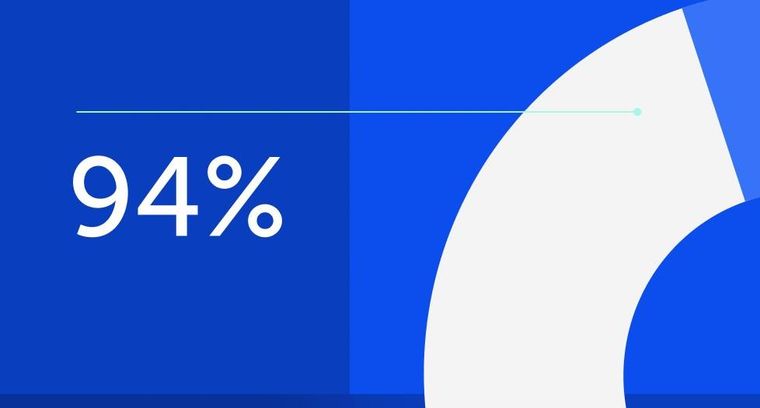
94% of researchers rate our articles as excellent or good
Learn more about the work of our research integrity team to safeguard the quality of each article we publish.
Find out more
ORIGINAL RESEARCH article
Front. Sports Act. Living, 30 January 2023
Sec. Injury Prevention and Rehabilitation
Volume 5 - 2023 | https://doi.org/10.3389/fspor.2023.1090119
This article is part of the Research TopicNeuromuscular Diagnostics and Sensorimotor Performance in Training and Therapy - Beyond the Pure Biomechanical ApproachView all 10 articles
A good body-balance helps to prevent slips, trips and falls. New body-balance interventions must be explored, because effective methods to implement daily training are sparse. The purpose of the current study was to investigate acute effects of side-alternating whole-body vibration (SS-WBV) training on musculoskeletal well-being, flexibility, body balance, and cognition. In this randomized controlled trial, participants were randomly allocated into a verum (8.5 Hz, SS-WBV, N = 28) or sham (6 Hz, SS-WBV, N = 27) condition. The training consisted of three SS-WBV series that lasted one-minute each with two one-minute breaks in between. During the SS-WBV series, participants stood in the middle of the platform with slightly bent knees. During the breaks in between, participants could loosen up. Flexibility (modified fingertip-to-floor method), balance (modified Star Excursion Balance Test), and cognitive interference (Stroop Color Word Test) were tested before and after the exercise. Also, musculoskeletal well-being, muscle relaxation, sense of flexibility, sense of balance, and surefootedness were assessed in a questionnaire before and after the exercise. Musculoskeletal well-being was significantly increased only after verum. Also, muscle relaxation was significantly higher only after verum. The Flexibility-Test showed significant improvement after both conditions. Accordingly, sense of flexibility was significantly increased after both conditions. The Balance-Test showed significant improvement after verum, and after sham. Accordingly, increased sense of balance was significant after both conditions. However, surefootedness was significantly higher only after verum. The Stroop-Test showed significant improvement only after verum. The current study shows that one SS-WBV training session increases musculoskeletal well-being, flexibility, body balance and cognition. The abundance of improvements on a light and portable platform has great influence on the practicability of training in daily life, aiming to prevent slip trips and falls at work.
Slips, trips and falls (STF) are the most frequent accidents in Switzerland, causing 27.7% of all accidents, i.e., about 70,000 workers, in 2020 (1). Also, STF are the most expensive accidents and yielded 41% of all accident expenditure for the years 2014 to 2018 combined. In order to be able to reduce STF incidents, risk factors must be identified (2).
One important risk factor of falls is a weak balance (3), which is related to muscle weakness (4). However, to predict fallers is difficult, because there are several risk factors, which include motor, sensory, and cognitive processes (3). Due to loss of balance being a possible influence of individual frailties on STF (5), balance trainings are recommended to reduce STF (6, 7). Most tested balance trainings often are time-consuming, need much advise, put other regulatory efforts like change of clothes or place which increase regulatory demands at work and therefore low compliance and drop out often occurs (8, 9). The training goal with respect to prevention is that the training is short and easy to administer but also easy to adjust to individual condition and there is no need for change of clothes, shoes, or location (10). In addition, there should be a benefit from each single training session that is noticeable. Thereby, it would be an advantage, when the benefit of a single training is not only improved body balance but includes other improvements like improved mental functions as well. Multiple benefits make it more likely that the training is accepted and becomes a routine behaviour. Also, to increase long-term adherence, it is important to build a routine around a person's lifestyle (11).
In the current study, whole-body vibration (WBV) training is introduced as an exercise-based health-intervention to improve balance with the aim to reduce STF not only in terms of improving motor and sensory, but also cognitive processes.
Long lasting vibrations are biomechanical risk factors that contribute to the development of musculoskeletal pain (12). Other mentioned biomechanical risk factors are heavy load lifting, bending and twisting and remaining in a static position over longer time (13). Vibration exposure from driving vehicles or from vibrating, hammering or rotating work equipment may lead to musculoskeletal and neurological disorders, depending on strength, frequency, duration of action, working method and body posture (14, 15). For example, vibration experienced by construction workers handling compressed air hammers or truck drivers during long-term journeys can cause vascular, neurological and musculoskeletal problems, as well as disturbances of the lumbar spine and the associated nervous system (16).
However, a large number of studies have shown that shorter exposure on vibration can also have a prophylactic effect on musculoskeletal discomfort when range of vibration frequency, amplitude and duration are properly dosed. Thus, in addition to a reduction of musculoskeletal disorders, WBV training can also promote improvements in sensorimotor and muscular performance, balance, functional mobility, bone mineral density, maximum and rapid force, stretch reflexes, and speed of movement (17–25).
WBV exercises are easily applied (10). As the exercise is not exhausting, users usually do not sweat during training sessions. WBV is easily adapted to individual level of body balance and fitness. Hence, users do not have to change clothes or shoes, or take a shower afterwards, which could be important in occupational settings or in healthy young adults, where users do not want to waste time on an intense worksite activity training. High training durations often result in a lack of participation and compliance rate (8, 9). According to worksite training studies, the duration of one WBV training session is about 10 min (26, 27), which is half the time participants usually have to invest in worksite activity trainings (28).
After brief instructions concerning the correct body posture and the handling of the vibration platform, participants can start WBV exercises, which have proven to gain high compliance rates (26, 29). A three-month WBV-intervention with employees suffering from chronic low-back pain, revealed a compliance rate of 81.1%, with two to three recommended trainings per week (29). A four-week WBV-intervention study with office-workers of a Swiss federal department even revealed a training attendance of 129%, therefore more than the instructed three trainings per week (26).
In their systematic review, Oliveira and colleagues (30) found adverse events in only 55 of 1,833 volunteers, who mentioned experiencing for example back pain, pain in their legs, or dizzy sensations. With only 3% adverse events, WBV training is therefore considered to be relatively safe (30). Rogan and colleagues (31) have differed three types of WBV. Sinusoidal vertical WBV (SV-WBV) and sinusoidal side-alternating WBV (SS-WBV), which use a single vibrating platform, and stochastic resonance WBV (SR-WBV), which functions with two independent powered platforms, which can be comparable to skis.
Vibration frequencies among sinusoidal WBV are constant, whereas SR-WBV works with unpredictable random frequencies forcing the human body to constantly adapt its neural and muscular reactions (32). To the best of our knowledge, SR-WBV was originally developed to increase performance of professional ski athletes. Nowadays it is used in different sports as injury prevention, but also in therapies with Parkinson patients (33), stroke patients (34), frail elderly (32), or patients with chronic low back pain (35). However, devices working with SR-WBV are big, heavy and expensive and thus rather used in physiotherapy or in fitness centers than at home.
In the current study, SS-WBV is applied by using a light and portable platform, which seems to be ideal as a training device whether working from home or onsite. Although, SS-WBV seems to have a higher effect than other forms of WBV on bone mineral density (30), in terms of load, Rohlmann and colleagues (36) showed that the maximum load on the vertebral body was lower in SS-WBV (15%) than in SV-WBV (27%). This might be due to the fact, that in comparison to SV-WBV where both legs move up and down at the same time, in SS-WBV, the oscillations take place around a pivot at the center of the platform. Due to this, users have to alternate vibrations between both sides, i.e., when the right foot moves up, the left foot moves down, and vice versa (30, 37). The different types of vibrating platforms are illustrated in Figure 1.
Figure 1. Different types of vibrating platforms. Whole-body vibration (WBV) types from left to right: sinusoidal vertical (SV-WBV), sinusoidal side-alternating (SS-WBV), and stochastic resonance (SR-WBV); source: mediplate.ch; SR-WBV customized by the author.
WBV training has proven to be a safe and useful way to improve performance in athletes among different sports (38). However, as elite athletes already have a high level of performance, WBV training often leads to bivalent results, because it might be too unspecific to improve sport-specific strength, flexibility or balance (39, 40). Not only have recent studies revealed that WBV training is especially beneficial to improve stability or functional mobility in the elderly population (41), in patients with low-back pain (42) or in stroke patients (43), promising effects, such as increased balance and musculoskeletal well-being were also found at the workplace, e.g., in office workers who spend much time in sitting position (26). Recently, even individuals affected with COVID-19 who performed WBV training have exhibited improvement in inflammatory status and an overall improvement in quality of life. Moreover, a reduction of time in intensive care units in severely affected patients was also identified (44).
The current study aims to add knowledge on SS-WBV training effects by use of an experimental design. Experimental evidence for SS-WBV training effects is an important first step before an examination of this portable platform as training device for work from home and onsite occurs in future studies. Therefore, the focus of the current study is on acute musculoskeletal and cognitive effects of SS-WBV in laboratory, unprecedentedly using a light and portable platform with young and healthy participants. Although SS-WBV effects would be expected to be stronger in an older and unhealthy sample, effects are also expected to be meaningful in young and healthy individuals.
Another goal of the current study is to be able to observe side-effects. Health risks increase simultaneously as vibration intensity and exposure increase. However, according to Seidel et al. (1986), vibrations under 20 Hz are safe (45). In the current study, frequencies under 10 Hz are applied, thus side effects are not expected. If no side-effects are observed, future studies might include older people or frail individuals as well.
Body balance depends on musculoskeletal and cognitive function. Together with attention, cognitive flexibility and decision making, inhibitory control belongs to the executive functions (46). Executive functions are located in the prefrontal cortex and are responsible for higher order cognitive abilities, e.g., volitational control over goal-directed behavior (47–50). Thus, goal-directed behavior may be volitionally achieved by deliberately suppressing dominant, automatic responses or impulsive reactions (49, 51). For example, when we walk in the park our automatic tendency would be to place one foot in front of the other. However, when suddenly facing a slippery or unstable surface, inhibitory control helps us to stop this automatic behavioral tendency, which must quickly be modified (52).
Two subcomponents of inhibitory control are motor response inhibition, i.e., the process of revoking an impulsive reaction, and cognitive interference inhibition, i.e., the ability to withstand stimuli related interference of the external environment (53, 54). The latter is subject of the Stroop Test and is subsequently referred to as “cognitive interference”.
Due to the incongruent occurrence of two stimuli (color and description) in the validated Stroop Test (55), the examinee perceives the occurrence of the stimuli as unwanted, sometimes even disturbing, which are two of the defining characteristics of cognitive interference (56). To enable the required performance, participants must ignore the written name of the color. This allows them to name the ink color of the word, which is a goal-directed behavior that requires a little more processing time. Hence, a resulting correlation with poorer performance seems obvious (56).
According to Bolton and Richardson (2022), inhibitory control has proven to be a significant and unique factor in fall prevention (52). Motor training combined with cognitive interference tasks plays an important role, especially for people with Parkinson's disease (46) or older people who participate in fall prevention training (57). These results prompted us to go a step further. Fall-safe older people are more active and safer than their peers, which in turn can lead to a change in physical well-being and not only includes physical activity and balance, but flexibility as well (58). As recently demonstrated, vibration can improve balance in older people (59), as well as in individuals with metabolic syndrome (60) preventing falls and injuries. Thus, it could be that vibration contributes to improved surefootedness. Faes et al. (2018) were able to show that WBV improved surefootedness - and that WBV has a positive effect on balance in addition to surefootedness in healthy individuals (26).
Regular training is especially important with regard to balance, which is an important part of physical well-being, daily mobility and therefore general ability to function in everyday life (61). This is consistent with improved neuromuscular control leading to a better postural stability achieved through WBV training (62). Furthermore, good postural stability has a positive influence on balance (58).
Specifically with regard to the young and healthy participants in the current study, McClain and Shallen (2015) demonstrated that WBV can improve participants fitness, thus also balance, better than static training. In another study conducted by Despina et al. (2020), WBV training resulted in superior short-term performance improvements in flexibility, strength and balance compared to an equivalent exercise without vibration (63) and thus confirmed similar results from Ritzman et al. (2014) (64). Exercise and training programs that include WBV can therefore provide additional benefits for young and well-trained adults.
The current study aims to find new insights in WBV and their effect on musculoskeletal well-being, muscle relaxation, sense of flexibility, sense of balance, and surefootedness. Specifically, based on previous research as stated above, musculoskeletal well-being, flexibility, and balance should be increased and cognitive interference decreased after one training session of SS-WBV. These results are hypothesized to be found only in the experimental group (8.5 Hz) and not in the control group (6 Hz):
Musculoskeletal well-being and muscle relaxation assessed with questionnaire is expected to be increased after one training session of SS-WBV with a vibration frequency of 8.5 Hz but not of 6 Hz (H1). Also, flexibility assessed through the modified fingertip-to-floor method (mFTF) is expected to be increased after one training session of SS-WBV with a vibration frequency of 8.5 Hz but not of 6 Hz (H2). Furthermore, balance measured with the modified Star Excursion Balance Test (mSEBT) is expected to be increased after one training session of SS-WBV with a vibration frequency of 8.5 Hz but not of 6 Hz (H3). Lastly, cognitive interference measured with the Stroop Color Word Test is expected to be decreased after one training session of SS-WBV with a vibration frequency of 8.5 Hz but not of 6 Hz (H4).
The study was performed in consensus with all requirements defined by the Swiss Society of Psychology and was conducted with the understanding and the consent of the human subject. The Ethical Committee of the responsible University faculty (University of Bern) has approved the study (Nr.: 2019-07-00005).
Number of participants was calculated using G-power software. A moderate effect size was chosen as a standard in this calculation (65). The required sample size for each exercising condition – verum and sham - was 28 participants, expecting a moderate effect size (d = 0.5) for the t-test analysis between two dependent means and a requirement of 90% power.
Participants with one or more of the following criteria were excluded: Being pregnant, having osteosynthesis material (such as implants or screws) in the body, musculoskeletal disorders, joint problems (especially regarding the knee, hip, and back), herniated discs, rheumatism (such as spondylitis, gout, osteoporosis, osteoarthritis), cardiovascular complaints, disorders related to the sense of balance (such as hearing loss). Also, participants were advised to attend the study in a rested state and must not have had any intensive workout within the previous 24 h, because of musculoskeletal and cognitive effects. In order to attend the Stroop Test (66), participants must also not suffer from red-green color blindness or take medication known to affect the central nervous system.
A number of 55 students and acquaintances signed up for the study. No participants had to be excluded before, during or after the experiment. Body mass index was calculated as a participants weight in kilograms divided by height in meters squared. Students who participated were reimbursed with one of 15 mandatory participant-hours by the associated university. Acquaintances were thanked with sweets after the experiment.
Two vibration platforms named MediPlate® (Dormena GmbH, Liestal, Switzerland) were used in the current study. They reach frequencies between 6 and 13 Hz of ball-bearing side-alternating (rocking) vibrations. The MediPlate® represents a transportable vibration platform as it weighs only 15.5 kg and is rather small (length: 77 cm, width: 44 cm, height: 12.5 cm). Amplitude is between 2 mm and 8 mm depending how participants place their feet on the platform. In the current study, participants exercised with an amplitude of about 5 mm.
The verum condition was set at a frequency of 8.5 Hz (Level 20). It is experienced as slightly higher than the minimal stimulation parameter of 6 Hz (Level 1), which was used as sham condition. Acceleration forces – calculated as f(max) = amplitude * (2π * frequency)2−were 12.8 m/s2 (1.3 g) in the verum condition and 5.3 m/s2 (0.5 g) in the sham condition. Thus, forces of the verum condition on the body were lower than walking, which reaches between 2.7 g and 3.7 g (67).
Since there were no studies on the MediPlate® vibration platform so far, our decisions concerning chosen frequencies rely on experience with various vibration training studies (10) combined with recommendations from the designers of MediPlate®. A blank control group without any vibration was not carried out to ensure that participants were unaware of their group-allocation.
Musculoskeletal well-being and muscle relaxation were assessed with a short version of the self-administered questionnaire of Burger et al. (2012) before and after the exercise (3). The questions started with the lead-in phrase, “How do you rate your personal sensations regarding muscles and joints (back, shoulders and neck, legs) at this moment?” and were answered on a 100-point-rating-scale from zero (“not at all comfortable/relaxed”) to 100 (“as comfortable/relaxed as possible”).
Flexibility was assessed through the modified fingertip-to-floor method (mFTF). Compared to the original fingertip-to-floor method (FTF), where participants stand on the floor, participants stand on a box when attending the mFTF. This is an advantage as measurements of participants who are able to touch the floor or reach beyond can still be included (68). Participants are asked to bend over as far as possible keeping their legs and arms straight, while the examiners measured the distance to the box. This procedure was repeated three times. Gauvin et al. (69) reported high test-retest (r = 0.98), as well as high inter-rater reliability (r = 0.95) for the mFTF.
Additionally, sense of flexibility was assessed in one question: “How flexible do you feel at this moment?” (17). Answers could range from 0 being “a lot worse than usual”, to 100 being “much better than usual”, and with 50 being “same as always”.
Balance was measured with the modified Star Excursion Balance Test (mSEBT) (70). In this test, dynamic balance is assessed in the eight directions anterior, anteromedial, medial, posteromedial, posterior, posterolateral, lateral und anterolateral with both high intra-test (r = 0.84 to 0.93) and test-retest reliability (r = 0.89 to 0.93) (70, 71). Excursion distances were normalized to individual leg length of each participant, in order to exclude effects related to gender, because males were found to have significantly greater excursion distances than females (72). Pozo-Cruz et al. (2011) stated that previous studies found similar balance-test results for the dominant and non-dominant leg (73). Also, in the current study, balance of both legs dominant and non-dominant were measured.
Additionally, sense of balance and surefootedness were assessed with two questions: “How do you rate your personal feelings about your balance at this moment?” and “How sure-footed do you feel at this moment?” (17). Answers could range from 0 being “a lot worse than usual”, to 100 being “much better than usual”, and with 50 being “same as always”.
Cognitive interference was measured with the Stroop Color Word Test (66). In this well-established test (74), participants are given color words that are written in color and are asked to indicate the ink color of the word, thus having to ignore the dominant tendency of reading the word.
In the current study a digital form of the Stroop Color Word Test was applied, using the Inquisit 5 Lab program (Millisecond Software, LLC, Seattle, USA) on a computer. After a test trial, the experimental trial started. It consisted of 84 randomly sampled items with congruent (color word, e.g., “red” and the ink color it is presented in is the same, hence red), incongruent (color word, e.g., “black” and the ink color it is presented in is not the same, e.g., green) and neutral items (colored rectangles in black, red, blue or green). Test duration was approximately 3 min.
Laird et al. (2005) describes cognitive interference to be the difference between incongruent items and a control condition, either congruent, neutral, or non-lexical items (75). Analogous to a previous study on cognitive effects from SR-WBV (76), congruent items are compared to neutral items in the present study. A higher difference between both conditions means higher cognitive interference and thus lower inhibitory control (77).
Because keyboards often differ in latency-time (78), reaction response boxes V1.0 (© immo electronics) were applied instead of keyboards. Four buttons, according to the four presented colors, were placed between participant and computer screen. Participants held index- and middle finger of each hand on the buttons during the test. Figure 2 shows the Stroop Test on a computer set-up.
Figure 2. Stroop test on a computer set-up. The Stroop Color Word Test was held on a computer. In a test trial, congruent (color word and the color it is presented in are the same), incongruent (color word and the color it is presented in are not the same) and neutral items (colored rectangles in red, green, blue or black) were shown. After a test trial, the 3-minutes experimental trial with 84 randomly sampled items started.
The experiment was carried out by 2 examiners (MH, SS) in a laboratory room at the University of Bern. While the first examiner guided the participants through the procedure, the second examiner acted as an assistant. These roles were changed regularly to prevent monotony. In order to standardize the procedure, examiners followed a strict case report form (CRF). No more than one participant could attend the experiment, which lasted approximately 50 min.
Before participants arrived, they were randomly allocated to verum (8.5 Hz) or sham (6 Hz) group by flipping a coin. Participants then read through the study-information and signed a consent form to declare their voluntary participation and the possibility to stop the experiment whenever they wanted. Although participants were unaware of their group-allocation or their vibration frequencies, blinding of the examiners was not feasible. The first examiner explained the overall procedure but did not reveal the group allocation. Participants attended the baseline-measurements, starting with the baseline questionnaire, Stroop Color Word Test, flexibility test (mFTF) and balance test (mSEBT).
As in previous studies using an SR-WBV vibrating plate (17, 26), the vibration exercise with the MediPlate® consisted of three series that lasted one-minute each with two one-minute breaks in between. Participants were instructed to stand in the middle of the platform facing forward in an upright position with slightly bent knees (i.e., a skiing posture) and with their arms hanging loosely at their sides. In the short break in between, participants could loosen up and prepare for the following series.
Focusing on immediate effects on cognitive interference, participants attended the post-measurements in the following order straight after the exercise: Stroop Color Word Test, flexibility test, balance test and questionnaire. The post-questionnaire was longer as it also included demographical questions. The whole procedure is shown in Figure 3.
Figure 3. Flowchart of the procedure. Baseline-measurements started with the baseline questionnaire and were followed by the Stroop Color Word Test, flexibility test (mFTF) and then balance test (mSEBT); The sinusoidal side-alternating vibration exercise with the MediPlate® consisted of three series that lasted one-minute each with two one-minute breaks in between; Focusing on immediate effects on cognitive interference, participants attended the post-tests (starting with the Stroop Color Word Test, followed by the mSEBT and then mFTF) before the post-questionnaire.
Musculoskeletal well-being, muscle relaxation, flexibility, balance, and cognitive interference were analyzed in a dependent sample t-test examining differences between baseline and exercising conditions using SPSS (version 25, SPSS, IBM Inc., United States). P-values were two-tailed with an α-level set at 5%. Collected variables were not approximately normally distributed (p < .05) as assessed by Shapiro–Wilk Tests. Thus, graphical approaches, skewness and kurtosis were included in the decision, showing all variables to be close to normal. Also, according to Field (79) analysis of the hypotheses can be considered robust against violations of the normal distribution when the group size is equal. Pearson's descriptive statistics for the collected variables are shown in Table 1. Results of t-tests for each exercising condition are shown in Table 2. Effect sizes are, according to Cohen (80) described as small (d = 0.2), medium (d = 0.5), and large (d ≥ 0.8). The formula for the calculation of effect sizes for dependent t-test results is according to Dunlap and colleagues (81):
Fifty-five healthy students and acquaintances (44 female; mean age = 23.04 years, SD = 6.33 years; mean height = 170.39 cm, SD = 9.09; mean weight=63.09, SD = 11.29; mean BMI = 21.40, SD = 3.03) took part in the current study. All participants were randomly assigned to verum (N = 28) or sham (N = 27) condition.
Verum and sham groups did not differ significantly in any demographic characteristics or in baseline variables (Table 1).
A significant effect on musculoskeletal well-being was found after verum SS-WBV (t = −2.26 p = .032, N = 28), but not after sham SS-WBV (t = 0.93, p = .359, N = 27). Compared to baseline measurement (verum: 75.64 ± 31.84; sham: 80.41 ± 18.20), musculoskeletal well-being increased significantly after verum (88.50 ± 11.54), but not after sham SS-WBV (76.81 ± 26.15). Effect sizes using Cohen's d (75) on musculoskeletal well-being in the verum condition was d = −0.495, and in the sham condition d = 0.151.
A significant effect on muscle relaxation was found after verum SS-WBV (t = −2.21, p = 0.032, N = 28), but not after sham SS-WBV (t = −1.16, p = .258, N = 27). Compared to baseline measurement (verum: 73.39 ± 30.62; sham: 72.56 ± 19.01), muscle relaxation was significantly increased after verum (85.82 ± 13.53), but not after sham SS-WBV (76.96 ± 4.85). Effect sizes using Cohen's d (80) on muscle relaxation in the verum condition was d = −0.501, and in the sham condition d = −0.192.
A significant effect in balance (dominant leg) was found after verum SS-WBV (t = −5.52, p < .001, N = 28) and also after sham SS-WBV (t = −6.01, p < .001, N = 27). Compared to baseline measurement (verum: 74.81 ± 10.39; sham: 72.41 ± 7.28), the balance (dominant leg) increased significantly in verum (82.28 ± 11.76) and in sham SS-WBV (76.90 ± 6.70). Effect size using Cohen's d (75) on balance (dominant leg) in the verum condition was d = −0.663, and in the sham condition d = −0.621.
A significant effect in balance (non-dominant leg) was found after verum SS-WBV (t = −4.52, p < .001, N = 28) and also after sham SS-WBV (t = −5.36, p < .001, N = 27). Compared to baseline measurement (verum: 73.45 ± 7.03; sham: 72.82 ± 5.98), balance (non-dominant leg) increased significantly in verum (80.05 ± 10.47) and in sham SS-WBV (77.79 ± 7.57). Effect size using Cohen's d (80) on balance (non-dominant leg) in the verum condition was d = −0.689, and in the sham condition d = −0.695.
A significant effect in sense of balance was found after verum SS-WBV (t = −3.82, p = .001, N = 28), and also after sham SS-WBV (t = −4.69, p < .001, N = 27). Compared to baseline measurement (verum: 47.50 ± 6.12; sham: 48.96 ± 6.50), sense of balance increased significantly in verum (54.71 ± 10.31) and in sham SS-WBV (56.78 ± 10.82). Effect size using Cohen's d (80) on sense of balance in the verum condition was d = −0.824, and in the sham condition d = −0.86.
A significant effect in surefootedness was found after verum SS-WBV, (t = −2.66, P = .013, n = 28), but not after sham SS-WBV (t = −0.80, p = .429, N = 27). Compared to baseline measurement (verum: 48.86 ± 4.20; sham: 51.22 ± 7.07), surefootedness increased significantly in verum (53.61 ± 9.96), but not in sham SS-WBV (52.89 ± 8.43). Effect size using Cohen's d (80) on surefootedness in the verum condition was d = −0.581, and in the sham condition d = −0.213.
A significant effect in flexibility was found after verum SS-WBV (t = −5.63, p < .001, N = 28) and also after sham SS-WBV (t = −6.51, p < .001, n = 27). Compared to baseline measurement (verum: 3.77 ± 9.69; sham: 6.25 ± 11.03), flexibility increased significantly in verum (6.21 ± 8.61) and in sham SS-WBV (7.82 ± 11.03). Effect size using Cohen's d (80) on flexibility in the verum condition was d = −0.261, and in the sham condition d = −0.137.
A significant effect in sense of flexibility was found after verum SS-WBV (t = −4.55, p < .001, N = 28) and also after sham SS-WBV (t = −3.89, p = .001, N = 27). Compared to baseline measurement (verum: 44.04 ± 8.47; sham: 48.48 ± 11.85), flexibility increased significantly in verum (57.11 ± 12.22) and in sham SS-WBV (56.74 ± 13.40). Effect size using Cohen's d (80) on sense of flexibility in the verum condition was d = −1.244, and in the sham condition d = −0.650.
A significant smaller interference effect was found after verum SS-WBV (t = 2.14, p = .042, N = 28), but not after sham SS-WBV (t = −0.98, p = .335, N = 28). Compared to baseline measurement (verum: 145.96 ± 136.77; sham: 49.24 ± 439.90), the difference in reaction time between incongruent and control items decreased significantly after verum (86.97 ± 101.34), but not after sham SS-WBV (137.36 ± 162.07). Effect sizes using Cohen's d (80) on cognitive interference in the verum condition was d = 0.486, and in the sham condition d = −0.265.
Overall, promising effects were found for the verum WBV condition, but not for the sham condition, indicating acute musculoskeletal and cognitive effects of SS-WBV. More precisely, musculoskeletal well-being and muscle relaxation increased after SS-WBV with a vibration frequency of 8.5 Hz but not of 6 Hz (H1). Also, flexibility assessed through the modified fingertip-to-floor method (mFTF) as well as through a single-item question increased after both conditions, 8.5 Hz as well as 6 Hz. (H2). Sense of balance, which was assessed with a single-item question only increased after SS-WBV with a vibration frequency of 8.5 Hz but not of 6 Hz. However, balance measured with the modified Star Excursion Balance Test (mSEBT) improved after both SS-WBV conditions, 8.5 Hz and 6 Hz (H3). Lastly, cognitive interference measured with the Stroop Color Word Test decreased after SS-WBV with a vibration frequency of 8.5 Hz but not of 6 Hz (H4).
The aim of the current study was to conduct the acute effects of WBV training using a light and portable platform, incorporating the use of several physiological tests and questionnaires. Body balance performance measure included not only musculoskeletal outcomes, but also cognition. After one SS-WBV exercise, different physiological and cognitive measurements have shown improvement, with effect sizes for WBV training being small to moderate. Results might indicate that different variables could be sensitive for different vibration frequencies.
WBV training has proven its health promoting effects in various outcomes such as higher musculoskeletal well-being, better flexibility and increased balance (17, 26, 27, 82, 83). Musculoskeletal well-being and flexibility improved after one session of WBV-training only in the verum group. This supports previous findings where it was shown, that WBV increases flexibility (63, 83), because vibration increases blood circulation and generates more heat, which facilitates flexibility. Additionally, WBV causes muscles to contract and relax, which may raise the pain threshold and could lead to participants being able to stretch further while experiencing less pain (84, 85).
Piecha et al. (2014) have shown that WBV increases postural stability (55), which allows us to move safely, which could be related to improved surefootedness. One reason for increased postural stability could be enhanced muscle strength (86). Thus, changes in muscle strength might play a significant role in increasing postural stability and should be addressed in future studies. An increase in surefootedness was significant in the verum group, but not in the sham group, indicating that participants walked more safely after higher vibration stimulation. Balance-Tests however showed not only the verum (8.5 Hz), but also the sham group (6 Hz) increased in balance. One could assume that this result might be related to a training effect on the balance test. However, this might also indicate that WBV also effects balance when light frequencies are applied, maybe because proprioceptive training does not need as high frequencies as e.g., relaxation and musculoskeletal well-being.
Having a good balance is associated with less falls (87), because sensorimotor performance is better (88). But this is not the only explanation. Less falls are also related to better cognitive performance (89, 90), especially with executive functions (91, 92), such as inhibition. For example, Hausdorff et al. (2005) measured inhibition with the Stroop Test in non-demented older adults and have shown that a lower performance in the Stroop Test was also linked to a lower gait performance (93).
Recent studies have shown that cognition may be enhanced through WBV training in mice and in humans (94). In their randomized controlled trial, Boerema and colleagues postulated that daily vibration trainings over 5-weeks improve motor performance and reduce arousal-induced home cage activity in mice (94). In humans, WBV training improved brain function tested with the Stroop Color-Word test. Accordingly, a recently published review from a Brazilian research group on the effects of WBV on different cognitive variables described cognitive enhancement through training and suggests more clinical trials to establish beneficial training parameters (95).
Findings of cognitive effects after WBV training are still rare and the underlying processes are not fully understood yet. Studies with mice have shown increased cholinergic activity after WBV (94). Also, cholinergic activity in humans is positively associated with Stroop Test results (96). Therefore, improvement of inhibitory control in humans may be due to enhanced cholinergic activity increased by WBV training. On the contrary, improved inhibitory control after (repeated) WBV training may be due to the connection of sensory brain regions and the prefrontal cortex. Sensory stimulation, as perceived while executing WBV training, enhances neurotransmission not only in sensory brain regions, but also in the prefrontal cortex (97). However, this finding might be unique for WBV compared to other forms of physical activity (e.g., walking), because Sanders and colleagues have not found any cognitive effects in older persons with dementia after participation in a walking and lower limb strength training program over 12 weeks (98).
Finally, the finding that inhibitory control may be improved through WBV could be an important implication for occupational stress research. Stress at work impairs inhibitory control (99), while inhibitory control is a personal resource that helps to deal with high work demands. Inhibitory control has shown to be related with mindfulness in early adolescence (100) and mindfulness has been shown to be a personal resource that reduces work stress in line with the job demands-resources model (101). According to Lee and Chao (2012), inhibitory control is important for psychological well-being and for achieving mindfulness, and therefore may help to reduce interference from emotional distractors (e.g., an angry face, a negative thought, or a negative event) (102). Thus, people may intentionally avoid emotional distractors and can focus on desired or goal-related information promoting their own well-being (103). This could be noteworthy for future studies exploring personal resources to cope with work demands, but also to reduce cognitions that are related with weaker musculoskeletal function, such as fear- avoidance beliefs, maladaptive back beliefs, and concerns of falling.
The cognitive enhancement was measured with the Stroop Color Word Test. Inhibition and therefore cognition improved from pre to post intervention. Interestingly, these effects were shown in healthy young participants, mostly students who are expected to already have a high level of attention. As in previous WBV-studies on inhibitory control (104–106), a Stroop Test was implemented immediately after the exercise. Future studies may also take long-term effects of SS-WBV on cognition into account.
Further studies may also focus on the aging workforce who could profit the most from SS-WBV interventions focusing on gait performance and frequency of falls, since these have been shown to be related with Stroop Test results (93). In line with this, training parameters concerning different outcome variables must be defined, so users understand which methods (e.g., SR-WBV, SS-WBV), frequencies, and training durations should be applied, if they not only want to increase bone density or reduce musculoskeletal pain, but also improve balance and inhibitory control.
Overall, SS-WBV has shown to be an appropriate way to improve different health-related outcomes. In this initial step, SS-WBV exercise has shown to increase inhibitory control in a young and healthy sample. Implemented as a worksite intervention, SS-WBV is expected to improve balance and reduce falls, especially in older workers.
Research on falls and gait control differ between single falls and recurrent falls. On the one hand, single falls are known as accidental falls, and mostly due to extrinsic reasons, e.g., environmental or housing conditions (88, 89). On the other hand, recurrent falls are often usually based on intrinsic reasons, e.g., advanced age, diseases, or gait disorders (107). Recurrent fallers could profit from interventions such as preventive and therapeutic exercises, in order to improve mobility (108). Because only few effective treatment possibilities exist to effectively improve gait control and balance for fall prevention, new intervention possibilities must be explored (109).
SS-WBV is easily applied and has shown to be effective in improving balance among different studies (26, 63, 64). Interestingly, SS-WBV might affect balance in different ways: Firstly, SS-WBV might improve balance by a proprioceptive training of muscles (110). Secondly, SS-WBV might improve balance through relaxation of stiff muscles, and hence less weakened proprioceptive information in sensory tissues (111, 112), and pain inhibition (113). Thirdly, studies have shown increased inhibitory control, i.e., less cognitive interference, after WBV exercises. This might also indicate a contribution to the prevention of slip trip and fall incidents, because not only gait performance (93), but also falls (90–92) seem to be connected with Stroop Test results. However, underlying mechanisms need to be further explored to fully understand the relationship between WBV, inhibitory control, balance and falls.
In the current study, WBV exercises were applied on a transportable and manageable vibration platform which does not take up much space and time, because participants are not likely to sweat and would not need to change clothes or shower after WBV-training. Furthermore, WBV-training is very short. In the current study, three minutes of SS-WBV stimulation already showed positive effects. Due to these benefits and the positive physical and cognitive outcomes that were found in the current study, a next step could be to study SS-WBV health-interventions at work. Faes et al. (2018) found promising effects in increased balance and musculoskeletal well-being in office workers who spend a substantial amount of time in sitting position (26). Because several physiological and cognitive measures have been improved, positive effects are not only related to less falls, but may also be linked to better life satisfaction and personal well-being of employees, which could lead to more satisfied employees and better work performances (114, 115).
The described effects of the current study were only observed directly after one training session. Further studies should address long-term effects of repeated SS-WBV trainings. Another limitation of the current study pertains to the “chosen” vibration frequencies. Because the lowest possible frequency (6 Hz) of the SS-WBV platform did not differ enough with the verum condition (8.5 Hz), effects on balance were observed in both conditions. To study effects on balance, the sham-group should possibly experience lower or no vibration frequencies, e.g., control group. However, no vibration frequency would carry the problem of the blindness of participants, because one might guess their group allocation when nothing happens. Finally, our study relates to a relatively young age of participants. Since slip, trip and falls are especially common in the elderly, further studies with older people are necessary.
The raw data supporting the conclusions of this article will be made available by the authors, without undue reservation.
The studies involving human participants were reviewed and approved by Ethikkomission der Universität Bern. The participants provided their written informed consent to participate in this study.
FY designed the study, organized the experiment, analyzed the data, and wrote the manuscript, RSC helped design the study and wrote the manuscript, HML performed the measurements, analyzed the data, and wrote the manuscript, EA supervised the design of the study and analysis and wrote the manuscript. All authors contributed to the article and approved the submitted version.
Open access funding provided by Lucerne University Of Applied Sciences And Arts.
We thank Samira Spring and Marina Herlig for testing the participants. We also thank Andreas Saladin for his contribution in providing and installing the vibration plates.
The authors declare that the research was conducted in the absence of any commercial or financial relationships that could be construed as a potential conflict of interest.
All claims expressed in this article are solely those of the authors and do not necessarily represent those of their affiliated organizations, or those of the publisher, the editors and the reviewers. Any product that may be evaluated in this article, or claim that may be made by its manufacturer, is not guaranteed or endorsed by the publisher.
1. SUVA. Unfallstatistik UVG 2020 (2020). Available at: https://www.suva.ch/de-CH/material/Dokumentationen/unfallstatistik-uvg-ssuv-2020 (cited October 14, 2021).
2. Collins JW, Bell JL, Gronqvist R, Courtney TK, Lombardi DA, Sorock GS, et al. Multidisciplinary research to prevent SLIP, TRIP, and FALL (STF) incidents among hospital workers. Contemp Ergon. (2008) 2008:693.
3. Wallmann HW. The basics of balance and falls. Home Health Care Manag Pract. (2009) 21(6):436–9. doi: 10.1177/1084822309337189
4. Horlings CG, Van Engelen BG, Allum JH, Bloem BR. A weak balance: the contribution of muscle weakness to postural instability and falls. Nat Clin Pract Neurol. (2008) 4(9):504–15. doi: 10.1038/ncpneuro0886
5. Kemmlert K, Lundholm L. Slips, trips and falls in different work groups—with reference to age and from a preventive perspective. Appl Ergon. (2001) 32(2):149–53. doi: 10.1016/S0003-6870(00)00051-X
6. Maki BE, Sibley KM, Jaglal SB, Bayley M, Brooks D, Fernie GR, et al. Reducing fall risk by improving balance control: development, evaluation and knowledge-translation of new approaches. J Saf Res. (2011) 42(6):473–85. doi: 10.1016/j.jsr.2011.02.002
8. Kaewthummanukul T, Brown KC. Determinants of employee participation in physical activity: critical review of the literature. AAOHN J. (2006) 54(6):249–61. doi: 10.1177/216507990605400602
9. Robroek SJ, Van Lenthe FJ, Van Empelen P, Burdorf A. Determinants of participation in worksite health promotion programmes: a systematic review. Int J Behav Nutr Phys Act. (2009) 6(1):26. doi: 10.1186/1479-5868-6-26
10. Elfering A, Zahno J, Taeymans J, Blasimann A, Radlinger L. Acute effects of stochastic resonance whole body vibration. World J Orthop. (2013) 4(4):291. doi: 10.5312/wjo.v4.i4.291
11. Arlinghaus KR, Johnston CA. The importance of creating habits and routine. Am J Lifestyle Med. (2019) 13(2):142–4. doi: 10.1177/1559827618818044
12. Ramond-Roquin A, Bodin J, Serazin C, Parot-Schinkel E, Ha C, Richard I, et al. Biomechanical constraints remain major risk factors for low back pain. Results from a prospective cohort study in French male employees. Spine J. (2015) 15(4):559–69. doi: 10.1016/j.spinee.2013.05.040
13. O’Reilly N. Get up, stand up: Evidence on sedentary working shows employees need to get moving. Personnel Today (2016). https://www.personneltoday.com/hr/get-stand-evidence-sedentary-working-shows-employees-need-get-moving/.
14. Johanning E. Whole-body vibration-related health disorders in occupational medicine–an international comparison. Ergonomics. (2015) 58(7):1239–52. doi: 10.1080/00140139.2015.1005170
15. Scherrer AM. Risikofaktor vibrationen: so schützen sie die gesundheit ihrer mitarbeitenden. SUVA (2012).
16. Bovenzi M. Health effects of mechanical vibration. G Ital Med Lav Ergon. (2005) 27(1):58–64. PMID: 15915675.15915675
17. Burger C, Schade V, Lindner C, Radlinger L, Elfering A. Stochastic resonance training reduces musculoskeletal symptoms in metal manufacturing workers: a controlled preventive intervention study. Work. (2012) 42(2):269–78. doi: 10.3233/WOR-2012-1350
18. Elfering A, Thomann J, Schade V, Radlinger L. Stochastic resonance whole body vibration reduces musculoskeletal pain: a randomized controlled trial. World J Orthop. (2011) 2:116–20. doi: 10.5312/wjo.v2.i12.116
19. Lau RW, Liao LR, Yu F, Teo T, Chung RC, Pang MY. The effects of whole body vibration therapy on bone mineral density and leg muscle strength in older adults: a systematic review and meta-analysis. Clin Rehabil. (2011) 25(11):975–88. doi: 10.1177/0269215511405078
20. Marín PJ, Rhea MR. Effects of vibration training on muscle strength: a meta-analysis. J Strength Cond Res. (2010) 24(2):548–56. doi: 10.1519/JSC.0b013e3181c09d22
21. Merriman H, Jackson K. The effects of whole-body vibration training in aging adults: a systematic review. J Geriatr Phys Ther. (2009) 32(3):134–45. doi: 10.1519/00139143-200932030-00009
22. Rehn B, Lidström J, Skoglund J, Lindström B. Effects on leg muscular performance from whole-body vibration exercise: a systematic review. Scand J Med Sci Sports. (2002) 17(1):2–11. doi: 10.1111/j.1600-0838.2006.00578.x
23. Ritzmann R, Kramer A, Gruber M, Gollhofer A, Taube W. EMG Activity during whole body vibration: motion artifacts or stretch reflexes? Eur J Appl Physiol. (2010) 110(1):143–51. doi: 10.1007/s00421-010-1483-x
24. Rogan S, Hilfiker R, Schmid S, Radlinger L. Stochastic resonance whole-body vibration training for chair rising performance on untrained elderly: a pilot study. Arch Gerontol Geriatr. (2012) 55(2):468–73. doi: 10.1016/j.archger.2012.02.011
25. Slatkovska L, Alibhai SM, Beyene J, Hu H, Demaras A, Cheung AM. Effect of 12 months of whole-body vibration therapy on bone density and structure in postmenopausal women: a randomized trial. Ann Intern Med. (2011) 155(10):668–79. doi: 10.7326/0003-4819-155-10-201111150-00005
26. Faes Y, Maguire C, Notari M, Elfering A. Stochastic resonance training improves balance and musculoskeletal well-being in office workers: a controlled preventive intervention study. Rehabil Res Pract. (2018) 2018:9. doi: 10.1155/2018/5070536
27. Elfering A, Arnold S, Schade V, Burger C, Radlinger L. Stochastic resonance whole-body vibration, musculoskeletal symptoms, and body balance: a worksite training study. Saf Health Work. (2013) 4(3):149–55. doi: 10.1016/j.shaw.2013.07.002
28. Lechner L, de Vries H. Effects of an employee fitness program on reduced absenteeism. J Occup Environ Med. (1997) 39(9):827–31. doi: 10.1097/00043764-199709000-00005
29. Kaeding TS. Whole-Body vibration training in workplace-health promotion: a promising intervention? Austin Sports Med. (2017) 2(2):1018. Austin Sports Med. (2017) 2(2):1018.
30. Oliveira LC, Oliveira RG, Pires-Oliveira DAA. Effects of whole body vibration on bone mineral density in postmenopausal women: a systematic review and meta-analysis. Osteoporos Int. (2016) 27(10):2913–33. doi: 10.1007/s00198-016-3618-3
31. Rogan S, de Bruin ED, Radlinger L, Joehr C, Wyss C, Stuck NJ, et al. Effects of whole-body vibration on proxies of muscle strength in old adults: a systematic review and meta-analysis on the role of physical capacity level. Eur Rev Aging Phys Act. (2015) 12(1):12. doi: 10.1186/s11556-015-0158-3
32. Kessler J, Radlinger L, Baur H, Rogan S. Effect of stochastic resonance whole body vibration on functional performance in the frail elderly: a pilot study. Arch Gerontol Geriatr. (2014) 59(2):305–11. doi: 10.1016/j.archger.2014.06.005
33. Herren K, Hängärtner C, Oberli A, Radlinger L. Cardiovascular and metabolic strain during stochastic resonance therapy in stroke patients. Physioscience. (2009) 5(01):13–7. doi: 10.1055/s-0028-1109140
34. Haas CT, Turbanski S, Kessler K, Schmidtbleicher D. The effects of random whole-body-vibration on motor symptoms in Parkinson's Disease. NeuroRehabilitation. (2006) 21(1):29–36. doi: 10.3233/NRE-2006-21105
35. Washif JA, Teichmann J, Kok LY, Schmidtbleicher D. Using stochastic resonance and strength training as part of a rehabilitation programme for recurrent low back pain treatment: a case study. J Exerc Rehabil. (2019) 15(1):139–47. doi: 10.12965/jer.1836532.266
36. Rohlmann A, Schmidt H, Gast U, Kutzner I, Damm P, Bergmann G. In vivo measurements of the effect of whole body vibration on spinal loads. Eur Spine J. (2014) 23(3):666–72. doi: 10.1007/s00586-013-3087-8
37. Rauch F. Vibration therapy. Dev Med Child Neurol. (2009) 51(s4):166–8. doi: 10.1111/j.1469-8749.2009.03418.x
38. Morel DS, da Fontoura Dionello C, Moreira-Marconi E, Brandão-Sobrinho-Neto S, Paineiras-Domingos LL, Souza PL, et al. Relevance of whole body vibration exercise in sport: a short review with soccer, diver and combat sport. Afr J Tradit Complement Altern Med. (2017) 14(4S):19–27. doi: 10.21010/ajtcam.v14i4S.3
39. Sharma S, Hayat Z. Effects of whole-body vibration on sports performance: a systematic review and meta-analysis. Sci Sports. (2022). 37(4):231–43. doi: 10.1016/j.scispo.2021.06.015
40. Hortobágyi T, Lesinski M, Fernandez-del-Olmo M, Granacher U. Small and inconsistent effects of whole body vibration on athletic performance: a systematic review and meta-analysis. Eur J Appl Physiol. (2015) 115(8):1605–25. doi: 10.1007/s00421-015-3194-9
41. Alzaben N, Kim IJ. Effect of whole-body vibration technology in preventing fall incidence: a review. 2022 Advances in science and engineering technology international conferences (ASET). IEEE (2022). p. 1–6.
42. Wang XQ, Gu W, Chen BL, Wang X, Hu HY, Zheng YL, et al. Effects of whole-body vibration exercise for non-specific chronic low back pain: an assessor-blind, randomized controlled trial. Clin Rehabil. (2019) 33(9):1445–57. doi: 10.1177/0269215519848076
43. Xie L, Yi SX, Peng QF, Liu P, Jiang H. Retrospective study of effect of whole-body vibration training on balance and walking function in stroke patients. World J Clin Cases. (2021) 9(22):6268. doi: 10.12998/wjcc.v9.i22.6268
44. Sañudo B, Seixas A, Gloeckl R, Rittweger J, Rawer R, Taiar R, et al. Potential application of whole body vibration exercise for improving the clinical conditions of COVID-19 infected individuals: a narrative review from the world association of vibration exercise experts (wavex) panel. Int J Environ Res Public Health. (2020) 17(10):3650. doi: 10.3390/ijerph17103650
45. Seidel H, Heide R. Long-term effects of whole-body vibration: a critical survey of the literature. Int Arch Occup Environ Health. (1986) 58(1):1–26. doi: 10.1007/BF00378536
46. Marinelli L, Quartarone A, Hallett M, Frazzitta G, Ghilardi M. The many facets of motor learning and their relevance for Parkinson's Disease. Clin Neurophysiol. (2017) 128:1127–41. doi: 10.1016/j.clinph.2017.03.042
47. Yuan P, Raz N. Prefrontal cortex and executive functions in healthy adults: a meta-analysis of structural neuroimaging studies. Neurosci Biobehav Rev. (2014) 42:180–92. doi: 10.1016/j.neubiorev.2014.02.005
48. Baddeley AD, Della Sala S. Working memory and executive control. Phil. Trans. R. Soc. Lond. B (1996) 351(1346):1397–404. doi: 10.1098/rstb.1996.0123
49. Miyake A, Friedman NP, Emerson MJ, Witzki AH, Howerter A, Wager TD. The unity and diversity of executive functions and their contributions to complex “frontal lobe” tasks: a latent variable analysis. Cogn Psychol. (2000) 41(1):49–100. doi: 10.1006/cogp.1999.0734
50. de Ridder DTD, Lensvelt-Mulders G, Finkenauer C, Stok FM, Baumeister RF. Taking stock of self-control: a meta-analysis of how trait self-control relates to a wide range of behaviors. Pers Soc Psychol Rev. (2012) 16(1):76–99. doi: 10.1177/1088868311418749
51. Cummings JL. Clinical neuropsychiatry. London and New York: Grune & Stratton (1985). doi: 10.1002/hup.470020212
52. Bolton DAE, Richardson JK. Inhibitory control and fall prevention: why stopping matters. Front Neurol. (2022) 13:853787. doi: 10.3389/fneur.2022.853787
53. Wen HJ, Tsai CL. Neurocognitive inhibitory control ability performance and correlations with biochemical markers in obese women. Int J Environ Res Public Health. (2020) 17(8):2726. doi: 10.3390/ijerph17082726
54. Garon N, Bryson SE, Smith IM. Executive function in preschoolers: a review using an integrative framework. Psychol Bull. (2008) 134(1):31. doi: 10.1037/0033-2909.134.1.31
55. Piecha M, Juras G, Krol P, Sobota G, Polak A, Bacik B. The effect of a short-term and long-term whole-body vibration in healthy men upon the postural stability. PLoS One. (2014) 9(2):e88295. doi: 10.1371/journal.pone.0088295
56. Sarason IG, Pierce GR, Sarason BR, editors. Cognitive interference: theories, methods, and findings. Hillsdale, NJ, US: Lawrence Erlbaum Associates, Inc (1996). Vol. xii, p. 441.
57. Granacher U, Muehlbauer T, Bridenbaugh S, Bleiker E, Wehrle A, Kressig R. Balance training and multi-task performance in seniors. Int J Sports Med. (2010) 31(05):353–8. doi: 10.1055/s-0030-1248322
58. Garatachea N, Molinero O, Martínez-García R, Jimenez-Jimenez R, Gonzalez-Gallego J, Marquez S. Feelings of well being in elderly people: relationship to physical activity and physical function. Arch Gerontol Geriatr. (2009) 48(3):306–12. doi: 10.1016/j.archger.2008.02.010
59. Zhang L, Weng C, Liu M, Wang Q, Liu L, He Y. Effect of whole-body vibration exercise on mobility, balance ability and general health status in frail elderly patients: a pilot randomized controlled trial. Clin Rehabil. (2014) 28(1):59–68. doi: 10.1177/0269215513492162
60. Paineiras-Domingos LL, da Cunha Sá-Caputo D, Reis AS, Francisca Santos A, Sousa-Gonçalves CR, Dos Anjos EM, et al. Assessment through the short physical performance battery of the functionality in individuals with metabolic syndrome exposed to whole-body vibration exercises. Dose Response. (2018) 16(3):1559325818794530. doi: 10.1177/1559325818794530
61. Fuchs D. Dancing with gravity—why the sense of balance is (the) fundamental. Behav Sci. (2018) 8(1):7. doi: 10.3390/bs8010007
62. Fort A, Romero D, Bagur C, Guerra M. Effects of whole-body vibration training on explosive strength and postural control in young female athletes. J Strength Cond Res. (2012) 26(4):926–36. doi: 10.1519/JSC.0b013e31822e02a5
63. Despina T, George D, George T, Sotiris P, Alessandra DC, George K, et al. Short-term effect of whole-body vibration training on balance, flexibility and lower limb explosive strength in elite rhythmic gymnasts. Hum Mov Sci. (2014) 33:149–58. doi: 10.1016/j.humov.2013.07.023
64. Ritzmann R, Kramer A, Bernhardt S, Gollhofer A. Whole body vibration training-improving balance control and muscle endurance. PloS One. (2014) 9(2):e89905. doi: 10.1371/journal.pone.0089905
65. Faul F, Erdfelder E, Lang AG, Buchner A. G* power 3: a flexible statistical power analysis program for the social, behavioral, and biomedical sciences. Behav Res Methods. (2007) 39(2):175–91. doi: 10.3758/BF03193146
66. Stroop JR. Studies of interference in serial verbal reactions. J Exp Psychol. (1935) 18(6):643. doi: 10.1037/h0054651
67. LaFortune MA. Three-dimensional acceleration of the tibia during walking and running. J Biomech. (1991) 24(10):877–86. doi: 10.1016/0021-9290(91)90166-K
68. Buckup K. Klinische tests an knochen, muskeln und gelenken. Stuttgart, New York, S: Thieme (2009).
69. Gauvin MG, Riddle DL, Rothstein JM. Reliability of clinical measurements of forward bending using the modified fingertip-to-floor method. Phys Ther. (1990) 70(7):443–7. doi: 10.1093/ptj/70.7.443
70. Hertel J, Braham RA, Hale SA, Olmsted-Kramer LC. Simplifying the star excursion balance test: analyses of subjects with and without chronic ankle instability. J Orthop Sports Phys Ther. (2006) 36(3):131–7. doi: 10.2519/jospt.2006.36.3.131
71. Gribble PA, Kelly SE, Refshauge KM, Hiller CE. Interrater reliability of the star excursion balance test. J Athl Train. (2013) 48(5):621–6. doi: 10.4085/1062-6050-48.3.03
72. Gribble PA, Hertel J. Considerations for normalizing measures of the star excursion balance test. Meas Phys Educ Exerc Sci. (2003) 7(2):89–100. doi: 10.1207/S15327841MPEE0702_3
73. Pozo-Cruz BD, Hernández Mocholí MA, Adsuar JC, Parraca JA, Muro I, Gusi N. Effects of whole body vibration therapy on main outcome measures for chronic non-specific low back pain: a single-blind randomized controlled trial. J Rehabil Med. (2011) 43(8):689–94. doi: 10.2340/16501977-0830
74. Boonstra AM, Oosterlaan J, Sergeant JA, Buitelaar JK. Executive functioning in adult ADHD: a meta-analytic review. Psychol Med. (2005) 35(8):1097–108. doi: 10.1017/S003329170500499X
75. Laird AR, McMillan KM, Lancaster JL, Kochunov P, Turkeltaub PE, Pardo JV, et al. A comparison of label-based review and ALE meta-analysis in the stroop task. Hum Brain Mapp. (2005) 25(1):6–21. doi: 10.1002/hbm.20129
76. Faes Y, Rolli Salathé C, Cébe C, Szukics A, Elfering A. Musculoskeletal and cognitive effects of stochastic resonance whole body vibration: a randomized controlled trial. Revista Hospital Universitário Pedro Ernesto (HUPE). (2020) 19(1):20–30.
77. Mathias JL, Wheaton P. Changes in attention and information-processing speed following severe traumatic brain injury: a meta-analytic review. Neuropsychology. (2007) 21(2):212. doi: 10.1037/0894-4105.21.2.212
78. Luu D, Aptekar-Cassels W, Hanson L, Murphy K. Keyboard latency (2017). Available from: https://danluu.com/keyboard-latency/.
81. Dunlap WP, Cortina JM, Vaslow JB, Burke MJ. Meta-analysis of experiments with matched groups or repeated measures designs. Psychol Methods. (1996) 1(2):170. doi: 10.1037/1082-989X.1.2.170
82. Hartman-Petrycka M, Lebiedowska A, Stolecka-Warzecha A, Szumski A, Błońska-Fajfrowska B. The influence of stochastic resonance whole-body vibration on women over 50 years of age—preliminary studies based on Patients’ own experiences. Appl Sci. (2021) 11(9):3980. doi: 10.3390/app11093980
83. Sa-Caputo DC, Paineiras-Domingos LL, Oliveira R, Neves MF, Brandão A, Marin PJ, et al. Acute effects of whole-body vibration on the pain level, flexibility, and cardiovascular responses in individuals with metabolic syndrome. Dose Response. (2018) 16(4):1559325818802139. doi: 10.1177/1559325818802139
84. Fowler BD, Palombo KT, Feland JB, Blotter JD. Effects of whole-body vibration on flexibility and stiffness: a literature review. Int J Exerc Sci. (2019) 12(3):735. PMID: 31156749; PMCID: PMC6533098.31156749
85. Rasti E, Rojhani-Shirazi Z, Ebrahimi N, Sobhan MR. Effects of whole body vibration with exercise therapy versus exercise therapy alone on flexibility, vertical jump height, agility and pain in athletes with patellofemoral pain: a randomized clinical trial. BMC Musculoskelet Disord. (2020) 21(1):1–9. doi: 10.1186/s12891-020-03732-1
86. Jordan MJ, Norris SR, Smith DJ, Herzog W. Vibration training: an overview of the area, training consequences, and future considerations. J Strength Cond Res. (2005) 19(2):459–66. doi: 10.1519/13293.1
87. Papalia GF, Papalia R, Diaz Balzani LA, Torre G, Zampogna B, Vasta S, et al. The effects of physical exercise on balance and prevention of falls in older people: a systematic review and meta-analysis. J Clin Med. (2020) 9(8):2595. doi: 10.3390/jcm9082595
88. MacKinnon CD. Chapter 1 - sensorimotor anatomy of gait, balance, and falls. In: Day BL, Lord SR, editors. Balance, gait, and falls. Minneapolis, MN, United States: Elsevier (2018). p. 3–26. (Handbook of Clinical Neurology; vol. 159). Available at: https://www.sciencedirect.com/science/article/pii/B978044463916500001X.
89. Jabbar KA, Tan DGH, Seah WT, Lau LK, Pang BWJ, Ng DHM, et al. Enhanced gait variability index and cognitive performance in Asian adults: results from the yishun study. Gait Posture. (2022) 97:216–21. doi: 10.1016/j.gaitpost.2022.07.156
90. Anstey KJ, Wood J, Kerr G, Caldwell H, Lord SR. Different cognitive profiles for single compared with recurrent fallers without dementia. Neuropsychology. (2009) 23(4):500. doi: 10.1037/a0015389
91. Buracchio TJ, Mattek NC, Dodge HH, Hayes TL, Pavel M, Howieson DB, et al. Executive function predicts risk of falls in older adults without balance impairment. BMC Geriatr. (2011) 11(1):74. doi: 10.1186/1471-2318-11-74
92. Herman T, Mirelman A, Giladi N, Schweiger A, Hausdorff JM. Executive control deficits as a prodrome to falls in healthy older adults: a prospective study linking thinking, walking, and falling. J Gerontol A Biol Sci Med Sci. (2010) 65(10):1086–92. doi: 10.1093/gerona/glq077
93. Hausdorff JM, Yogev G, Springer S, Simon ES, Giladi N. Walking is more like catching than tapping: gait in the elderly as a complex cognitive task. Exp Brain Res. (2005) 164(4):541–8. doi: 10.1007/s00221-005-2280-3
94. Boerema AS, Heesterbeek M, Boersma SA, Schoemaker R, de Vries EF, van Heuvelen MJ, et al. Beneficial effects of whole body vibration on brain functions in mice and humans. Dose Response. (2018) 16(4):1–10. doi: 10.1177/1559325818811756
95. de Souza Freitas AC, Gaspar JF, Rossi Marques de Souza GC, Inamonico JH, Bachur CK, Coelho-Oliveira AC, et al. The effects of whole-body vibration on cognition: a systematic review. Rev Bras Crescimento Desenvolv Hum. (2022) 32(1):108–19. doi: 10.36311/jhgd.v32.12864
96. Bohnen NI, Kaufer DI, Hendrickson R, Ivanco LS, Lopresti BJ, Constantine GM, et al. Cognitive correlates of corticalcholinergic denervation in Parkinson'sdisease And parkinsonian dementia. J Neurol. (2006) 253(2):242–7. doi: 10.1007/s00415-005-0971-0
97. Braak H, Braak E, Yilmazer D, Bohl J. Topical review: functional anatomy of human hippocampal formation and related structures. J Child Neurol. (1996) 11(4):265–75. doi: 10.1177/088307389601100402
98. Sanders LMJ, Hortobágyi T, Karssemeijer EGA, Van der Zee EA, Scherder EJA, Van Heuvelen MJG. Effects of low-and high-intensity physical exercise on physical and cognitive function in older persons with dementia: a randomized controlled trial. Alzheimers Res Ther. (2020) 12(1):1–15. doi: 10.1186/s13195-019-0546-4
99. Roos LE, Knight EL, Beauchamp KG, Berkman ET, Faraday K, Hyslop K, et al. Acute stress impairs inhibitory control based on individual differences in parasympathetic nervous system activity. Biol Psychol. (2017) 125:58–63. doi: 10.1016/j.biopsycho.2017.03.004
100. Oberle E, Schonert-Reichl KA, Lawlor MS, Thomson KC. Mindfulness and inhibitory control in early adolescence. J Early Adolesc. (2012) 32(4):565–88. doi: 10.1177/0272431611403741
102. Lee YC, Chao HF. The role of active inhibitory control in psychological well-being and mindfulness. Pers Individ Dif. (2012) 53(5):618–21. doi: 10.1016/j.paid.2012.05.001
103. Grover SL, Teo ST, Pick D, Roche M. Mindfulness as a personal resource to reduce work stress in the job demands-resources model. Stress Health. (2017) 33(4):426–36. doi: 10.1002/smi.2726
104. Fuermaier AB, Tucha L, Koerts J, van Heuvelen MJ, van der Zee EA, Lange KW, et al. Good vibrations–effects of whole body vibration on attention in healthy individuals and individuals with ADHD. PLoS ONE. (2014) 9(2):e90747. doi: 10.1371/journal.pone.0090747
105. Regterschot GRH, Van Heuvelen MJ, Zeinstra EB, Fuermaier AB, Tucha L, Koerts J, et al. Whole body vibration improves cognition in healthy young adults. PLoS ONE. (2014) 9(6):e100506. doi: 10.1371/journal.pone.0100506
106. den Heijer AE, Groen Y, Fuermaier AB, van Heuvelen MJ, van der Zee EA, Tucha L, et al. Acute effects of whole body vibration on inhibition in healthy children. PloS ONE. (2015) 10(11):e0140665. doi: 10.1371/journal.pone.0140665
107. Dunn JE, Rudberg MA, Furner SE, Cassel CK. Mortality, disability, and falls in older persons: the role of underlying disease and disability. Am J Public Health. (1992) 82(3):395–400. doi: 10.2105/AJPH.82.3.395
108. Tromp AM, Smit JH, Deeg DJH, Bouter LM, Lips P. Predictors for falls and fractures in the longitudinal aging study Amsterdam. J Bone Miner Res. (1998) 13(12):1932–9. doi: 10.1359/jbmr.1998.13.12.1932
109. Noll DR. Management of falls and balance disorders in the elderly. J Am Osteopath Assoc. (2013) 113(1):17–22. PMID: 23329802.23329802
110. Trans T, Aaboe J, Henriksen M, Christensen R, Bliddal H, Lund H. Effect of whole body vibration exercise on muscle strength and proprioception in females with knee osteoarthritis. Knee. (2009) 16(4):256–61. doi: 10.1016/j.knee.2008.11.014
111. Brumagne S, Cordo P, Lysens R, Verschueren S, Swinnen S. The role of paraspinal muscle spindles in lumbosacral position sense in individuals with and without low back pain. Spine. (2000) 25(8):989–94. doi: 10.1097/00007632-200004150-00015
112. Mazzocchio R, Scarfò GB, Mariottini A, Muzii VF, Palma L. Recruitment curve of the soleus H-reflex in chronic back pain and lumbosacral radiculopathy. BMC Musculoskelet Disord. (2001) 2(1):1–8. doi: 10.1186/1471-2474-2-4
113. Ruhe A, Fejer R, Walker B. Center of pressure excursion as a measure of balance performance in patients with non-specific low back pain compared to healthy controls: a systematic review of the literature. Eur Spine J. (2011) 20(3):358–68. doi: 10.1007/s00586-010-1543-2
Keywords: whole-body vibration (WBV), musculoskeletal, flexibility, balance, cognition, inhibition, stroop-color-word interference task, SLIP trip and fall accidents
Citation: Faes Y, Rolli Salathé C, Herlig ML and Elfering A (2023) Beyond physiology: Acute effects of side-alternating whole-body vibration on well-being, flexibility, balance, and cognition using a light and portable platform A randomized controlled trial. Front. Sports Act. Living 5:1090119. doi: 10.3389/fspor.2023.1090119
Received: 4 November 2022; Accepted: 3 January 2023;
Published: 30 January 2023.
Edited by:
Heiner Baur, Bern University of Applied Sciences, SwitzerlandReviewed by:
Mario Bernardo-Filho, Rio de Janeiro State University, Brazil© 2023 Faes, Rolli Salathé, Herlig and Elfering. This is an open-access article distributed under the terms of the Creative Commons Attribution License (CC BY). The use, distribution or reproduction in other forums is permitted, provided the original author(s) and the copyright owner(s) are credited and that the original publication in this journal is cited, in accordance with accepted academic practice. No use, distribution or reproduction is permitted which does not comply with these terms.
*Correspondence: Yannik Faes eWFubmlrLmZhZXNAaHNsdS5jaA==
†ORCID Yannik Faes orcid.org/0000-0002-0062-9058 Marina Luna Herlig orcid.org/0000-0003-0904-7211 Achim Elfering orcid.org/0000-0003-4274-0261
Specialty Section: This article was submitted to Injury Prevention and Rehabilitation, a section of the journal Frontiers in Sports and Active Living
Disclaimer: All claims expressed in this article are solely those of the authors and do not necessarily represent those of their affiliated organizations, or those of the publisher, the editors and the reviewers. Any product that may be evaluated in this article or claim that may be made by its manufacturer is not guaranteed or endorsed by the publisher.
Research integrity at Frontiers
Learn more about the work of our research integrity team to safeguard the quality of each article we publish.