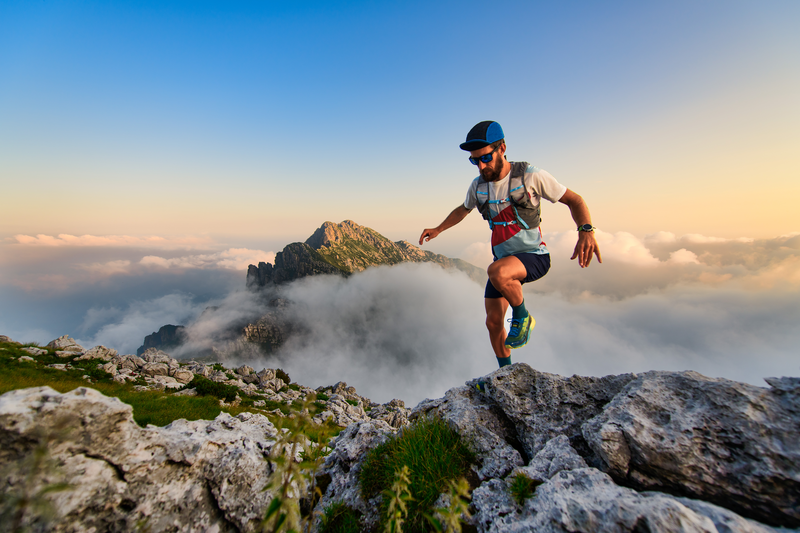
94% of researchers rate our articles as excellent or good
Learn more about the work of our research integrity team to safeguard the quality of each article we publish.
Find out more
ORIGINAL RESEARCH article
Front. Space Technol.
Sec. Advanced Space Engineering
Volume 6 - 2025 | doi: 10.3389/frspt.2025.1550526
This article is part of the Research Topic Next generation of materials for space applications View all 5 articles
The final, formatted version of the article will be published soon.
You have multiple emails registered with Frontiers:
Please enter your email address:
If you already have an account, please login
You don't have a Frontiers account ? You can register here
This study investigates the potential of Microbially Induced Calcium Carbonate Precipitation (MICP) as a repair technique for consolidated (sintered) bricks made from Lunar Highland Simulant-1 (LHS-1), aiming to extend their functional lifespan in extra-terrestrial conditions. Sintered bricks (compressive strength ∼50 MPa) were fabricated with embedded holes, V-shaped notches, and semi-circular notches to simulate structural failure. The compressive strength of these modified bricks was assessed, revealing a significant reduction in strength due to stress concentrations around these cavities. Following this, the cavities were filled with a MICP-based soil slurry, resulting in a notable recovery of compressive strength (∼28-54%), although not to the levels of the original material. Scanning electron microscopy (SEM) analysis demonstrated strong interfacial bonding between the MICP filler and the sintered substrate, indicating the effectiveness of the repair method. Additionally, Digital Image Correlation (DIC) was used to track the crack propagation and growth under the loading conditions. Instances of crack propagation through the MICP interface highlight areas for further investigation. The findings underscore the viability of MICP as a sustainable solution for repairing construction materials, aligning with contemporary practices aimed at enhancing durability and reducing dependency on Earth.
Keywords: Biocementation process, Lunar regolith simulant, Space bricks, Lunar habitation, Repairing
Received: 23 Dec 2024; Accepted: 06 Mar 2025.
Copyright: © 2025 Gupta, Kulkarni, Naik, Viswanathan and Kumar. This is an open-access article distributed under the terms of the Creative Commons Attribution License (CC BY). The use, distribution or reproduction in other forums is permitted, provided the original author(s) or licensor are credited and that the original publication in this journal is cited, in accordance with accepted academic practice. No use, distribution or reproduction is permitted which does not comply with these terms.
* Correspondence:
Aloke Kumar, Indian Institute of Science (IISc), Bangalore, India
Disclaimer: All claims expressed in this article are solely those of the authors and do not necessarily represent those of their affiliated organizations, or those of the publisher, the editors and the reviewers. Any product that may be evaluated in this article or claim that may be made by its manufacturer is not guaranteed or endorsed by the publisher.
Research integrity at Frontiers
Learn more about the work of our research integrity team to safeguard the quality of each article we publish.