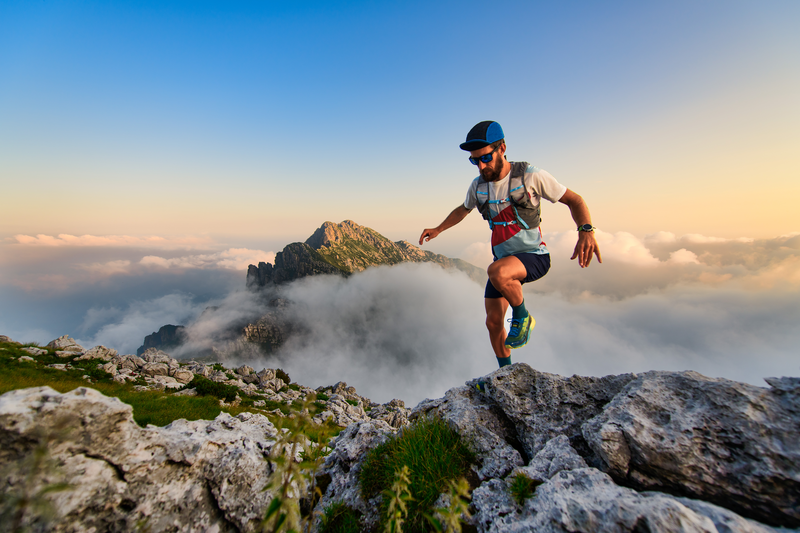
94% of researchers rate our articles as excellent or good
Learn more about the work of our research integrity team to safeguard the quality of each article we publish.
Find out more
ORIGINAL RESEARCH article
Front. Sports Act. Living , 24 October 2023
Sec. Sports Science, Technology and Engineering
Volume 5 - 2023 | https://doi.org/10.3389/fspor.2023.1213655
Introduction: Heart rate (HR) monitors are rarely used by people living with disabilities (PLWD), and their accuracy is undocumented. Thus, this study aims to describe the HR response during the Team Twin co-running program and, secondly, to assess the agreement and accuracy of using HR monitors among PLWD.
Methods: This 16-week single-arm observational study included 18 people with various disabilities. During the study, the subjects wore a Garmin Vivosmart 4 watch (wrist). To evaluate the agreement and accuracy we applied Garmin’s HRM-DUAL™ chest-worn HR monitors for comparison with the Vivosmart 4. The HR response analysis was performed descriptively and with a mixed regression model. The HR agreement and accuracy procedure was conducted on a subsample of five subjects and analyzed using Lin’s concordance analysis, Bland and Altman’s limits of agreement, and Cohen’s kappa analysis of intensity zone agreement. This study was prospectively registered at Clinical Trials.gov (NCT04536779).
Results: The subjects had a mean age of 35 (±12.6), 61% were male, 72% had cerebral palsy were 85% had GMFCS V-IV. HR was monitored for 202:10:33 (HH:MM:SS), with a mean HR of 90 ± 17 bpm during training and race. A total of 19% of the time was spent in intensity zones between light and moderate (30%–59% HR reserve) and 1% in vigorous (60%–84% HR reserve). The remaining 80% were in the very light intensity zone (<29% HR reserve). HR was highest at the start of race and training and steadily decreased. Inter-rater agreement was high (k = 0.75), limits of agreement were between −16 and 13 bpm, and accuracy was acceptable (Rc = 0.86).
Conclusion: Disability type, individual, and contextual factors will likely affect HR responses and the agreement and accuracy for PLWD. The Vivosmart 4, while overall accurate, had low precision due to high variability in the estimation. These findings implicate the methodical and practical difficulties of utilizing HR monitors to measure HR and thus physical activity in adapted sports activities for severely disabled individuals.
People living with disabilities (PLWD), are heavily affected by their disability, which, for the majority, result in significantly poorer health, sedentary behavior, and premature death (compared to the general population). Premature death increases with severity of disability and with time (1–6). Adapted sports and physical activity (PA) have well-documented health benefits for PLWD (7, 8). Due to the functional limitations posed by disability, PLWD are often unable to participate in PA (9–14). Wheelchair-dependent individuals see the fewest opportunities for participation in PA (15). In Denmark, the Team Twin organization offers participation in indirect PA (“an activity where a person unable to partake in physical activity on their own, is joined by someone who is able, and together partake in PA” (16 p. 2)) for wheelchair-dependent persons, conducted in a social community consisting of PLWD and able-bodied volunteers. A previous pilot study from 2018 indicates that Team Twin participation is associated with increased social (e.g., connections and relations with peers and volunteers) and mental health (e.g., developing an identity) for PLWD subjects (17). The researchers monitored participants’ heart rate (HR) during Team Twin training sessions, further indicating intensity levels consistent with moderate to vigorous PA (17). Other indirect PA studies examine HR responses in therapeutic riding and power wheelchair soccer among PLWD, found similar HR-responses (18–20). Thus, there is an unresolved potential for severely disabled persons to gain physiological benefits from indirect PA. However, the evidence base is yet uncertain and more studies using rigorous and valid methodologies are needed to determine whether a physiological response occurs when participating in indirect PA.
Monitoring of HR (bpm), usually placed on the wrist or chest, is a non-invasive and commonly used proxy method for examining exercise intensity, and used in general populations, rehabilitation, and disease prevention programs (21). HR sensors have lately gained popularity in disability research (22). They are used to determine PA and intensity levels (21, 23), and chest ECG HR monitors have shown superior accuracy over wrist worn PPG, compared to the golden standard, standard medical ECG HR monitors (24–30). Recent reviews, evaluation designs, and adaptation of sports and rehabilitation programs find variance in the devices’ abilities (including accuracy and agreement) to assess biomechanical, locomotion, and physiological parameters of PA among PLWD, however mainly among high-performance athletes (e.g., Paralympics) (22, 31). Furthermore, the authors of the reviews address the major challenge of applying wearable technologies for PLWD resulting in a sizeable inter-subject variability and sufficiently lack of accuracy (22, 31). Moreover, the literature about HR monitors among severely disabled persons who participate in recreational PA is insufficient, and the validity of HR monitors could be challenged more due to various disabilities (affecting limbs, bodily posture, and movement). Lack of accuracy affected by time variations, wearing of the device, exercise type, context (laboratory vs. real world setting), individual behaviors, health condition, and applied devices (27–29, 32–35), highlights the importance of future research validating wearable sensors. Especially in specific populations, including PLWD (22, 27, 36), to assess the method suitability when using HR monitors to prescribe PA and intensity among PLWD who participate in recreation physical activities, where HR monitors are scarcely used.
Thus, this 16-week single-arm observational study primarily aims to describe HR response during participation of PLWD in the Team Twin co-running program and, secondly, to assess the agreement and accuracy of using HR monitoring in a subset of the population and context.
This is an observational, single-arm study with no control group. The study is a sub-project from the “When Movement Moves”, described elsewhere (16). During a 16-week program, subjects wore a wrist-based HR monitor. The study was conducted in concordance with the Declaration of Helsinki (37), registered and approved by the Ethics Committee of Denmark’s Capital Region (Journal no.: H-20010668), including a pre-registration at Clinical Trials.gov (NCT04536779). All subjects gave written informed consent prior to inclusion.
The Team Twin organization provides an adapted running activity for PLWD (henceforth referred to as handiathletes) and has ten clubs across Denmark. It has approximately 450 members, 150 handiathletes and 300 volunteer-runners (38).
The activity has the same format across the individual Team Twin clubs. A standard session is comprised of three phases, the before, during, and after phases. Sessions are typically scheduled on Sunday mornings. Before; runners and athletes meet and handiathletes are assisted in transferring from their wheelchair to running chair. The route is planned, and members and associates have casual conversations. During; co-running, handiathletes are sat in their running chairs driven by a runner. After; casual conversation, eating, and re-hydrating before end of session. The organization furthermore participate in numerous official races (half- and full marathons and other running events) national and international during their season.
We invited all 150 handiathlete members to participate. Between January and March 2021, 22 were enrolled (15%), representing six clubs. One withdrew after enrolment, and three either dropped out and or were excluded for non-adherence. Thus, 18 handiathletes were included in the analyses of this study (flowchart in Supplementary Figure S1). Inclusion criteria were 1) affiliation to a Team Twin club and 2) 18 + years of age. Characteristics of the sample were obtained between April and June, 2021. The study period began mid-June and finished in October 2021.
All handiathletes were equipped with a Garmin Vivosmart 4 watch (39). The Vivosmart 4 was selected based on a practical approach taking the population into account. Following practical criteria was essential for the device selection, 1) suitable for relatively small wrists (due to disabilities) to fit all subjects properly, 2) comfortable to wear day and night for four months, to strengthen compliance, 3) long battery life (7 days) to avoid recharging daily and limit interference with the subject’s everyday life. The Vivosmart 4 did comprise the required features for the overall study (16) (sleep and HR monitoring) and the price accommodated the project budget. We personalized the devices based on the participants’ age, sex, height, and weight. All widgets (e.g., total steps per day and body battery (39)) were pre-emptively removed from the display to prevent interference. All obtained data were uploaded to individual Garmin profiles only accessible for the research team. All handiathletes, relatives and caretakers received a comprehensive walkthrough of the Vivosmart 4 (39). We employed the “Running” activity profile provided by Garmin (39) for tracking Team Twin sessions. We instructed the handiathletes and volunteer-runners to activate/deactivate to track only during the actual co-running (the “during”-phase).
The Vivosmart 4 was placed on the athlete’s preferred wrist. It was worn both day and night, the latter to estimate resting heart rate (RHR) with the highest accuracy (40). RHR was estimated from the lowest 30-minute average calculated over the 24 h (40). We excluded the first two weeks of the RHR-data to gain further accuracy to accommodate a subject-specific calibration period (41).
We estimated peak heart rate (HRmax) using Fernhall et al.’s formula (42), which takes disability into account. We calculated the individual heart rate reserve (HRR) by estimating a mean RHR across the whole period (excluding the calibration weeks described above) and subtracting it from the estimated HRmax (HRR = HRmax-RHR). Based on the HRR, we determined %HRR (resulting in intensity levels) by the equation from Karvonen (43). Intensity levels based on HRR instead of HR-dependent estimation (e.g., %HRmax) is recommended for avoiding over- or underestimation. The HRR method consider 1) individual assessment; which reflects the RHR, which differs from person to person, and thus less sensitive to over- or underestimation, 2) accuracy in submaximal exercise; more accurate to predict exercise intensity at submaximal intensity levels due to various factors like stress, fatigue, or medication use, and 3) cardiorespiratory level: individuals with higher fitness levels tend to have lower RHR resulting in a larger HRR and potentially higher exercise intensities. Thus, it is more appropriate for people with different levels of cardiovascular fitness to apply HRR estimation (21, 44).
To target individual HR intensity zones, we applied the intensity division from two guidelines (21, 45), and estimated HR for each participant reflecting the distribution from Table 1 (individual HR reflecting the intensity levels from Table 1 can be seen in Supplementary Table S1). We applied the predefined intensity zones and used the HRmax estimation from Fernhall et al. (42) as a frame to categorize HR responses in relation to the intensity of PA.
A convenience subsample of 5 subjects (n = 5) were recruited for the evaluation of device agreement and accuracy. The sample consisted of subject who voluntary agreed, were accessible, and able to wear the HRM-DUAL™. We compared the wrist-worn photoplethysmography-based Vivosmart 4 with a Garmin HRM-DUAL™ chest electrocardiogram-based HR monitor, connected to a Garmin Forerunner 265. Both devices were worn simultaneously during a Team Twin session, the Forerunner 265 was placed on the running chair, not the athlete. Each athlete wore two monitors and acted as their own controls (concurrent validity). A researcher attached the wrist-worn and the chest-worn monitor as instructed (39, 46) and simultaneously activated/deactivated the monitors. During this study, we assessed the two devices using methods accommodating accuracy, agreement, and inter-rater agreement between the HR monitors (as described under 2.7.2. HR agreement—secondary objective).
When using commercial HR monitors, including the one used in this study, continuous measurements (every second or other predefined time-interval) were not a standard output (24). The Vivosmart 4 sampling frequency is HR-determined instead of a pre-defined time interval. Meaning that the time frequency keeps in the same timeslot until the HR exceeds ±3 bpm. Thus, we used an HTML script to transform the HR data into a second-by-second time-interval dataset (see Supplementary File S1). Data was then trimmed with a ±15 s moving average filter, rounded to the nearest whole number, to accommodate interference, potential time displacement (when activating/deactivating the wrist/chest HR monitors), and device-specific measuring errors. This approach was applied to both datasets (wrist and chest). For the wrist data, we also compressed HR data into a one-minute mean and transferred the HR into %HRR (intensity levels) to fit the frame of intensity zones (Table 1). All statistical analyses were conducted with STATA version 17 (StataCorp LP, College Station, TX, USA). We applied a two-sided test with a significance level of 0.05% and 95% confidence intervals (16).
Descriptive statistics were used to determine time-in-zone (as described under 2.5—Heart rate measures). We performed a mixed regression analysis, with subjects as random effects, to investigate HR responses during and between training and races. Mean one-minute %HRR was the dependent variable, while time (in minutes), and race/training, serving as factor variables, were included with an interaction term being the independent variable in the model. It was adjusted for sex, age, and length of the individual training/race session and visually validated for Gaussian distribution, homoscedasticity, and best linear unbiased estimate of participant distribution. No violation of the assumptions was detected.
We applied Lin et al.’s concordance correlation coefficient (Rc) to estimate accuracy and to detect deviance from the ideal trend line (the 45° concordance line) (47–49). Based on previous accuracy studies, we deemed a Rc > 0.80 acceptable to demonstrate accuracy between the wrist and the chest monitors (25, 26). Bland & Altman plots were used to estimate agreement between the two HR monitors within ±1.96 SD (50). Inter-rater agreement between the time spent at different intensity zones (Table 1) was evaluated with Cohen’s kappa coefficient (κ). We applied the coefficient thresholds from Landis et al. for the interpretation of the association, who characterized the values as <0 (no agreement), 0–0.20 (slight), 0.21–0.40 (fair), 0.41–0.60 (moderate), 0.61–0.80 (substantial), and 0.81–1 (almost perfect agreement) (51).
Our sample consisted of seven females and eleven males aged 19–65 (mean 35.4 years). In-depth characteristics are found in Table 2. Cerebral palsy (CP) was the most frequently self-reported diagnose (72%). Other conditions reported were muscular dystrophy, physical and mental disability, inherited neurodegenerative diseases, and multiple sclerosis (28%). Of those with CP, most were diagnosed with quadriplegia (92%), with a Gross Motor Function Classification System (GMFCS) at IV-V (85%). Due to their various conditions, eight handiathletes (44%) used both non- and prescription-only drugs on a daily base. These included, but were not limited to, Sirdalud, Euthyrox, Buscopan, medicinal cannabis etc. Common side-effects of these drugs might influence coronary circulation, resulting in palpitation, frequent pulse, increased RHR and extrasystole. During the 16-week program, the handiathletes had an average of seven sessions (±4) with the Vivosmart 4 activated and a mean HR of 90 (±16.8) bpm (20 ± 13.2%HRR) across race and training. An average session lasted 77 (±24) minutes and covered 10.5 (±4) km. The subsample (n = 5) used for the agreement evaluation consisted solely of males with GMFCS IV-V, had slightly higher mean HR during sessions, and a lower-than-average-sample BMI and weight (Supplementary Table S2).
A total of 130 sessions, 113 training (162 h, 18 m, and 46 s), and 17 races (39 h, 51 m, 47 s), gave a total monitoring time of 202:10:33 (HH:MM:SS) recorded with the Vivosmart 4. Table 3 depicts time spent in each intensity zone (cf. Table 1). Most time (80%) was spent in the very-light intensity zone, which equals sedentary behavior. All subjects sustained an HR response consistent with light and moderate intensity zones; however, the duration in each level varied considerably across the group. Nine handiathletes (50%) reached the vigorous intensity zone at one or more points during the program period, and the duration in it varied between 0.02% and 6.6% of their total recorded time (Supplementary Figure S2).
Table 3. Total time and time proportions spent in each intensity zone during Team Twin activity measured by wrist HR monitor.
We found a decline-in-HR-over-time tendency during both training and races. This tendency is depicted in Figure 1, which presents the %HRR-responses for all handiathletes grouped by race or training participation. Further, the plot illustrates a variance in the %HRR with increasing duration, meaning that dispersion increases with time. The median training duration was 79 min (25 p; 63 min, 75 p; 105 min), and the median race duration was 153 min (25 p; 105 min, 75 p; 175 min) (Figure 1). The greater dispersion after the 79th and 153rd min, respectively, are likely due to significantly fewer observations, as few handiathletes had training/race sessions lasting for more than 105 and 175 min (75% percentile), respectively. During race participation, we found a higher mean %HRR (intensity level), compared to training sessions, 26%HRR (95%CI 20.9; 31.0) vs. 19%HRR (95%CI 14.1; 24.0), respectively. A significantly higher mean of 6.8%HRR (95%CI: 6.1; 7.4, p < 0.000) adjusted for sex, age, and duration of the session, in favor of race participation was found (see graph in Supplementary Figure S3). A sensitivity analysis revealed the same results when only including handiathletes who had data from both race and training sessions (n = 13) (data not shown).
Figure 1. One-minute mean of %HRR-responses (intensity) during training (n = 113) and races (n = 17), adjusted for age, sex, and length of session. The figure illustrates %HRR-responses (intensity) for Race (A) and Training (B), combined for all handiathletes. The y-axis is %HRR and the x-axis is time in minutes. The yellow (top), dark green (middle) and light green (bottom) dashed vertical lines indicates the threshold for very-light (<29% HRR), light (30%-39% of HRR) and, moderate (40%–59% of HRR) intensity levels reflecting Table 1. The red- and blue-dashed vertical lines represents the x-axes 25th, 50th, and 75th percentiles, respectively.
A total of 11:26:25 (HH:MM:SS) (equaling 41185 HR values) were collected for the second-by-second analysis, evaluating the agreement between the wrist and chest monitors. The mean time subjects wore the devices was 01:55:04 (min; 01:02:09, max; 02:37:38) (HH:MM:SS). We conducted six wrist and chest evaluations on five handiathletes. Two out of the six evaluations were conducted during a race.
Across the evaluation of agreement and second-by-second analysis, the wrist monitor showed an acceptable mean accuracy with the chest HR monitor [Rc = 0.86 (CI 95% 0.863; 0.868)] (Figure 2A). The Bland & Altman plot reveals that dispersion occurs during the whole spectrum of HR intensities; however, more excellent dispersion with increasing HR. That means, with an increase in HR, the agreement between the two monitors decreases, resulting in greater heteroscedasticity. The limits of agreement between the wrist and the chest monitor fall within −16 and +13 bpm. Despite an overall acceptable accuracy, the individual variation from Lin’s Rc-coefficient and the agreement from the Bland & Altman plots vary notably (Rc between 0.05–0.91 and Bland & Altman plot between −27 and +18, see Supplementary Figure S4). Cohens kappa’s coefficient reveals a substantial inter-rater agreement between the two HR monitors of the intensity zones (k = 0.75), with an overall agreement of 92%. The chest monitor generally registers more time at higher intensity zones than the wrist monitor (e.g., total time in moderate-intensity zone intercepted for the wrist HR monitor; 0:53:12 (HH:MM:SS), and for the chest HR monitor; 1:01:46 (HH:MM:SS)). The distribution and inter-rater agreement between the Vivosmart 4 and the HRM-DUAL™ are available in Supplementary Table S3.
Figure 2. Lin’s concordance correlation coefficient (A) and Bland & Altman plot (B) for wrist and chest agreement and accuracy analysis. Figure 2A illustrates Lin’s et al. concordance correlation coefficient (Rc) data point arounds the 45° concordance line. Figure 2B represents the Bland & Altman plots, estimating total agreement within ±1.96 SD between the wrist and chest HR monitors (Vivosmart 4 and HRM-DUAL™, respectively).
The primary findings include that PLWD elicit heart rate responses equal to very light intensity zones (sedentary) with intensity fragments in light to moderate zones and sporadically vigorous intensity, with a considerable variation in the duration of higher intensities across the sample when participating in the Team Twin co-running indirect activities. Moreover, evaluating the agreement and accuracy of the Vivosmart 4 we observed that the wrist-worn monitor provided an overall acceptable accuracy, agreement, and substantial inter-rater agreement. compared to the chest worn HRM-DUAL™; however, it was not consistently precise.
The Team Twin pilot study of 2018 (17) indicated that two out of three subjects reached HR levels consistent with the moderate intensity zones, sustained for long periods and sometimes vigorous intensity zone during training sessions and official races (17). It concluded that HR responses were consistent with a cardiorespiratory fitness impact (17) due to arousal and locomotion (isometric muscle stimulation). Our findings, however, contradict the notion, as only a limited amount of the total recorded time reached moderate intensity zones or above. We identified spikes in the HR of selected individuals, sustaining intensity levels above the very-light intensity zone (sedentary), but they were few. Reflecting on the Team Twin co-running activity (movement by sitting in a chair pushed by others), a significant proportion of HR correlating to sedentary behavior was expected. Although no other studies evaluating Team Twin were discovered, prior studies evaluating HR response for individuals with CP and quadriplegia participating in indirect adapted sports exist. A study on power wheelchair sports has shown HR levels of both light (20) and moderate intensity (19). One study indicated that the type of disability (e.g., cerebral palsy (including subtypes (52) and muscular dystrophy) influenced HR responses, where persons with cerebral palsy reached a higher HR response than people with other types of disabilities (19). Same pattern of increased intensity levels (%HRR) during therapeutic riding was observed among CP diagnosed persons and correlated with increased GMFCS-levels (18). Older studies in this field document significantly higher energy expenditure among persons with cerebral palsy when performing the same activities as able-bodied peers (53–56). Thus, the reason for some handiathletes reaching higher intensity levels may be attributed to a disability-specific influence.
It is worth noting, however, that as handiathletes are sedentary in the Team Twin program, the assumption that their HR response will correspond expectedly to that of physical activity, which is defined as “any bodily movement produced by skeletal muscles that requires energy expenditure” (7 p. 15), may not be just uncertain; it might be erroneous to use HR as a proxy for PA in this specific population and in this context.
An argument can, however, be made that the usual disability-dictated locomotive patterns (of CP) may be triggered to a greater extent during Team Twin sessions, as the running chair encounters obstacles, bumps, turns and moves with a higher pace than usual in their everyday life. This could, however speculative, result in hyperreflexia, increased muscle tone, isometric muscle stimulation, and involuntary and uncontrolled movement, as indicated in the pilot study (17) and discussed in the studies of power wheelchair activities (19, 20). The stimulation from the activity could, strictly speaking, affect their bodies and provoke higher energy expenditure, resulting in HR responses, despite participating in otherwise sedentary activity (19, 53). However, further investigation with different methods is needed to determine if a greater rate of energy expenditure is at play. Other explanations of the increased HR responses include disability type (19), medication use, psychological arousal (extra-sensory perception), the activation of the sympathetic nervous system from adrenaline rush due to emotions such as excitement, nervousness, fear, anxiety, fidgeting, or joy (33, 34, 44, 57). All factors are likely to impact HR response, combined with the well-known individual factors affecting cardiorespiratory fitness (age, genetic, sex, social, and psychological factors (21)), contributing to the HR response identified here. This explanation should be seen in the light of the steadily decreasing HR response from start to finish (Figure 1), where feelings like expectation, excitement, and nervousness at the beginning of a co-running session may be more strongly at play than the (physical) activity itself. The pilot study on Team Twin described the exaggerated and increased movement due to the excitement, joy, and happiness inherent to participation in the co-running sessions (17), which may partly explain the increase in HR. Our findings support the notion, as we observed significantly higher HR response during official races than in regular Team Twin training sessions, where the context is different, and stakes may be perceived as higher. During races, handiathletes might experience affection from cheering spectators, an excited atmosphere, and a competitive setting, increasing their excitement and, in turn, movements in the upper body and limbs. Thus, the combination of increased within-chair movement, psychological arousal, disability- and (potential) medication-induced HR responses, race/training context, and individual variation are likely to contribute to HR responses. Considering these factors and the sedentary nature of the Team Twin activity, the definition of PA (7) and the (declining) HR response (Figure 1) observed in this study further complicates the questions of whether this is energy expenditure (PA) or other factors-induced HR responses, more than the Team Twin co-running program activity itself or a combination of multiple factors.
Lin’s Rc coefficient revealed a mean acceptable accuracy between the wrist and the chest HR monitor across all subjects, consistent with similar studies (24, 25, 27). Our study, however, is the first evaluation of this specific wearable (Vivosmart 4) and population (24–30, 35). The limit of agreement falls within −16 and +13 and does not reveal any systematic bias or variability. Sartor et al. state that ±15 bpm is a great limit of agreement considering a second-by-second comparison (27) while the present study shows greater accuracy than other comparison studies (24, 25, 58, 59). HR accuracy studies among the general population imply that an increase in HR is possibly associated with increased upper-body movement (24, 32) and types of exercise involving more movement in the limbs (e.g., use or no use of arms on an elliptical trainer), highly affects accuracy among different wrist HR monitors (25, 26). However, some studies do not find the same inaccuracy-error regarding intensity and type of exercise (28, 29). These inconsistencies are likely due to methodology, protocol, and individual differences. In the present study, we observed a great individual variance in accuracy among the subjects included in the evaluation (see Supplementary Figure S4). This finding could be due to errors caused by movement, and in this case, by disability-induced upper-body (uncontrolled) movement and flexion of the wrist. The difference between the handiathletes with the most and least wrist/chest agreement was seen among handiathlete C and F (see Supplementary Figure S4). Their individual disability-specific conditions and diagnoses differed significantly. One lives with a disability prognosticate great hypokinesia (no muscle activity and thus no movement in limbs), and the other with an appearance similar with CP induced dyskinesia (characterized by rapid involuntary and abnormal movement (52)). Thus, it is likely that the disability-specific conditions affecting locomotion result in major subject-specific-agreement and variation (22), coupled with other factors affecting accuracy, as described by Garmin (e.g., non-proper fit on the wrist, physical characteristics and type of exercise, and intensity of the activity (60)). Although the chest HR monitor seems more sensitive to higher intensity levels compared to the Vivosmart 4, most of the time was captured within the same intensity zones (92% agreement). Further, the Vivosmart 4, used among PLWD, does not reach the same accuracy as other wrist-worn HR devices have demonstrated among the general population (e.g., Apple Watch and Mio Fuse (24–26, 29)). Thus, HR accuracy and agreement seems to be highly affected by individual variation and disability characteristics and can, for some individuals, cause measurement errors.
A main strength of this study is the second-by-second comparison of HR-data (27). Additionally, applying the HRR to estimate intensity levels, rather than a standard formula (e.g., % of HRmax) strengthens the validity of each individual (21, 34). Applying the chest-worn monitor as the reference device seems reliable due to a high overall accuracy between chest monitors and golden standard electrocardiograms for HR monitoring (24–30, 35, 36), especially under the circumstances of “real world settings” where other HR validation studies also apply commercial chest worn HR monitors (61–63). However, a limitation of the study may be that no validation studies have examined the Garmin HRM-DUAL™ among the general population nor among PLWD.
The study also faced other limitations. We only managed to recruit five subjects and conducting a total of six evaluations due to limited resource and time and difficulties of recruiting the handiathletes. Some of the reasons for the sparse subsample (n = 5) was 1) few available days (only Sundays) due to not all planned visits were allocated to conduct the evaluation (some visits where scheduled for interviews and observation), 2) difficulties attaching the strap to all subjects due to their many layers of clothes and disabilities, and 3) some subjects refused to partake while the felt uncomfortable getting undressed to get the strap attached. Thus, our data basis is quite small and expected to be selection bias.
Despite applying the most valid methods available for the HRR estimation, a total of 7 h and 19 min was registered below the estimated RHR (<0%HRR) (data not shown). This is why we see 95% CI below zero in Figure 1. This questions 1) the accuracy of the estimated RHR from the Vivosmart 4, 2) the accuracy of the Vivosmart 4 during Team Twin activities, 3) the methods we applied to easily (and accurately) estimate HR responses among PLWD, and 4) the influence of the potentially falsely increased RHR due to the side-effects of drugs among 44% of the handiathletes (Table 2) and the well-known CP-induced increased RHR (64). During a post hoc estimation, we observed that handiathletes who used drugs with potential side-effects on the coronary circulation and pulse obtained significantly higher HR intensity zones than those who have not been prescribed those drugs (data not shown). This elaborates further the questions of HR as a measurement for PA-induced energy expenditure among PLWD with increased use of medications (with potential effects on HR).
A significant limitation of the study is that HRmax could not be directly measured, as no handiathletes reached peak HR. HRmax estimations are acceptable when no direct HRmax measure is feasible (21). Thus, the equation from Fernhall et al. (42) was used to estimate HRmax in relation to the selected intensity thresholds (21, 45). Using more wide-spread equations for HRmax estimation (e.g., Gellish et al. (65) or Fox et al. (66)), the proportion of time spent in higher intensity zones decreased (see Supplementary Table S4). However, apart from the very hard intensity zone, all intensity levels were generally alike, regardless of the method used to estimate HRmax. In general, quantifying intensity zones and interpreting HR response as an assumed function of PA among this population, with the accessible tools, applied methods, and taken approaches, are highly challenging, however, we believe we have optimized the use of the chosen techniques and methods. Ultimately, the level of accuracy will depend on the specific use and activity performed by the user.
Subjects faced significant challenges in operating the Vivosmart 4 due to the incompatibility between its design (tiny and touch screen (39)) and disabilities impairing both fine and gross motor function. This meant that turning on/off the device during sessions relied on runners’ and relatives’ help. Still, remembering to activate the watch was challenging, and much data was not monitored compared to the actual participation rate (Table 2). A larger device with buttons instead of a touchscreen would likely have better accommodated the participants’ motor function. However, a wrist-worn wearable seems more suitable, compatible, and accessible for this population than a chest-worn monitor due to the challenge of attaching it on severely PLWD.
Some handiathletes did not wear the watch precisely as described in the manual (39). During observations at Team Twin training sessions, we noted an improper fit of the watch (too loose/tight and wrong position on the wrist (39)). Sometimes, the PPG lens was dirty—which could impact its accuracy. The placement on either the left or right wrist could also affect the accuracy, although prior studies on the general population did not find accuracy variation between the left and right wrist (25). However, Garmin highlights that “wrist flexing” can prohibit accuracy (60), which inhibits the agreement and accuracy Thus, in a population with hyperreflexia due to CP attaching the HR monitor to the less affected side should be considered.
Garmin also highlights some essential factors to accommodate optimal conditions for the chest-monitor-accuracy (e.g., being wet to maintain electrical connection, correct positioning, warming up, not wearing synthetic fabrics due to the development of static electricity etc (46)). Some of those issues were difficult to accommodate among the PLWD, e.g., optimal chest positioning, due to abnormal posture and contact between monitor strap and running chair-back changing its fit over a session.
The findings from this study are limited to the specific population and activity form of recreational indirect PA regarding the external validity. However, our reflections based on the use of HR-monitors among PLWD, and the methodological challenges can be generalized to other groups of PLWD, with specific conditions that could affect the validity of a HR monitor. Despite that, generalize conditions across various disabilities in relation to this specific topic may be challenging, due to all the different factors affecting HR monitoring. Thus, applying and selecting HR monitors (either wrist- or chest-worn) among population with specific conditions should be considered according to the issues addressed in the study.
People with severe functional limitations participating in the Team Twin co-running program demonstrate HR responses consistent with time spend mostly in very-light intensity. The Vivosmart 4 reached acceptable accuracy, but low precision as the estimate was highly variable. Our study further implicates major methodological challenges and suitability when using HR as a proxy for PA and HR monitors among severely disabled persons participating in indirect adapted sports activities. Future studies, practitioners, or health professional applying HR monitors among individuals living with severely disabilities, should consider how individual conditions may challenge the accuracy of the HR monitors and thus, the selection including attachment of the devices.
The original contributions presented in the study are included in the article/Supplementary Material, further inquiries can be directed to the corresponding author.
The studies involving humans were approved by The Ethics Committee of Denmark’s Capital Region. The studies were conducted in accordance with the local legislation and institutional requirements. Written informed consent for participation in this study was provided by the participants’ legal guardians/next of kin. Written informed consent was obtained from the individual(s) for the publication of any potentially identifiable images or data included in this article.
AJ: Conceptualization, Methodology, Software, Formal analysis, Investigation, Data curation, Writing – original draft preparation. MT: Conceptualization, Methodology, Validation, Writing – review and editing, Supervision, Project administration, Funding acquisition. ME: Investigation, Data curation, Software, Writing – review and editing. MRL: Conceptualization, Methodology, Funding acquisition, Supervision, Writing – review and editing. CBP: Conceptualization, Methodology, Validation, Writing – review and editing, Supervision, Project administration. All authors contributed to the article and approved the submitted version.
The author(s) declare financial support was received for the research, authorship, and/or publication of this article.
This research was funded equally by Helsefonden and Bevica Fonden.
The research team would like to provide our greatest acknowledgments to the subjects enrolled in the study and to the Team Twin foundation. Also, a special thanks to the co-runners from the respective Team Twin clubs involved in the study. Your engagement and help during data collection regarding the wearables made the study possible and feasible. A special thanks to Mark P.P. Lyngbaek3, MD. PhD student, for examining and mapping the medicaments, with potential side-effects on the circulatory system, used among the participants.
The authors declare that the research was conducted in the absence of any commercial or financial relationships that could be construed as a potential conflict of interest.
All claims expressed in this article are solely those of the authors and do not necessarily represent those of their affiliated organizations, or those of the publisher, the editors and the reviewers. Any product that may be evaluated in this article, or claim that may be made by its manufacturer, is not guaranteed or endorsed by the publisher.
The Supplementary Material for this article can be found online at: https://www.frontiersin.org/articles/10.3389/fspor.2023.1213655/full#supplementary-material
Supplementary File S1
HR transformation example.
Supplementary Figure S1
Flowchart.
Supplementary Figure S2
Time spend in each intensity level per participant.
Supplementary Figure S3
Training vs. Race bar graph.
Supplementary Figure S4
Individual Bland & Altman plots.
Supplementary Table S1
Intensity levels estimated for each participant.
Supplementary Table S2
Subsample and remaining sample characteristics.
Supplementary Table S3
Cross tabulation Cohen’s kappa coefficient.
Supplementary Table S4
HRmax estimations influence on interpretation.
1. WHO. World report on disability 2011. 20 Avenue Appia, 1211 Geneva 27, Switzerland: World Health Organization (2011). Report No.: 9241564180.
2. Ryan JM, Allen E, Gormley J, Hurvitz EA, Peterson MD. The risk, burden, and management of non-communicable diseases in cerebral palsy: a scoping review. Dev Med Child Neurol. (2018) 60(8):753–64. doi: 10.1111/dmcn.13737
3. Carty C, van der Ploeg HP, Biddle SJH, Bull F, Willumsen J, Lee L, et al. The first global physical activity and sedentary behavior guidelines for people living with disability. J Phys Act Health. (2021) 18(1):86–93. doi: 10.1123/jpah.2020-0629
4. Hombergen SP, Huisstede BM, Streur MF, Stam HJ, Slaman J, Bussmann JB, et al. Impact of cerebral palsy on health-related physical fitness in adults: systematic review. Arch Phys Med Rehabil. (2012) 93(5):871–81. doi: 10.1016/j.apmr.2011.11.032
5. Mcphee PG, Claridge EA, Noorduyn SG. Gorter JW. Cardiovascular disease and related risk factors in adults with cerebral palsy: a systematic review. Dev Med Child Neurol. (2019) 61(8):915–23. doi: 10.1111/dmcn.14028
6. Benner JL, Hilberink SR, Veenis T, Stam HJ, Van Der Slot WM, Roebroeck ME. Long-term deterioration of perceived health and functioning in adults with cerebral palsy. Arch Phys Med Rehabil Psychol. (2017) 98(11):2196–205. doi: 10.1016/j.apmr.2017.03.013
7. World Health Organization. WHO Guidelines on physical activity and sedentary behaviour. Geneva: World Health Organization (2020). Report No.: 9240014888.
8. Martin Ginis KA, van der Ploeg HP, Foster C, Lai B, McBride CB, Ng K, et al. Participation of people living with disabilities in physical activity: a global perspective. Lancet. (2021) 398:443–55. doi: 10.1016/S0140-6736(21)01164-8
9. Rimmer JH, Riley B, Wang E, Rauworth A. Accessibility of health clubs for people with mobility disabilities and visual impairments. Am J Public Health. (2005) 95(11):2022–8. doi: 10.2105/AJPH.2004.051870
10. Diaz R, Miller EK, Kraus E, Fredericson M. Impact of adaptive sports participation on quality of life. Sports Med Arthrosc. (2019) 27(2):73–82. doi: 10.1097/JSA.0000000000000242
11. Hammel J, Magasi S, Heinemann A, Gray DB, Stark S, Kisala P, et al. Environmental barriers and supports to everyday participation: a qualitative insider perspective from people with disabilities. Arch Phys Med Rehabil. (2015) 96(4):578–88. doi: 10.1016/j.apmr.2014.12.008
12. Rimmer JH, Riley B, Wang E, Rauworth A, Jurkowski J. Physical activity participation among persons with disabilities: barriers and facilitators. Am J Prev Med. (2004) 26(5):419–25. doi: 10.1016/j.amepre.2004.02.002
13. Ives B, Clayton B, Brittain I, Mackintosh C. ‘I’ll always find a perfectly justified reason for not doing it’: challenges for disability sport and physical activity in the United Kingdom. Sport in Society. (2021) 24(4):588–606. doi: 10.1080/17430437.2019.1703683
14. Martin JJ. Benefits and barriers to physical activity for individuals with disabilities: a social-relational model of disability perspective. Disabil Rehabil. (2013) 35(24):2030–7. doi: 10.3109/09638288.2013.802377
15. Duvall J, Satpute S, Cooper R, Cooper RA. A review of adaptive sport opportunities for power wheelchair users. Disabil Rehabil Assist Technol. (2021) 16(4):407–13. doi: 10.1080/17483107.2020.1767220
16. Jørgensen A, Petersen CB, Eghøj M, Toftager M. When movement moves: study protocol for a multi-method pre/post evaluation study of two programmes; the danish team twin and cycling without age. Int J Environ Res Public Health. (2021) 18(19):10008. doi: 10.3390/ijerph181910008
17. Winther H, Kissow A-M, Pedersen MT, Sandahl C. Forundersøgelse om team tvilling: Når bevægelse bevæger: om betydningen af positive kropsoplevelser, ligeværdige relationer og identitetsudviklende fællesskaber for mennesker med store bevægelsesbegrænsninger. English; pilot study on team twin: when movement moves: the importance of positive body experiences, equal relationships and identity-developing communities for people with severe movement limitations. København: University of Copenhagen (2018).
18. Dirienzo LN, Dirienzo LT, Baceski DA. Heart rate response to therapeutic riding in children with cerebral palsy: an exploratory study. Pediatr Phys Ther. (2007) 19(2):160–5. doi: 10.1097/PEP.0b013e31804a57a8
19. Barfield JP, Malone LA, Collins JM, Ruble SB. Disability type influences heart rate response during power wheelchair sport. Med Sci Sports Exerc. (2005) 37(5):718–23. doi: 10.1249/01.MSS.0000161807.77552.8B
20. Barfield JP, Newsome L, Malone LA. Exercise intensity during power wheelchair soccer. Arch Phys Med Rehabil. (2016) 97(11):1938–44. doi: 10.1016/j.apmr.2016.05.012
21. Riebe D, Ehrman JK, Liguori G, Magal M. ACSM’s guidelines for exercise testing and prescription. 10th edition. Medicine ACoS. Philadelphia: Wolters Kluwer (2018).
22. Rum L, Sten O, Vendrame E, Belluscio V, Camomilla V, Vannozzi G, et al. Wearable sensors in sports for persons with disability: a systematic review. Sensors (Basel, Switzerland). (2021) 21(5):1858. doi: 10.3390/s21051858
23. Thompson WR. Worldwide survey of fitness trends for 2022. ACSM’s Health Fit J. (2022) 26(1):11–20. doi: 10.1249/FIT.0000000000000732
24. Wang R, Blackburn G, Desai M, Phelan D, Gillinov L, Houghtaling P, et al. Accuracy of wrist-worn heart rate monitors. JAMA Cardiology. (2017) 2(1):104–6. doi: 10.1001/jamacardio.2016.3340
25. Gillinov S, Etiwy M, Wang R, Blackburn G, Phelan D, Gillinov AM, et al. Variable accuracy of wearable heart rate monitors during aerobic exercise. Med Sci Sports Exerc. (2017) 49(8):1697–703. doi: 10.1249/MSS.0000000000001284
26. Pasadyn SR, Soudan M, Gillinov M, Houghtaling P, Phelan D, Gillinov N, et al. Accuracy of commercially available heart rate monitors in athletes: a prospective study. Cardiovasc Diagn Ther. (2019) 9(4):379–85. doi: 10.21037/cdt.2019.06.05
27. Sartor F, Gelissen J, Van Dinther R, Roovers D, Papini GB, Coppola G. Wrist-worn optical and chest strap heart rate comparison in a heterogeneous sample of healthy individuals and in coronary artery disease patients. BMC Sports Sci Med Rehabil Vol. (2018) 10(1):1–10. doi: 10.1186/s13102-018-0090-8
28. Stahl SE, An H-S, Dinkel DM, Noble JM, Lee J-M. How accurate are the wrist-based heart rate monitors during walking and running activities? Are they accurate enough? BMJ Open Sport Exerc Med. (2016) 2(1):e000106. doi: 10.1136/bmjsem-2015-000106
29. Hough P, Glaister M, Pledger A. The accuracy of wrist-worn heart rate monitors across a range of exercise intensities. J Phys Activ Res. (2017) 2(2):112–6. doi: 10.12691/jpar-2-2-8
30. Laukkanen RM, Virtanen PK. Heart rate monitors: state of the art. J Sports Sci. (1998) 16(Suppl:S3-7):3–7. doi: 10.1080/026404198366920
31. Díaz S, Stephenson JB, Labrador MA. Use of wearable sensor technology in gait, balance, and range of motion analysis. Appl Sci. (2020) 10(1):234. doi: 10.3390/app10010234
32. Lee CM, Gorelick M. Validity of the smarthealth watch to measure heart rate during rest and exercise. Meas Phys Educ Exerc Sci. (2011) 15(1):18–25. doi: 10.1080/1091367X.2011.539089
33. Montoye HJ. Introduction: evaluation of some measurements of physical activity and energy expenditure. Med Sci Sports Exerc. (2000) 32(9):S439–S41. doi: 10.1097/00005768-200009001-00001
34. Strath SJ, Swartz AM, Bassett DR, O’brien WL, King GA, Ainsworth BE. Evaluation of heart rate as a method for assessing moderate intensity physical activity. Med Sci Sports Exerc. (2000) 32(9):S465–S70. doi: 10.1097/00005768-200009001-00005
35. Fuller D, Colwell E, Low J, Orychock K, Tobin MA, Simango B, et al. Reliability and validity of commercially available wearable devices for measuring steps, energy expenditure, and heart rate: systematic review. JMIR Mhealth Uhealth. (2020) 8(9):e18694. doi: 10.2196/18694
36. Sartor F, Papini G, Cox LGE, Cleland J. Methodological shortcomings of wrist-worn heart rate monitors validations. J Med Internet Res. (2018) 20(7):e10108. doi: 10.2196/10108
37. World_Medical_Association. Declaration of Helsinki. Ethical principles for medical research involving human subjects WMA general assembly. Seoul, Korea: The World Medical Association. (2008). Available at: https://www.wma.net/wp-content/uploads/2018/07/DoH-Oct2008.pdf.
38. Team Twin—We Run Together. Team twin foundation. (2020). [Cited 2022 13th September]. Available at: https://teamtvilling.dk/.
39. Garmin. Vívosmart® 4 owner’s manual. (2021). [updated March; Cited 2022 November]. Available at: https://www8.garmin.com/manuals/webhelp/vivosmart4/EN-US/vivosmart_4_OM_EN-US.pdf
40. Garmin. Garmin support center. (2022). [Cited 2022 November]. Available at: https://support.garmin.com/en-US/?faq=F8YKCB4CJd5PG0DR9ICV3A.
41. Mouritzen NJ, Larsen LH, Lauritzen MH, Kjær TW. Assessing the performance of a commercial multisensory sleep tracker. PloS One. (2020) 15(12):e0243214. doi: 10.1371/journal.pone.0243214
42. Fernhall B, Mccubbin JA, Pitetti KH, Rintala P, Rimmer JH, Millar AL, et al. Prediction of maximal heart rate in individuals with mental retardation. Med Sci Sports Exerc. (2001) 33(10):1655–60. doi: 10.1097/00005768-200110000-00007
43. Karvonen MJ. The effects of training on heart rate: a longitudinal study. Ann Med Exp Biol Fenn. (1957) 35:307–15.13470504
44. Garber CE, Blissmer B, Deschenes MR, Franklin BA, Lamonte MJ, Lee I-M, et al. American college of sports medicine position stand. Quantity and quality of exercise for developing and maintaining cardiorespiratory, musculoskeletal, and neuromotor fitness in apparently healthy adults: guidance for prescribing exercise. (2011) 43(7):1334–59. doi: 10.1249/MSS.0b013e318213fefb
45. Strath SJ, Kaminsky LA, Ainsworth BE, Ekelund U, Freedson PS, Gary RA, et al. Guide to the assessment of physical activity: clinical and research applications. Circulation. (2013) 128(20):2259–79. doi: 10.1161/01.cir.0000435708.67487.da
46. Garmin. Putting on the heart rate monitor Garmin. (2022). [Cited 2022 November]. Available at: https://www8.garmin.com/manuals/webhelp/hrm-dual/EN-US/GUID-D766457C-6F30-4004-9386-1681CB2C74C6.html
47. Lawrence IKL. A concordance correlation coefficient to evaluate reproducibility. Biometrics. (1989) 45(1):255–68. doi: 10.2307/2532051
48. Lawrence IKL. A note on the concordance correlation coefficient. JMIR Cardio. (2000) 56:325. doi: 10.1177/1536867X0200200206
49. Steichen TJ, Cox N. A note on the concordance correlation coefficient. Stata J. (2002) 2(2):183–9. doi: 10.1177/1536867X0200200206
50. Bland J M, Altman D G. Statistical methods for assessing agreement between two methods of clinical measurement. Lancet. (1986) 327(8476):307–10. doi: 10.1016/S0140-6736(86)90837-8
51. Landis JR, Koch GG. The measurement of observer agreement for categorical data. Biometrics. (1977) 33(1):159–74. doi: 10.2307/2529310
53. Rose J, Gamble JG, Medeiros J, Burgos A, Haskell WL. Energy cost of walking in normal children and in those with cerebral palsy: comparison of heart rate and oxygen uptake. J Pediatr Orthop. (1989) 9(3):276–9. doi: 10.1097/01241398-198905000-00004
54. Tobimatsu Y, Nakamura R, Kusano S, Iwasaki Y. Cardiorespiratory endurance in people with cerebral palsy measured using an arm ergometer. Arch Phys Med Rehabil. (1998) 79(8):991–3. doi: 10.1016/S0003-9993(98)90099-5
55. Unnithan VB, Dowling JJ, Frost G, Bar-Or O. Role of cocontraction in the O2 cost of walking in children with cerebral palsy. Med Sci Sports Exerc. (1996) 28(12):1498–504. doi: 10.1097/00005768-199612000-00009
56. Unnithan VB, Dowling JJ, Frost G, Bar-Or O. Role of mechanical power estimates in the O2 cost of walking in children with cerebral palsy. Med Sci Sports Exerc. (1999) 31(12):1703. doi: 10.1097/00005768-199912000-00003
57. Schwartz GE, Weinberger DA, Singer JA. Cardiovascular differentiation of happiness, sadness, anger, and fear following imagery and exercise. Psychosom Med. (1981) 43(4):343–64. doi: 10.1097/00006842-198108000-00007
58. Wallen MP, Gomersall SR, Keating SE, Wisløff U, Coombes JS. Accuracy of heart rate watches: implications for weight management. PLoS One. (2016) 11(5):e0154420. doi: 10.1371/journal.pone.0154420
59. Chow H-W, Yang C-C. Accuracy of optical heart rate sensing technology in wearable fitness trackers for young and older adults: validation and comparison study. JMIR Mhealth Uhealth. (2020) 8(4):e14707. doi: 10.2196/14707
60. Garmin. The heart rate sensor on my garmin watch is not accurate Garmin. (2022). [Cited 2022 November]. Available at: https://support.garmin.com/en-US/?faq=xQwjQjzUew4BF1GYcusE59
61. Rozanski GM, Aqui A, Sivakumaran S, Mansfield AJJc. Consumer wearable devices for activity monitoring among individuals after a stroke: a prospective comparison. JMIR Cardio. (2018) 2(1):e8199. doi: 10.2196/cardio.8199
62. Brazendale K, Decker L, Hunt ET, Perry MW, Brazendale AB, Weaver RG, et al. Validity and wearability of consumer-based fitness trackers in free-living children. Int J Exerc Sci. (2019) 12(5):471.30899354
63. Gorny AW, Liew SJ, Tan CS, Müller-Riemenschneider FJJm, uHealth. Fitbit Charge HR Wireless Heart Rate Monitor: Validation Study Conducted Under Free-Living Conditions. (2017) 5(10):e8233. doi: 10.2196/mhealth.8233
64. Gąsior JS, Zamunér AR, Silva LEV, Williams CA, Baranowski R, Sacha J, et al. Heart rate variability in children and adolescents with cerebral palsy-a systematic literature review. J Clin Med. (2020) 9(4):1141. doi: 10.3390/jcm9041141
65. Gellish RL, Goslin BR, Olson RE, Mcdonald A, Russi GD, Moudgil VK. Longitudinal modeling of the relationship between age and maximal heart rate. Medicine Science in Sports and Exercise. (2007) 39(5):822–9. doi: 10.1097/mss.0b013e31803349c6
Keywords: adapted physical activity (APA), wearable technology, Team Twin co-running, heart rate (BPM), people living with disabilities, sensor accuracy, heart rate reserve
Citation: Jørgensen A, Toftager M, Eghøj M, Ried-Larsen M and Bjørk Petersen C (2023) Heart rate responses, agreement and accuracy among persons with severe disabilities participating in the indirect movement program: Team Twin—an observational study. Front. Sports Act. Living 5:1213655. doi: 10.3389/fspor.2023.1213655
Received: 28 April 2023; Accepted: 22 September 2023;
Published: 24 October 2023.
Edited by:
Eling D. de Bruin, ETH Zürich, SwitzerlandReviewed by:
François Trudeau, Université du Québec à Trois-Rivières, Canada© 2023 Jørgensen, Toftager, Eghøj, Ried-Larsen and Bjørk Petersen. This is an open-access article distributed under the terms of the Creative Commons Attribution License (CC BY). The use, distribution or reproduction in other forums is permitted, provided the original author(s) and the copyright owner(s) are credited and that the original publication in this journal is cited, in accordance with accepted academic practice. No use, distribution or reproduction is permitted which does not comply with these terms.
*Correspondence: Andreas Jørgensen YWpvckBzZHUuZGs=
Abbreviations HR, heart rate; HH:MM:SS, hours; minutes; seconds; Rc, lin’s concordance correlation coefficient; κ, cohen’s kappa coefficient; PLWD, people living with disabilities; PA, physical activity; bpm, beats per minute; RHR, resting heart rate; HRmax, heart rate max; HRR, heart rate reserve; %HRR, percentage of heart rate reserve; CP, cerebral palsy; GMFCS, gross motor function classification system.
Disclaimer: All claims expressed in this article are solely those of the authors and do not necessarily represent those of their affiliated organizations, or those of the publisher, the editors and the reviewers. Any product that may be evaluated in this article or claim that may be made by its manufacturer is not guaranteed or endorsed by the publisher.
Research integrity at Frontiers
Learn more about the work of our research integrity team to safeguard the quality of each article we publish.