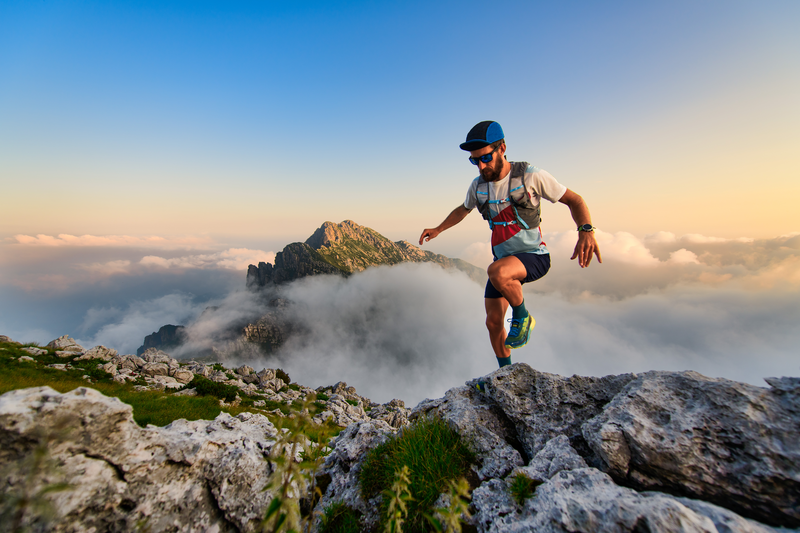
94% of researchers rate our articles as excellent or good
Learn more about the work of our research integrity team to safeguard the quality of each article we publish.
Find out more
BRIEF RESEARCH REPORT article
Front. Sports Act. Living , 16 November 2022
Sec. Sport and Exercise Nutrition
Volume 4 - 2022 | https://doi.org/10.3389/fspor.2022.1033842
This article is part of the Research Topic Pre-workout Nutrition View all 8 articles
Background: Limited research is available on the potential impact of creatine monohydrate administration before or after workouts among athletes. This study aimed to investigate the effects of pre- vs. post-exercise creatine monohydrate supplementation on resistance training adaptations and body composition.
Methods: In a randomized, double-blind, placebo-controlled, parallel design, 34 healthy resistance-trained male and female athletes were randomly assigned and matched according to fat free mass to consume a placebo, or 5-g dose of creatine monohydrate within 1 h before training, or within 1 h after training for 8 weeks, while completing a weekly resistance training program. Participants co-ingested 25-gram doses of both whey protein isolate and maltodextrin along with each assigned supplement dose. Body composition, muscular strength, and endurance, along with isometric mid-thigh pull were assessed before and after the 8-week supplementation period. A 3 × 2 mixed factorial (group x time) ANOVA with repeated measures on time were used to evaluate differences.
Results: All groups experienced similar and statistically significant increases in fat free mass (+1.34 ± 3.48 kg, p = 0.04), upper (+2.21 ± 5.69 kg, p = 0.04) and lower body strength (+7.32 ± 10.01 kg, p < 0.001), and decreases in body mass (−1.09 ± 2.71 kg, p = 0.03), fat mass (−2.64 ± 4.16 kg, p = 0.001), and percent body fat (−2.85 ± 4.39 kg, p < 0.001).
Conclusions: The timing of creatine monohydrate did not exert any additional influence over the measured outcomes.
Ergogenic aids are commonly used to improve performance or augment exercise training adaptations (1, 2). Creatine monohydrate (1, 3–6) supplementation is well-established for its ability to increase intramuscular creatine concentration and its subsequent ergogenic potential as seen by commonly reported increases in fat-free mass accretion, augmented muscle morphology changes, and improvements in muscular strength, endurance, and power (3, 7, 8). Altering the timing of when nutrients are delivered has been established as a potential strategy to augment recovery from stressful exercise while also impacting adaptations to regular exercise training (9). While the majority of the research regarding the effects of nutrient timing strategies has centered upon the delivery of macronutrients (9), interest exists to better understand how the timing of other micronutrients or single ingredients, such as creatine monohydrate, may affect recovery and exercise training adaptations (10). For example, early work (11) demonstrated that ingesting a combination of carbohydrate + protein + creatine (Cr) in close proximity (vs. morning and evening ingestion) to each workout over 10 weeks, elicited greater increases in intramuscular creatine, lean tissue accretion, cross-sectional area of type II muscle fibers, and exercise performance were observed. While this study highlighted the potential advantage of ingesting creatine in close proximity to a workout, additional questions remain whether or not ingesting creatine monohydrate in isolation, before or after exercise sessions, offers any advantages. In 2013, Antonio and Ciccone (12) conducted a 4-week resistance training and concluded that creatine ingestion (5 grams) post-exercise led to greater improvements in fat-free mass and strength when compared to ingesting an identical 5-gram dose before each workout. Conclusions from this study were limited, however, due to the short supplementation period, no placebo group, and the statistical approach employed by the investigators. Candow et al. later published two separate investigations in older adults spanning 12 weeks (13) and 8 months (14), respectively, which both reported no differential impact of creatine timing on resistance training adaptations. Similarly, a 2021 study by Forbes et al. (15) indicated that Cr timing did not alter improvements in muscle thickness and strength after 8 weeks of supplementation (2 days per week on training days only) while following a unilateral resistance training program. As it stands, more research is needed to better investigate the impact of creatine timing on exercise training adaptations in young, athletic populations over several weeks of exercise training and supplementation. Therefore, the aim of the current study was to evaluate the effects of 8 weeks of timed creatine supplementation on resistance training adaptations in college-aged male and female athletes. Based upon previous research which has highlighted an upregulation of anabolic signaling mechanisms (16) and hyperemia post-exercise (17), coupled with an increase an insulin sensitivity from the added carbohydrate (18), we hypothesize that post-exercise creatine consumption would lead to increased kinetics pertaining to creatine transport and result in greater improvements in strength and body composition throughout the study protocol.
In a double-blind, placebo-controlled, parallel design, study participants were randomized based upon fat-free mass into one of three groups to ingest creatine (before or after workouts) or a placebo daily throughout an 8-week supplementation period. Study participants were randomized into one of three supplement groups using block randomization, counter-balanced for sex, and matched for fat-free mass. Each group consumed their assigned dose or placebo within 1 h before exercise or within 1 h following exercise and were recommended to consume first thing in the morning and in the evening, after 5:00 p.m. hours on non-training days.
All participants completed a 12-week resistance training and conditioning program. To homogenize neurological responses to resistance training, a 4-week (no supplementation) run-in period was completed, prior to participants completing baseline testing and began the supplementation protocol, while continuing to follow the resistance training program for an additional 8 weeks. Before and after the supplementation and resistance training period, body mass, body water, and body composition were assessed in addition to changes in muscular strength, muscular endurance, and lower-body power (Figure 1). This study was retroactively registered on ClinicalTrials.gov as NCT05451498.
Thirty-four apparently healthy male (n = 18) and female (n = 16) collegiate athletes (mean ± SD; age = 19.8 ± 1.5 years; height = 175.1 ± 9.7 cm; body mass = 82.5 ± 21.9 kg; body mass index = 26.5 ± 4.8 kg/m2) from women's volleyball (n = 3), women's soccer (n = 13), and football (n = 18) volunteered to participate in this study. To be eligible, participants were required to be current members of the university's athletic program, participating in their prescribed off-season resistance training program, between the ages of 19–30, and medically cleared to participate in their respective sport. Exclusion criteria included, any individual who is currently being treated or is diagnosed with a cardiac, respiratory, circulatory, musculoskeletal, metabolic obesity (defined as body mass index >35 kg/m2 and body fat >30%), immune, autoimmune, psychiatric, hematological, neurological, or endocrinological disorder or disease, anabolic steroid use, allergies to milk or soy (lecithin), and are currently pregnant or become pregnant at any point throughout the study (females only). Further, participants were required to stop taking any ergogenic agents (pre-workouts, creatine, protein, beta-alanine, etc.) 4weeks prior to and throughout the entire study protocol.
A CONSORT diagram is provided in Figure 2 to outline the enrollment and group allocation process. The experimental protocol was approved by the Rocky Mountain University of Health Professions Institutional Review Board on 9 February 2022, with code 2021-28. All participants were informed of their obligations and risks associated with the study protocol and provided their written consent on an IRB-approved informed consent document prior to participation.
Before each visit, participants were instructed to fast overnight and abstain from exercise, caffeine, nicotine, and alcohol for at least 24 h. During the initial assessment, participant height was assessed to the nearest ± 0.5 cm using a stadiometer with their shoes removed and standing erect on flat feet. Body mass was measured prior to all study visits using a self-calibrating digital balance (Tanita BWB-627A, Tokyo, Japan) and was recorded to the nearest ± 0.1 kg.
A field-based three-compartment (3CFIELD) model was utilized to estimate body composition and determine fat mass (FM), fat-free mass (FFM), and total body water (TBW) by combining estimates of body density (BD) derived from skinfold measurements, and TBW using bioimpedance spectroscopy (BIS) (19–21). This combination of methods has been previously shown to provide more accurate body composition estimates than if used as individual techniques (20). Further, recent evidence suggests that minimal differences exist between a 3C and 4C model (19, 22).
To ensure adequate hydration, participants were instructed to follow a hydration protocol as hydration status was not assessed prior to testing. Total body water (TBW) was assessed using bioelectrical impedance spectroscopy (SFB7, Impedimed Corp., Carlsbad, CA) as previously described (23). Test-retest reliability using bioelectrical impedance spectroscopy is available for fat mass (CV: 5.86%, ICC: 0.98, SEM: 280.9 grams) and fat-free mass (CV: 1.72%, ICC: 0.99, SEM: 285.1 grams) in a cohort (n = 40) of healthy college-aged men and women (23).
Skinfold measurements were completed on the right side of the body by the same trained investigator according to the recommendations by Jackson and Pollock (24). A calibrated Lange caliper was used to take duplicate measurements at each of the seven sites (chest, mid-axilla, triceps, abdomen, suprailium, subscapular, and thigh) for both men and women and used to estimate body density using the Siri equation (25). Fat-free mass, fat mass, and body fat percentage then calculated using a 3C model (20) were recorded. Skinfold technician test-retest reliability in the current study was CV: 0.35%, ICC: 0.985 (95% CI: 0.976, 0.991), SEM: 1.37 millimeters.
A standardized warmup consisting of dynamic bodyweight movements including walking lunges, squats, and leg swings was completed prior to assessing maximal torque using an isometric mid-thigh pull (IMTP) as previously described (23). Study participants completed three, maximal-effort attempts with 60 s of rest allowed between each attempt. The highest value was recorded and used for later analysis. Isometric mid-thigh pull assessments have been shown to be strongly associated with athletic performance outcomes such as strength (26, 27), sprint performance (27, 28), and agility performance (27).
Maximal strength [one-repetition maximum (1 RM)] and muscular endurance [repetitions to fatigue (RTF) at 80% 1 RM] were assessed in the following sequence: (a) back squat strength, (b) back squat endurance, (c) bench press strength, and (d) bench press endurance. Approximately 5 min of rest separated each assessment. All protocols were consistent with the National Strength and Conditioning Association (NSCA) and previously described (27, 28).
As highlighted previously, all study participants completed 12 weeks of resistance training at a frequency of 4 days per week which was designed and supervised by the university strength and conditioning coach. The first 4 weeks of resistance training (16 workouts) commenced before the initiation of the supplementation period and baseline testing to acclimate participants and homogenize training responses to the program. Following the initial 4-week resistance training block, an additional 8 weeks of resistance training was completed (~32 workouts) that coincided with the supplementation protocol. Each workout consisted of mostly multi-joint exercises with free weights that targeted all major muscle groups [i.e., rack/hang cleans, bench press (flat and incline), push press, rear deltoid rows/pull apart, pendlay rows, low rows, pulldowns, triceps extensions, bicep curls, back and belt squats, front and side planks, glute/ham raises, and box/seated jumps]. A progressive overload scheme was followed to facilitate increases in strength and lean body mass. The program was divided into three phases: phase 1 (< 60–70% 1 RM) for weeks 1–4, phase 2 (70–80% 1 RM) for weeks 4–8, and phase 3 (80–95% 1 RM) for weeks 8–12. Attendance was required daily and compliance was calculated as the percentage of completed workouts. Participants were deemed compliant if they completed at least 90% of the assigned workouts throughout the study protocol.
Participants were requested to submit a three-day dietary intake log two times throughout the study (weeks 0 and 8). Study participants used MyFitnessPal (Under Armor, Baltimore, MD) to create individualized profiles and self-report energy and macronutrient intake. The 3-day average was computed for later analysis, however, compliance to completion of the dietary records was poor with only five of the 34 athletes completing all requested days.
Two doses of the assigned supplement (or placebo) were ingested each day by all study participants for 8 weeks. In a double-blind manner, the PRE group was instructed to ingest a 5-gram dose of creatine before exercise and a 5-gram dose of placebo (maltodextrin) after exercise. The POST group ingested a 5-gram dose of placebo (maltodextrin) before exercise and ingested a 5-gram dose of creatine after exercise. The PLA group ingested a 5-gram dose of placebo (maltodextrin) before exercise and a 5-gram dose of placebo (maltodextrin) after exercise. All doses were ingested 1 h before exercise and within 1 h after exercise. On non-training days, participants ingested the assigned supplement dose first thing in the morning (immediately upon waking) and their other assigned dose after 5:00 p.m. Participants consumed each assigned supplement along with a 25-gram dose of whey protein isolate and a 25-gram dose of carbohydrate powder. The carbohydrate and protein were added to aid in blinding, promote compliance, and facilitate optimal exercise training adaptations throughout the study protocol. Compliance to the supplementation regimen was not monitored as the principal investigator and the head strength and conditioning coach were able to communicate daily with participants regarding their compliance to the study protocol.
All supplements were provided to participants in powder form and were of similar texture, bitterness, appearance, and sweetness. All supplements were weighed and blinded by research personnel not involved in testing. The whey protein isolate and maltodextrin were provided by Argopur Dairy Cooperative (La Crosse, WI) and the creatine was provided by 1st Phorm, LLC (St. Louis, MO).
The occurrence of adverse events was collected through spontaneous reporting by the study participants or the interaction of the principal investigator with study participants. When participants reported adverse events, they reported the frequency as well as the severity (“mild”, “moderate”, “severe”) of any adverse event.
All analyses were completed using Microsoft Excel and the Statistical Package for the Social Sciences (v23; SPSS Inc., Chicago IL). Data were considered statistically significant when the probability of a type I error was 0.05 or less. Primary endpoints for this investigation were changes in fat-free mass and back squat 1 RM. Secondary endpoints were fat mass, % fat, total body water, bench press 1 RM, bench press repetitions to fatigue, and back squat repetitions to fatigue. A 3 × 2 mixed factorial (group × time) ANOVA with repeated measures on time were used to determine any statistically significant differences for time and group main effects and group × time interaction effects. All data are presented as means ± standard deviations and the presence of any statistical outlier was evaluated by Grubbs' test and removed from analysis. Reliability statistics were determined using intraclass correlation and agreement between Pearson correlations.
Of the 47 participants who were randomized and enrolled in the study, eight (PRE = 2, POST = 4, PLA = 2) withdrew due to personal reasons unrelated to the study, and one participant was non-compliant with the protocol (PLA = 1). An additional four participants (PRE = 2, PLA = 2) withdrew due to undesired side effects relating to weight gain that were possibly related to supplementation. In total, 34 participants completed the study and were included in the final analysis (PRE = 12; POST = 11; PLA = 11). Final compliance with recording their workout information was 90.4% for PRE-group, 94.5% for POST-group, and 93.3% for PLA-group.
Throughout the study, 17 adverse events were reported (13 were abdominal symptoms, three were nausea, and one was due to periodic headaches). Of these, n = 9 were mild, n = 7 were moderate, n = 1 severe. The severe adverse event (POST = 1) was related to dizziness but was determined to not be related to the study. All adverse events were resolved by the end of the first 2 weeks. One participant who reported an adverse event (PLA = 1) withdrew due to reasons directly related to supplementation.
One-way ANOVA on pre-supplementation data indicated no baseline differences (p > 0.05) between groups for age (p = 0.21), height (p = 0.36), body mass (p = 0.69), body mass index (p = 0.76), % body fat (p = 0.26), fat-free mass (p = 0.73), back squat 1RM (p = 0.69), bench press 1 RM (p = 0.63), and IMTP peak force (p = 0.55).
Dietary data were collected during the first (PRE = 4; POST = 6, PLA = 4) and final (PRE = 3; POST = 2; PLA = 1) week of supplementation. Using a One-way ANOVA, no differences in dietary intake (i.e., total energy, carbohydrate, fat, and protein; Supplementary Table 1) were observed at baseline between groups for energy and macronutrient intake. Due to limited post-protocol data, paired-samples t-test were utilized to determine changes from baseline. No differences were observed between data points across time (p > 0.05). Similar outcomes were revealed when all data were represented relative to body mass (Supplementary Table 1).
Supplementary Table 2 presents body mass, total body water, intracellular water (ICW), extracellular water (ECF), and body composition changes. No significant (p > 0.05) group × time interaction effects were observed for body mass, body mass index, body water compartments, or body composition parameters. Main effects for time were observed for body mass (p = 0.03, ηp2 = 0.15), body mass index (p = 0.03, ηp2 = 0.15), total body water (p = 0.02, ηp2 = 0.18) and intracellular water (p = 0.006, ηp2 = 0.22).
No significant group × time interaction effects were observed for fat-free mass (p = 0.11), fat mass (p = 0.33), and % body fat (p = 0.38). Main effects for time were observed for fat free mass (Figure 3) (p = 0.02, ηp2 = 0.17), % fat (p < 0.001, ηp2 = 0.35), and fat mass (p < 0.001, ηp2 = 0.33) (Supplementary Table 2).
Figure 3. Change in fat-free mass (Week 8 - Week 0) in kilograms. Cr Post, Post-exercise creatine supplementation; Cr Pre, Pre-exercise creatine supplementation; PLA, Placebo supplementation. For each respective shape, the filled shapes (■▴•) are female participants, and the non-filled shapes (△°□) are male participants.
As seen in Supplementary Table 3, no significant (p > 0.05) group x time interaction effects were observed for any back squat or bench press performance parameters. Significant main effects for time were observed for back squat 1RM (p < 0.001, ηp2 = 0.35), back squat 1RM normalized to body mass (p < 0.001, ηp2 = 0.45), bench press 1RM (p = 0.04, ηp2 = 0.13), and bench press 1RM normalized to body mass (p < 0.001, ηp2 = 0.32), while no significant (p > 0.05) changes over time were observed for any other performance parameter (Supplementary Table 3). Finally, total resistance training load-volume (sets × reps × load) was calculated for the entire 8-week protocol. No significant differences between groups were found for total load-volume (PRE: 58,505 ± 35,887 kg vs. POST: 88,873 ± 58,672 kg vs. PLA: 70,408 ± 43,928 kg, p = 0.32, ηp2 = 0.07).
The purpose of this investigation was to examine the effects of timed creatine monohydrate supplementation (pre- vs. post-exercise) on resistance training adaptations in college-aged male and female athletes. Results from this investigation indicated that timing of creatine monohydrate exerted no further change in key outcomes such as fat-free mass (p = 0.11, Figure 3) and back squat 1RM (p = 0.87, Figure 4) in addition to other performance and body composition variables (see Supplementary Tables 2, 3). Beyond this observation, we also reported significant improvements in several body composition and performance variables, which provide sound evidence that the training program and supplementation provided throughout this study instigated changes in all study participants from baseline. Overall, the key findings from the present investigation indicate that the ingestion, nor the timing, of creatine monohydrate in combination with carbohydrate and whey protein did not exert any influence on performance or body composition outcomes in healthy, college-aged male and female athletes following 8-weeks of resistance training.
Figure 4. Change in squat 1RM performance (Week 8 - Week 0) in kilograms. Cr Post, Post-exercise creatine supplementation; Cr Pre, Pre-exercise creatine supplementation; PLA, Placebo supplementation. For each respective shape, the filled shapes (■▴•) are female participants, and the non-filled shapes (△°□) are male participants.
The primary rationale for this investigation was based upon the combined tenets of creatine supplementation (3, 29) and nutrient timing (9). In this respect, previous research has established the efficacy of combining creatine supplementation with resistance training (3, 7, 8, 30, 31) for its ability to augment adaptations over time, while several investigations and reviews have highlighted the potential impact of nutrient timing (9–11, 32–34). Currently, a small number of original investigations have explored the potential efficacy of timed creatine monohydrate administration, displaying mixed outcomes. In this respect, a recent review paper that discussed creatine timing (35) included six studies, with only two studies providing some level of evidence for heightened adaptations in regards to the manipulation of when creatine was ingested, in favor of post-exercise Cr supplementation (as compared to pre-exercise) (12, 14). From these studies, one should consider that the Candow study (14) was completed in elderly individuals, which limits its generalizability to younger, athletic populations and the primary population upon which the efficacy of creatine supplementation has been built. Moreover, the Antonio study (12), while completed in healthy active men, lasted 4 weeks in duration, had no placebo group, employed a liquid creatine formulation with no loading phase, did not assess body composition or lower-body performance changes, and used magnitude-based inferences; a statistical approach that has been challenged by some scientific journals and statisticians (36–38). Outside of these two investigations, the remaining studies have failed to identify any differences in resistance training adaptations between pre- vs. post-exercise consumption of creatine monohydrate. Therein, the results from the present investigation are in agreement with those studies which did not identify any added benefit of ingesting a daily dose of creatine monohydrate either before or after resistance exercise and therefore does not support our hypothesis. It is possible that the post-exercise hyperemia (17) and purported enhancement of creatine transport via increased insulin sensitivity (18) was not sufficient to elicit meaningful differences in measured outcomes over time; however, more work is needed to identify the specific mechanism of action.
Results from the present investigation also indicate that a daily dose of creatine monohydrate did not exert any additional benefit for improvements in body composition or performance outcomes, when compared to the placebo group; in the presence of provisional protein and carbohydrates. While somewhat unexpected due to the widespread literature supporting the benefits of creatine, the exclusion of a loading phase in our study as well as the co-ingestion of carbohydrate and protein on two occasions each day, may have limited the ability to discern differences between groups over the time frame within which our study was completed. In this respect, a loading phase is commonly employed with creatine monohydrate supplementation studies, with the goal of robustly increasing intramuscular phosphocreatine levels by 20–40% in a 5–7-day time period (39). We chose not to employ a loading phase due to the challenges associated with how to execute a loading phase, while also maintaining the blinding and timing intervention that was central to this project's aim. Previous work by Hultman et al. (39) has shown that after ~28 days of single 5-gram doses of creatine intramuscular creatine is significantly increased, which, in the present study, should have allowed for an additional 4 weeks to examine if the timing of creatine was relevant. Another key consideration was that we decided to provide two daily doses of 25 grams of maltodextrin carbohydrate and 25 grams of whey protein isolate, which provided an additional 50 grams of carbohydrate and 50 grams of whey protein isolate per day to all study participants. This decision was made for three primary reasons. First, to help ensure each participant was provided with an efficacious dose of essential amino acids and energy to promote an anabolic environment throughout the study protocol. Previous research has demonstrated that essential amino acids (EAA), in optimal dosages, maximally stimulates rates of muscle protein synthesis, particularly when ingested in close proximity to a resistance-training bout, and also that the presence of CHO may further enhance this response (8, 40, 41). Moreover, when creatine is added to whey protein, studies have indicated that a greater improvement in lean mass may occur when compared to whey protein or CHO alone (42). Thus, it is possible that any additional ergogenic potential derived from creatine administration was clouded by co-ingestion of protein and carbohydrates and the absence of a loading phase. Certainly, one could point to the findings of Cribb and Hayes (11) to refute our suggestion that added carbohydrate and protein clouded our ability to identify creatine-mediated changes, but the dosing regimen provided by Cribb and Hayes also delivered over two times the amount of creatine each day as what was delivered in the present study, which likely maximized intramuscular creatine much quicker than the dosing regimen utilized in the current study. The inclusion of a true control group in the Cribb study could have helped to further explore this possibility. The second reason for co-ingestion of carbohydrates and protein was to aid in blinding the administration of either creatine monohydrate or the placebo to our study participants. The third and final reason was to improve recruitment efforts, whereby all participants were minimally provided two daily doses of carbohydrates and protein to help maximize the potential for augmented training outcomes as no other compensation was provided.
The overall training outcomes realized by the resistance training program from the current study were largely consistent with other commonly reported training adaptations following off-season strength and conditioning programs in the literature (43–47). In this regard, main effects over time were observed, which illustrated improvements in upper- (2.8%) and lower-body strength (7.3%), as well as positive adaptations to several body composition outcomes including fat-free mass, fat mass, percent fat, and total body water. The decrease in body mass was somewhat unexpected, but the positive changes in fat-free mass, fat mass, and percent body fat do align with the observed changes in body mass.
Several strengths are evident from the present study starting with the randomized, double-blind, placebo-controlled approach, with more study participants per group than what has been previously reported in the literature (12, 13, 15). Another key strength was the 4-week period of resistance training that occurred in all supplementation groups prior to initiation of the supplementation protocol. This decision was made due to the variety of ages and genders of participants in the present study. While it is acknowledged that 4 weeks of training does not replace the neuromuscular adaptation observed with more advanced training ages, the younger training ages of some of the study participants did likely benefit from this period of resistance training. Certainly, our study was not without limitations. Most notably was our extremely poor compliance to recording of dietary intake. As mentioned previously in the paper and despite repeated reminders and efforts by the research team to complete food records, we are left with very limited data to quality dietary intake throughout the study. While we are encouraged by the significant main effects of time observed for fat-free mass accretion in all groups (Figure 3), we are not able to communicate how the quality of the diet consumed did or did not further support these observed changes. Thus, the reader is strongly encouraged to consider this when evaluating our findings and conclusions. Future research should seek ways to maximize dietary intake reporting by their study cohorts. The lack of dual x-ray absorptiometry (DEXA) to assess body composition would have provided a more robust body composition measure [i.e., four compartment (4C) model], although some have argued there is minimal difference between a 3C (used in the present study) and 4C model (19, 22). The low compliance regarding dietary intake logs was another shortcoming of the current study. Additionally, no measure of hydration status was taken, although participants were strongly encouraged to follow a hydration protocol before testing sessions. Lastly, no measures of initial creatine levels, muscle fiber morphology, blood flow kinetics, muscle cross-sectional area, myogenic transcription factors, or hormonal properties were measured in the current study as one or all of these measures would have helped to mechanistically explain some of our findings.
In conclusion, the current investigation examined the impact of 8 weeks of timed creatine monohydrate supplementation (pre-exercise vs. post-exercise) on resistance training adaptations in college-aged male and female athletes. It was revealed that the timing of creatine monohydrate in combination with carbohydrate and whey protein did not exert any differential effects for performance or body composition outcomes in healthy, college-aged men and women.
The raw data supporting the conclusions of this article will be made available by the authors, without undue reservation.
This study involving human participants was reviewed and approved by Rocky Mountain University of Health Professions Institutional Review Board. The participants provided their written informed consent to participate in this study.
ND, AJ, MM, and CK designed study. ND, AH, AJ, and CK did data collection. ND and CK analyzed data and prepared initial draft. All authors approved final version.
This research was partially supported by a research grant from Rocky Mountain University of Health Professions and internal funding provided by the Exercise and Performance Nutrition Laboratory at Lindenwood University.
The authors would like to thank the study participants for their commitment to this study protocol and the blinded research team in the Exercise and Performance Nutrition Lab at Lindenwood University for their assistance with packaging, labeling, and blinding of the supplements. The authors are particularly appreciative of the generosity shown by Agropur Dairy Cooperative (La Crosse, WI, www.agropur.com) for their donation of the whey protein isolate and maltodextrin in addition to commercially blending, packaging, and shipping the supplements. In particular, the authors would like to publicly acknowledge and thank Aaron Martin of Agropur for his cooperation and excitement toward this project. Finally, a special thanks are also due to Coach Aaron Bozarth (Midland University) for the implementation of the resistance training program and his cooperation throughout the project.
The authors declare that the research was conducted in the absence of any commercial or financial relationships that could be construed as a potential conflict of interest.
All claims expressed in this article are solely those of the authors and do not necessarily represent those of their affiliated organizations, or those of the publisher, the editors and the reviewers. Any product that may be evaluated in this article, or claim that may be made by its manufacturer, is not guaranteed or endorsed by the publisher.
The Supplementary Material for this article can be found online at: https://www.frontiersin.org/articles/10.3389/fspor.2022.1033842/full#supplementary-material
1. Kerksick CM, Wilborn CD, Roberts MD, Smith-Ryan A, Kleiner SM, Jäger R, et al. ISSN exercise & sports nutrition review update: research & recommendations. J Int Soc Sports Nutr. (2018) 15:38. doi: 10.1186/s12970-018-0242-y
2. Williams MH. Facts and fallacies of purported ergogenic amino acid supplements. Clin Sports Med. (1999) 18:633–49. doi: 10.1016/S0278-5919(05)70173-3
3. Kreider RB, Kalman DS, Antonio J, Ziegenfuss TN, Wildman R, Collins R, et al. International society of sports nutrition position stand: safety and efficacy of creatine supplementation in exercise, sport, and medicine. J Int Soc Sports Nutr. (2017) 14:18. doi: 10.1186/s12970-017-0173-z
4. Maughan RJ, Burke LM, Dvorak J, Larson-Meyer DE, Peeling P, Phillips SM, et al. IOC consensus statement: dietary supplements and the high-performance athlete. Int J Sport Nutr Exerc Metab. (2018) 28:104–25. doi: 10.1123/ijsnem.2018-0020
5. Antonio J, Candow DG, Forbes SC, Gualano B, Jagim AR, Kreider RB, et al. Common questions and misconceptions about creatine supplementation: what does the scientific evidence really show? J Int Soc Sports Nutr. (2021) 18:1–17. doi: 10.1186/s12970-021-00412-w
6. Fazio C, Elder CL, and Harris MM. Efficacy of alternative forms of creatine supplementation on improving performance and body composition in healthy subjects: a systematic review. J Strength Condit Res. (2022) 36:2663–70. doi: 10.1519/JSC.0000000000003873
7. Kreider RB. Effects of creatine supplementation on performance and training adaptations. Mol Cell Biochem. (2003) 244:89–94. doi: 10.1007/978-1-4615-0247-0_13
8. Volek JS, Kraemer WJ, Bush JA, Boetes M, Incledon T, Clark KL, et al. Creatine supplementation enhances muscular performance during high-intensity resistance exercise. J Am Diet Assoc. (1997) 97:765–70. doi: 10.1016/S0002-8223(97)00189-2
9. Kerksick CM, Arent S, Schoenfeld BJ, Stout JR, Campbell B, Wilborn CD, et al. International society of sports nutrition position stand: nutrient timing. J Int Soc Sports Nutr. (2017) 14:33. doi: 10.1186/s12970-017-0189-4
10. Stecker RA, Harty PS, Jagim AR, Candow DG, and Kerksick CM. Timing of ergogenic aids and micronutrients on muscle and exercise performance. J Int Soc Sports Nutr. (2019) 16:37. doi: 10.1186/s12970-019-0304-9
11. Cribb PJ, and Hayes A. Effects of supplement timing and resistance exercise on skeletal muscle hypertrophy. Med Sci Sports Exerc. (2006) 38:1918–25. doi: 10.1249/01.mss.0000233790.08788.3e
12. Antonio J, and Ciccone V. The effects of pre versus post workout supplementation of creatine monohydrate on body composition and strength. J Int Soc Sports Nutr. (2013) 10:36. doi: 10.1186/1550-2783-10-36
13. Candow DG, Zello GA, Ling B, Farthing JP, Chilibeck PD, McLeod K, et al. Comparison of creatine supplementation before versus after supervised resistance training in healthy older adults. Res Sports Med. (2014) 22:61–74. doi: 10.1080/15438627.2013.852088
14. Candow DG, Vogt E, Johannsmeyer S, Forbes SC, and Farthing JP. Strategic creatine supplementation and resistance training in healthy older adults. Appl Physiol Nutr Metab. (2015) 40:689–94. doi: 10.1139/apnm-2014-0498
15. Forbes SC, Krentz JR, and Candow DG. Timing of creatine supplementation does not influence gains in unilateral muscle hypertrophy or strength from resistance training in young adults: a within-subject design. J Sports Med Phys Fitness. (2021) 61:1219–25. doi: 10.23736/S0022-4707.20.11668-2
16. Willoughby DS, Stout JR, and Wilborn CD. Effects of resistance training and protein plus amino acid supplementation on muscle anabolism, mass, and strength. Amino Acids. (2007) 32:467–77. doi: 10.1007/s00726-006-0398-7
17. Collier SR, Diggle MD, Heffernan KS, Kelly EE, Tobin MM, and Fernhall B. Changes in arterial distensibility and flow-mediated dilation after acute resistance vs. aerobic exercise. J Strength Cond Res. (2010) 24:2846–52. doi: 10.1519/JSC.0b013e3181e840e0
18. Burke LM, van Loon LJC, and Hawley JA. Postexercise muscle glycogen resynthesis in humans. J Appl Physiol. (2017) 122:1055–67. doi: 10.1152/japplphysiol.00860.2016
19. Nickerson BS, Tinsley GM, and Esco MR. Validity of field and laboratory three-compartment models in healthy adults. Med Sci Sports Exerc. (2019) 51:1032–9. doi: 10.1249/MSS.0000000000001876
20. Esco MR, Nickerson BS, Fedewa MV, Moon JR, and Snarr RL. A novel method of utilizing skinfolds and bioimpedance for determining body fat percentage via a field-based three-compartment model. Eur J Clin Nutr. (2018) 72:1431–8. doi: 10.1038/s41430-017-0060-3
21. Forslund AH, Johansson AG, Sjödin A, Bryding G, Ljunghall S, and Hambraeus L. Evaluation of modified multicompartment models to calculate body composition in healthy males. Am J Clin Nutr. (1996) 63:856–62. doi: 10.1093/ajcn/63.6.856
22. Nickerson BS, and Tinsley GM. Utilization of BIA-derived bone mineral estimates exerts minimal impact on body fat estimates via multicompartment models in physically active adults. J Clin Densitom. (2018) 21:541–9. doi: 10.1016/j.jocd.2018.02.003
23. Moon JM, Ratliff KM, Blumkaitis JC, Harty PS, Zabriskie HA, Stecker RA, et al. Effects of daily 24-gram doses of rice or whey protein on resistance training adaptations in trained males. J Int Soc Sports Nutr. (2020) 17:60. doi: 10.1186/s12970-020-00394-1
24. Jackson AS, and Pollock ML. Practical assessment of body composition. Phys Sportsmed. (1985) 13:76–90. doi: 10.1080/00913847.1985.11708790
25. Siri WE. Body composition from fluid spaces and density: analysis of methods. 1956. Nutrition. (1993) 5:480–92.
26. Beckham G, Mizuguchi S, Carter C, Sato K, Ramsey M, Lamont H, et al. Relationships of isometric mid-thigh pull variables to weightlifting performance. J Sports Med Phys Fitness. (2013) 53:573–81.
27. Wang R, Hoffman JR, Tanigawa S, Miramonti AA, La Monica MB, Beyer KS, et al. Isometric mid-thigh pull correlates with strength, sprint, and agility performance in collegiate rugby union players. J Strength Cond Res. (2016) 30:3051–6. doi: 10.1519/JSC.0000000000001416
28. Townsend JR, Bender D, Vantrease WC, Hudy J, Huet K, Williamson C, et al. Isometric midthigh pull performance is associated with athletic performance and sprinting kinetics in division I men and women's basketball players. J Strength Cond Res. (2019) 33:2665–73. doi: 10.1519/JSC.0000000000002165
29. Wax B, Kerksick CM, Jagim AR, Mayo JJ, Lyons BC, and Kreider RB. Creatine for exercise and sports performance, with recovery considerations for healthy populations. Nutrients. (2021) 13:1915. doi: 10.3390/nu13061915
30. Forbes SC, Little JP, and Candow DG. Exercise and nutritional interventions for improving aging muscle health. Endocrine. (2012) 42:29–38. doi: 10.1007/s12020-012-9676-1
31. Candow DG, Chilibeck PD, and Forbes SC. Creatine supplementation and aging musculoskeletal health. Endocrine. (2014) 45:354–61. doi: 10.1007/s12020-013-0070-4
32. Schoenfeld BJ, and Aragon AA. Is there a postworkout anabolic window of opportunity for nutrient consumption? Clearing up controversies. J Orthop Sports Phys Ther. (2018) 48:911–4. doi: 10.2519/jospt.2018.0615
33. Esmarck B, Andersen JL, Olsen S, Richter EA, Mizuno M, and Kjaer M. Timing of postexercise protein intake is important for muscle hypertrophy with resistance training in elderly humans. J Physiol. (2001) 535 (Pt 1):301–11. doi: 10.1111/j.1469-7793.2001.00301.x
34. Arent SM, Cintineo HP, McFadden BA, Chandler AJ, and Arent MA. Nutrient timing: a garage door of opportunity? Nutrients. (2020) 12:1948. doi: 10.3390/nu12071948
35. Candow DG, Forbes SC, Roberts MD, Roy BD, Antonio J, Smith-Ryan AE, et al. Creatine o'clock: does timing of ingestion really influence muscle mass and performance? Front Sports Act Living. (2022) 4:893714. doi: 10.3389/fspor.2022.893714
36. Sainani KL. The problem with “magnitude-based inference.” Med Sci Sports Exerc. (2018) 50:2166–76. doi: 10.1249/MSS.0000000000001645
37. Sainani KL, Lohse KR, Jones PR, and Vickers A. Magnitude-based inference is not bayesian and is not a valid method of inference. Scand J Med Sci Sports. (2019) 29:1428–36. doi: 10.1111/sms.13491
38. Butson ML. Will the numbers really love you back: Re-examining magnitude-based inference. SportRxiv [Preprint]. (2017). doi: 10.31236/osf.io/e3vs6
39. Hultman E, Söderlund K, Timmons JA, Cederblad G, and Greenhaff PL. Muscle creatine loading in men. J Appl Physiol. (1996) 81:232–7. doi: 10.1152/jappl.1996.81.1.232
40. Tipton KD, Borsheim E, Wolf SE, Sanford AP, and Wolfe RR. Acute response of net muscle protein balance reflects 24-h balance after exercise and amino acid ingestion. Am J Physiol Endocrinol Metab. (2003) 284:E76–89. doi: 10.1152/ajpendo.00234.2002
41. Miller SL, Tipton KD, Chinkes DL, Wolf SE, and Wolfe RR. Independent and combined effects of amino acids and glucose after resistance exercise. Med Sci Sports Exerc. (2003) 35:449–55. doi: 10.1249/01.MSS.0000053910.63105.45
42. Burke DG, Chilibeck PD, Davidson KS, Candow DG, Farthing J, and Smith-Palmer T. The effect of whey protein supplementation with and without creatine monohydrate combined with resistance training on lean tissue mass and muscle strength. Int J Sport Nutr Exerc Metab. (2001) 11:349–64. doi: 10.1123/ijsnem.11.3.349
43. Lanhers C, Pereira B, Naughton G, Trousselard M, Lesage FX, and Dutheil F. Creatine supplementation and lower limb strength performance: a systematic review and meta-analyses. Sports Med. (2015) 45:1285–94. doi: 10.1007/s40279-015-0337-4
44. Lanhers C, Pereira B, Naughton G, Trousselard M, Lesage FX, and Dutheil F. Creatine supplementation and upper limb strength performance: a systematic review and meta-analysis. Sports Med. (2017) 47:163–73. doi: 10.1007/s40279-016-0571-4
45. Chilibeck PD, Kaviani M, Candow DG, and Zello GA. Effect of creatine supplementation during resistance training on lean tissue mass and muscular strength in older adults: a meta-analysis. Open Access J Sports Med. (2017) 8:213–26. doi: 10.2147/OAJSM.S123529
46. Branch JD. Effect of creatine supplementation on body composition and performance: a meta-analysis. Int J Sport Nutr Exerc Metab. (2003) 13:198–226. doi: 10.1123/ijsnem.13.2.198
Keywords: supplementation, pre-workout, post-workout, peri-workout, strength, endurance, body composition, fat-free mass
Citation: Dinan NE, Hagele AM, Jagim AR, Miller MG and Kerksick CM (2022) Effects of creatine monohydrate timing on resistance training adaptations and body composition after 8 weeks in male and female collegiate athletes. Front. Sports Act. Living 4:1033842. doi: 10.3389/fspor.2022.1033842
Received: 01 September 2022; Accepted: 31 October 2022;
Published: 16 November 2022.
Edited by:
Guilherme Artioli, Manchester Metropolitan University, United KingdomReviewed by:
Lívia Gonçalves, University of California, San Francisco, United StatesCopyright © 2022 Dinan, Hagele, Jagim, Miller and Kerksick. This is an open-access article distributed under the terms of the Creative Commons Attribution License (CC BY). The use, distribution or reproduction in other forums is permitted, provided the original author(s) and the copyright owner(s) are credited and that the original publication in this journal is cited, in accordance with accepted academic practice. No use, distribution or reproduction is permitted which does not comply with these terms.
*Correspondence: Nicholas E. Dinan, ZGluYW5AbWlkbGFuZHUuZWR1
Disclaimer: All claims expressed in this article are solely those of the authors and do not necessarily represent those of their affiliated organizations, or those of the publisher, the editors and the reviewers. Any product that may be evaluated in this article or claim that may be made by its manufacturer is not guaranteed or endorsed by the publisher.
Research integrity at Frontiers
Learn more about the work of our research integrity team to safeguard the quality of each article we publish.