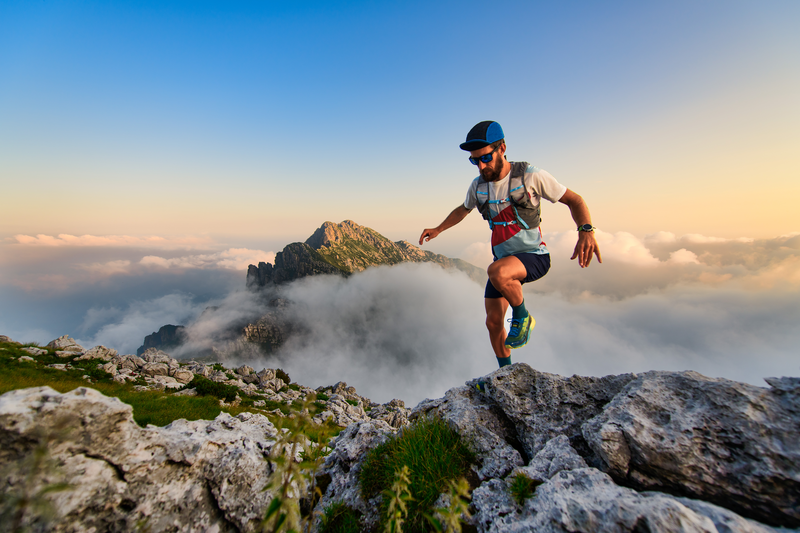
95% of researchers rate our articles as excellent or good
Learn more about the work of our research integrity team to safeguard the quality of each article we publish.
Find out more
REVIEW article
Front. Space Technol. , 02 April 2025
Sec. Space Exploration
Volume 6 - 2025 | https://doi.org/10.3389/frspt.2025.1505823
As the National Aeronautics and Space Administration (NASA), international space agencies, and commercial spaceflight programs set their sights on missions to the Moon and Mars, understanding the impact of spaceflight on astronauts’ health and performance becomes increasingly important. High-fidelity ground-based and space-based simulations of hazards induced by these missions can be used to conduct research that will help mitigate potential adverse outcomes in behavioral health and performance. In this review, current classifications of NASA’s research analogs are discussed, along with their strengths and limitations for effectively assessing risk to Moon- and Mars-bound astronauts’ behavioral health and performance. Recommendations are proposed for future consideration when designing high-fidelity analogs of spaceflight, which emphasize the importance of standardizing protocols, maintaining safety, and addressing ethical standards for future research and for developing analogs of mission-specific habitats.
Human exploration of space presents a multitude of challenges that must be overcome to protect astronauts’ health and performance. As the National Aeronautics and Space Administration (NASA), international space agencies, and commercial spaceflight programs set their sights on missions to the Moon and Mars, mitigating the impact of space conditions on astronauts’ health and performance becomes increasingly important. The effects of prolonged exposure to spaceflight hazards, including microgravity, radiation, isolation and confinement, increased distance from Earth, and hostile, closed environments, need to be understood and mitigated to ensure astronauts’ wellbeing during and after future exploration missions.
Research is underway to help characterize and mitigate the effects of the spaceflight hazards crews will face during future exploration-class missions. Ground-based and space-based simulations—called analogs—replicate key characteristics of space missions and can be used to conduct research that will help inform the likelihood and consequence of adverse outcomes in astronauts’ behavioral health and performance. Analogs that offer a more genuine representation of a specific mission or class of missions, provide a platform through which behavior and performance can be more confidently studied and mitigated. Results from relevant research in analogs of spaceflight, therefore, can help inform the design of future missions, and can be used to define recommendations and guidelines for protecting crew health and performance.
In this review, current classifications of NASA’s isolation and confinement research analogs are discussed, along with strengths and limitations of some of the platforms available for addressing research questions related to behavioral health and performance. The aim of evaluating these spaceflight analogs is to highlight the value of these platforms and to identify additional capabilities needed for reducing risks to behavioral health and performance during lunar and martian missions.
A brief overview of recent behavioral health and performance research conducted in spaceflight analogs is presented, along with the challenges of translating research findings to outcomes in real space missions. Limitations of current analogs and the applicability of the research findings gathered in these settings are also discussed. Recommendations are proposed for consideration when designing future high-fidelity analogs for studying the behavioral health and performance impacts of exploration missions, which emphasize the importance of standardizing protocols, ensuring safety, and addressing ethical standards for future research and for developing dedicated analogs of mission-specific habitats.
Spaceflight analogs are settings that simulate features of the spaceflight environment (Bell et al., 2020) and serve as suitable platforms for informing research questions and/or developing operational concepts (Cromwell and Neigut, 2020). These spaceflight analogs can be broadly divided into 2 categories: (1) those that simulate aspects of spaceflight missions, and (2) those that induce physiological changes akin to changes experienced during spaceflight. The first category of spaceflight analogs can be further categorized into two main types: isolated, confined, and extreme (ICE) environments; and isolated, confined, and controlled (ICC) environments. Both ICE and ICC environments can be valuable for simulating the psychological and physiological impacts of prolonged isolation, although each has distinct advantages and disadvantages in research contexts.
ICE environments, such as Antarctic stations or remote outposts, offer the advantage of naturally occurring conditions that are difficult to fully replicate in controlled environments. These include extreme external conditions, limited resources, and the necessity of self-sufficiency, which contribute to a more authentic experience of isolation and confinement. However, ICE settings also introduce variability and environmental factors that are harder to control, which can impact the consistency of data.
Conversely, ICC environments, such as laboratory-based confinement studies, provide controlled and reproducible conditions, which allow for more precise manipulation of variables, consistent monitoring, and structured experimental protocols. ICC environments are beneficial for isolating specific factors, making it easier to study certain psychological and physiological responses to confinement. However, these settings may lack the authenticity of natural stressors found in ICE environments, which could affect the external validity of findings.
Over the years, contrasting findings have been reported regarding the impact of analog environments on behavioral health and performance, i.e., both salutogenic (health-promoting) and negative effects have been observed. For example, some positive adaptations such as improved stress resilience over time, have been reported during studies in Antarctic stations and simulated isolation environments; however, missions in isolation analogs have also been associated with negative psychological responses, such as heightened anxiety, irritability, depressive symptoms, and interpersonal conflict (e.g., Palinkas and Suedfeld, 2008). As noted recently by De La Torre et al. (2024), studies in isolation environments reveal mixed outcomes, with some crewmembers maintaining performance and cohesion, while others experience increased tension and stress responses during extended missions. These findings underscore the complex and often individualized responses to spaceflight analog environments, highlighting the need for tailored behavioral health countermeasures to support spaceflight.
Note: This review addresses spaceflight analogs used to support research using humans and does not address radiation-specific laboratories such as the Brookhaven National facility.
ICE environments are often in remote locations with extreme weather conditions, such as Antarctic Stations (e.g., South Pole, Palmer), which limit travel, accessibility, and the possibility of emergency evacuation of crewmembers. Scientists and other professionals living and working in these ICE environments primarily focus on conducting specialized field research or training, therefore, human research endeavors are incorporated as a secondary objective. Crewmembers can enroll in research studies that assess the effects of isolation and stress on individuals while they carry out their primary research or training objectives (Cromwell and Neigut, 2020).
Research conducted in ICE environments has provided insights into changes in behavior and performance that could occur on the International Space Station (ISS) or during future extended duration missions beyond low Earth orbit (LEO). For example, Antarctica winter-over crewmembers have experienced disruption of circadian rhythms, as has been shown in astronauts on the ISS (Flynn-Evans et al., 2016). Studies have shown that crewmembers overwintering in Antarctica often experience disruptions in their sleep-wake cycles, leading to fatigue and cognitive impairments (Palinkas and Suedfeld, 2008); on the ISS, astronauts are often subject to schedule shifting, which can severely disrupt their body’s internal clock. Both the ISS and Antarctic environments therefore pose challenges to maintaining a regular day-night cycle, which is crucial for protecting sleep duration and sleep architecture (Nasrini et al., 2020).
Antarctic research has demonstrated that most individuals do not experience clinically significant changes, but rather, that prolonged isolation and confinement can be associated with decreases in positive emotions and increases in physical symptoms for some individuals (Alfano et al., 2021). Whether these declines in wellbeing are indicators of a later adverse behavioral condition is unknown, given that most winter-over expeditions conclude within a year. Evaluating the effectiveness of countermeasures to support behavioral health in an environment such as Antarctica may help determine future methods for testing and implementing targeted mitigation strategies during long-duration exploration missions. ICE environments generally offer a high level of psychological fidelity to distant exploration spaceflight missions, however, the operational nature of these platforms often limits research opportunities. For example, it is often not feasible to assign individuals who winter-over to highly controlled conditions to assess systematic measurements of effects. Crewmembers at these facilities have field work to conduct, limiting their availability to participate in research studies. Additionally, the number of crewmembers can fluctuate throughout the mission duration (roughly around a year), and team sizes are often much larger than typical astronaut crews on a long-duration space mission (Cromwell et al., 2021). Additionally, because crews of stations such as McMurdo no longer undergo psychological selection or screening, selection criteria will vary substantially between Antarctic station crewmembers and astronauts.
ICC environments are settings where isolation and confinement are maintained under regulated conditions. Unlike remote ICE facilities, ICC locations tend to be physically more accessible. Participants of research studies conducted in ICC environments perform as crewmembers of a simulated space mission and are typically isolated and confined to habitats that replicate exploration transit vehicles or extraterrestrial habitats.
ICC platforms provide a realistic simulation of certain aspects of space missions, particularly isolation and confinement, which are critical for studying outcomes related to behavioral health, team dynamics, and operationally relevant task performance. Similar to the limitations of ICE environments, ICC platforms do not replicate the full range of spaceflight hazards encountered in actual space exploration environments, such as microgravity, which impacts physiological functions such as fluid distribution and muscle usage. Microgravity cannot be effectively simulated in ICC settings unless the study is conducted in a bed rest isolation and confinement facility.
Additionally, although ICC platforms can simulate delayed communication with Earth to some extent, they may not fully capture the impacts of prolonged communication delays that astronauts experience during deep-space missions. The simulation aspect of ICC platforms can lower the psychological fidelity of communication delays owing to “distance from Earth”. The physical environment of exploration vehicles, including spatial limitations and equipment, can also be challenging to replicate precisely in ICC habitats.
ICC platforms can be designed to immerse participants in realistic spaceflight activities and scenarios, enabling future systems, interfaces, and tasks to be evaluated and tested. Spaceflight-relevant stressors including increased social isolation, confinement, altered sleep patterns, and elevated stress levels, can affect cognitive abilities, including visuo-spatial orientation skills and their underlying neural mechanisms (Lukavský, 2014). ICC platforms can, therefore, be used to elicit stress responses, and then effectiveness of specific countermeasures can be tested. Future missions will include intelligent onboard technologies to support the crew responding to an anomaly, and technologies that assist the crew by providing relevant knowledge to help them make informed decisions in the absence of immediate ground support. Although human-in-the-loop testing in a laboratory setting is an extremely valuable step when developing and validating such countermeasures, assessing these systems in an exploration-like analog allows for a fuller assessment in a realistic mission-like environment. ICC environments are particularly helpful for assessing changes in crew performance over extended durations because these facilities allow crews to live and conduct simulated mission operations over periods spanning many weeks or months.
The Human Exploration Research Analog (HERA) at NASA Johnson Space Center (Figures 1, 2) and the Scientific International Research in a Unique terrestrial Station (SIRIUS) at the Nazemnyy Eksperimental’nyy Kompleks (NEK) (Figures 3, 4) are examples of ICC facilities. NASA’s HERA is a 2-tiered module attached to a hygiene module and a simulated airlock (see Figure 2). NASA conducted its first HERA Campaign in 2014, which included a complement of studies on a team of four astronaut-like individuals in the HERA facility over a 7-day mission. These missions were repeated 4 times, each with a new set of four crewmembers, over a period of 1 year. In more recent years, HERA campaigns have included a complement of studies repeated 4 times a year in missions lasting around 45 days. Each 1-year campaign typically ‘sets’ a standard operational scenario with a consistent tempo and series of stressors, i.e., severe sleep restriction schedule throughout HERA Campaign 4 (2017–2018). More information on HERA can be found in Appendix A.
Figure 1. NASA’s Human Exploration Research Analog (HERA) facility at Johnson Space Center. Source: NASA JSC HERA Facility. Source: https://www.nasa.gov/hera-about-hera/.
Figure 2. Diagram of the 148.6 m3 interior of the Human Exploration Research Analog (HERA), which supports four crewmembers. Source: NASA HERA Facility and Capabilities Information https://www.nasa.gov/wp-content/uploads/2016/05/2019_hera_facility_capabilities_information.pdf.
Figure 3. Scientific International Research in Unique Terrestrial Station (SIRIUS) Nazemnyy Eksperimental’nyy Kompleks (NEK) habitat. Accessed 15 August 2024. https://www.nasa.gov/mission/nek-and-sirius/.
Figure 4. Diagram of the interior of Scientific International Research in Unique Terrestrial Station (SIRIUS) Nazemnyy Eksperimental’nyy Kompleks (NEK) habitat, which supports six crewmembers. Accessed 15 August 2024. https://www.nasa.gov/mission/nek-and-sirius/.
Research in ICC environments has helped to characterize both individual and team behavioral health and performance outcomes. Similar to spaceflight and ICE experiences, most findings and reports from ICC environments highlight positive experiences without adverse outcomes. Specific challenges in some individuals however can arise.
As an example, in their assessment of performance changes over 45-days under the HERA Campaign 4 sleep restriction protocol (5 nights of 5 h in bed, followed by a two nights of 8-h sleep opportunity, repeated throughout each 45-day mission), Flynn-Evans and colleagues (2023) found a significant worsening of performance from the beginning to the end of the mission for mean reaction time, response speed, and fastest 10% reaction time. Researchers have also shown that individuals performing a spaceflight-like task in HERA can demonstrate performance impairments over time, even under nominal conditions (Stankovic et al., 2023), suggesting such isolation and confinement analogs can impact performance of complex tasks. Additionally, at a team level, creativity and conceptual performance has been shown to decrease later in the mission when compared to performance early in the mission (Larson et al., 2019). Other performance measures in HERA however, such as performance on the Cognition test battery, have been reported to be mostly steady or improved over time under nominal schedules (Dev et al., 2024).
Studies in the NEK facility in Moscow have further characterized the potential psychological effects of prolonged exposure to confinement and isolation. For example, three crewmembers who participated in the 520-day simulated mission to Mars in the NEK reported increasing levels of stress, physical exhaustion, and workload throughout the mission, with one of these individuals reporting increasing symptoms of depression (Basner et al., 2014). A more recent international analog campaign included six participants in an 8-month simulated spaceflight mission in the NEK. Lungeanu and colleagues (2023) found that these isolated participants developed and maintained more motivating relationships than non-isolated teams, but also faced fewer viable relationships and sustained fragmentation.
In summary, both ICE and ICC environments contribute uniquely to our understanding of isolation and confinement. ICE settings offer realism and ecological validity, whereas ICC environments provide control and replicability, allowing researchers to balance the advantages and limitations of each approach based on their specific research objectives.
The second category of spaceflight analogs induce physiological adaptations without simulating all spaceflight conditions. Long-duration (60 days or more) of head-down tilt bed rest is a prime example of this category (Sundblad et al., 2016). Bed-rest analogs simulate the effects of microgravity on the body, impacting musculoskeletal, cardiovascular, and sensorimotor systems, while also allowing researchers to measure the psychological responses to prolonged inactivity and confinement. This dual focus on both physiological and psychological functions may enhance the relevance of ICC platforms for studying the multifaceted challenges of space exploration.
Lying in a bed for extended periods with the head tilted slightly downward, simulates the effects of microgravity. This position induces fluid shifts towards the upper body, emulating the bodily fluid redistribution astronauts experience during spaceflight (Lee et al., 2019). This change of gravity loading affects multiple physiological systems, notably the musculoskeletal, cardiovascular, sensorimotor systems, and potentially behavioral health systems, as summarized in Table 1 (Basner et al., 2021; Scott et al., 2020; Richter et al., 2017; Scott et al., 2011). Muscle atrophy and bone loss are induced during bed rest as a direct consequence of prolonged inactivity (Sundblad et al., 2016). Additionally, recent studies have determined that bed rest impacts the sensorimotor system (Mulavara et al., 2018).
Assessments of behavioral data collected during 60 days of bed rest show an increasing tendency over time towards negative valence, as noted by Basner et al. (2021). Subjective ratings of sickness, physical exhaustion, mental fatigue, and stress increased over time throughout the duration of bed rest, and additional deterioration occurred during the post bed-rest recovery phase (Basner et al., 2021). Likewise, a study by Brauns et al. (2021) showed that participants in a 60-day bed-rest study experienced mood decrements, emotion recognition challenges, and other behavioral changes. Vestibular and emotional dysregulation can occur together because the vestibular and emotional systems share neural pathways in regions such as the insular and anterior cingulate cortex (Preuss et al., 2014a; Preuss et al., 2014b; Clément et al., 2020). This shared functionality is evident in these brain areas, where vestibular and emotional networks overlap, suggesting that vestibular signals play a role in affective control and decision-making outcomes (Carmona et al., 2009; Preuss et al., 2014a; Preuss et al., 2014b).
These findings may be related to the inherent isolation of bed-rest participants; unlike spaceflight missions, where astronauts work in teams towards a common goal, current bed-rest study participants are typically isolated, therefore, bed-rest participants have less social and team interactions than astronauts during a space mission. The experience of bed rest can therefore feel even more isolating than spaceflight, presenting an intriguing interplay between physiology and psychology. Bed rest can be used to study the interaction of multiple factors, including fluid shifts, variations in CO2 concentrations, glymphatic changes, and sleep, but in its current common design, bed rest does not fully capture important social and team aspects of behavioral health and performance.
Flight-based analogs of spaceflight conditions currently include parabolic flights, which induce short-term bouts of microgravity (multiple exposures each lasting an average of 20 s over the course of approximately 120 min), enabling researchers to study the acute effects of microgravity on a range of human performance outcomes. Relevant outcomes measured encompass physiology, fluid shifts, and sensorimotor function. Such studies have furthered the understanding of how the human body reacts to microgravity, even for a brief or transitional period (Kermorgant et al., 2020). Parabolic flights can also be used to ensure equipment intended for use on the ISS functions optimally in the real conditions of the spaceflight environment. Although, in certain circumstances data obtained in parabolic flight is very translatable to spaceflight, the short-term exposure to microgravity limits translating outcomes for long-duration space missions, and minimal behavioral health and performance focused research has been conducted on this platform to date.
Flight-based studies provide a direct exposure to microgravity and the spaceflight environment, but they also come with limitations relative to future exploration missions, in addition to logistical and cost challenges. Researchers can use the ISS to study the physiological, neurological, and psychological effects that occur with long-duration exposure to microgravity in LEO (Kermorgant et al., 2020), and studies on the ISS have provided insights into some of the challenges astronauts will encounter on extended missions, such as prolonged exposures to weightlessness and radiation. Radiation exposure levels on the ISS however, are relatively low compared to radiation exposures anticipated during long-term interplanetary missions.
Much has been learned from the ISS in preparation for future missions regarding protecting sleep and circadian rhythms, particularly through the scheduling of tasks and through habitat design (Flynn-Evans et al., 2023). High tempo lunar missions in vehicles and habitats that will be much smaller than the ISS will require alternate mitigation strategies. Additionally, communication challenges and changes in the roles of personnel in the mission control center (MCC) and ISS astronauts during exploration missions are expected to impact scheduling, extravehicular activity (EVA), and MCC support. The relatively close proximity of the ISS to Earth and the real-time communication between MCC and the crew limits the ability to simulate the delayed MCC communications and support that will occur during future exploration missions—conditions that will require crews to function more autonomously with interfaces and processes that are developed accordingly (Gore et al., 2021).
The increasing presence of commercial vehicles in LEO offers another potential platform for conducting spaceflight research. Depending on the provider and the nature of the missions, such endeavors offer unique opportunities for human spaceflight research that will prepare for exploration missions. Recent commercial missions include the Inspiration-4 SpaceX mission, during which 4 civilian SpaceX astronauts embarked on a 3-day mission at a peak altitude of 364 miles. Human research studies facilitated by SpaceX, the Translational Research Institute for Space Health, and other partners, addressed key aspects such as biological and behavioral changes. Presently, the duration of these commercial missions spans minutes to several days, and planning is underway for future extended commercial missions in LEO. These commercial spaceflight platforms may provide more comprehensive opportunities for conducting research on behavioral health and performance in preparation for future exploration missions.
Each terrestrial platform offers unique capabilities and constraints that should be taken into consideration when determining whether an analog is a good fit for addressing specific research questions (Keeton et al., 2011; Cromwell et al., 2021). To date, studies in ICC environments have furthered our understanding of the challenges of future spaceflight, in part by offering a standardized setting where conditions, such as temperature, humidity, mission duration, work-rest schedules, and food options, can be consistently controlled. This uniformity ensures a reliable foundation for research that minimizes potential confounding variables.
Although many analogs are designed to mimic the conditions of space to the best possible extent, they may not fully capture many of the challenges faced in space—including the psychological sense of true separation from Earth. ICC platforms are designed to mimic the isolation and confinement astronauts experience during long-duration missions, and often include authentic scenarios and tasks to elicit as close to realistic responses anticipated during future spaceflight missions. Current preliminary evidence however suggests that, in comparison to current spaceflight, missions in platforms such as the HERA have been limited in their ability to induce spaceflight-like behavioral responses. In their assessment of subjective stress ratings over multiple analog platforms, Dev and colleagues (2024) reported that participants of just one of 4 HERA analog campaigns demonstrated similar subjective responses as those of ISS crewmembers for ratings across constructs such as stress and workload. Notably, the campaign that elicited the responses that aligned most closely with the responses of spaceflight crews was HERA Campaign 4, which ran a series of 4, 45-day missions during which participants were consistently restricted to 5 h of sleep (time in bed) for five nights a week, and up to 8 h of sleep (time in bed) two nights a week. Hence, additional efforts are needed to augment current spaceflight analogs so that they are more analogous to spaceflight.
Although the ISS itself offers a high-fidelity platform for studying the hazards of future exploration missions, it does not fully replicate the mission characteristics of either a short-duration lunar mission or a transit to Mars. For example, unlike the relatively infrequent amounts of communication delays experienced between the MCC and the ISS, lunar missions will face roundtrip communication latencies of 4 to 14 seconds (e.g., Parisi et al., 2024). Although 4 to 14 s communication delays may seem minimal, during critical situations even such short delays can have significant implications. Astronauts on missions to the lunar surface will also have limited exercise and food options, significantly less vehicle space and privacy than that provided by the ISS, and limited re-supply of resources. The lack of real time communication with Earth will force operations towards increasing autonomy; crews will also need to be more responsive during emergencies due to limited options for evacuation.
Studies of the effects of short-term communication delays could be conducted on the ISS, but such studies are resource intensive and lack fidelity to future missions, given that ISS systems are likely not the same as systems on future vehicles. The ISS was designed between 1984 and 1993 and was built to be controlled from the ground, whereas future vehicles include more recent advances in technology and automation. Although understanding ISS systems and procedures is essential for defining future mission processes, platforms with higher fidelity to future vehicles are needed.
During exploration missions, reliance on ground control will decrease, especially during missions to Mars (Robinson et al., 2019). The communication delays between Earth and Mars will increase to approximately 20 min one way. This substantial delay will require a shift in the operational paradigm regarding support from MCC. Crews on Mars missions will have to become more autonomous, making critical decisions in real-time without the immediate guidance of MCC support. The duration of these missions will also be significantly longer; a Mars mission will last several months to years.
As noted by Clément and colleagues (2020), radiation exposure will be a crucial factor during future lunar and martian missions due to its potential long-term health impacts on astronauts. Missions beyond Earth’s magnetosphere expose astronauts to significantly higher levels of cosmic radiation, which can affect various physiological systems and is associated with increased cancer risk, central nervous system effects, and cognitive impacts. As noted by Sishc and colleagues (2022), targeted mitigations are needed for risks associated with space radiation exposure to ensure astronauts are safe during and after missions to Mars.
Although it is not ethical to incorporate radiation exposures when studying humans in analogs of spaceflight, radiation is an underlying hazard that could impact the long-term health of crewmembers, which highlights the complexities of maintaining crew health and safety in deep space (Miller et al., 2022). Understanding the impacts of exposure to cosmic radiation is essential for developing comprehensive countermeasures to ensure crewmembers’ wellbeing on future lunar and martian missions. Terrestrial rodent studies are used to assess the effects of the radiation exposure crewmembers are anticipated to receive beyond LEO (Dev et al., 2025; Mao et al., 2024; Alaghband et al., 2023; Miller et al., 2022; Mhatre et al., 2021; Willey et al., 2021), which are much higher than the exposures incurred by astronauts on the ISS. A description of these studies is beyond the scope of this paper; however, it is important to note that efforts are being conducted to translate the anticipated radiation exposure during lunar and martian missions to human health and performance outcomes (Alwood et al., 2023).
Efforts critical for designing habitats and implementing protective measures for Moon and Mars exploration include direct measurements of the space radiation environment around the Moon (George et al., 2024), developing and validating models to simulate radiation exposure at the lunar surface and subsurface that help to quantify the potential exposure astronauts would receive during lunar missions (Dobynde and Guo, 2021), and efforts to inform risk mitigation strategies for astronauts during Mars missions by analyzing data from the Mars Science Laboratory’s Radiation Assessment Detector to determine the radiation environment on the surface of Mars (Guo et al., 2021).
Choosing the best spaceflight analog in which to implement a research study is contingent upon the specific science objectives and the resulting characteristics needed to properly evaluate those objectives. Regarding research into human factors and behavioral performance, analogs can be used to characterize risk, identify biomarkers, refine measures, and/or test and evaluate tools and mitigation strategies in preparation for their application during spaceflight. Logistical factors, such as the degree of experimental control, the desired sample size, and the time between study approval and implementing the study, can also play a role in deciding which spaceflight analog to use. Refer to Table 2 for an outline of research categories and questions that may be considered when choosing an analog.
As the next phase of human spaceflight approaches, the capabilities of current spaceflight analogs should be evaluated to ensure these platforms accurately reflect future spaceflight scenarios. The ability to generalize findings from research in spaceflight analogs to characterize and mitigate behavioral health and performance risks in a future exploration mission depends in part on the fidelity of the analog itself, i.e., how closely it reproduces the environment, operations, and crew of lunar and martian missions.
Near-term Artemis lunar missions are expected to be short-duration, high-tempo missions with communication delays of around 4–15 s one-way. Eventually these missions will include crewmembers that are dispersed between the Gateway (the lunar orbiting vehicle), the lunar lander, and the lunar rover. Journeys to Mars are anticipated to include prolonged isolation, extended communication delays of up to 19 min one-way, increased autonomy with reduced ground support, and limited access to food, recreation options, and communication with family.
The escalation in complexity of these missions introduces new criteria that, when properly incorporated, can enhance the fidelity of future spaceflight analogs, ensuring a more genuine representation of what crews will experience during missions to the Moon or Mars. Increasing fidelity can likewise foster a higher level of genuine engagement from participants, which will produce tools and methods that can be translated with higher confidence into operational countermeasures and interventions. Technologies that support different aspects of a mission are typically developed in isolation, without considering the overlapping infrastructures of adjacent systems. Spaceflight analogs can also serve as a testbed for maturing technologies in an integrated environment analogous to the one in which they will be used (Wu and Vera, 2019).
To support increased crew autonomy, future vehicles will likely incorporate more automation and additional design considerations that are not required on the ISS because the ISS relies on support from the MCC. Furthermore, EVA on the lunar and martian surface will differ substantially from EVA conducted during ISS missions. Astronauts will also be exposed to higher levels of cosmic radiation when they travel beyond Earth’s magnetosphere than they do on the ISS. The effects of this increased radiation exposure require further study to understand and mitigate its long-term effects on crew health.
To address these evolving demands, new spaceflight analogs should be identified, or existing platforms enhanced, to better encapsulate the challenges of future exploration missions. Although no terrestrial analog can perfectly emulate every facet of a spaceflight, it’s important to consider the following:
• What are the key hazards to behavioral health and performance during future missions to the Moon and Mars?
• What simulated features are required to study the effects of these hazards and to better mitigate them?
• How can existing spaceflight analogs be adapted or modified to fit the objectives of a lunar or martian mission?
• If only limited spaceflight analogs exist to answer a specific research question, what are the desirable vs necessary requirements of a new platform that can provide a high-fidelity simulation of a lunar or martian mission?
The defining features of lunar and martian missions call for an evaluation of the science questions and resulting requirements to achieve mission-specific, high-fidelity simulations. Establishing clear and scientifically grounded specifications that align research objectives and data collection will allow for a better representation of future space missions that can be used to determine effective countermeasures for these missions.
Developing recommendations for high-fidelity analogs of near-term Artemis lunar missions, extended duration lunar missions, and martian missions will involve unique challenges and will incorporate some shared elements. NASA’s Design Reference Missions (DRM) provide a frame of reference of planned characteristics such as mission duration and operational characteristics. The DRMs and subsequent analog recommendations are aligned in Table 3. Ideally, spaceflight analogs should be modifiable or adaptable to simulate the dynamic nature of the environment and operations, and the psychological stressors of various mission types, from lunar surface stays to long-duration missions to Mars. Current spaceflight analogs vary in their capacity to accurately replicate features of the spaceflight environment, with many falling short in fully capturing certain elements of lunar or martian missions. Maintaining a balance between what is practically achievable in simulations and the ideal requirements will be optimal, because not every facet of the space environment can be replicated.
EVAs on the lunar and martian surfaces will substantially differ from those conducted on the ISS. Whereas EVA on the ISS is primarily focused on vehicle maintenance and occur in microgravity, lunar and martian EVA will require astronauts to navigate the planet surface in reduced gravity, which will affect mobility and will require new techniques and EVA equipment (León et al., 2021). Furthermore, the lunar and the martian regolith, which is composed of fine, abrasive dust, may pose significant challenges to the health of astronauts and the durability of equipment (see Figures 5, 6).
Figure 5. NASA’s Concept of Mars Extravehicular Activity: Partial-gravity, natural surface, partial atmosphere. Source: https://www.nasa.gov/directorates/stmd/prizes-challenges-crowdsourcing-program/nasa-marsxr-challenge/.
Figure 6. NASA’s Concept of Lunar Extravehicular Activity:Partial-gravity, natural surface, thermal vacuum. Source: https://www.nasa.gov/image-article/astronauts-lunar-surface-nasa-artist-concept-of-eva-astronauts-working-lunar-surface/.
Analogs of lunar and martian EVA should account for the technical and environmental complexities of the specific mission. Scenarios should cover both routine and off-nominal surface EVA, including habitat maintenance or assembly, geological sampling, and navigation. Innovations such as detachable rover modules and flexible modules for habitat-system assembly and repair activities will enhance the realism of these simulations and allow for a closer approximation of the physical and technical aspects of conducting EVA.
EVA on the initial lunar missions is anticipated to include two crewmembers on the surface. Simulations should include the low-light and/or low-angle lighting conditions that are expected near the poles or permanently shadowed regions to prepare astronauts for EVA in environments with minimal sunlight. Training with limited visibility, using lighting equipment on spacesuits and rovers, and conducting scientific operations in minimal lighting are essential for adapting to these conditions. Off-nominal scenarios, such as medical conditions, technical malfunctions, or communication latency, should also be simulated (Walton et al., 2023).
Simulations of EVA on Mars should include extended, multi-day EVA durations and variable surface and weather conditions (Coan et al., 2020). Scenarios should focus on crew autonomy, particularly when managing critical incidents such as equipment malfunctions or environmental hazards or prioritizing and managing limited resources, all while contending with the psychological stresses of increased isolation and confinement.
The volume and structure of lunar and martian surface habitats will differ dramatically from the ISS (see Figure 7). Surface habitats need to ensure adequate minimal volume for logistics, storage, systems, and crew living and work functions (Whitmire et al., 2014; Burke et al., 2022; Howard, 2021). Analogs of these habitats and modules should accurately reflect the logistical constraints and limited volume anticipated for these DRMs to mimic the specific activities and challenges of living and working in confined spaces (see Figures 7–10). Other factors that will contribute to creating a realistic living and working environment include noise levels, temperature regulation, task scheduling, and internal lighting conditions. If these elements are carefully controlled to simulate the actual conditions of space habitats, researchers can align these environmental factors with anticipated conditions of the internal habitat and the EVA environment. Research should reference the habitat-specific challenges, characteristics and requirements of Artemis, Gateway, and Mars habitats listed in Table 4.
Figure 7. NASA International Space Station comparison to length of a football field. The ISS is approximately 388 cu. m. Source: https://www.nasa.gov/space-and-football/.
Figure 8. NASA Orion Capsule crew module which is 9.0 cu. m. Source: https://www.nasa.gov/reference/orion-spacecraft/.
Figure 9. Rendering of NASA Gateway Habitat Module, which will be approximately 125 cu. m. Source: https://www.nasa.gov/mission/gateway/.
Figure 10. NASA lunar surface habitat concept that support a crew of two for approximately 30 days (Burke et al. 2022).
Because crews of exploration missions will be required to perform more autonomously, their workload will increase and operational processes may become more complex, especially when crewmembers are required to work in dispersed locations. It is critical that the composition of analog teams reflect the intricate social dynamics of real spaceflight crews including both sexes, and multiple nationalities, technical, and organizational backgrounds. Spaceflight analogs should support assessment of different team structures that focus on defining clear roles, responsibilities, and the importance of managing cultural and interpersonal differences. Behavior should be monitored continuously both at the team and individual levels to evaluate performance in autonomous decision-making scenarios and help determine ways through which safety and performance can be supported. Example Crew Health and Performance Characteristics and Analog Recommendations for Artemis, Gateway, and Mars habitats are listed in Table 5.
Systematically implementing sensitive, reliable, and validated measures is important for studying spaceflight-induced changes in behavioral health and performance. In addition, the ability to aggregate and analyze data garnered from multiple analog missions enriches the relevance and applicability of research findings, training outcomes, and risk mitigation strategies for spaceflight. Ensuring that analog platforms accurately reflect the specific mission characteristics and possess robust infrastructure for secure data collection, storage, and accessibility will be essential for effectively mitigating identified risks. NASA has established a set of standard measures that are conducted in analogs of spaceflight isolation such as in the HERA (in addition to standard measures collected on the ISS). These standard measures are the “gold standards” for helping characterize specific physiological and behavioral health outcomes and for providing insights into responses to space-like conditions. These include measures of sensorimotor function, cognitive function, immune markers, and sleep-wake activity (Theriot et al., 2024).
A global centralized database that houses data from spaceflight analog missions is essential to facilitate data pooling and insights across diverse analog environments. A repository would streamline data comparison and pattern analysis, provide detailed documentation to clarify data collection protocols and contexts that include analog characteristics, task specifics, and participant demographics. Standardizing data collection protocols and metadata documentation is also recommended because this will enhance the robustness and reproducibility of the research. Standard methods would allow results to be compared effectively and hypotheses to be refined. The central database should also host a suite of validated assessment tools that will allow researchers to access established metrics for crew performance and to evaluate behavioral health.
During the next phase of analog mission research, the ongoing use of multi-modal data collection tools should be considered, including wearable sensors and environmental tracking systems, which play a key role in providing real-time insights across a spectrum of parameters, from physical health to cognitive functioning and interpersonal dynamics. Physiological measurements offer promising avenues for unobtrusive monitoring, and an essential part of this process is establishing baseline performance levels and understanding normal variability when conducting mission-critical tasks. Further research is needed to establish consistent physiological markers across different cognitive states, such as workload or situational awareness, whose reliability may be influenced by fatigue, stress, and individual experience levels. In addition, assessing such changes in physiology and behavior relative to changes in operational and/or meaningful outcomes (e.g., task performance) provides a more comprehensive assessment for determining the impact and implications of such changes.
The use of advanced artificial intelligence (AI) and machine learning algorithms can help streamline the collection and analysis of datasets generated from these multi-modal data collection tools. For instance, AI-driven analytics could help modulate environmental conditions, workload, and even parameters surrounding social interactions. These technologies can be used to identify patterns and predictive markers that are not immediately apparent through traditional analysis methods, leading to more personalized and adaptive support systems within analog environments based on real-time data inputs.
AI-driven analytics may also help with the collection of unobtrusive measures. Tailoring unobtrusive metrics such as speed and accuracy measures to specific tasks and skills enhances the accuracy of measurements while a task is conducted without interference. Recent research has shown that voice recordings and speech analysis of conversations between crewmembers is a valuable non-intrusive metric for evaluating dynamics of human-machine interactions, such as workload (e.g., Paromita et al., 2023), and can provide insights to help develop training materials specifically tailored to teams and individuals.
Developing virtual and augmented reality tools that simulate more complex and varied environments offers another promising direction for future research. These technologies can create highly immersive and variable scenarios that mimic the unpredictability of real space missions, which could be used to provide invaluable training and evaluation opportunities. Research that is designing virtual reality scenarios to test and refine crew responses to unforeseen challenges and emergencies has shown some promising results, such as enhancing preparedness for actual spaceflight and resilience to future stressors (Finseth et al., 2023). Integrating these sophisticated simulations with physiological and psychological monitoring tools would provide a holistic method to prepare crewmembers for spaceflight, and fine-tuned mission parameters would ensure maximum safety and efficiency during spaceflight. These advancements will not only push the boundaries of current spaceflight analog capabilities but also significantly help crewmembers to prepare for long-duration space missions.
In conclusion, a multi-faceted, collaborative approach across the larger spaceflight community will further reduce risk to behavioral health and performance during future exploration missions. As new analog opportunities arise and provide additional platforms through which to address research questions, efforts that help increase fidelity to future mission scenarios can increase analogs’ value for risk reduction. Additionally, findings from carefully crafted research can serve to inform—while being informed by—the larger field of social sciences and humanities, providing a richer, interdisciplinary perspective. Recommendations are provided for those seeking to refine and/or develop analogs as a mechanism through which to help characterize and mitigate behavioral health and performance risks for future spaceflight.
KR: Writing–original draft, Writing–review and editing. AM: Conceptualization, Methodology, Data curation, Visualizations, Writing–original draft, Writing–review and editing. KG: Writing–original draft, Writing–review and editing. BG: Conceptualization, Methodology, Resources, Writing–original draft, Writing–review and editing. AW: Conceptualization, Data curation, Funding acquisition, Investigation, Methodology, Project administration, Resources, Supervision, Validation, Visualization, Writing–original draft, Writing–review and editing.
The author(s) declare financial support was received for the research and/or publication of this article. This study was funded by NASA’s Human Research Program.
The authors would like to extend their appreciation to additional contributors from NASA’s Human Research Program: Jancy McPhee (Chief Scientist Office) and Sara Whiting (Research Operations and Integration Element) for their insightful feedback and support in the development and writing stages of this review.
Authors KR, AM and KG were employed by KBR.
The remaining authors declare that the research was conducted in the absence of any commercial or financial relationships that could be construed as a potential conflict of interest.
The author(s) declare that no Generative AI was used in the creation of this manuscript.
All claims expressed in this article are solely those of the authors and do not necessarily represent those of their affiliated organizations, or those of the publisher, the editors and the reviewers. Any product that may be evaluated in this article, or claim that may be made by its manufacturer, is not guaranteed or endorsed by the publisher.
Alaghband, Y., Klein, P. M., Kramár, E. A., Cranston, M. N., Perry, B. C., Shelerud, L. M., et al. (2023). Galactic cosmic radiation exposure causes multifaceted neurocognitive impairments. Cell Mol. Life Sci. 80 (1), 29. doi:10.1007/s00018-022-04666-8
Alfano, C. A., Bower, J. L., Connaboy, C., Agha, N. H., Baker, F. L., Smith, K. A., et al. (2021). Mental health, physical symptoms, and biomarkers of stress during prolonged exposure to Antarctica's extreme environment. Acta Astronaut. 181, 405–413. doi:10.1016/j.actaastro.2021.01.051
Alwood, J. S., Mulavara, A. P., Iyer, J., Mhatre, S. D., Rosi, S., Shelhamer, M., et al. (2023). Circuits and biomarkers of the central nervous system relating to astronaut performance: summary report for a NASA-sponsored technical interchange meeting. Life (Basel) 13 (9), 1852. doi:10.3390/life13091852
Basner, M., Dinges, D. F., Mollicone, D. J., Savelev, I., Ecker, A. J., Di Antonio, A., et al. (2014). Psychological and behavioral changes during confinement in a 520-Day simulated interplanetary mission to Mars. PLOS One 9 (3), e93298. doi:10.1371/journal.pone.0093298
Basner, M., Stahn, A. C., Nasrini, J., Dinges, D. F., Moore, T. M., Gur, R. C., et al. (2021). Effects of head-down tilt bed rest plus elevated CO2 on cognitive performance. J. Appl. Phys. 130 (4), 1235–1246. doi:10.1152/japplphysiol.00865.2020
Bell, S. T., Roma, P. G., and Caldwell, B. J. (2020). “Special considerations for conducting research in mission-simulation analog environments: challenges, solutions, and what is needed,” in Psychology and human performance in space programs. Editors L. B. Landon, K. J. Slack, and E. Salas (Boca Raton, FL: CRC Press), 47–65.
Brauns, K., Friedl-Werner, A., Gunga, H. C., and Stahn, A. C. (2021). Effects of two months of bed rest and antioxidant supplementation on attentional processing. Cortex 141, 81–93. doi:10.1016/j.cortex.2021.03.026
Burke, C., Howard, R. L., and Kessler, P. (2022). “Internal layout of a lunar surface habitat,” in ASCEND AIAA. doi:10.2514/6.2022-4266
Carmona, J. E., Holland, A. K., and Harrison, D. W. (2009). Extending the functional cerebral systems theory of emotion to the vestibular modality: a systematic and integrative approach. Psych. Bull. 135 (2), 286–302. doi:10.1037/a0014825
Clément, G. R., Boyle, R. D., George, K. A., Nelson, G. A., Reschke, M. F., Williams, T. J., et al. (2020). Challenges to the central nervous system during human spaceflight missions to Mars. J. Neurophysiol. 123 (5), 2037–2063. doi:10.1152/jn.00476.2019
Coan, T., Fossum, M. E., Dunbar, A., and Cones, R. (2020). System concept of operations revision B. NASA. Houston, TX: Johnson Space Center. Available online at: https://www.nasa.gov/wp-content/uploads/2017/02/eva-exp-0042_xeva_system_con_ops_rev_b_final_dtd_10192020_ref_doc.pdf (Accessed February 11, 2025).
Cromwell, R. L., Huff, J. L., Simonsen, L. C., and Patel, Z. S. (2021). Earth-based research analogs to investigate space-based health risks. New Space 9 (4), 204–216. doi:10.1089/space.2020.0048
Cromwell, R. L., and Neigut, J. (2020). “Spaceflight research on the ground: managing analogs for behavioral health research,” in Psychology and human performance in space programs. Editors L. B. Landon, K. J. Slack, and E. Salas (Boca Raton, FL: CRC Press), 23–24.
De la Torre, G. G., Groemer, G., Diaz-Artiles, A., Pattyn, N., Van Cutsem, J., et al. (2024). Space analogs and behavioral health performance research: a systematic review. NPJ Microgravity 10 (1), 37. doi:10.1038/s41526-024-00437-w
Dev, S., Landon, L., Anderson, S., Miller, J., Begerowski, S., and Bell, S. (2024). The HFBP-EM Harmonized Dataset: comparing behavioral medicine and team risk between short and long duration spaceflight analogs. Galveston, Texas: NASA Human Research Program- Investigators’ Workshop.
Dev, S. I., Whiting, S. E., Nelson, G. A., Dotson, V., Mulavara, A., Schorn, J. M., et al. (2025). Evidence report: risk of adverse cognitive or behavioral changes and psychiatric disorders leading to in-mission health and performance and long-term health effects. Hum. Res. Program, Hum. Factors Behav. Perform. Elem. NASA. Houston, TX: Johnson Space CenterAvailable online at: https://humanresearchroadmap.nasa.gov/Evidence/other/HRP-ER-BMED-HFBP-20250210.pdf. Accessed February 11, 2025
Dobynde, M. I., and Guo, J. (2021). Radiation environment at the surface and subsurface of the Moon: model development and validation. J. Geophys. Res. Planets 126 (11), e2021JE006930. doi:10.1029/2021je006930
Finseth, T., Dorneich, M. C., Vardeman, S., Keren, N., and Franke, W. D. (2023). Real-time personalized physiologically based stress detection for hazardous operations. IEEE Access 11, 25431–25454. doi:10.1109/access.2023.3254134
Flynn-Evans, E. E., Barger, L. K., Kubey, A. A., Sullivan, J. P., and Czeisler, C. A. (2016). Circadian misalignment affects sleep and medication use before and during spaceflight. NPJ Microgravity 2 (1–6), 15019. doi:10.1038/npjmgrav.2015.19
Flynn-Evans, E. E., Glaros, Z. L., and Jansen, R. A. (2023). “Astronaut sleep duration varies by timing of scheduled sleep,” in Aerospace medical association (AsMA) 2023 conference.
George, S. P., Gaza, R., Matthiä, D., Laramore, D., Lehti, J., Campbell-Ricketts, T., et al. (2024). Space radiation measurements during the Artemis I lunar mission. Nature 634, 48–52. doi:10.1038/s41586-024-07927-7
Gore, B. F., Vera, A., Marquez, J., Holden, K., Amick, R., Dempsey, D., et al. (2021). “NASA evidence report: risk of inadequate human system integration architecture (HSIA),” in Human research program, human factors and behavioral performance element, johnston space center. TX: NASA. Available online at: https://humanresearchroadmap.nasa.gov/evidence/reports/Risk%20of%20Inadequate%20Human-System%20Integration%20Architecture%20approved.pdf.
Guo, J., Zeitlin, C., Wimmer-Schweingruber, R. F., Hassler, D. M., Ehresmann, B., Rafkin, S., et al. (2021). Radiation environment for future human exploration on the surface of Mars: the current understanding based on MSL/RAD dose measurements. A&R 29, 8. doi:10.1007/s00159-021-00136-5
Howard, R. L. (2021). Notional habitat science outfitting for a lunar surface habitat or Mars transit habitat. ASCEND AIAA. doi:10.2514/6.2021-4021
Keeton, K., Whitmire, A., Feiveson, A., Ploutz-Snyder, R., Leveton, L. B., and Shea, C. (2011). Analog assessment tool report. NASA/TP–2011-216146. Houston, TX: NASA.
Kermorgant, M., Nasr, N., Czosnyka, M., Arvanitis, D. N., Hélissen, O., Senard, J. M., et al. (2020). Impacts of microgravity analogs to spaceflight on cerebral autoregulation. Front. Physiol. 11, 778. doi:10.3389/fphys.2020.00778
Larson, L., Wojcik, H., Gokhman, I., DeChurch, L., Bell, S., and Contractor, N. (2019). Team performance in space crews: Houston, we have a teamwork problem. Acta Astronaut. 161. 108–114.
Lee, J. K., Koppelmans, V., Riascos, R. F., Hasan, K. M., Pasternak, O., Mulavara, A. P., et al. (2019). Spaceflight-associated brain white matter microstructural changes and intracranial fluid redistribution. JAMA Neurol. 76 (4), 412–419. doi:10.1001/jamaneurol.2018.4882
León, G. R., Nelson, G. R., and De Leon, P. (2021). “Stress and coping during simulated EVAs and habitat living,” in 50th international conference on environmental systems.
Lukavský, J. (2014). Changes in boundary extension effect during spatial confinement. Vis. Cogn. 22 (7), 996–1012. doi:10.1080/13506285.2014.941966
Lungeanu, A., DeChurch, L. A., and Contractor, N. S. (2023). A tale of three teams: effect of long-term isolation in SIRIUS-21 on crew interpersonal networks. Acta Astronaut. 212, 617–623. doi:10.1016/j.actaastro.2023.08.015
Mao, X. W., Pecaut, M. J., Stanbouly, S., and Nelson, G. (2024). Oxidative stress, neuroinflammation, and the blood-brain barrier biomarkers on the brain response to spaceflight. Life Sci. Space Res. (Amst). 43, 22–28. doi:10.1016/j.lssr.2024.08.001
Mhatre, S. D., Iyer, J., Puukila, S., Paul, A. M., Tahimic, C. G. T., Rubinstein, L., et al. (2021). Neuro-consequences of the spaceflight environment. Neurosci. Biobehav Rev. 132, 908–935. doi:10.1016/j.neubiorev.2021.09.055
Miller, K. B., Mi, K. L., Nelson, G. A., Norman, R. B., Patel, Z. S., and Huff, J. L. (2022). Ionizing radiation, cerebrovascular disease, and consequent dementia: a review and proposed framework relevant to space radiation exposure. Front. Physiol. 25 (3), 1008640. doi:10.3389/fphys.2022.1008640
Mulavara, A. P., Peters, B. T., Miller, C. A., Kofman, I. S., Reschke, M. F., Taylor, L. C., et al. (2018). Physiological and functional alterations after spaceflight and bed rest. Med. Sci. Sports Exerc. 50 (9), 1961–1980. doi:10.1249/mss.0000000000001615
Nasrini, J., Hermosillo, E., Dinges, D. F., Moore, T. M., Gur, R. C., and Basner, M. (2020). Cognitive performance during confinement and sleep restriction in NASA’s Human Exploration Research Analog (HERA). Front. Physiol. 11, 394. doi:10.3389/fphys.2020.00394
Palinkas, L. A., and Suedfeld, P. (2008). Psychological effects of Polar expeditions. Lancet 371 (9607), 153–163. doi:10.1016/s0140-6736(07)61056-3
Parisi, M., McTigue, K., Wu, S-C., Karasinski, J., Panontin, T., Landon, L., and Vera, A. (2024). Advances in Human Factors of Transportation, 148, 49–57. doi:10.54941/ahfe1005194
Paromita, P., Khader, A., Begerowski, S., Bell, S. T., and Chaspari, T. (2023). Linguistic and vocal markers of microbehaviors between team members during analog space exploration missions. IEEE Pervasive Comput. 22 (2), 7–18. doi:10.1109/mprv.2022.3232780
Preuss, N., Hasler, G., and Mast, F. W. (2014a). Caloric vestibular stimulation modulates affective control and mood. Brain Stimul. 7, 133–140. doi:10.1016/j.brs.2013.09.003
Preuss, N., Mast, F. W., and Hasler, G. (2014b). Purchase decision-making is modulated by vestibular stimulation. Front. Behav. Neurosci. 8, 51. doi:10.3389/fnbeh.2014.00051
Richter, C., Braunstein, B., Winnard, A., Nasser, M., and Weber, T. (2017). Human biomechanical and cardiopulmonary responses to partial gravity – a systematic review. Front. Physiol. 8, 583. doi:10.3389/fphys.2017.00583
Robinson, J. A., Waid, M. C., Korth, D., Rucker, M., and Renfrew, R. (2019). “Innovative approaches to using the international space station as a Mars transit analog,” in International astronautical congress. HQ-E-DAA-TN74078.
Scott, J. M., Downs, M., Buxton, R., Goetchius, E., Crowell, B., Ploutz-Snyder, R., et al. (2020). Disuse-induced muscle loss and rehabilitation: the national Aeronautics and space administration bed rest study. Crit. Care Explor 2 (12), e0269. doi:10.1097/cce.0000000000000269
Scott, J. M., Warburton, D., Williams, D., Whelan, S., and Krassioukov, A. (2011). Challenges, concerns and common problems: physiological consequences of spinal cord injury and microgravity. Nature 49 (1), 4–16. doi:10.1038/sc.2010.53
Sishc, B. J., Zawaski, J., Saha, J., Carnell, L. S., Fabre, K. M., and Elgart, S. R. (2022). The need for biological countermeasures to mitigate the risk of space radiation-induced carcinogenesis, cardiovascular disease, and central nervous system deficiencies. LSSR 35, 4–8. doi:10.1016/j.lssr.2022.06.003
Stankovic, A. S., Pryputniewicz, A., Holder, S., York, S. P., Handley, P. M., Karasinski, J. A., et al. (2023). Longitudinal impacts of simulated long-duration spaceflight missions on operationally relevant measures of human performance using a portable simulation platform. Hum. Factors 65 (6), 1130–1141. doi:10.1177/00187208221113629
Sundblad, P., Orlov, O., Angerer, O., Larina, I., and Cromwell, R. (2016). Standardization of bed rest studies in the spaceflight context. J. Appl. Physiol. (1985) 121 (1), 348–349. doi:10.1152/japplphysiol.00089.2016
Theriot, C. A., Bell, S. T., Anderson, S. R., Dev, S. I., Oswald, T., Smith, S. M., et al. (2024). “Standard measures in analogs of spaceflight,” in NASA human research program investigators workshop (HRP IWS).
Walton, M., Norcross, J., Sanders, R., Myers, S., Newby, N., and Ross, S. (2023). “Extravehicular activity on the lunar surface: mapping mitigation Risk consequence for crew needing assistance or rescue,” in 12th international association for the advancement of space safety (IAASS) conference.
Whitmire, A., Leveton, L., Broughton, H., Basner, M., and Kearney, A. (2014). Minimum acceptable net habitable volume for long-duration exploration missions subject matter expert consensus session report. Houston, TX: NASA. JSC-CN-32284.
Willey, J. S., Britten, R. A., Blaber, E., Tahimic, C. G. T., Chancellor, J., Mortreux, M., et al. (2021). The individual and combined effects of spaceflight radiation and microgravity on biologic systems and functional outcomes. J. Environ. Sci. Health C Toxicol. Carcinog. 39 (2), 129–179. doi:10.1080/26896583.2021.1885283
Wu, S.-C., and Vera, A. H. (2019). Supporting crew autonomy in deep space exploration: preliminary onboard capability requirements and proposed research questions. Mountain View, CA: NASA Ames Research Center. NASA/TM–2019–220345.
The images in Figures 1, 2 show NASA’s Human Exploration Research Analog (HERA) facility, which is located at the Johnson Space Center. The HERA facility is a unique research environment designed to simulate the isolated, confined, and resource-limited conditions astronauts would experience on deep-space missions, such as those to Mars or other distant celestial bodies. Let’s break down each component visible in the figure:
1. Main Habitat Structure: The central part of the HERA facility is a cylindrical habitat structure that is multi-leveled. This structure is designed to resemble the interior space and layout of a spacecraft or space station module. The upper level could be used for sleeping quarters, workstations, or storage, while the lower level contains more accessible living and operational areas.
2. External Staircase and Ramps: There is a staircase and a ramp leading up to the entrance of the habitat. This setup allows easy access to the simulated spacecraft interior, supporting ease of movement for crewmembers entering or exiting the habitat.
3. Entrance and Airlock Simulation: The primary entryway mimics an airlock, complete with a secure hatch door. This feature is essential for simulating space missions, where crewmembers would need to move between the habitat and exterior environments through airlocks to maintain internal pressure and safety.
4. Hygiene Module: To the right of the main habitat, there is a “Hygiene Module.” This module would typically include bathroom and shower facilities, designed to allow the crew to maintain hygiene in an isolated environment. The module’s separate designation and connection to the main structure indicate the modular nature of the habitat, reflecting the compartmentalized design of spacecraft to manage different functions.
Observation and Control Station: Adjacent to the habitat, there’s an area with equipment and a console where a researcher or technician monitors the habitat. This control station is essential for supporting the simulated environment, allowing researchers to monitor and control the habitat’s internal conditions, crew behavior, and experiment parameters.
Surrounding Environment: The entire HERA habitat is contained within a large facility at the Johnson Space Center. The walls of this facility are lined with reflective or insulated material, to control temperature or provide soundproofing for the experiments conducted inside. The reflective walls contribute to creating an isolated environment, helping replicate the conditions of a spacecraft away from natural light and sound.
More details regarding HERA can be found at https://nspires.nasaprs.com/external/viewrepositorydocument/cmdocumentid=961141/solicitationId=%7B467D3677-A1E4-7369-3FF2-E0FE15E7B326%7D/viewSolicitationDocument=1/June%202023%20NRA%20HERA%20Study%20Information%20Package.pdf.
Keywords: human space exploration, analog research environments, analog research fidelity, behavioral health and performance, space psychology
Citation: Rahill KM, Mulavara AP, George K, Gore BF and Whitmire AM (2025) Increasing fidelity in lunar and martian analogs for behavioral health and performance research. Front. Space Technol. 6:1505823. doi: 10.3389/frspt.2025.1505823
Received: 03 October 2024; Accepted: 28 February 2025;
Published: 02 April 2025.
Edited by:
Antonio Mattia Grande, Polytechnic University of Milan, ItalyReviewed by:
Carole Tafforin, Independent Researcher, Ethospace, FranceCopyright © 2025 Rahill, Mulavara, George, Gore and Whitmire. This is an open-access article distributed under the terms of the Creative Commons Attribution License (CC BY). The use, distribution or reproduction in other forums is permitted, provided the original author(s) and the copyright owner(s) are credited and that the original publication in this journal is cited, in accordance with accepted academic practice. No use, distribution or reproduction is permitted which does not comply with these terms.
*Correspondence: Alexandra M. Whitmire, YWxleGFuZHJhLm0ud2hpdG1pcmVAbmFzYS5nb3Y=
Disclaimer: All claims expressed in this article are solely those of the authors and do not necessarily represent those of their affiliated organizations, or those of the publisher, the editors and the reviewers. Any product that may be evaluated in this article or claim that may be made by its manufacturer is not guaranteed or endorsed by the publisher.
Research integrity at Frontiers
Learn more about the work of our research integrity team to safeguard the quality of each article we publish.