- Performance Fit Laboratory, BOA Technology Inc., Denver, CO, United States
Trail running participation has grown over the last two decades. As a result, there have been an increasing number of studies examining the sport. Despite these increases, there is a lack of understanding regarding the effects of footwear on trail running biomechanics in ecologically valid conditions. The purpose of our study was to evaluate how a Wrap vs. Lace closure (on the same shoe) impacts running biomechanics on a trail. Thirty subjects ran a trail loop in each shoe while wearing a global positioning system (GPS) watch, heart rate monitor, inertial measurement units (IMUs), and plantar pressure insoles. The Wrap closure reduced peak foot eversion velocity (measured via IMU), which has been associated with fit. The Wrap closure also increased heel contact area, which is also associated with fit. This increase may be associated with the subjective preference for the Wrap. Lastly, runners had a small but significant increase in running speed in the Wrap shoe with no differences in heart rate nor subjective exertion. In total, the Wrap closure fit better than the Lace closure on a variety of terrain. This study demonstrates the feasibility of detecting meaningful biomechanical differences between footwear features in the wild using statistical tools and study design. Evaluating footwear in ecologically valid environments often creates additional variance in the data. This variance should not be treated as noise; instead, it is critical to capture this additional variance and challenges of ecologically valid terrain if we hope to use biomechanics to impact the development of new products.
Introduction
Trail running participation, particularly in ultra-distances, has grown over the last two decades (1, 2). As a result, recent studies have examined the physiological predictors of trail running performance (3, 4), in-lab biomechanical changes resulting from prolonged downhill runs (5) and trail races (6), and biomechanics and physiology during outdoor trail running (7, 8). Despite this emerging research field, there is a lack of understanding regarding the effects of footwear on trail running. Unfortunately, most of this research is still done in the laboratory, where the unique challenges of trail running are not placed on the participants.
Footwear is a key piece of equipment that that impacts running performance in road running (9, 10). While road running is dominated by sagittal plane motion (11), trail running increases motion in the frontal and transverse planes (7, 11). As such, trail running exacts different demands on footwear than road running. Recent studies have demonstrated that shoe upper material (12) and footwear closure systems (13, 14) can impact biomechanical performance, specifically in non-sagittal plane motions. The upper and fit of footwear is considered a critical design feature by running footwear experts (15), however little research exists on the impact of uppers on trail running performance. While the benefits of alternative shoe upper designs were elucidated in-the-lab during agility-based movements, they have not been evaluated for running “in-the-wild.”
Despite the growing evidence of the validity of wearable technology (16), there is relatively little known about the effects of fit of footwear on the trail in ecologically relevant terrain. Inside of the lab, better fitting shoes have been related to reduced vertical loading rates (via force plates) and reduced pronation (eversion) velocities (17). Surrogates of such biomechanical metrics could be captured with wearable technologies such as inertial measurement units (IMUs) that contain accelerometers and gyroscopes. For instance, an IMU attached to the foot can measure foot eversion velocity near foot contact and peak acceleration – a surrogate for vertical loading rate (18). Additionally, IMUs have been used to characterize the terrain of uphill trail running vs. in-lab running through medial-lateral accelerations (7) – with runners exhibiting larger medial-lateral accelerations outdoors. As footwear uppers have been shown to influence agility movement transition times (12, 14) and frontal plane kinematics (13), medial-lateral accelerations may be sensitive to changes in the footwear upper.
Plantar pressure insoles are an additional wearable technology that may be used to evaluate footwear outside-of-the-lab. Plantar pressures have been used to study foot strike patterns on trail [with road shoes (19)], peak and mean pressures during marathons (20), and to quantify differences in pressure distribution during longitudinal training studies (21). Moreover, plantar pressure has revealed differences between lace-up boots and lace-free boots in the field; lace up boots reduce peak pressures under the heel and toes while increasing heel contact area (22). Inside the lab, both increased contact area and reduced peak heel and toe pressures have been shown to differentiate between comfortable orthotics (23) and different footwear features (17, 24). In total, both the heel and the toe region of plantar pressure have been shown to be sensitive to footwear fit and/or subjective comfort.
While evaluating the impact of footwear outside the lab often results in additional variation in the data, this variation is indicative of real-world usage and experiences. In fields such as cognition (25) and neuroscience (26), the effects of interventions can be missed if only tested in the laboratory due to a lack of context or ecological validity. While testing running biomechanics on a treadmill provides many observations in a tightly controlled setting, assessments of footwear on a treadmill may not generalize to overground running (27, 28) and will certainly not represent the challenges of the terrain where subjects will use trail footwear. As a result, using a treadmill to test trail running footwear limits the generalizability of findings and may be entirely inappropriate to assess this type of footwear. This limitation extends to other commonly tested biomechanical interventions such as prostheses, exoskeletons, and road running footwear. If we hope to bridge the gap between biomechanics and product design, researchers must expand their testing toolbox to include wearable sensors, explore the critical variation that will be present in the data, and design experiments in such a way to rigorously test product in ecologically relevant terrain.
The purpose of our study was twofold: (1) to evaluate how changing a trail shoe closure system impacts trail running biomechanics and performance, and (2) to highlight an experimental paradigm for testing biomechanical interventions on a trail. Specifically, we evaluated trail running in the La Sportiva Cyklon with a Wrap closure and a Lace closure with respect to preference and performance. We hypothesized that the Wrap closure would provide a better fit than the Lace closure. We anticipated a shoe with a better closure would: (1) reduce foot eversion velocity, (2) reduce loading rate as measured by peak acceleration or peak jerk, (3) reduce the medial/lateral acceleration, (4) reduce peak pressure in the toe and/or the heel, and (5) increase the contact area in the toe and/or the heel. We evaluated the peak jerk in addition to the peak acceleration as jerk provides a similar time-derivative as loading rate. We also evaluated subjective and objective (heart rate) measures of exertion to ensure that there was no difference in effort between the two shoes.
Materials and methods
Participants
Thirty subjects (15 male, 15 female means ± standard deviations: height: 172 ± 6 cm, mass: 65 ± 8 kg, age: 34 ± 8 yrs) provided written informed consent prior to participation in trail running. The protocol was approved by the Institutional Review Board (protocol: 22-BOAT-101). Participants with US men's shoe size 10 and 11 and US women's shoe size 7.5 and 9 were recruited for this study. To be eligible to participate, subjects were required to run at least 24 km per week and participate in trail running once per week (weather permitting) with no injuries that resulted in missing more than a week of running in the past six months. All participants ran in the La Sportiva Cyklon (Wrap) and a retrofitted La Sportiva Cyklon with Laces (Lace) (Figure 1).
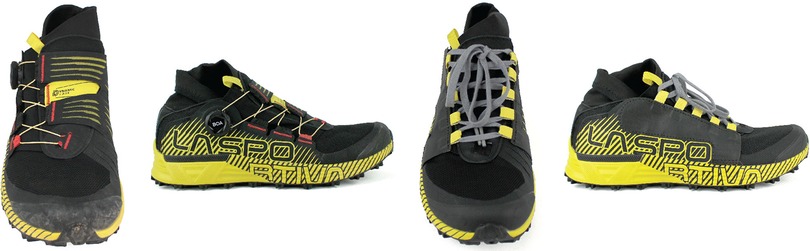
Figure 1. The La sportiva cyklon with the wrap upper (left) and the retro-fitted lace version of the shoe (right).
Protocol
The trail run was performed on a 1.6 km loop near Morrison, Colorado, USA (Figure 2) and had three sections. The uphill portion of the trail was approximately 580 m and had an average incline of four degrees (varied between 13 degrees uphill and one degree downhill). The top portion of the trail was more technical than the other two sections. This portion of the trail was approximately 520 m with an average incline of two degrees (varied between nine degrees uphill and two degrees downhill). The downhill portion of the trail was approximately 470 m with an average decline of six degrees (varied between three and 11 degrees downhill). The technicity of the different portions of the trail, according to the international mountain bicycling association, were intermediate (blue) for the uphill and downhill and advanced (black) for the top. The participants first ran a warm-up loop with a trained experimenter to become familiar with the terrain and the loop. The subjects then ran the loop another four times to test the shoes in a randomized counterbalanced order (A-B-B-A) to account for familiarization and fatigue. Subjects were encouraged to run at similar speeds between each lap. Data were collected from IMUs (±16 g and ±2,000°/sec at 1125 Hz with 16-bit sensitivity, ±200 g at 1,600 Hz with 13-bit sensitivity, IMeasureU, Denver, USA) attached to the heel counter, plantar pressure sensors (100 Hz, XSENSOR, Calgary, CAN), a global positioning system (GPS) watch (1 Hz, Suunto, Vantaa, Finland), and an optical heart rate sensor (1 Hz, Polar Verity Sense, Polar Electro Inc., Kempele, Finland) attached to the upper arm. Previous versions of this heart rate sensor have been validated against electrocardiograms during level and uphill running (29). GPS coordinates and heart rate data were synchronously recorded on the GPS watch. If subjects had a greater than one minute difference between their laps, their data were excluded from further analyses. Prior to each lap, subjects performed three synchronizing jumps to indicate when the subjects began running. After these jumps, the subject started the GPS watch and began running.
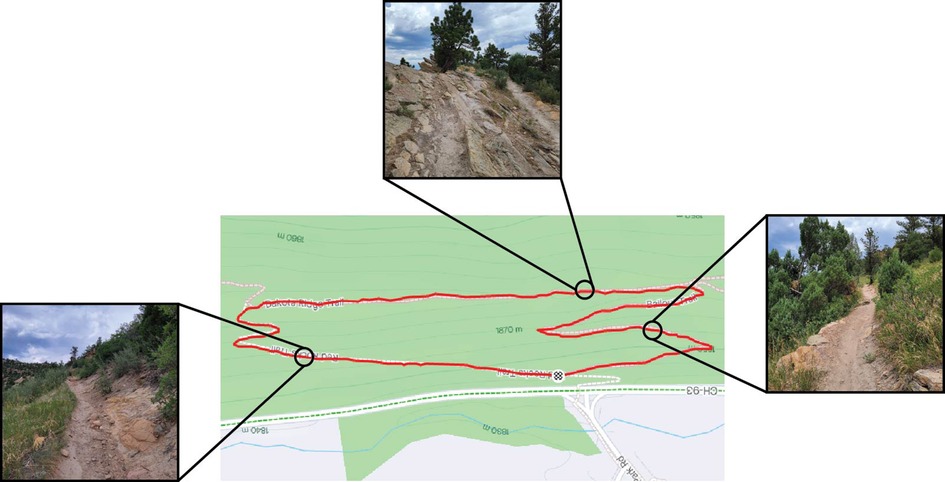
Figure 2. Topographic map with route subjects ran (shown in red). Subjects ran the route in a counter-clockwise fashion. Pictures show example terrain of the different trail sections: uphill (right), top (top), and downhill (left). The average uphill slope was +4°, the average top slope was +2°, and the average downhill slope was −6°. Topographic map was used with permissions from Strava (http://www.strava.com).
Subjective outcomes
After running the warm-up lap, the subjects reviewed the questionnaire. After each lap in a study condition, subjects rated their exertion on an ordinal scale of 0 (not tired) to 10 (exhausted). After the second run in each shoe, they responded to questions regarded the performance of the shoe for uphill, level, and downhill running. Subjects also rated their confidence while running in the shoe. Finally, subjects rated the overall fit, the forefoot fit, midfoot fit, and the heel fit. All questions besides the fit for the specific foot regions were rated on an ordinal scale from zero to 10, where zero was “poor” and 10 was “great”. For the specific foot regions (e.g., forefoot), zero corresponded with too loose, five was perfect and 10 was too tight. Similar ordinal scales have been shown to be a reliable assessment of footwear comfort during running (30). For the full questionnaire see Supplementary material.
Biomechanical outcomes
The IMUs (three-axis accelerometer, three-axis gyroscope) were used to determine running speed, peak acceleration (sometimes referred to as shock), jerk, medial-lateral acceleration ranges, and peak eversion angular velocity. We first combined the low-g and high-g accelerometer data to avoid saturation that can occur during running (31). This was accomplished by interpolating the high-g accelerometer (1,600 Hz) to the low-g accelerometer frequency (1,125 Hz) with the UNIX timestamps that accompanied each sample. The high-g accelerometer datapoints were then substituted for the low-g datapoints whenever the low-g accelerometer was saturated (at ±16 g). Running strides were then segmented based on the magnitude of the jerk squared (32). Next, both the accelerometer and gyroscope signals were bypass-filtered with a second-order, low-pass 50 Hz Butterworth filter. Using these filtered signals, the IMU-based running speed was determined based on previously published algorithms (32) that have been evaluated for different speeds and slopes of running (33). In short, we computed stride length and divided it by stride time, as defined by subsequent foot contact detections. Stride length was determined through double integrating the acceleration once it had been corrected for gravity. We utilized linear de-drifting to correct for integration error based on zero velocity updates at mid-stance (32, 34). Based on the filtered accelerometer signals, peak acceleration magnitude, peak jerk magnitude, and the medial/lateral accelerometer range [similar to (7)] over the stride were determined. For the peak eversion angular velocity, we filtered the original gyroscope signal with a second-order, bi-pass, low-pass 30 Hz Butterworth filter to better capture running kinematic frequencies and to limit the effects of transient peaks in the gyroscope signal near foot contact. The peak eversion angular velocity was then computed for the first 20% of the stride to capture the foot contact portion of the stride. All IMU metrics were extracted after the detected three hops for the entire running loop.
Average heel and toe contact area along with peak toe and peak heel pressures during the stance phase of running were extracted from the plantar pressure. Running stance phase for the plantar pressure was detected with implementing a gradient decent algorithm on the total insole force to provide foot-contact and toe-off events. The heel region was defined as the rear 20% of the plantar pressure sensor. The toe region was defined as the front 20% of the sensor. These two regions are defined though the XSENSOR software and approximately correspond to the respective regions of the anatomical foot. All pressure metrics were extracted after the detected three hops for the entire running loop.
The GPS coordinates from the watch were used to segment the trail into three portions: uphill, top technical, and downhill (see Figure 2 above) based on the latitude and longitude. As the GPS watch was started immediately after the three synchronizing hops, the biomechanical metrics were examined for each section separately. Average heart rate for each section was computed for each section to evaluate physiological exertion. All biomechanical data processing was performed in Python version 3.9.7.
Statistics
Linear mixed effects models were used to evaluate the statistical difference between the Wrap and Lace shoes for the subjective outcomes along with biomechanical outcomes. All statistical analyses were performed in R Statistical Software (version 4.1.2) with the LMER (35) and emmeans (36) packages. We first created a linear mixed effects model to evaluate the difference in subjective responses between the configurations (: Lace or Wrap) and average heart rate from each trail section that utilized an independent (random) intercept for each subject (Equation 1). Differences in average heart rate were based on estimated marginal means.
All other biomechanical outcomes (“Outcome”) were then evaluated with a mixed effects model with an independent (random) intercept for each subject and independent slope encoding the subject-specific effect of switching from one shoe condition to the other (Equation 2). In this model, the biomechanical outcomes (e.g., peak eversion velocity) from each detected stride (IMU) or step (plantar pressure) from each subject were utilized. Statistical differences for the biomechanical outcomes were evaluated separately for each of the different trail sections (i.e., uphill, top, downhill) as the biomechanical outcomes are speed dependent. For all statistical tests, α was set to 0.05. Presented percent differences were with respect to estimated marginal means. These models use a similar structure to recent work from our lab (13, 14) and represent a natural way to model the subject-specific responses to footwear (37).
Results
One subject was excluded from analyses because their trail running laps had a range greater than one minute (2 min. 23 s).
Subjective outcomes
The Wrap shoe was subjectively rated better for uphill, level, and downhill running (p ≤ 0.002, Table 1). The Wrap shoe provided subjectively more confidence than the Lace shoe (p < 0.001). Though the Wrap shoe was rated with a better overall fit (p < 0.001), the Wrap shoe was rated as tighter than the Lace shoe in the forefoot (p = 0.04) with no differences in rating for the midfoot or heel fit (p > 0.1).

Table 1. Subjective feedback regarding the performance of the shoes on different terrain and the fit of the shoes. Presented are study means ± standard deviations (N = 29).
Exertion outcomes
There were no statistical differences in either subjective (p = 0.46) or objective exertion outcomes (p > 0.17, Table 2). The average heart rate difference between Wrap and Lace shoes was less than one beat per minute on all sections of the trail (Table 2).
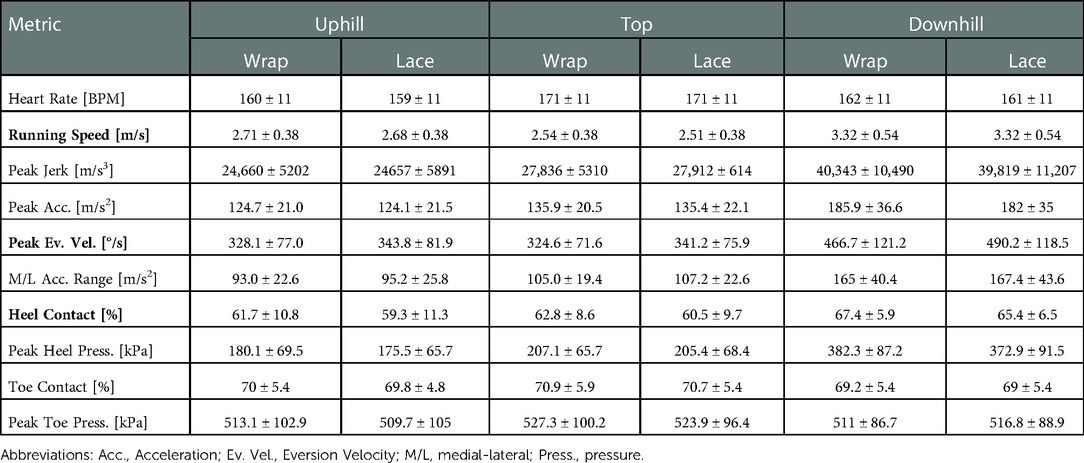
Table 2. Biomechanical metrics examined during the trail running loop. The bolded metrics indicate that there were significant differences between the Wrap and the Lace for each of the different trail sections. Presented are study means ± standard deviations (N = 29).
IMU outcomes
Subjects ran slightly faster in the Wrap than in the Lace shoe on all three sections of the trail (average difference: 0.03 to 0.05 m/s, p < 0.022, Figure 3, Table 2). Peak jerk, peak acceleration, and the medial/lateral acceleration range were not significantly different between the two shoes for any of the trail sections (p > 0.35, p > 0.08, and p > 0.20, respectively). The peak eversion velocity was on average 5% lower in the Wrap than the Lace for the uphill (p = 0.025), technical top (p = 0.008), and downhill (p = 0.043) portions of the trail.
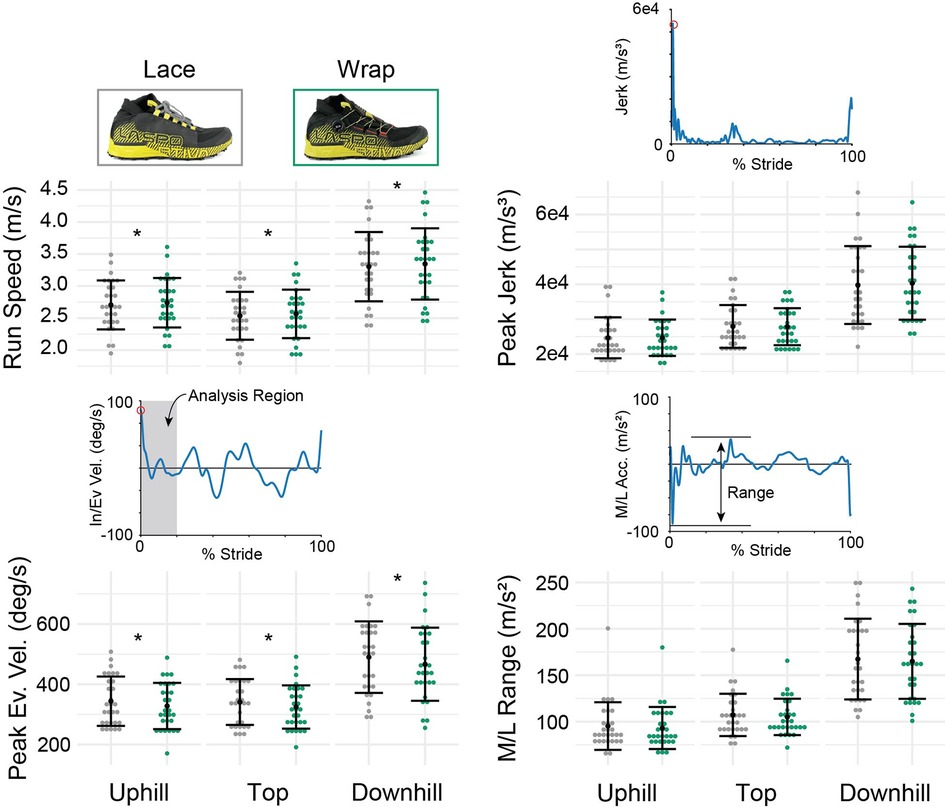
Figure 3. IMU-based outcomes. The individual dots represent subject-average results. The black dots and adjoining error bars represent study averages and standard deviations (N = 29). Asterisks (*) indicate significant differences between the Lace and the Wrap shoes for each of the different sections of the trail. Above the peak jerk, peak eversion velocity (Ev. Vel.), and medial/lateral acceleration range (M/L Range) are example curves from one subject and one stride to demonstrate how the metric was computed.
Pressure outcomes
The average percent heel contact area during the stance phase of running increased by an average magnitude of 2% in the Wrap over the Lace for all sections of the trail (p < 0.001, Figure 4, Table 2). The average toe contact area during the stance phase of running was not different between the two shoes for any section of the trail (p > 0.21). Both the peak heel and toe pressures did not differ between the two shoes for any section of the trail (p > 0.087).
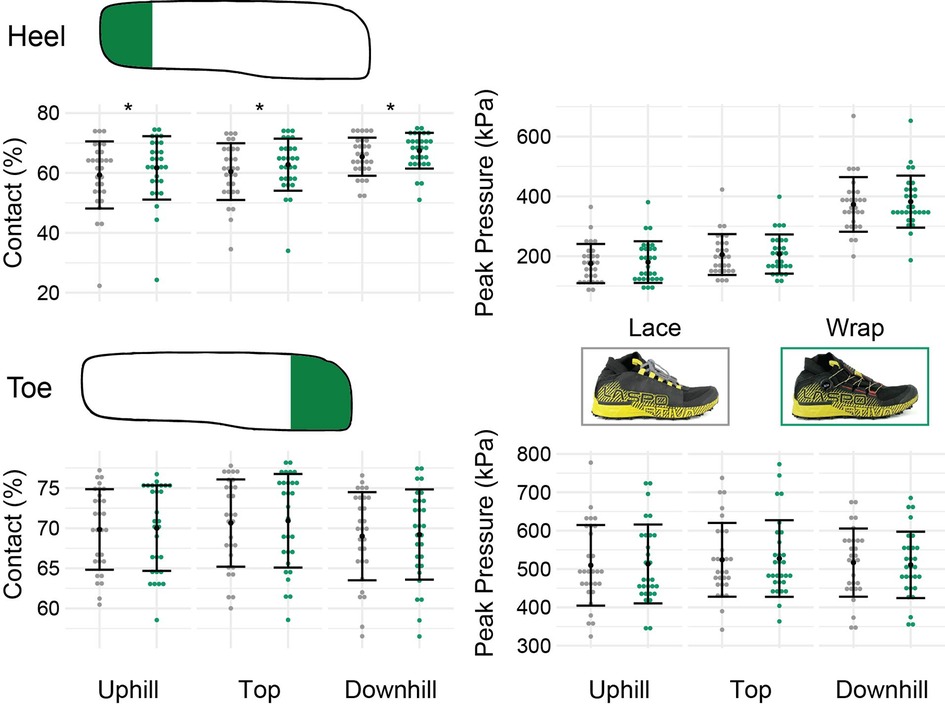
Figure 4. Plantar pressure outcomes. The top row are heel outcomes with a depiction of the heel region in the XSENSOR insoles. The bottom rows are toe outcomes with a depiction of the toe region in the XSENSOR insoles. The individual dots represent subject-average results. The black dots and adjoining error bars represent study averages and standard deviations (N = 29). Asterisks (*) indicate significant differences between the lace and the wrap shoes for each of the different sections of the trail.
Discussion
This was the first study to evaluate trail-specific footwear on ecologically valid terrain. We observed a statistically significant decrease in peak eversion velocity, slightly faster running speed, and increase in heel contact area in the Wrap shoe with no differences in qualitative or quantitative measures of exertion. These biomechanical changes were accompanied by greater qualitative scores in overall fit and confidence while running on each part of the trail. Similarly, various BOA wrap configurations have reduced frontal plane kinematics and times to change direction during agility movements and were accompanied by increased qualitative fit (13). In contrast to two of our hypotheses, there were no differences in peak accelerations or medial-lateral accelerations in the Wrap Shoe. We also did not observe any differences in the toe contact area, peak toe pressure, nor the peak heel pressure. Given the observed differences in footwear conditions, we propose the counterbalanced testing of product on a trail with wearable sensors is a valid design that should be used for trail product and may be extended to evaluate other biomechanical interventions.
Biomechanical features of fit
The Wrap shoe exhibited biomechanical features of a better fitting shoe along with subjects preferring the overall fit over the lace. We observed a significant decrease in peak eversion velocity in the Wrap shoe relative to Lace on each segment of the trail. Similar effects have been observed in better fitting footwear (17). Such a reduction may indicate that there is a better coupling between the footwear and the shoe. In fact, several subjects anecdotally mentioned that there was more relative motion (or slop) in the Lace condition. Future studies may want to examine the connection between this frontal plane velocity and the risk of ankle sprains. Medial and lateral ankle sprains result from excessive eversion/inversion angular velocities and angles (39, 40) and ankle sprains are the most common injury amongst trail runners (38).
We did not observe a difference between the Wrap and Lace in surrogate measures for vertical loading rate, which has been associated with footwear fit (17). Peak acceleration is proposed as a wearable-based surrogate for vertical loading rate (18), we also investigated peak jerk as it provides the same time-derivative as vertical loading rate. The acceleration and jerk magnitudes were dominated by the vertical and anterior-posterior directions, which could indicate these measurements are more sensitive to sagittal plane fit. As we did not observe a difference in the peak jerk nor the peak acceleration magnitudes, but we did observe a difference in the peak eversion velocity: the Wrap shoe has greater implications for footwear fit in the frontal plane rotation rather than the sagittal plane.
In addition to examining biomechanical metrics related to fit, we explored the relations between subjective feedback and metrics from wearables. Such connections between the perception of fit and biomechanics have been previously established using plantar pressure (23). We observed a significant increase in the heel contact area in the Wrap shoe which may contribute to the improvement in the “Downhill Performance” score (Table 1). Over a third of the subjects reported (in the free form response, see Supplementary Material) that they were sliding forward within the Lace shoe during downhill running which could result in less heel contact area. However, the heel contact area may have multiple implications as the Wrap had improved heel contact area on each of the different sections of the trail. We speculate that while the foot may slide forward within the shoe during downhill running, it may slip in the vertical direction during uphill running as runners tend to transition towards a midfoot strike pattern (19), challenging the ability of the upper to hold the heel in contact with the midsole. Seven subjects stated that the Lace had more heel slipping, which could implicate this metric as a quantitative estimate for heel hold. In the future, new technologies may be needed to better connect subjective feedback regarding footwear sliding with biomechanical outcomes such as plantar pressure that can measure shear [which has thus far only been prototyped (41)].
The debate: Highly controlled vs. ecologically valid
There are trade-offs between performing tightly controlled experiments within the lab compared with more variable data obtained in ecologically valid environments. Mainly, there is greater variability on the trail vs. in the lab. This variability can manifest from changes in temperature, trail conditions, subject foot placement, wind, and more. While laboratory settings tightly control these variables, trail running shoes need to perform in such conditions and therefore need to be tested as such. The variation in data collection conditions increases the variability in biomechanical outcomes, resulting in the need to recruit enough subjects and collect enough observations per subject (e.g., steps) to maintain statistical power. A mixed effects model that includes each individual step from each subject allows for the inter-individual variability in biomechanical measures to be accounted for in the analysis. Critically, footwear features such as fit and outsole features are often designed to mitigate the exact challenges that contribute to this variability such as sloppy rocks and steep gradients. Therefore, to test these design features it is essential to test these products on a trail. To the second aim of our study, we performed a repeatable experimental design to test trail products in an ecologically valid setting while limiting confounding variables. We segmented the trail into three unique sections via GPS (uphill, flat, and downhill) to be included as a covariate, instructed all participants to run at similar speeds for each section of a trail, and used a trail loop that could be completed four times within a single session without substantial changes in RPE so subjects could be tested in a counterbalanced order. We also measured their running speed, heart rate, and rating of perceived exertion, which could be included as covariates in our statistical model. For instance, including running speed (measured via IMU, Figure 3) within a statistical model (Equation 3) did not change the outcomes of the study; however, it did reduce the p-values for each of the IMU outcome significant findings.
Trail terrain is a critical benefit to evaluating the effects of footwear features. In the laboratory, it is common to evaluate shoe features [e.g., (42, 43)] and biomechanical adaptations (44) on an instrumented treadmill. Treadmill running instigates unique biomechanics [as compared to overground (27),], which can be altered with changing the treadmill belt surface (45) or technicity (11). Such unique adaptations can influence footwear feature biomechanical effects (28). Furthermore, transitioning from the treadmill to the trail induces unique biomechanical adaptations (7). As the footwear we studied here are intended to be used on trail, we propose that these shoes must be tested with the specific biomechanical adaptations that are instigated by trail running. Though we observed difference between the lace and wrap upper configurations on trail, it is an open question as to if footwear interventions instigate similar biomechanical effects indoors vs. outdoors.
Limitations
There are several limitations to acknowledge. Both the experimenters and the subjects were unable to be blinded to the footwear conditions. Additionally, the insole and IMU data were not able to be aligned post-hoc. As such, statistical analyses that account for running speed (Equation 3) could not be performed with the plantar pressure outcomes.
Conclusion
The wrap shoe provided improved qualitative and quantitative fit as compared to the lace version of the same shoe. Moving forward, shoe manufacturers and trail runner alike may want to choose shoes with wraps over laces for enhanced fit.
Data availability statement
The raw data supporting the conclusions of this article will be made available by the authors, without undue reservation.
Ethics statement
The studies involving human participants were reviewed and approved by Pearl IRB. The patients/participants provided their written informed consent to participate in this study.
Author contributions
Conceptualization, E.C.H., K.H., and D.F.; Methodology, E.C.H., K.H., and D.F.; Software, E.C.H., and D.F.; Validation, E.C.H., K.H., and D.F.; Formal Analysis, E.C.H.; Investigation, E.C.H., K.H., and D.F.; Resources, D.F.; Data Curation, E.C.H.; Writing – Original Draft Preparation, E.C.H.; Writing – Review & Editing, E.C.H., K.H., and D.F.; Visualization, E.C.H.; Supervision, D.F.; Project Administration, D.F.; Funding Acquisition, D.F. All authors contributed to the article and approved the submitted version.
Funding statement
This study received funding from BOA Technology Inc. The funder was not involved in the study design, collection, analysis, interpretation of data, the writing of this article or the decision to submit it for publication. All authors declare no other competing interests.
Acknowledgments
We would like to thank Hannah McDermott for retrofitting the La Sportiva shoes. We would also like to thank Kelsey Amoroso for her help with subject recruitment and data collections. Finally, we would like to thank Milena Singletary, Ife Olawore, Bethany Kilpatrick, and Adam Luftglass for help with data collection. We would like to thank Brett Vladika for the support in the early stages of the research process.
Conflict of interest
The authors of this paper are employees of BOA Technology Inc., the funder of this study.
Publisher's note
All claims expressed in this article are solely those of the authors and do not necessarily represent those of their affiliated organizations, or those of the publisher, the editors and the reviewers. Any product that may be evaluated in this article, or claim that may be made by its manufacturer, is not guaranteed or endorsed by the publisher.
Supplementary material
The Supplementary Material for this article can be found online at https://www.frontiersin.org/articles/10.3389/fspor.2022.1076609/full#supplementary-material.
References
1. Hoffman MD, Ong JC, Wang G. Historical analysis of participation in 161 km ultramarathons in North America. Int J Hist Sport. (2010) 27(11):1877–91. doi: 10.1080/09523367.2010.494385
2. Scheer V. Participation trends of ultra endurance events. Sports Med Arthrosc. (2019) 27(1):3–7. doi: 10.1097/JSA.0000000000000198
3. de Waal SJ, Gomez-Ezeiza J, Venter RE, Lamberts RP. Physiological indicators of trail running performance: a systematic review. Int J Sports Physiol Perform. (2021) 16(3):325–32. doi: 10.1123/ijspp.2020-0812
4. Lemire M, Hureau TJ, Favret F, Geny B, Kouassi BYL, Boukhari M, et al. Physiological factors determining downhill vs uphill running endurance performance. J Sci Med Sport. (2021) 24(1):85–91. doi: 10.1016/j.jsams.2020.06.004
5. Khassetarash A, Vernillo G, Martinez A, Baggaley M, Giandolini M, Horvais N, et al. Biomechanics of graded running: part II—joint kinematics and kinetics. Scand J Med Sci Sports. (2020) 30(9):1642–54. doi: 10.1111/sms.13735
6. Vercruyssen F, Tartaruga M, Horvais N, Brisswalter J. Effects of footwear and fatigue on running economy and biomechanics in trail runners. Med Sci Sports Exerc. (2016) 48(10):1976–84. doi: 10.1249/MSS.0000000000000981
7. Nicot F, Sabater-Pastor F, Samozino P, Millet GY, Rupp T. Effect of ground technicity on cardio-respiratory and biomechanical parameters in uphill trail running. Eur J Sport Sci. (2021) 22:1–11. doi: 10.1080/17461391.2021.1995507
8. Giandolini M, Horvais N, Rossi J, Millet GY, Morin JB, Samozino P. Effects of the foot strike pattern on muscle activity and neuromuscular fatigue in downhill trail running. Scand J Med Sci Sports. (2017) 27(8):809–19. doi: 10.1111/sms.12692
9. Worobets J, Wannop JW, Tomaras E, Stefanyshyn D. Softer and more resilient running shoe cushioning properties enhance running economy. Footwear Sci. (2014) 6(3):147–53. doi: 10.1080/19424280.2014.918184
10. Hoogkamer W, Kipp S, Kram R. The biomechanics of competitive male runners in three marathon racing shoes: a randomized crossover study. Sports Med. (2019) 49(1):133–43. doi: 10.1007/s40279-018-1024-z
11. Gantz AM, Derrick TR. Kinematics and metabolic cost of running on an irregular treadmill surface. J Sports Sci. (2018) 36(10):1103–10. doi: 10.1080/02640414.2017.1357829
12. Subramanium A, Honert EC, Cigoja S, Nigg BM. The effects of shoe upper construction on mechanical ankle joint work during lateral shuffle movements. J Sports Sci. (2021) 39:1791–9. doi: 10.1080/02640414.2021.1898174
13. Harrison K, Feeney D, Pryhoda MK, Dicharry J, Nelson NM, Shelburne KB, et al. Alternative upper configurations during agility-based movements: part 2, joint-level biomechanics. Footwear Sci. (2021) 13(2):167–80. doi: 10.1080/19424280.2021.1899296
14. Pryhoda MK, Wathen RJ, Dicharry J, Shelburne KB, Feeney D, Harrison K, et al. Alternative upper configurations during agility-based movements: part 1, biomechanical performance. Footwear Sci. (2021) 13(1):91–103. doi: 10.1080/19424280.2020.1853824
15. Honert EC, Mohr M, Lam WK, Nigg S. Shoe feature recommendations for different running levels: a delphi study. PLoS One. (2020) 15(7):e0236047. doi: 10.1371/journal.pone.0236047
16. Benson LC, Clermont CA, Bošnjak E, Ferber R. The use of wearable devices for walking and running gait analysis outside of the lab: a systematic review. Gait Posture. (2018) 63:124–38. doi: 10.1016/j.gaitpost.2018.04.047
17. Hagen M, Hennig EM. Effects of different shoe-lacing patterns on the biomechanics of running shoes. J Sports Sci. (2009) 27(3):267–75. doi: 10.1080/02640410802482425
18. Napier C, Willy RW, Hannigan BC, McCann R, Menon C. The effect of footwear, running speed, and location on the validity of two commercially available inertial measurement units during running. Front Sports Act Living. (2021) 3:643385. Available at: https://www.frontiersin.org/articles/10.3389/fspor.2021.643385. doi: 10.3389/fspor.2021.643385
19. Mo S, Chan ZYS, Lai KKY, Chan PPK, Wei RXY, Yung PSH, et al. Effect of minimalist and maximalist shoes on impact loading and footstrike pattern in habitual rearfoot strike trail runners: an in-field study. Eur J Sport Sci. (2021) 21(2):183–91. doi: 10.1080/17461391.2020.1738559
20. Hohmann E, Reaburn P, Tetsworth K, Imhoff A. Plantar pressures during long distance running: an investigation of 10 marathon runners. J Sports Sci Med. (2016) 15(2):254–62. Available at: https://www.jssm.org/researchjssm-15-254.xml.xml#27274662
21. Escamilla-Martínez E, Gómez-Martín B, Fernández-Seguín LM, Martínez-Nova A, Pedrera-Zamorano JD, Sánchez-Rodríguez R. Longitudinal analysis of plantar pressures with wear of a running shoe. Int J Environ Res Public Health. (2020) 17(5):1707. doi: 10.3390/ijerph17051707
22. Dobson JA, Riddiford-Harland DL, Steele JR. Effects of wearing gumboots and leather lace-up work boots on plantar loading when walking on a simulated underground coal mine surface. Footwear Sci. (2018) 10(3):139–48. doi: 10.1080/19424280.2018.1486461
23. Chen H, Nigg BM, de Koning J. Relationship between plantar pressure distribution under the foot and insole comfort. Clin Biomech. (1994) 9(6):335–41. doi: 10.1016/0268-0033(94)90062-0
24. Hennig EM, Valiant GA, Liu Q. Biomechanical variables and the perception of cushioning for running in Various types of footwear. J Appl Biomech. (1996) 12(2):143–50. doi: 10.1123/jab.12.2.143
25. Osborne-Crowley K. Social cognition in the real world: reconnecting the study of social cognition with social reality. Rev Gen Psychol. (2020) 24(2):144–58. doi: 10.1177/1089268020906483
26. Parsons TD. Virtual reality for enhanced ecological validity and experimental control in the clinical, affective and social neurosciences. Front Hum Neurosci. (2015) 9:660. doi: 10.3389/fnhum.2015.00660
27. Nigg BM, De Boer RW, Fisher V. A kinematic comparison of overground and treadmill running. Med Sci Sports Exerc. (1995) 27(1):98–105. doi: 10.1249/00005768-199501000-00018
28. Chambon N, Delattre N, Guéguen N, Berton E, Rao G. Shoe drop has opposite influence on running pattern when running overground or on a treadmill. Eur J Appl Physiol. (2015) 115(5):911–8. doi: 10.1007/s00421-014-3072-x
29. Hettiarachchi IT, Hanoun S, Nahavandi D, Nahavandi S. Validation of polar OH1 optical heart rate sensor for moderate and high intensity physical activities. PLoS One. (2019) 14(5):e0217288. doi: 10.1371/journal.pone.0217288
30. Miller JE, Nigg BM, Liu W, Stefanyshyn DJ, Nurse MA. Influence of foot, leg and shoe characteristics on subjective comfort. Foot Ankle Int. (2000) 21(9):759–67. doi: 10.1177/107110070002100908
31. Bailey GP, Harle R. Assessment of foot kinematics during steady state running using a foot-mounted IMU. Procedia Eng. (2014) 72:32–7. doi: 10.1016/j.proeng.2014.06.009
32. Zrenner M, Gradl S, Jensen U, Ullrich M, Eskofier BM. Comparison of different algorithms for calculating velocity and stride length in running using inertial measurement units. Sensors (Basel). (2018) 18(12). Available at: https://www.ncbi.nlm.nih.gov/pmc/articles/PMC6308955/ doi: 10.3390/s18124194
33. Honert EC, Nigg SR, Nigg BM, Blades S. Validation of running speed across different speeds and slopes using Kinetyx sensory insoles. Kinetyx. 2022. Available at: https://www.kinetyx.tech/white-paper-002-running-speed
34. Skog I, Handel P, Nilsson J, Rantakokko J. Zero-Velocity detection—an algorithm evaluation. IEEE Trans Biomed Eng. (2010) 57(11):2657–66. doi: 10.1109/TBME.2010.2060723
35. Bates D, Mächler M, Bolker B, Walker S. Fitting linear mixed-effects models using lme4. J Stat Soft. (2015) 67(1):1–48. Available at: http://www.jstatsoft.org/v67/i01/ doi: 10.18637/jss.v067.i01
36. Lenth RV, Buerkner P, Herve M, Jung M, Love J, Miguez F, et al. emmeans: Estimated Marginal Means, aka Least-Squares Means. 2022. Available at: https://CRAN.R-project.org/package=emmeans
37. Wilkinson RD, Mazzo MR, Feeney DF. Rethinking the statistical analysis of neuromechanical data. Exerc Sport Sci Rev. (2023) 51(1):43–50. doi: 10.1249/JES.0000000000000308
38. Hamill J, Hercksen J, Salzano M, Udofa A, Trudeau MB. The prevalence of injuries in trail running: influence of trails, terrains and footwear. Footwear Sci. (2022) 14(2):113–21. doi: 10.1080/19424280.2022.2048901
39. Wade FE, Mok KM, Fong DTP. Kinematic analysis of a televised medial ankle sprain. Asia-pacific journal of sports medicine, arthroscopy. Rehabil Technol. (2018) 12:12–6. doi: 10.1016/j.asmart.2018.02.001
40. Kristianslund E, Bahr R, Krosshaug T. Kinematics and kinetics of an accidental lateral ankle sprain. J Biomech. (2011) 44(14):2576–8. doi: 10.1016/j.jbiomech.2011.07.014
41. Miller JD, Miller AJ, Frazer LL, Templin TN, Eliason TD, Berkland CJ. A soft 3-dimensional force sensor for in-shoe ground reaction force measurement. In Ottawa. CAN. North American Congress of Biomechanics (NACOB) (2022). https://nacob.org/wp-content/uploads/2022/08/WednesdayThursday.pdf
42. Clarke TE, Frederick EC, Cooper LB. Effects of shoe cushioning upon ground reaction forces in running. Int J Sports Med. (1983) 04(4):247–51. doi: 10.1055/s-2008-1026043
43. Hoitz F, Mohr M, Asmussen M, Lam WK, Nigg S, Nigg B. The effects of systematically altered footwear features on biomechanics, injury, performance, and preference in runners of different skill level: a systematic review. Footwear Sci. (2020) 0(0):1–23. doi: 10.1080/19424280.2020.1773936
44. Honert EC, Ostermair F, von Tscharner V, Nigg BM. Changes in ankle work, foot work, and tibialis anterior activation throughout a long run. J Sport Health Sci. (2022) 11(3):330–8. doi: 10.1016/j.jshs.2021.02.003
Keywords: wearable sensors, IMU, plantar pressure, footwear upper, footwear features
Citation: Honert EC, Harrison K and Feeney D (2023) Evaluating footwear “in the wild”: Examining wrap and lace trail shoe closures during trail running. Front. Sports Act. Living 4:1076609. doi: 10.3389/fspor.2022.1076609
Received: 21 October 2022; Accepted: 14 December 2022;
Published: 6 January 2023.
Edited by:
Athanassios Bissas, University of Gloucestershire, United Kingdom© 2023 Honert, Harrison and Feeney. This is an open-access article distributed under the terms of the Creative Commons Attribution License (CC BY). The use, distribution or reproduction in other forums is permitted, provided the original author(s) and the copyright owner(s) are credited and that the original publication in this journal is cited, in accordance with accepted academic practice. No use, distribution or reproduction is permitted which does not comply with these terms.
*Correspondence: Eric C. Honert ZXJpYy5ob25lcnRAYm9hdGVjaG5vbG9neS5jb20=
Specialty Section: This article was submitted to Biomechanics and Control of Human Movement, a section of the journal Frontiers in Sports and Active Living