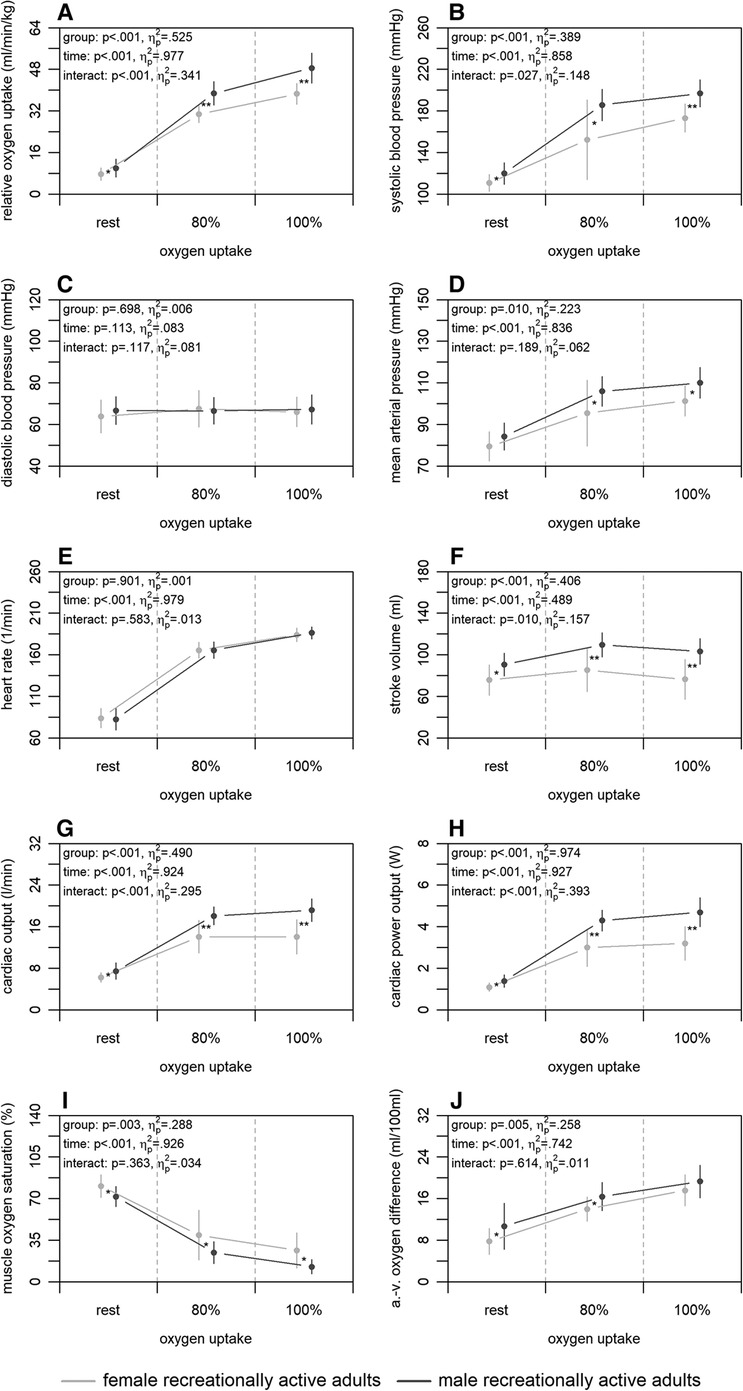
Figure 2. Relative oxygen uptake (A), central (B–H), and peripheral factors (I, J) of the male and female recreationally active adults at rest, 80, and 100% of maximum oxygen uptake. a.-v., arterio-venous. Means and standard deviations are shown. The asterisks * and ** indicate differences at p < .05 and <.001, respectively.
3.3. Endurance capacity
Figure 3 shows the mean differences in central and peripheral factors of oxygen uptake between highly trained endurance athletes and male recreationally active adults. There were no significant group differences for any of the factors (p ≥ .065) albeit up to medium effect sizes (). Time differences were statistically significant for all factors (p < .001) with large effect sizes (), with exception of the diastolic blood pressure (p = .218; ). Interaction differences were statistically significant for the stroke volume, cardiac output, and muscle oxygen saturation (p ≤ .004) supported by large effect sizes ().
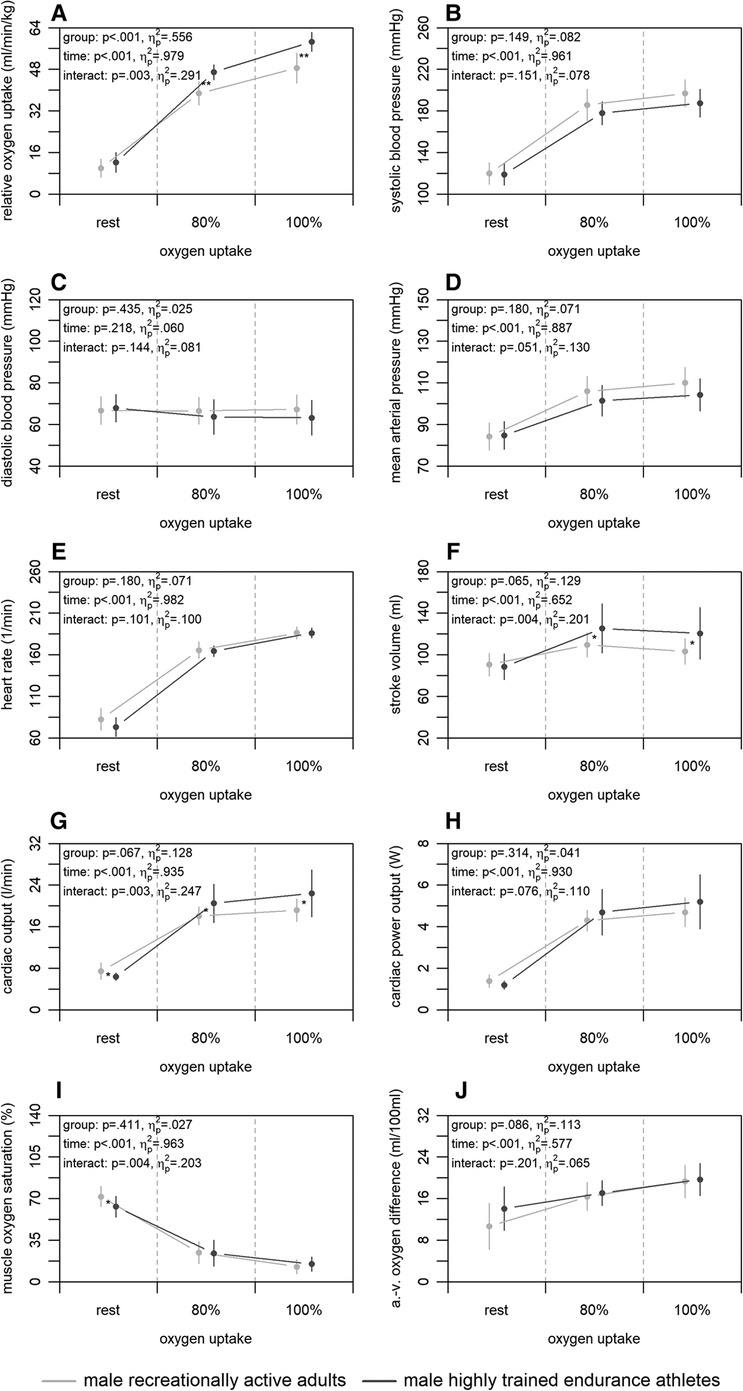
Figure 3. Relative oxygen uptake (A), central (B–H), and peripheral factors (I, J) of the male highly trained endurance athletes and recreationally active adults at rest, 80, and 100% of maximum oxygen uptake. a.-v., arterio-venous. Means and standard deviations are shown. The asterisks * and ** indicate differences at p < .05 and <.001, respectively.
3.4. Chronic heart failure
Figure 4 shows the mean differences in central and peripheral factors of oxygen uptake between male chronic heart failure patients and elders. There were statistically significant group differences for heart rate and arterio-venous oxygen difference (p ≤ .037) with large effect sizes (). For all factors, time differences were statistically significant (p < .001) supported by large effect sizes (), with exception of the arterio-venous oxygen difference (p = .068; ). Interaction differences were statistically significant for the systolic blood pressure, mean arterial pressure, heart rate, muscle oxygen saturation, and arterio-venous oxygen difference (p ≤ .014) supported by large effect sizes ().
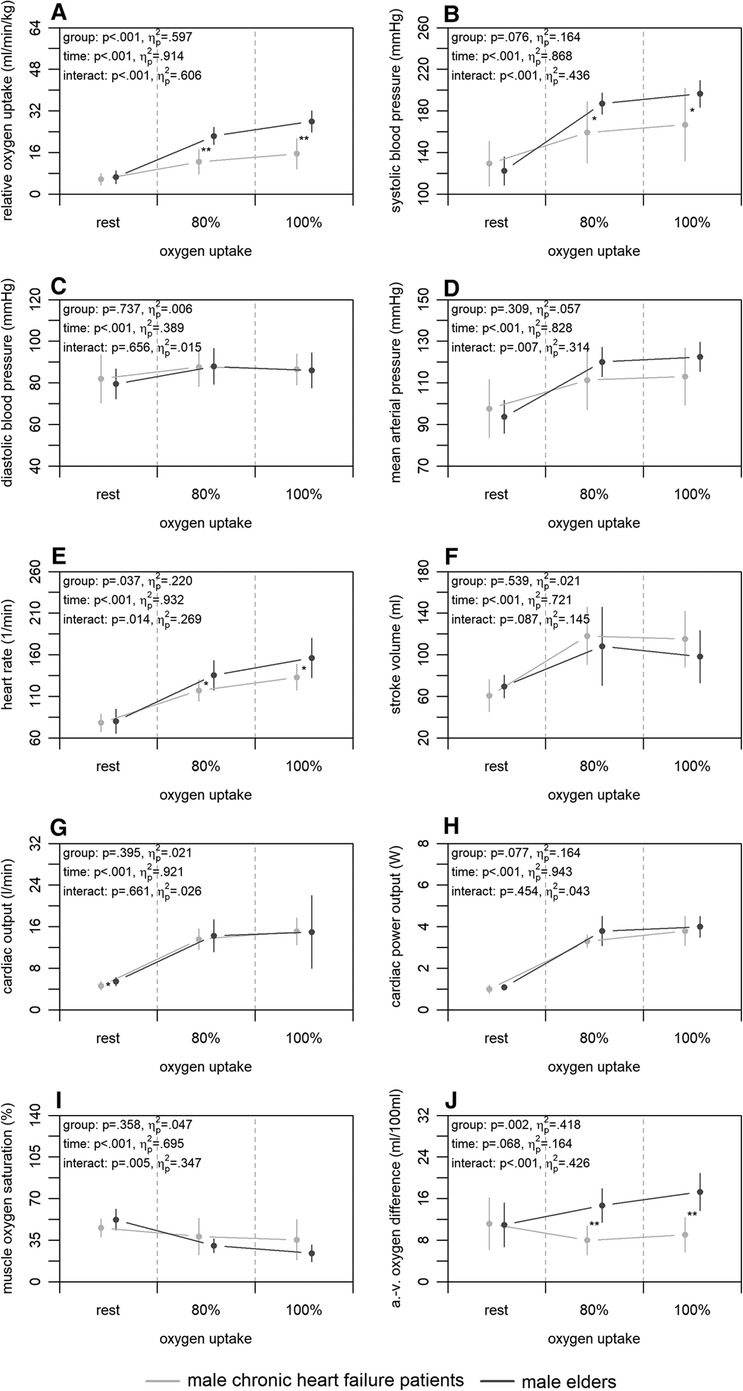
Figure 4. Relative oxygen uptake (A), central (B–H), and peripheral factors (I, J) of the male chronic heart failure patients and elders at rest, 80, and 100% of maximum oxygen uptake. a.-v., arterio-venous. Means and standard deviations are shown. The asterisks * and ** indicate differences at p < .05 and <.001, respectively.
4. Discussion
As far as we know, this is the first study to give an experimental framework of the effects of age, sex, endurance capacity, and chronic heart failure on non-invasively and continuously measured central and peripheral factors of oxygen uptake by the same standardised methodological approach. Our study revealed new insights into the relationship and differences between maximum oxygen uptake and underlying factors in six different groups and may help to optimise specific diagnostics and interventions in sport science and medicine as well as indirectly question the validity of the used non-invasive and continuous technologies. The main findings were that (i) between children, adults, and elders, central factors of oxygen uptake differed, whereas peripheral factors were similar with exception of the comparison between children and adults; and (ii) male and female adults showed differences in both central and peripheral factors. Also, (iii) male highly trained endurance athletes indicated higher central factors compared to male recreationally active adults, while peripheral factors were similar; and (iv) patients with chronic heart failure had similar values in central factors compared to elders, but peripheral factors differed.
4.1. Age
Concerning our first main finding, non-invasively and continuously measured central factors of oxygen uptake differed between age groups, while peripheral factors were similar with exception of the comparison between children and adults. Compared to the children, the recreationally active adults showed a higher relative maximum oxygen uptake (Figure 1A). This finding was accompanied by a higher stroke volume, cardiac output, and cardiac power output of the adults at 100% of maximum oxygen uptake (Figure 1F–H), as reported previously using a non-continuous rebreathing technique or transthoracic echocardiography (22, 27). Therefore, the higher maximum oxygen uptake of the adults could be due to the higher cardiac output and this in turn to the higher stroke volume compared to the children. These findings are plausible, as they are possibly caused by the generally larger heart volume of adults (51, 52). Furthermore, the adults showed a higher maximum arterio-venous oxygen difference and a tendency of a lower muscle oxygen saturation at 100% of maximum oxygen uptake (Figure 1I,J). These outcomes are also in line with previous studies calculating peripheral resistance (22, 27). The differences may possibly be caused by a higher haemoglobin concentration of the adults (51, 52) on the one hand. On the other, they could be due to differences in the ability of myoglobin to store oxygen or to facilitate oxygen diffusion into the mitochondria (53). Regardless of the reason, which cannot be precisely identified here, the findings indicate that the peripheral utilisation of oxygen seems to be limited in prepubescent age, requiring more research to clarify. The second comparison regarding the effect of age was between adults and elders. The results showed a higher relative maximum oxygen uptake of the adults (Figure 1A). Complementary, the adults had a higher heart rate, cardiac output, and cardiac power output at 100% of maximum oxygen uptake (Figure 1E,G,H). Previous studies also reported these findings applying non-continuous rebreathing techniques (23, 54); however, one additionally stated a lower stroke volume in younger compared to older adults (23). It is known that with increasing age, maximum heart rate decreases, which is an inevitable and irreversible process (55). Therefore, it can be concluded that the lower maximum heart rate of the elders resulting in a lower cardiac output is the main reason for the lower maximum oxygen uptake compared to adults. This can be assumed, since the peripheral factors of both groups were similar at 100% of maximum oxygen uptake (Figure 1I,J). Former studies calculating peripheral factors or using the invasive cyanmethemoglobin method also revealed these findings (23, 28). However, one study using near-infrared spectroscopy showed a higher peripheral utilisation of oxygen in younger compared to older adults (21). This could be due to a loss of capillaries and a decrease in volume density of the mitochondria and their enzyme activity in the muscle with increasing age (51, 52). The explanations given here for the age effect, are again confirmed by the last comparison between children and elders. The elders had a lower relative maximum oxygen uptake (Figure 1A). However, the elders showed a higher stroke volume, cardiac output, and cardiac power output but lower heart rate as well as similar peripheral factors at 100% of oxygen uptake (Figure 1E–J). Taken together, mainly central factors, and in adulthood especially heart rate, are influenced by age, whereas there only seems to be a small effect on the peripheral factors of oxygen uptake.
4.2. Sex
Our second main finding was that male and female recreationally active adults differ regarding non-invasively and continuously measured central and peripheral factors of oxygen uptake. The comparison showed a higher absolute and relative maximum oxygen uptake of the male adults (Figure 2A). At central level, males had a higher stroke volume, cardiac output, and cardiac power output at 100% of maximum oxygen uptake (Figure 2F–H). Similar results were also shown by a previous study using a non-continuous rebreathing technique (23), which detected a higher stroke volume and cardiac output in men compared to women, independent of age. It is morphologically known that males on average have a disproportionally larger heart compared to females (56), which may be the cause of the detected differences in stroke volume and cardiac output. The possible higher efficiency of the heart in males is supported by their equally higher cardiac power output (Figure 2H), even though females tend to have a higher ejection fraction (56). Additionally, a study (23) relativised stroke volume to fat-free mass to compensate for group differences. Doing the same with the results of the present study, it shows that the fat-free mass might be decisive for the higher stroke volume of the males (data not shown). Regarding the peripheral factors, our findings showed lower muscle oxygen saturation and higher arterio-venous oxygen difference of the males at 100% of maximum oxygen uptake (Figure 2I,J). Current literature calculating peripheral factors shows contradictory results (23, 24). However, these studies were conducted at submaximal load only or on a treadmill and may therefore not be completely comparable to our study. Nevertheless, our results suggest a higher peripheral utilisation of oxygen in males, which may result from the higher haemoglobin concentration (51, 52) or differences in myoglobin functions (53) compared to females.
4.3. Endurance capacity
The third main finding was that male highly trained endurance athletes had higher non-invasively and continuously measured central factors of oxygen uptake compared to recreationally active adults, while peripheral factors were similar. Here, the athletes showed a higher relative maximum oxygen uptake (Figure 3A). This was accompanied by a higher stroke volume and cardiac output, while heart rate and cardiac power output were similar at 100% of maximum oxygen uptake (Figure 3E–H). Earlier studies using non-continuous rebreathing techniques support these findings (23, 25). Only one study also applying a non-continuous rebreathing technique (28) did not detect differences in central factors between competitive and recreational runners. However, the study was conducted in a submaximal manner and the authors extrapolated the findings to a maximal setting. The results presented in our study may be caused by a physiologic hypertension of the heart muscle by endurance training (57). Furthermore, both groups showed similar muscle oxygen saturation and arterio-venous oxygen difference at 100% of maximum oxygen uptake (Figure 3I,J), which is also in line with former studies calculating peripheral factors or using the invasive cyanmethemoglobin method (23, 25, 28). Therefore, peripheral factors do not seem to be responsible for the higher oxygen uptake of the highly trained endurance athletes, even though previous studies show that endurance training leads to a capillarisation and an increase in volume density of mitochondria and their enzyme activity in the muscles (58, 59). Nevertheless, endurance training seems to have an effect on peripheral factors in females and elders (23), which was, however, not investigated in the current study.
4.4. Chronic heart failure
Concerning our last main finding, the chronic heart failure patients had similar values in non-invasively and continuously measured central factors of oxygen uptake compared to elders, but peripheral factors differed. Between both groups, the chronic heart failure patients showed a lower relative maximum oxygen uptake in patients (Figure 4A). At central level, only differences in heart rate, which was lower in patients, were detected at 100% of maximum oxygen uptake (Figure 4E). This is plausible as the lower heart rate may be caused by the intake of betablockers (60). Generally, our results on central factors differ from those revealed in previous studies. Two studies using a non-continuous rebreathing technique or transthoracic echocardiography (26, 29) showed lower stroke volume and cardiac output in chronic heart failure patients under maximum load. Yet, the ejection fraction of patients in both studies was similar to that in our patients and thus does not seem to be the reason for the inconsistent results. Regarding the peripheral factors, chronic heart failure patients showed lower arterio-venous oxygen difference and a tendency of muscle oxygen saturation being higher compared to elders at 100% of maximum oxygen uptake (Figure 4I,J). Again, these results are not in line with those of a former study applying catheters (26). Our results show a possibly lower peripheral oxygen utilisation of the chronic heart failure patients, which may be caused by effects such as muscle atrophy, reduction of mitochondrial density, and decrease of mitochondrial enzyme activity (61, 62) as well as reduced capillary density (63). These overall contradictory results throughout the literature illustrate that chronic heart failure cannot be generalised and depends on many other intra- and interindividual factors (64). Both central and peripheral factors can have an influence on the reduced maximum oxygen uptake in chronic heart failure patients regardless of their ejection fraction. Such knowledge can be used for exercise prescription (65), for which more interventional research is required.
5. Limitations
While our study increases the knowledge on central and peripheral factors of oxygen uptake, few limitations should be acknowledged. Firstly, the reliability of the applied bioreactance analysis and near-infrared spectroscopy decreases with increasing load (48, 49), which may have had an impact on our outcomes. Especially in the context of the applied bioreactance analysis, as a non-invasive and continuous approach to measure cardiac output, its validity is still controversially discussed (66). Although the implemented bioreactance analysis shows acceptable validity compared to thermodilution catheter in patients (47), more evaluation studies across different exercise modalities and loads are needed. Secondly, our study is limited by a not a priori conduced statistical power analysis and small sub-group sample sizes (n = 10–15) being mostly underpowered. However, in total, 76 participants were investigated by a standardised measurement approach. Additionally, effect sizes being independent of sample size were calculated. More research evaluating both non-invasive technologies in larger groups is needed. Thirdly, concerning the age and sex effect, we did not consider the biological age and menstrual cycle, respectively. This may have had an impact on our results and should be looked at in future studies for which our study can serve as a framework. Lastly, there is an ongoing discussion regarding criteria to clarify exhaustion (67). In our study, we carefully applied and modified the criteria of exhaustion for the different groups of participants from previous research (36–40). Based on the raw data during maximum load (Supplementary Material), we are sure that only exhausted participants were considered.
6. Conclusion
The present study shows that age, sex, endurance capacity, and chronic heart failure affect central and peripheral factors of oxygen uptake measured by non-invasive and continuous technologies. Since most of our findings support pioneer work using invasive or non-continuous measures, the validity of our applied technologies is indirectly confirmed. Our outcomes allow direct comparison between different groups serving as reference data and framework for subsequent studies in sport science and medicine aiming to optimise diagnostics and interventions in athletes and patients.
Data availability statement
The original contributions presented in the study are included in the article/Supplementary Material, further inquiries can be directed to the corresponding author.
Ethics statement
The studies involving humans were approved by the Ethics Commitee of the University of Wuppertal, Wuppertal, Germany (MS/JE 29.11.11; MS/BB 180321). The studies were conducted in accordance with the local legislation and institutional requirements. Written informed consent for participation in this study was provided by the participants’ legal guardians/next of kin.
Author contributions
JB, MTCB, and MWH contributed to the conception and design of the study. MWH supervised the study. JB and MTCB collected and organised the data. JB and DTW performed the statistical analysis. CB programmed the software. CB and DTW visualised the data. JB wrote the original draft of the manuscript. MTCB, CB, DTW, JF, and MWH reviewed and edited the original draft. All authors contributed to the article and approved the submitted version.
Acknowledgments
The authors would like to thank Lina Stawiarski, Lena Hövekamp, Laura Koenen, and Ken Oberließen for their assistance to collect the data. The authors acknowledge support from the German Research Foundation (DFG) and Leipzig University within the program of Open Access Publishing.
Conflict of interest
The authors declare that the research was conducted in the absence of any commercial or financial relationships that could be construed as a potential conflict of interest.
Publisher's note
All claims expressed in this article are solely those of the authors and do not necessarily represent those of their affiliated organizations, or those of the publisher, the editors and the reviewers. Any product that may be evaluated in this article, or claim that may be made by its manufacturer, is not guaranteed or endorsed by the publisher.
Supplementary material
The Supplementary Material for this article can be found online at https://www.frontiersin.org/articles/10.3389/fspor.2023.1218948/full#supplementary-material
References
1. Jette M, Sidney K, Blumchen G. Metabolic equivalents (METS) in exercise testing, exercise prescription, and evaluation of functional capacity. Clin Cardiol. (1990) 13(8):555–65. doi: 10.1002/clc.4960130809
2. Wells GD, Norris SR. Assessment of physiological capacities of elite athletes & respiratory limitations to exercise performance. Paediatr Respir Rev. (2009) 10(3):91–8. doi: 10.1016/j.prrv.2009.04.002
3. Ezzatvar Y, Izquierdo M, Nunez J, Calatayud J, Ramirez-Velez R, Garcia-Hermoso A. Cardiorespiratory fitness measured with cardiopulmonary exercise testing and mortality in patients with cardiovascular disease: a systematic review and meta-analysis. J Sport Health Sci. (2021) 10(6):609–19. doi: 10.1016/j.jshs.2021.06.004
4. Albouaini K, Egred M, Alahmar A, Wright DJ. Cardiopulmonary exercise testing and its application. Postgrad Med J. (2007) 83(985):675–82. doi: 10.1136/hrt.2007.121558
5. Levine BD. VO2max: what do we know, and what do we still need to know? J Physiol. (2008) 586(1):25–34. doi: 10.1113/jphysiol.2007.147629
6. Bassett DR Jr., Howley ET. Limiting factors for maximum oxygen uptake and determinants of endurance performance. Med Sci Sports Exerc. (2000) 32(1):70–84. doi: 10.1097/00005768-200001000-00012
7. Malhotra R, Bakken K, D'Elia E, Lewis GD. Cardiopulmonary exercise testing in heart failure. JACC Heart Fail. (2016) 4(8):607–16. doi: 10.1016/j.jchf.2016.03.022
8. Paap D, Takken T. Reference values for cardiopulmonary exercise testing in healthy adults: a systematic review. Expert Rev Cardiovasc Ther. (2014) 12(12):1439–53. doi: 10.1586/14779072.2014.985657
9. Kovacs R, Baggish AL. Cardiovascular adaptation in athletes. Trends Cardiovasc Med. (2016) 26(1):46–52. doi: 10.1016/j.tcm.2015.04.003
10. Wilson JR, Rayos G, Yeoh TK, Gothard P, Bak K. Dissociation between exertional symptoms and circulatory function in patients with heart failure. Circulation. (1995) 92(1):47–53. doi: 10.1161/01.cir.92.1.47
11. Brochhagen J, Coll Barroso MT, Baumgart C, Freiwald J, Hoppe MW. Non-invasively measured central and peripheral factors of oxygen uptake differ between patients with chronic heart failure and healthy controls. BMC Cardiovasc Disord. (2020) 20(1):378. doi: 10.1186/s12872-020-01661-4
12. Arya VK, Al-Moustadi W, Dutta V. Cardiac output monitoring—invasive and noninvasive. Curr Opin Crit Care. (2022) 28(3):340–7. doi: 10.1097/MCC.0000000000000937
13. Barroco AC, Sperandio PA, Reis M, Almeida DR, Neder JA. A practical approach to assess leg muscle oxygenation during ramp-incremental cycle ergometry in heart failure. Braz J Med Biol Res. (2017) 50(12):e6327. doi: 10.1590/1414-431X20176327
14. Jones TW, Houghton D, Cassidy S, MacGowan GA, Trenell MI, Jakovljevic DG. Bioreactance is a reliable method for estimating cardiac output at rest and during exercise. Br J Anaesth. (2015) 115(3):386–91. doi: 10.1093/bja/aeu560
15. Myers J, Gujja P, Neelagaru S, Burkhoff D. Cardiac output and cardiopulmonary responses to exercise in heart failure: application of a new bio-reactance device. J Card Fail. (2007) 13(8):629–36. doi: 10.1016/j.cardfail.2007.05.009
16. Klasnja AV, Jakovljevic DG, Barak OF, Popadic Gacesa JZ, Lukac DD, Grujic NG. Cardiac power output and its response to exercise in athletes and non-athletes. Clin Physiol Funct Imaging. (2013) 33(3):201–5. doi: 10.1111/cpf.12013
17. Myers J, Froelicher VF. Hemodynamic determinants of exercise capacity in chronic heart failure. Ann Intern Med. (1991) 115(5):377–86. doi: 10.7326/0003-4819-115-5-377
18. Nicoletti I, Cicoira M, Zanolla L, Franceschini L, Brighetti G, Pilati M, et al. Skeletal muscle abnormalities in chronic heart failure patients: relation to exercise capacity and therapeutic implications. Congest Heart Fail. (2003) 9(3):148–54. doi: 10.1111/j.1527-5299.2002.01219.x
19. Marin T, Moore J. Understanding near-infrared spectroscopy. Adv Neonatal Care. (2011) 11(6):382–8. doi: 10.1097/ANC.0b013e3182337ebb
20. Barstow TJ. Understanding near infrared spectroscopy and its application to skeletal muscle research. J Appl Physiol. (2019) 126(5):1360–76. doi: 10.1152/japplphysiol.00166.2018
21. Ferri A, Adamo S, Longaretti M, Marzorati M, Lanfranconi F, Marchi A, et al. Insights into central and peripheral factors affecting the “oxidative performance” of skeletal muscle in aging. Eur J Appl Physiol. (2007) 100(5):571–9. doi: 10.1007/s00421-006-0371-x
22. Cheatham CC, Mahon AD, Brown JD, Bolster DR. Cardiovascular responses during prolonged exercise at ventilatory threshold in boys and men. Med Sci Sports Exerc. (2000) 32(6):1080–7. doi: 10.1097/00005768-200006000-00007
23. Ogawa T, Spina RJ, Martin WH 3rd, Kohrt WM, Schechtman KB, Holloszy JO, et al. Effects of aging, sex, and physical training on cardiovascular responses to exercise. Circulation. (1992) 86(2):494–503. doi: 10.1161/01.cir.86.2.494
24. Wheatley CM, Snyder EM, Johnson BD, Olson TP. Sex differences in cardiovascular function during submaximal exercise in humans. Springerplus. (2014) 3:445. doi: 10.1186/2193-1801-3-445
25. Yamaji K, Miyashita M. Differences in cardio-respiratory responses to exhaustive exercise between athletes and non-athletes. Eur J Appl Physiol Occup Physiol. (1978) 38(4):233–8. doi: 10.1007/BF00423106
26. Esposito F, Mathieu-Costello O, Shabetai R, Wagner PD, Richardson RS. Limited maximal exercise capacity in patients with chronic heart failure: partitioning the contributors. J Am Coll Cardiol. (2010) 55(18):1945–54. doi: 10.1016/j.jacc.2009.11.086
27. Nottin S, Vinet A, Stecken F, Nguyen LD, Ounissi F, Lecoq AM, et al. Central and peripheral cardiovascular adaptations during a maximal cycle exercise in boys and men. Med Sci Sports Exerc. (2002) 34(3):456–63. doi: 10.1097/00005768-200203000-00012
28. Hagberg JM, Allen WK, Seals DR, Hurley BF, Ehsani AA, Holloszy JO. A hemodynamic comparison of young and older endurance athletes during exercise. J Appl Physiol. (1985) 58(6):2041–6. doi: 10.1152/jappl.1985.58.6.2041
29. Pugliese NR, Fabiani I, Mandoli GE, Guarini G, Galeotti GG, Miccoli M, et al. Echo-derived peak cardiac power output-to-left ventricular mass with cardiopulmonary exercise testing predicts outcome in patients with heart failure and depressed systolic function. Eur Heart J Cardiovasc Imaging. (2019) 20(6):700–8. doi: 10.1093/ehjci/jey172
30. Lavdaniti M. Invasive and non-invasive methods for cardiac output measurement. Int J Caring Sci. (2008) 1(3):112–7.
31. Peyton PJ, Wallin M, Hallbäck M. New generation continuous cardiac output monitoring from carbon dioxide elimination. BMC Anesthesiol. (2019) 19:28. doi: 10.1186/s12871-019-0699-5
32. Kouz K, Michard F, Bergholz A, Vokuhl C, Briesenick L, Hoppe P, et al. Agreement between continuous and intermittent pulmonary artery thermodilution for cardiac output measurement in perioperative and intensive care medicine: a systematic review and meta-analysis. Crit Care. (2021) 25(1):125. doi: 10.1186/s13054-021-03523-7
33. McKay AKA, Stellingwerff T, Smith ES, Martin DT, Mujika I, Goosey-Tolfrey VL, et al. Defining training and performance caliber: a participant classification framework. Int J Sports Physiol Perform. (2022) 17(2):317–31. doi: 10.1123/ijspp.2021-0451
34. Niemeijer VM, Spee RF, Schoots T, Wijn PF, Kemps HM. Limitations of skeletal muscle oxygen delivery and utilization during moderate-intensity exercise in moderately impaired patients with chronic heart failure. Am J Physiol Heart Circ Physiol. (2016) 311(6):H1530–H9. doi: 10.1152/ajpheart.00474.2016
35. Evans M, Nguo K, Boneh A, Truby H. The validity of bioelectrical impedance analysis to measure body composition in phenylketonuria. JIMD Rep. (2018) 42:37–45. doi: 10.1007/8904_2017_75
36. Löllgen H, Leyk D. Exercise testing in sports medicine. Dtsch Arztebl Int. (2018) 115:409–16. doi: 10.3238/arztebl.2018.0409
37. Steinacker JM, Liu Y, Reißnecker S. Abbruchkriterien bei der ergometrie. Dtsch Z Sportmed. (2002) 53(7-8):228–9.
38. Wisloff U, Stoylen A, Loennechen JP, Bruvold M, Rognmo O, Haram PM, et al. Superior cardiovascular effect of aerobic interval training versus moderate continuous training in heart failure patients: a randomized study. Circulation. (2007) 115(24):3086–94. doi: 10.1161/CIRCULATIONAHA.106.675041
39. Armstrong N, Welsman JR. Peak oxygen uptake in relation to growth and maturation in 11- to 17-year-old humans. Eur J Appl Physiol. (2001) 85(6):546–51. doi: 10.1007/s004210100485
40. Bar-Or O. New and old in pediatric exercise physiology. Int J Sports Med. (2000) 21(Suppl 2):S113–6. doi: 10.1055/s-2000-8502
41. Bar-Or O. Rating of perceived exertion in children and adolescents: clinical aspects. In: Ljunggren G, D’omic S, editors. Psychophysics in action. Berlin, Heidelberg: Springer (1989). p. 105–13.
42. Earnest CP, Wharton RP, Curch TS, Lucia A. Reliability of the lode excalibur sport ergometer and applicability to computrainer electromagnetically braked cycling training device. J Strength Conditioning Res. (2005) 19(2):344–8. doi: 10.1519/R-15714.1
43. Rodger SM, Plews DJ, McQuillan J, Driller MW. Evaluation of the Cyclus ergometer and the stages power meter against the SRM crankset for measurement of power output in cycling. J Sci Cycling. (2016) 5(3):16–22.
44. Hoppe MW, Sperlich B, Baumgart C, Janssen M, Freiwald J. Reliabilität ausgewählter parameter der fahrraderdospirometrie anhand des PowerCube-ergo-atemgasanalysators. Sportverl Schportschad. (2015) 29:173–9. doi: 10.1055/s-0034-1399096
45. Nowotny B, Nowotny PJ, Strassburger K, Roden M. Precision and accuracy of blood glucose measurements using three different instruments. Diabet Med. (2012) 29(2):260–5. doi: 10.1111/j.1464-5491.2011.03406.x
46. Marik PE. Noninvasive cardiac output monitors: a state-of the-art review. J Cardiothorac Vasc Anesth. (2013) 27(1):121–34. doi: 10.1053/j.jvca.2012.03.022
47. Squara P, Denjean D, Estagnasie P, Brusset A, Dib JC, Dubois C. Noninvasive cardiac output monitoring (NICOM): a clinical validation. Intensive Care Med. (2007) 33(7):1191–4. doi: 10.1007/s00134-007-0640-0
48. Barroso MT C, Hoppe MW, Boehme P, Krahn T, Kiefer C, Kramer F, et al. Test-retest reliability of non-invasive cardiac output measurement during exercise in healthy volunteers in daily clinical routine. Arq Bras Cardiol. (2019) 113(2):231–9. doi: 10.5935/abc.20190116
49. Crum EM, O'Connor WJ, Van Loo L, Valckx M, Stannard SR. Validity and reliability of the Moxy oxygen monitor during incremental cycling exercise. Eur J Sport Sci. (2017) 17(8):1037–43. doi: 10.1080/17461391.2017.1330899
50. Cohen J. Statistical power analysis for the behavioral sciences. 2nd ed. Hillsdale NJ: Erlbaum (1988).
53. Kamga C, Krishnamurthy S, Shiva S. Myoglobin and mitochondria: a relationship bound by oxygen and nitric oxide. Nitric Oxide. (2012) 26(4):251–8. doi: 10.1016/j.niox.2012.03.005
54. Ridout SJ, Parker BA, Smithmyer SL, Gonzales JU, Beck KC, Proctor DN. Age and sex influence the balance between maximal cardiac output and peripheral vascular reserve. J Appl Physiol. (2010) 108(3):483–9. doi: 10.1152/japplphysiol.00985.2009
55. Christou DD, Seals DR. Decreased maximal heart rate with aging is related to reduced beta-adrenergic responsiveness but is largely explained by a reduction in intrinsic heart rate. J Appl Physiol. (2008) 105(1):24–9. doi: 10.1152/japplphysiol.90401.2008
56. St. Pierre SRS, Peirlinck M, Kuhl E. Sex matters: a comprehensive comparison of female ad male hearts. Front Physiol. (2022) 13:831179. doi: 10.3389/fphys.2022.831179
57. Pluim BM, Zwinderman AH, van der Laarse A, van der Wall EE. The athlete's Heart. A meta-analysis of cardiac structure and function. Circulation. (2000) 101(3):336–44. doi: 10.1161/01.cir.101.3.336
58. Andersen P, Henriksson J. Capillary supply of the quadriceps femoris muscle of man: adaptive response to exercise. J Physiol. (1977) 270(3):677–90. doi: 10.1113/jphysiol.1977.sp011975
59. Hoppeler H, Howald H, Conley K, Lindstedt SL, Claassen H, Vock P, et al. Endurance training in humans: aerobic capacity and structure of skeletal muscle. J Appl Physiol. (1985) 59(2):320–7. doi: 10.1152/jappl.1985.59.2.320
60. Hollmann W, Strüder HK, Predel HG, Tagarakis CVM. Spiroergometrie. Stuttgart: Schattauer (2006).
61. Drexler H, Riede U, Munzel T, Konig H, Funke E, Just H. Alterations of skeletal muscle in chronic heart failure. Circulation. (1992) 85(5):1751–9. doi: 10.1161/01.cir.85.5.1751
62. Massie BM, Simonini A, Sahgal P, Wells L, Dudley GA. Relation of systemic and local muscle exercise capacity to skeletal muscle characteristics in men with congestive heart failure. J Am Coll Cardiol. (1996) 27(1):140–5. doi: 10.1016/0735-1097(95)00416-5
63. Duscha BD, Kraus WE, Keteyian SJ, Sullivan MJ, Green HJ, Schachat FH, et al. Capillary density of skeletal muscle: a contributing mechanism for exercise intolerance in class II-III chronic heart failure independent of other peripheral alterations. J Am Coll Cardiol. (1999) 33(7):1956–63. doi: 10.1016/s0735-1097(99)00101-1
64. Francis GS, Cogswell R, Thenappan T. The heterogeneity of heart failure: will enhanced phenotyping be necessary for future clinical trial success? J Am Coll Cardiol. (2014) 64(17):1775–6. doi: 10.1016/j.jacc.2014.07.978
65. Lamberti V, Palermi S, Franceschin A, Scapol G, Lamberti V, Lamberti C, et al. The effectiveness of adapted personalized motor activity (AMPA) to improve health in individuals with mental disorders and physical comorbidities: a randomized controlled trial. Sports (Basel). (2022) 10(3):30–40. doi: 10.3390/sports10030030
66. Pestel G, Fukui K, Higashi M, Schmidtmann I, Werner C. Metaanlysen zur messgenauigkeit nichtinvasiver hämodynamischer Überwachungstechnologien bei erwachsenen. Die Anaesthesiologie. (2018) 67:409–25. doi: 10.1007/s00101-018-0452-3
Keywords: catheter, exercise physiology, exercise testing, haemodynamics, new technologies
Citation: Brochhagen J, Coll Barroso MT, Baumgart C, Wasmus DT, Freiwald J and Hoppe MW (2023) Age, sex, endurance capacity, and chronic heart failure affect central and peripheral factors of oxygen uptake measured by non-invasive and continuous technologies: support of pioneer work using invasive or non-continuous measures. Front. Sports Act. Living 5:1218948. doi: 10.3389/fspor.2023.1218948
Received: 9 May 2023; Accepted: 21 August 2023;
Published: 5 September 2023.
Edited by:
Luca Paolo Ardigò, NLA University College, NorwayReviewed by:
Stefano Palermi, University of Naples Federico II, ItalySusanna Rampichini, Università degli studi di Milano, Italy
© 2023 Brochhagen, Coll Barroso, Baumgart, Wasmus, Freiwald and Hoppe. This is an open-access article distributed under the terms of the Creative Commons Attribution License (CC BY). The use, distribution or reproduction in other forums is permitted, provided the original author(s) and the copyright owner(s) are credited and that the original publication in this journal is cited, in accordance with accepted academic practice. No use, distribution or reproduction is permitted which does not comply with these terms.
*Correspondence: Joana Brochhagen am9hbmEuYnJvY2hoYWdlbkB1bmktbGVpcHppZy5kZQ==
†These authors have contributed equally to this work and share first authorship