- 1University of Urbino Carlo Bo, DISB – Biomolecular Sciences Department, Urbino, Italy
- 2INSERM U970 Paris Centre de Recherche Cardiovasculaire (PARCC), Paris, France
- 3Department of Pharmacology, Hôpital Européen Georges Pompidou, Assistance Publique Hôpitaux de Paris, Université de Paris, Paris, France
- 4Assistance Publique des Hôpitaux de Paris, Hôpital Européen Georges Pompidou, Pharmacology Unit, Paris, France
The space environment is incredibly hostile, and humans are vulnerable in such conditions. Astronauts encounter various stress factors during a space journey, including radiation, microgravity, forceful acceleration during launch, altered magnetic fields, and confinement. These stressors significantly impact the human body homeostasis, leading to physio-pathological adaptations, loss of bone density, muscle atrophy, cardiovascular deconditioning, alterations in liver function, vestibular adaptations, and immune system dysregulation. These alterations can potentially influence drug pharmacokinetics and pharmacodynamics, affecting the efficacy and safety of medications administered to astronauts. Due to the limited number of studies on pharmaceuticals conducted in microgravity conditions, it’s challenging to assess the effectiveness and stability of these medications during spaceflight. The objective of the present work is to compare the state-of-the-art knowledge on PK/PD changes and factors likely to affect them during spaceflight, with the subjective perception of the problem by a collection of separate interviews conducted with seven experts in the field. The interviewees were chosen as “experts,” i.e., representatives in a specific discipline, who possess knowledge and experience in space pharmacology, physiology, or biology. Thus, our panel included astronauts, space surgeons, and scientists aiming to bridge the lack of experimental data in the literature. Each interview explores assorted aspects of space physiology and pharmacology, including drug use and storage onboard the ISS; notable consideration has arisen regarding the current research gaps and future space expeditions. All the interviews were held remotely using online conferencing software. None of the interviewees could provide a comprehensive overview regarding potential changes in drugs PK/PD in microgravity conditions. Further, any medication brought on board (whether as part of an astronaut’s medical kit or stored in the ISS pharmacy) is destroyed, thereby suppressing the possibility of analyzing any degradation products resulting from long-term exposure to microgravity and radiation. According to these results, the use of drugs without understanding how they are genuinely absorbed, distributed, metabolized, and excreted in microgravity conditions is concerning, posing risks for drug effectiveness. Conducting genotyping and phenotyping on astronauts would be beneficial for developing personalized pharmacological countermeasures for each astronaut and anticipating expected drug metabolism changes during space missions.
1 Introduction
Human health faces significant risks in the context of space travel. Since NASA (National Aeronautics and Space Administration) is launching the Artemis Program to bring humans back to the Moon, medication will be essential in maintaining human health in space. These exploration missions will differ from the current Low Earth Orbit (LEO) missions, including significantly reduced habitable space for astronauts, smaller cargo, communication delay, and lack of rapid emergency medical return (Dello et al., 2022; Blue et al., 2019a). In this context, including pharmacotherapy becomes imperative for effective risk management (Figure 1), enabling the sustained presence of humans in space and on the Moon’s surface.
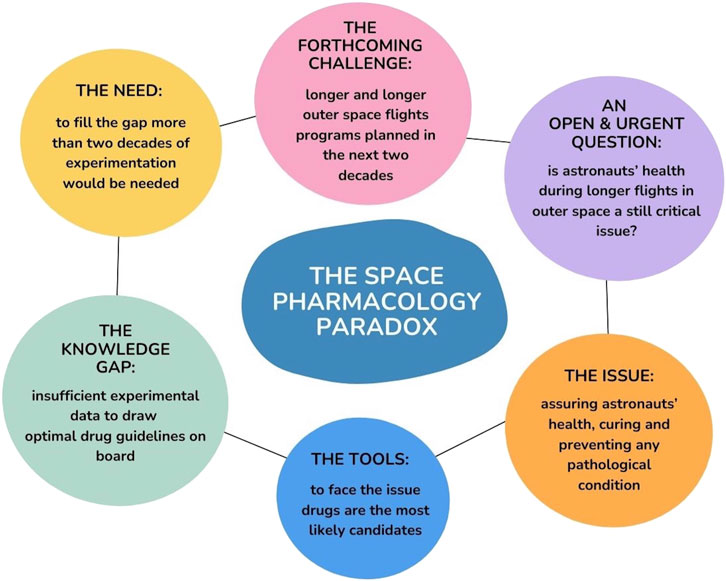
Figure 1. Graphical abstract of the space pharmacology paradox: including the most important open questions, issues, gaps, and the necessities to bridge these gaps, along with future challenges.
Astronauts encounter various stress factors during a space journey, including cosmic radiations, microgravity, strong acceleration during launch, altered magnetic fields, mechanical vibrations, pressure changes, close environment, isolation, and distance from Earth. In addition, the human body shifts dynamically across different time points, including pre-flight training, launch, extravehicular activity (EVA), and post-flight recovery (Cope et al., 2022; Demontis et al., 2017). This combination of stressful stimuli leads to several pathophysiological consequences affecting all organs and systems, including (but not limited to), negative calcium balance with loss of bone density, muscle atrophy, cardiovascular and hematic changes, vestibular adaptations, immune system dysregulation, gastrointestinal disturbances, metabolic and endocrine changes, and sleep disorders. Furthermore, astronauts must face noise, less sanitation, impaired circadian rhythm, and dietary modifications (Demontis et al., 2017; Eyal and Derendorf, 2019; Kast et al., 2017). These modifications are generally called physiological adaptations, however, their acute effect may mimic diseases, and since reversibility is not warranted, they are on the verge of being called deadaptations. For remaining factual, we shall call them space-induced changes (SIC). All SIC may change drugs’ pharmacokinetics (PK)–how the body acts on the medication–and, eventually, pharmacodynamics (PD)–how medication acts on the body. Indeed, changes in fluid shifts might impact the absorption and distribution of administered medications, thereby drug concentrations. Changes in the quantity or function of enzymes involved in drug transport and metabolism could affect drug exposure, affecting the effectiveness or severity of adverse effects. Furthermore, tissue remodeling could alter the expression of drug targets, potentially influencing therapeutic outcomes and expectations (Dello et al., 2022; Blue et al., 2019a; VirginiaE and Smith, 2020). Despite SIC and potential pharmacological modifications, drugs are used on board the International Space Station (ISS) as they do on Earth. In fact, pharmaceutical research has not been regarded as a priority. Without considering these potential alterations in drugs PK/PD during spaceflight, there is a significant risk of compromising drug efficacy and safety, jeopardizing the crew’s health (White, 1998).
There is a lack of studies in the literature addressing drug absorption, distribution, metabolism, and elimination in a microgravity setting. Moreover, investigating the correlation between liver/metabolic changes correlated to drug metabolism during space flight remains incomplete. This lack of knowledge stems typically from logistical and methodological impediments, arising from the requirement for serial sampling and the extensive storage of fragile samples (Derobertmasure et al., 2023). To date, only a few in-flight studies (Supplementary Table S1, Table adapted from Derobertmasure, 2022) have been conducted (Polyakov et al., 2022; Cintrón et al., 1987a; Cintrón et al., 1987b; Kovachevich et al., 2009; Boyd et al., 2009), most of which have used salivary sampling as a detection method rather than blood samples due to the reasons mentioned above. It is worth pointing out that salivary sampling is a flawed method to study PK/PD due to the unpredictability of drug transfer crossover into saliva and the lack of correlation between salivary concentration and plasma concentration (Blue et al., 2019a). Moreover, using saliva to measure drug concentrations could introduce additional variability and potential inaccuracies (Eyal, 2020). Nonetheless, given its simplicity and non-invasive nature, salivary samples have been mainly used for evaluating drug PK in crewmembers during space missions (Blue et al., 2019a; Cintrón et al., 1987a). Additionally, another constraint adding complexity to the data interpretation in these studies is the limited number of subjects, and the variability in sampling time across mission days, routes of administration, formulations, and dietary intake (Eyal, 2020). Moreover, a notable inconsistency exists when comparing studies focusing on the same medication. Thus, we cannot draw significant conclusions.
Due to the limited frequency of space flights and challenges in conducting experiments on board of spacecrafts, scientists employ terrestrial models to simulate weightlessness. The frequently employed model involves head-down bed rest, mimicking the bone and muscle losses as well as the fluid shift from the lower to the upper body observed in astronauts (Dello et al., 2022; Blue et al., 2019a; Gandia et al., 2006). Indeed, bed rest is recognized as the model of choice for simulating microgravity on the ground. Participants are required to lie in a bed tilted towards the head end (typically at 6° below the horizontal) for a duration spanning 5–60 days. All daily activities are carried out in bed, including eating and showering (European Space Agency - Bedrest Studies, 2024). There has been some inconsistency in drug PK studies using the head-down tilt bed rest model, which might be attributable to the medication’s properties and the lack of standardized protocols (Dello et al., 2022). It should also be stressed that one of the most significant gaps in PK research is the absence of in-flight validation of the bed rest model. Thus, a common point among these results is challenging to identify. Additionally, it's important to highlight that no studies validating the bed rest model as a suitable analog to spaceflight are available. However, the pharmacokinetics of numerous medications, including antibiotics, anesthetics, pain relievers, and anti-motion agents, were studied primarily utilizing the bed rest model (Polyakov et al., 2022; Gandia et al., 2006; Gandia et al., 2003; Schuck et al., 2005; Saivin et al., 1995). Supplementary Table S2 (Table adapted from Derobertmasure, 2022) provides an overview of some studies carried out in bed rest.
Few studies have been conducted regarding astronauts’ drug assumptions. A decade-long retrospective analysis of medication usage data was performed, including 24 crewmembers who participated in 20 space missions lasting over 30 days. Data highlighted that commonly utilized medications aboard the ISS addressed sleep disturbances, pain, congestion, and allergies (Wotring, 2011). More recently, Wotring and Smith’s study reported 5,766 medication use entries, with an average of 20,6 ± 8,4 entries per subject per flight week (VirginiaE and Smith, 2020). This study collected medication usage data throughout the entire duration of spaceflight missions undertaken by six astronauts and the initial week following their return to capture medication usage associated explicitly with the landing phase. They designed an iOS application to gather information on medication use, such as medication name, dose, frequency, indication, perceived efficacy, and side effects. It is essential to underline that only five subjects concluded the study because one subject did not complete the ground control data collection. As highlighted in previous studies, common drugs used during spaceflights were sleep medications and pain relievers. Moreover, subjects noted, on average, a modest increase in their medication intake during spaceflight compared to their usage while on Earth (VirginiaE and Smith, 2020).
Considering the presently available data, we can’t answer the question: “What impacts does spaceflight have on pharmacokinetics or pharmacodynamics?.” Meaning that it is mandatory to start conducting concrete, well-formulated studies in microgravity with a standardized protocol. Concurrently, monitoring the use of medications on board the ISS is compulsory, both to assess commonly used drugs and to evaluate perceived effectiveness or possible side effects that could arise. The present work objective is to confront the state of the art knowledge on PK/PD changes and factors likely to affect them during spaceflight, with the subjective perception of the problem by interviews conducted with seven experts in the field. This experience-based interview approach allowed us to provide a closer view of the field of space pharmacology on the eve of challenging spaceflight programs.
2 Materials and methods
To bridge the lack of in-flight pharmacological data, we interviewed seven international experts in the field. Purposive sampling was used to identify the interviewees. Email invitations for recruitment were sent out to potential participants, selected through a process of iterative identification within professional and personal networks. Our panel included astronauts (n = 1), space surgeons (n = 1), and international scientists active in the field of space pharmacology (n = 4), and space biology (n = 1). The authors emphasize that the data presented herein, notably the interview recordings and transcripts, were extracted from a master’s thesis (Barchetti, 2024).
All the interviews were conducted using online conferencing tools (Google Meet or Microsoft Teams), recorded, transcribed, and subjected to a comprehensive thematic analysis. Supplementary Table S3 provides an overview of the structured questionnaire (total question number = 74), which has been customized based on the background of each interviewee. In the present work, only the most salient answers of the various interviewees have been reported. Interviewee 1 (I.1) did not respond to Q. 3 (“Can you outline the medications that are frequently used onboard the ISS?”) as it involved some confidential information. Thus, we referred to the current literature available on this topic (VirginiaE and Smith, 2020; Wotring, 2015). The last 2 interviewees (I.6/7) were interviewed together, with the last interviewee (I.7) being a PhD student of Professor I.6.
Figure 2 provides an overview of the main themes examined in the interviews. In particular, the questionnaire included:
• 22 questions (30% of the total amount) focused on the primary medical challenges encountered by astronauts (e.g., bone loss, cardiovascular deconditioning, space motion sickness, etc.,) along with the pharmacological and non-pharmacological countermeasures presently used to mitigate or address these issues;
• 14 questions (19%) regarding the main pharmacological issues/gaps, including potential PK/PD changes, drug side effects, polypharmacy, drug-drug interactions, drug-food interactions, drug formulations, radiation protection, and pharmacological studies in space;
• 13 questions (18%) focused on future perspectives and the role of pharmacological therapy in upcoming lunar and Martian space missions;
• 9 questions (12%) regarding drug usage onboard the ISS (including both pre- and post-flight periods);
• 6 questions (8%) dedicated to the astronaut’s personal experience onboard the ISS;
• 5 questions (7%) concerning the storage and management of medications onboard the ISS;
• 5 questions (7%) dedicated to specific research outcomes conducted by the interviewees.
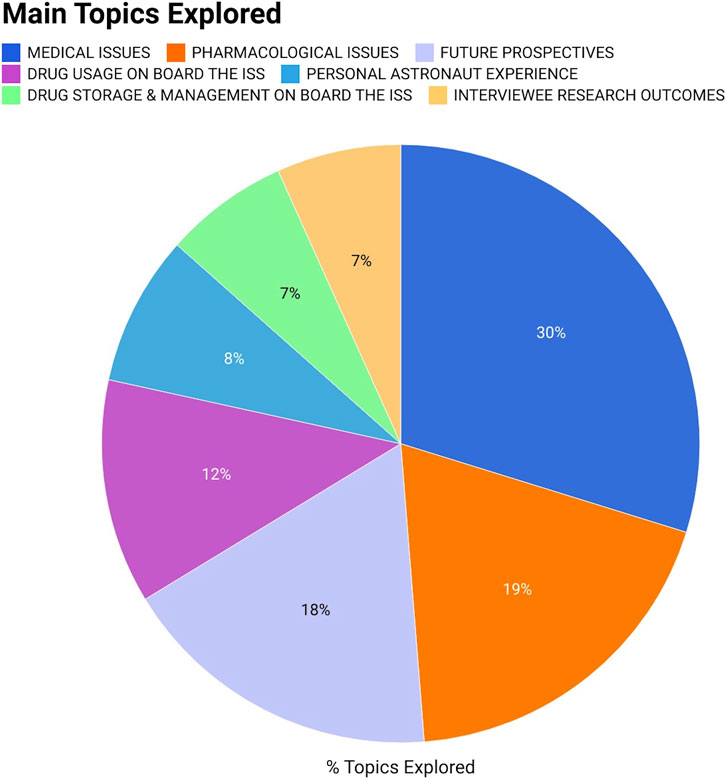
Figure 2. Graphical representation in percentage of the various topics addressed during the interviews. Specifically, the questions asked included: 22 questions on medical issues and related pharmacological countermeasures; 14 questions regarding the main pharmacological issues/gaps (including potential PK/PD alterations, drug side effects, polypharmacy, drug-drug interactions, drug-food interactions, drug formulations, radiation protection, and pharmacological studies in space); 13 questions on future perspectives and the role of pharmacological therapy in upcoming space missions; 9 questions regarding drug usage onboard the ISS (including pre- and post-flight periods); 6 questions dedicated to the personal astronaut experience onboard the ISS; 5 questions concerning the storage and management of medications onboard the ISS; 5 questions concerning the outcomes of research conducted by the interviewees.
It should be stressed that the information reported from the interviews are subjective, or data from studies conducted by the interviewees, and does not represent the official positions of the various Space Agencies (ESA and NASA in this context).
3 Results
In order to understand the origin and potential impact of the nearly total absence of experimental data in the literature, this work seeks to provide insights obtained from interviews with seven international experts in the field. The interview with a European Space Agency (ESA) Flight Surgeon (I.1), provided significant details about the major physiological issues encountered by astronauts and the use of drugs on board the ISS. The interview with a retired astronaut (I.2) aimed to collect a real-life perspective on how medications are managed and used in space on board the ISS. The third interviewee (I.3) offered substantial insights into managing medications at the Johnson Space Center (JSC) and the ISS. Further, the fourth interviewee (I.4) emphasized aspects of astropharmacy and personalized medicine in space. The fifth interviewee (I.5) addressed the issue of preventing structural, energetic, and genomic cellular stress conditions in the space environment. Finally, the last interviewees (I.6/7) provided a comprehensive overview of the cardiovascular health among astronauts, emphasizing the need to develop new protocols for studying drugs’ PK and PD in microgravity conditions. The key findings from the conducted interviews can be summarized as follows:
• None of the interviewees were able to provide a comprehensive overview regarding potential changes in PK and PD in microgravity due to the paucity of available literature.
• A new approach is needed for studying PK parameters in microgravity.
• An “open pharmacy” is available on the ISS, no medication is kept locked under a code, and a crew member is temporarily certified as a pharmacist.
• Any medication brought on board (whether as part of an astronaut’s medical kit or stored in the ISS pharmacy) is destroyed and not returned to Earth.
• Monitoring of medication usage on board is insufficient and this highlights the importance of the pharmacist’s role within the space medical team.
• ESA and NASA have adopted two different approaches regarding the medical kits for future missions (to the Moon or Mars).
The perspectives provided by multiple researchers in the field, along with the direct testimony of an astronaut, aim to raise awareness of the scientific community toward a deeper focus on the topic of space pharmacology, including the use and management of drugs in space. Medical-pharmaceutical research should be encouraged, particularly in anticipation of the future Lunar and Martian expeditions planned by ESA, NASA, and other Space Agencies. These missions will differ from current ones (LEO missions), particularly regarding mission duration, lack of resupply capability, prolonged exposure to space radiation, and absence of immediate medical emergency return. Considering these circumstances, it will be necessary to adopt new measures in healthcare assistance to ensure the crew’s safety.
The following sections contain only the interviewees’ most significant answers.
3.1 Interview with an ESA flight surgeon (I.1)
Q. 2 How are drugs managed on board the ISS? Do astronauts have free access to the medicines stocked on board?
“Yes, they have. People need to understand that astronauts normally perform a six-month mission. It’s like living in a little village, and you can have the normal medical events you would have in any normal human. […] Astronauts can use a pharmacy on the ISS for regular medications. There’s a section that needs permission usage, but there’s nothing locked under a code. […] There is, of course, written something like “restricted medication - call your flight surgeon before using” […]. They also have medical kits to use for their personal medication.”
Q. 4 Space motion sickness (SMS) is one of the most common conditions associated with spaceflight and generally occurs during the first days of flight. What drug do astronauts use for this type of problem?
“Yes, SMS happens in some mild form in 70% of astronauts, 30% would get vomiting, and a very minor percentage do become incapacitated and need to inject parenteral medication. The usual drug that we use, also before the launch, is meclizine […]. Promethazine is available on board as oral suppositories and injectables in case things go very bad, and it’s considered a rescue medication. Promethazine is an old antihistaminic with many side effects, so we use it very carefully to block a situation where the astronaut cannot stop vomiting. So, when an astronaut uses promethazine, they have to be very mindful of the side effects and, therefore, know that at that point, that crewmember will be “useless” and need to go to sleep. […] All the drugs that they would use as a regular use, they tried before. We can talk about a tailor-made basis: if the astronaut tries the medication and doesn’t feel any decrement performance, they can take it. Of course, there is a recommendation to avoid sedative medications also before launch, but it’s not forbidden.”
Q. 6 Sleeping medications have been used to manage sleep disruption during spaceflight from the beginning of the space program. What are the main reasons for this use (for instance, noise), and what drugs do ESA astronauts use to promote sleep?
“[…] First, sleep medication use is necessary because they don’t have a good circadian rhythm. This is due to the absence of the regular light-time pattern. There is this rule of switching off lights on the Station at certain hours […] We tried to keep the circadian rhythm in terms of lighting, but it was not easy. The second thing is that there is an increase in vehicle traffic. The Station is full of people, and supplies need to arrive. So, orbital dynamics dictate when the vehicle docks at the Station, and they do it at very random hours. It is widespread during the mission to have 2/3/4 sleep shifts, where during the three previous days, you start to wake up earlier, earlier, and earlier, so, in advance of a docking that will occur for instance in the middle of the night. […].”
Q. 8/9 Is it possible for an astronaut to develop hypertension due to cardiovascular deconditioning and/or ageing? If yes, how do you treat these astronauts? One of the major problems during space flight is cardiovascular deconditioning. Data have shown increased vascular stiffness, decreased distensibility, and potentially increased venous thromboembolism risk. […] In your professional estimation, how could we handle this issue? Could drugs be used to prevent this situation?
“With the same approach we would take on Earth. Hypertension may be disqualifying, depending on the level, for selection at the beginning of your career. […] We track their nutrition and exercise and train them to do all the healthy habits to delay any cardiovascular appearance. […] You may have arterial stiffness or serial damage, but very few of our astronauts have had cardiovascular events. […] You can consider the use of drugs for this issue when you start doing very long-duration flights or if you start finding certain cardiovascular events. That would be to treat a minority of crewmembers because the majority do not have it. Why do I have to give a drug for something that does not cause me a problem? […].”
Q. 13 Are these types of exercises sufficient to prevent loss of bone mass and muscle mass?
“No, they are insufficient to keep it equal than before, but they are more than enough to keep it within reasonable limits […]. Exercise is beneficial, what we don’t know is which part is more beneficial. Each crew member exercises 12 hours a week, and now the question is: could we do half of it? Could it be sufficient? Well, that’s a challenging question to answer, so what is the correct dose of exercise? We don’t know exactly. The Sprint study, which was just published, was about doing sprints instead of routine exercise to see if it could reduce the amount of exercise, and it could.”
Q. 15/16 The future Lunar Gateway and Artemis missions beyond LEO will demand increased autonomous decision-making and activities from astronauts, considering the delays in communication and evacuation […]. Is there a likelihood of increased drug usage during this type of mission? Does the space available on board pose any concerns or challenges? What type of medical kit will be necessary on the Lunar Gateway, and how will it differ from the current one on board the ISS?
“I don’t know if there will be a likelihood of increased drug use. But the problem is space: the ISS is a “luxury boat”. […] The missions to the Moon will be in a tiny spacecraft that may get a few kilos of medical supplies. […] This type of mission will require a more accurate risk balance. It is not as huge as it was in the Apollo missions, where you can find huge chances of dying, which is harsh, so we’re not going to send people on those chances. […] Volume and mass for these missions are a big challenge. […] Which are the most important drugs? […] If you start thinking of the most important drugs, you don’t know where to put the limit. In the same way, if you think of the commonly used drugs onboard, you will go on the Moon only with aspirin, ibuprofen, paracetamol, and sleep medication. […]
NASA has handled the most common and most likely conditions based on the current experience. NASA takes the approach of doing a risk assessment of what they have seen on the ground, what they have seen on ISS, and what they may encounter (for instance, lunar dust or more radiation). They are using a risk-based-drug approach. ESA is taking another approach, and we hope to merge both (most used and most important drugs). Our approach does not look at what pathology is there, but what are the common denominator to all pathologies that can make me the smallest kit possible. […] Now the problem is: for how long? When will you stop the treatment? […]
Both approaches lead to a risk. […] It is a complex dilemma for which we don’t have a clear solution. But 1 day, we will have to take this decision and take the risks. I hope we will make the right decision.”
3.2 Interview with a retired astronaut (I.2)
Q. 3 How frequently did you confer with your flight surgeon in a week? Do you consult the flight surgeon for medication recommendations, or do you have certain autonomy to make that choice independently?
“Every week, we hold a video conference with a doctor to tell him how it’s going on board. These video conferences are confidential. […] There are categories of medications that are restricted to being only able to be administered by a doctor. Those are on the space station. According to US laws, you cannot even touch this medication. […] Only a certified pharmacist can give you restricted medication. So, what happens during the period when you are in the Station? There is somebody on the crew who is temporarily certified as a pharmacist. If the doctor tells them to give you a controlled medication, this is like writing a prescription. […].”
Q. 4 Have you encountered any issues throughout your three space missions?
“One of the things that I suffered through the mission was eye dryness. […] I had a really bad sensation of dry eyes, […] and I suffered quite a lot. I tried to use drops, I tried to use everything, but I could never clear up the situation. We never understood why I was having this dryness. They asked me to use an eye ointment and ophthalmic antibiotic, I did it, but it did not solve the problem. We don’t know what happened. […] When I came back to Earth it resolved spontaneously.
[…] Something was definitely not right with my eyes. For example, I had, at a certain point, a really high intraocular pressure, and this was absolutely abnormal. We have this measurement periodically, and the doctors found out that I had a really high intraocular pressure. This is kind of interesting because I don't suffer any of these problems on Earth. […].”
Q. 5 Did you encounter difficulties performing your tasks on board the ISS due to this eye dryness?
“Well, I would say that, besides that, my eyes were burning constantly. There was a point where I had burning eyes by the end of the day. So, it was not easy even to read or do something by the end of the day […].”
Q. 6 Although there is a drug or medical specialist in flight with you, before leaving Earth, do you receive any training on the use of auto-medication or the use of over-the-counter medications?
“I would say that we haven’t received anything specific about this, and at the same time, I think we are capable enough of exchanging information. What happens is that before the flight the crew surgeon will ask you, what kind of medication do you want? And this time, you are supposed to mention what you want, even if you take some regular medication […]. And that will give you enough to sustain two or three weeks of capabilities without touching the official medicine inventory. Because touching the official medicine inventory is a pain in the neck. As soon as you touch it, some medicines are missing, so they must send it up. So, if they know you have a problem with something, they prefer to send you up with the medications that you need, without having to go and touch the other system. So, everybody flies with a little package of medicine. I still remember, for example, when the doctor asked me what medication I wanted, I said: “Nothing”. They looked at me very surprised. […] I use medications only if it’s absolutely necessary.
[…] An interesting thing is that, when you leave the Station, you need to clean everything you have brought up on the Station with you. If you used less medication than you brought, you can’t leave it there. You throw them away because otherwise things get out of control […].”
Q. 7 Have you ever tested any medications before the mission, in order to check for potential side effects?
“Yes. NASA will make you test all medications that are on board. […] They will let you try one dose of everything. They send you a form that you must fill in in case of any side effects. Therefore, once on board, you are aware of the medications that may have caused you any side effects.”
Q. 8 How does it feel to return to Earth after several months in microgravity? Did you experience drops in pressure or fainting?
“I didn’t experience anything that had to do with blood pressure drops, but I did have a serious … let’s call it instability problem, with vomiting and nausea. […].”
3.3 Interview with an expert in space pharmacology, and former NASA Johnson Space Center senior scientist (I.3)
Q. 1 What are the primary physiological alterations that could significantly affect drug pharmacokinetics in space, in your opinion?
“Who knows? And I would argue that my opinion on this point is of no value at all. We need some different models, and we need to get the right model to get the right data. […] What we need are some data”
Q. 3 Could you describe the storage protocol for medications on board the ISS? Is NASA repackaging all the drugs? What are the methods and frequency of sending medications to the ISS?
“Regarding repackaging, the Johnson Space Center (JSC) pharmacists have considerably cut back on this practice. They’re now using the original manufacturing packaging as much as possible. […] The medications that are repackaged are put into a small plastic container. It’s not very protective at all. But those and the manufacturer’s packaging are put inside a big, sturdy fabric bag with clear labels attached. The fabric provides good protection from light. There is no significant protection from humidity or oxygen in any of these.
At JSC, part of what they’re doing there in the pharmacy group, they monitor the expiration date, the lot number of everything they send up, and someone is tracking that. So sometime before a medication expires, […] they’ll start working on getting a new shipment sent up on one of the next resupply vehicles. When it arrives, the astronauts are directed to take the old medication and put it on one of the vehicles, like Progress, that burns up on reentry, along with all the other trash. So essentially, they throw it out, and it gets incinerated. […]
I got some (expired medications) returned to Earth once for a chemical examination. But that was a particular case. Usually, down mass on a vehicle, even from the space station, is extremely hard to come by […].”
Q. 5 Data have shown that two doses were taken in 19% of nights when sleep aids were used. Specifically, individuals woke up earlier than planned and took an additional sleep aid on the same night. […] How can individual crew members most effectively and safely use sleep and alertness medications before and during spaceflight?
“[…] they’re not recommended to take two doses the same night. But just like when a physician prescribes for one of his patients to have a sleep aid medication at home, that person may use it differently than they were told. […]. Briefly, it’s hard to sleep in space. We don’t know exactly why. So maybe we should try to fix whatever that is. You know, is it the lighting? Is it noise? Is it CO2 concentrations? Is it the workload? Is it the stress? Maybe we should fix that. I would much rather solve the problem that way than have them take any medicines.”
Q. 8 […] Some studies illustrate that the combination of exercise associated with Alendronate or Vitamin D may help protect bone health during a long-duration space mission. However, there may be situations where a crew member cannot exercise, for instance, due to injury, sickness, or even equipment failure. In this case, what would be your approach?
“This has been discussed many times and hasn’t happened yet. When the exercise equipment breaks, fixing it is the top priority. […]. But what would happen if someone couldn’t exercise? Alendronate has been validated in space. And I think that the data on it are very, very clear. It is not routinely used. A good diet and exercise are preferred over any medication. And the bisphosphonates have significant side effects […]. So, it probably would be a good idea to have another potential strategy for a countermeasure on a long-duration mission. But that has not yet been developed. […] A long-acting depot injection or some implant that releases the drug over time could be a possibility.”
Q. 10 Is physical exercise sufficient to prevent bone and muscle mass loss for long-duration exploration missions? Is there value in considering the use of medication, or do you believe that focusing on maintaining a healthy diet and incorporating supplements would be a more effective solution?
“Regarding the exercise, no, they won’t be sufficient. For the second question, I will say both. […].”
Q. 12 From your perspective, is there an insufficiency in ensuring adequate medication protection to maintain stability and shelf life during spaceflight? If so, how can we improve this protection?
“We have no protection against radiation, but we don’t have that for anything or anyone in space. So, there are technical hurdles to be solved. Shielding is not as easy as most people think. We need new materials, we need a new strategy, the radiation scientists have to figure that out.”
Q. 15 The future Lunar Gateway and Artemis missions beyond LEO will demand increased autonomous decision-making and activities from astronauts, considering the delays in communication and evacuation. Is there a likelihood of increased drug usage during missions like these? What kind of drugs will be needed for these kinds of missions?
“With the relatively short duration missions planned for Lunar Gateway, I think if there’s any change in medication use, it may be related to the tasks that the astronauts will be performing rather than other aspects of their mission, like the duration. Those missions are not planned to be lengthy, but they may involve more activities like mining or construction. So rather than an astronaut on an extravehicular activity, just stepping out into microgravity and doing some things on the Station with hand and power tools, they may be much more physically active. And using many more tools and being exposed to rocks and dust, the risk of traumatic injury, I think, will be much higher. So it may be that we have to treat a traumatic injury, and that hasn’t happened much with station missions. […].”
3.4 Interview with an expert professor in the field of astropharmacy (I.4)
Q. 1 What are the primary physiological alterations that could significantly affect drug pharmacokinetics in space?
“[…] I would say probably the liver and the kidney. I’m kind of worried about which one can remove the toxins. […] The next one I worry about is the kidney because we are also not good at understanding all the potential kidney alterations.”
Q. 6 Regarding the assumption of drugs, astronauts can consult their flight surgeons and have unrestricted access to a medication kit for self-administration. What concerns related to potential polypharmacy and potential drug-drug interactions could be noted in the current medical procedures for space travel?
“[…] I would anticipate that they won’t be taking drugs unnecessarily. But, as a pharmacist, I would feel concerned that there might be some overdosing concerns. There is a study that says that some astronauts have been double-dosing on sleeping pills on the same night. It’s not “addicted behavior,” but probably, the medicine is not being effective. That’s why it becomes a bit dangerous because you don’t know how the body is processing the drug. I think it could lead to overdosing without knowing. […] In addition to drug-drug interactions, we also need to consider, and it gets more complicated, drug- food interactions and drug-microbiome interactions as well. […] So, personalized medicines are the way to go […].”
Q. 7 Regarding the drug-related stability on the ISS, several studies demonstrated formulation failures in chemical potency and physical standards after space storage. What potential issues could arise, and what measures would you employ to resolve them?
“The potential issues are medicines not having the therapeutic effect and or having toxic compounds. What would you employ to resolve them? It’s a much more complicated question. We need stability studies in the space environment at the fundamental level, so there are a few sound studies. Some studies were supposed to study stability, but this didn’t happen for various reasons, such as funding. Once we know what is causing them to be destabilized or how fast they are degrading, whether it’s the vibration or because they are unpacked from their original package. Then we can think about a proper solution. A standard solution that people always mention is to print medicine when needed, but the technology is not quite ready yet. And even if that technology is ready, how can you ensure its quality? How do you regulate it? Because you need something to say that this is the right quality of medicine, and then who will regulate the manufacturing? Who’s going to check if the machine is sterile? So, I don’t have a perfect solution for stability. […] If we consider the ISS, drug stability is not a big problem because the resupply is easy. I think the question should be further away.”
Q. 9 The future missions beyond LEO might include medication-related challenges such as errors in medication use, adverse effects, therapy failure, improper storage conditions, and a lack of medication supply. How should we manage medication to ensure the crew’s safety in future missions? Which types of medications will be required for upcoming lunar expeditions?
“I think we are not even there at the first step for this type of mission. First of all, the healthcare team has many doctors, but there are no pharmacists. The first step is not having one pharmacist because the person will be overwhelmed with many things. I think there should be a team of pharmacists in every medical team. […] All healthcare professionals should have some representation. […] Our voices (pharmacists) are not there. […] As pharmacists, we should also consider space tourists who may want to go to the Moon for two weeks, as well as commercial workers. I think these people will need a different consideration, and not just medicines but in terms of healthcare.”
3.5 Interview with a professor of molecular biology (I.5)
Q. 1 You conducted a study aboard the International Space Station known as the Endothelial Project. What were the motivations behind this investigation, and what specific outcomes did you aim to achieve?
“The motivation of the study was based on some observations made on astronauts upon their return to Earth from space mission and on a body of literature describing results on animal models of different types, showing that there is a suffering of the endothelium of small caliber blood vessels justifying an investigation to understand the reasons for this, let’s say, malfunctioning. In a few words, I could say that many of the health problems reported by astronauts could be associated with such malfunctioning of the endothelium, the molecular basis of which was unknown. […].”
Q. 3 Could you provide a more in-depth explanation of the results you’ve obtained?
“We noticed that microgravity and space radiation have a specific effect on cells: sometimes, this effect is coherent in the same direction […], and in some instances, they have a contrasting effect […]. We noticed that in space-flown cells, microgravity and space radiation had opposite effects, and the net effect was that autophagy was inhibited. Thus, during spaceflight in endothelial cells, autophagy is not working properly. We know that autophagy is an emergency system for the cell to respond to harsh environmental situations. […] This is very interesting because once you find specific pathways that you are interested in, you can try to find drugs that can interfere with them, knowing that you can interfere with a pathway related to the effect of lack of gravity or radiation. […].”
Q. 5 From your perspective, how could we prevent these structural, energetic, and genomic stress conditions (also thinking of future long exploration missions to the Moon or Mars)?
“[…] There are two ways to prevent these alterations: one way is the use of drugs, for example. We hope to find drugs that interact with autophagy or other pathways. We should use drugs to prevent the effect of Reactive Oxygen species (ROS), which are very dangerous to all the molecules of the cells and the body. […] In the future, a possible game-changer will be represented by hibernation or synthetic torpor. We have a project that is about to start, to study the metabolism of some tissues that have been exposed to space: hibernated animals are very much protected by radiation, and we don’t really know why. […].”
3.6 Interview with a cardiologist and pharmacology professor and a PhD student (I.6/7)
Q. 1 What significant cardiovascular alterations does an astronaut encounter during a space mission, and what implications do these changes have on the astronaut’s health? Could such effects worsen on the Moon or during long-duration space missions?
“[…] The longer you stay in microgravity, the more you experience muscle loss and a kind of shrinkage of the cardiovascular system, which becomes hypotrophic. You’ve got a decrease in the heart size and vascular dimensions. Because of the muscle loss, so you have a decrease in the metabolic demand by the muscles, meaning that the heart does not have to provide as much work to perform, and the arteries do not withstand the same level of flow, and the same level of blood pressure induced by exercise. […] Meanwhile, you also have vascular aging, with increased stiffness equivalent to the one we see during aging in humans on Earth. This increase in stiffness is equivalent to 20 years of aging, and we don’t know the reversibility of this phenomenon. If you take the whole picture, you have a heart and vessels de-adapted for physical exercise. At the same time, you’ve got an increase in stiffness, which is equivalent to aging, and we don’t know exactly the reversibility of this phenotype once you’re back on Earth […].”
Q. 2 One of the major problems during space flight is cardiovascular deconditioning. […] In your professional estimation, how could we handle this issue, and what countermeasures could we adopt to prevent this critical cardiovascular risk factor?
“We know that the actual countermeasures, which are physical exercise and nutritive countermeasures, are not sufficient to contract that is the deconditioning. […] Classical drugs, like angiotensin receptor antagonists or ACE inhibitors, have proven that they can improve stiffness independently from blood pressure changes. […] We also know that anti-diabetic drugs like metformin or dapagliflozin can improve arterial stiffness independently from blood pressure changes. So, these are good candidate drugs for preventing this issue. But for this, we need to know more about the pharmacokinetics and pharmacodynamics of these drugs during space flight, which is unknown.”
Q. 7/8 Your team developed an easy sampling method on Dried Urine Spot (DUS) to simultaneously quantify 5 anti-hypertensive drugs in human urine. What were the motivations behind this investigation, and what specific outcomes did you aim to achieve? Could this analysis method benefit future studies on drugs in space?
“We don’t have any preventive treatments for early vascular aging, and the pharmacokinetics in this area are lacking. So, there was an urgent need to develop a new pharmacology protocol […]. We set the hypothesis that microsampling and the technique of LC-MS could be a solution for developing pharmacologic studies based on DUS and DBS (Dried Blood Spot). The primary goal of this work was to validate this approach. […] With the DUS and DBS methods, this type of analysis can be done at home, it can be done anywhere, and you don't need to hospitalize the patient. With this method, we can determine whether the patient is truly exposed to the drug. I’m not speaking of adherence; I’m just speaking about exposure to the drug. I see big applications. […] It can be applied also during expeditions in submarines, every time you are in an isolated place or extreme environment with no contact, you can apply this technique. So, it can be a big game changer. We can say that space research could also be very helpful on Earth. Making a full pharmacokinetic profile of every patient that you have is difficult because it would be time-consuming, you would need a day of hospitalization, and you would need a lot of resources. In selected patients, those who have intolerance or resistance, you can test the pharmacokinetics of drugs and have a tailor-made treatment for them adapted to their profile.”
Q. 9 Orthostatic Intolerance is one of the major challenges an astronaut must face upon reentering Earth. Medical assistance may not be available for future missions beyond LEO. What solutions can be adopted in the future beyond LEO spaceflight?
“[…] For astronauts who are in good health, you can eventually use midodrine, a vasoconstrictor. You can imagine doing that on the Moon. But again, you need to test the pharmacokinetics and pharmacodynamic response beforehand to avoid having severe hypertension on the Moon, in addition to orthostatic hypotension. That’s something to be tested. […] For prevention, I believe that physical countermeasures, […] should go along pharmacological prevention.”
Q. 12 What pharmaceutical countermeasures are needed on a long-duration space mission, for instance, future Moon and Mars missions, and how will these countermeasures differ from those used today on the ISS? What pharmaceutical challenges would we face for this type of travel?
“[…] The drugs should be chosen with the most comprehensive therapeutic range; we should know their renal or hepatic elimination to predict the interaction amplitude. We should also see the effect of plasma protein binding on the distribution of the drugs. […]
I can tell you my wildest dreams. My dream is to have a full pharmacokinetic profile in astronauts before launch. […] So, personalized medicine based on the astronauts’ pharmaceutical profile, to have a new program in terms of which drug is the combination is the best. There will never be one drug alone, but a combination of drugs. So, I imagine that you can have a pill, a polypill with, let’s say, 75 mg of irbesartan, 500 mg of metformin, dapagliflozin, and hydrochlorothiazide. That could be a good start. Then, we need to supply this drug to the astronauts on the ISS. On the Moon or Mars, it will be much more complicated.”
4 Discussion
Insights from multiple researchers in the field were crucial for this research, which intends to encourage the scientific community and the Space Agencies toward a more intense exploration of space pharmacology. Various aspects were emphasized, including medication storage and handling protocol, the drugs most frequently used, and the primary health challenges encountered by astronauts. Additionally, several viewpoints on the future of space pharmacology were highlighted.
As previously emphasized, there is a substantial deficit of pharmacological data related to the space environment. This lack of knowledge regarding potential modifications concerning the PK of drugs during space travels (including alterations in absorption, distribution, metabolism, and excretion) could jeopardize the health and safety of astronauts instead of assisting in treating the condition. In response to the first question posed to I.3 concerning the primary SIC that could have a notable impact on drug pharmacokinetics in space, her answer was succinct: “Who knows?”. There is a notable gap in the literature regarding drug distribution, metabolism, or elimination in space. Moreover, there is no evidence of the distribution of active principles or their metabolites during and after spaceflight-induced fluid shifts. The complete investigation of the correlation between liver changes and medication metabolism during space flight remains incomplete. In addition, comprehensive research on the liver enzymatic pattern is deficient, which should also address the polymorphic aspect of metabolic pathways. Thus, we don’t have the data to define which SIC impacts which pharmacological parameters. Nonetheless, as the fourth interviewee I.4 noted in Q. 1, SIC in the liver and kidney could alter parameters like drug metabolism or excretion, leading to increased side effects or therapeutic failure. Indeed, experimental animals exposed to microgravity conditions have been reported to exhibit significant alterations in the hepatic content of metabolic enzymes from the CY450 family and other liver enzymes (Dello et al., 2022), including a reduction of catalase and glutathione (GS) reductase (Hollander et al., 1998), and significant CYP1A2, 2E1, and 2C29 content rises (Eyal and Derendorf, 2019). Thus, in a space setting, the increased levels of CYP2E1 combined with the reduction of GS reductase could lead, for instance, to severe liver damage after acetaminophen administration. Thus, SIC in the liver and kidney, combined with the lack of knowledge about the PK of drugs in microgravity conditions, could lead to “overdosing without knowing” (I. 4, Q. 6), leading to potentially toxic effects.
The lack of knowledge about the fate of drugs typically stems from logistical and methodological difficulties: collecting blood samples on the ISS is highly complex, and there are additional issues related to the storage, stability, and retrieval of the collected samples. Furthermore, the subject and relative sample numbers are small, leading to difficulties obtaining conclusive results. To address this challenge, it has been recently developed and validated a novel approach for the concurrent quantification of five antihypertensive medications: the dried urine spots (DUS) method (I. 6/7, Q. 7/8) (Derobertmasure et al., 2023). In the context of a PK study on the ISS, the astronaut will collect micro-urine samples with the help of a micropipette on a card. These cards will be stored on the ISS and transported to Earth for analysis by a pharmacology laboratory. The results show that DUS cards are robust in analyzing different hypertensive drugs across a wide array of storage conditions (Derobertmasure et al., 2023). With its minimal biological sampling volume, compact size, and extended stability, the DUS method will facilitate pharmacokinetic studies in remote environments, where traditional sampling and storage may be unfeasible. This method could therefore be a game changer in the context of pharmacological sampling onboard the ISS and future spacecrafts. This method can also be applied to blood (Dried Blood Spot) and has been validated during parabolic flight through a caffeine pharmacokinetic study campaign as a proof of concept (NCT: NCT06431984).
Moreover, two key aspects that emerged during the interviews need to be stressed: the astronaut’s ocular issue, and the double dosage of sleep aids (Barger et al., 2014). The use of medications by I.2 during his space missions on the ISS has been significantly limited: “[…] I still remember, for example, when the doctor asked me what medication I wanted, I said: “Nothing”. They looked at me very surprised. […] I use medications only if it’s absolutely necessary. […]” (Q. 6). Nonetheless, during his stay aboard the ISS, the astronaut suffered from severe eye dryness and elevated intraocular pressure: “One of the things that I suffered through the mission was eye dryness. […] I had a really bad sensation of dry eyes, […] and I suffered quite a lot. […] Something was definitely not right with my eyes. For example, I had, at a certain point, a really high intraocular pressure, and this was absolutely abnormal […]” (Q. 4). Is this experience a typical occurrence? Absolutely. Dry eye disease (DED) is the prevalent ocular condition encountered among astronauts. Symptoms of DED consist of sensations mimicking conjunctivitis. More than 30% of expedition crew members aboard the ISS and around 15% of shuttle crew members documented signs or symptoms of DED during their time in space (Ax et al., 2023). DED may be multifactorial, related to exposure to microgravity, radiation, or due to the peculiar environment of the Space Station. The astronaut’s answer to Q. 5 (did you encounter difficulties performing your tasks on board the ISS due to this eye dryness?) was negative, although his eyes were “burning constantly”. The interviewee also mentioned that eye burning was sometimes a limiting issue by the end of the day, impeding recreation and relaxing activities such as reading. How and how much these apparently benign issues may impact on astronaut wellbeing and performances - especially in long term missions - uncertain and unknown. Vision is crucial for any type of activity on board. Without a doubt, an astronaut struggling with vision or feeling constant eye discomfort could hamper the mission and jeopardize the crew’s safety. The lack of effectiveness of any pharmacological remedy (ranging from eye drops to antibiotic ointments, Q.4) in resolving this issue highlights the necessity for deeper exploration into space pharmacology and appropriate drug usage in space. Furthermore, an important fact shared by I.2 is the discovery of elevated intraocular pressure (Q. 4). Microgravity leads to the redistribution of fluid from the lower body to the head, leading to tissue swelling and increased intraocular and cranial pressure (Ax et al., 2023; Lee et al., 2020). The resultant changes in eye health have been identified as spaceflight-associated neuro-ocular syndrome (SANS) (Ax et al., 2023; Lee et al., 2020; Macias et al., 2020). The exact pathogenesis of SANS remains unclear, and multiple potential risk factors might cause it. Exposure to microgravity initially leads to elevated intraocular pressure, which subsequently gradually returns to baseline levels during extended space missions (Ax et al., 2023; Lee et al., 2020; Macias et al., 2020). We don’t know precisely when the astronaut experienced this increased intraocular pressure. What we can say is that, as emphasized by the astronaut himself, this type of problem only occurred during his stay in space, thus it should be causally linked to the spatial environment. A comprehensive understanding of the specific mechanisms driving these fluctuations in intraocular pressure during space travel is crucial for developing effective pharmaceutical countermeasures. In addition, several drugs commonly used on ISS have anticholinergic activities (anti-H1 particularly), and it is interesting that iatrogeny was not evoked as a possible cause of the eye problems of the astronaut.
The second crucial point to underscore is the notable example of improper drug usage that occurred with the reported use of 2 sleep medications on the same night by some astronauts (Wotring, 2015; Barger et al., 2014). As previously reported, and confirmed by the first and third interviewees, sleep aids are frequently used on board the ISS. The main reasons for this frequent use are altered circadian rhythm and an increase in vehicle traffic (docking) (I.1, Q. 6). As emphasized by numerous drug regulatory agencies, taking 2 doses of sleep aid on the same night is strongly discouraged. This is certainly not a case of addictive behavior (I.4, Q. 6); rather, the double dosage could be likely due to the medication not being effective. Thus, the possible double usage could be linked to a faster metabolism of the drug, leading to reduced bioavailability and a shorter duration of action. This aspect is worrying, especially considering the potential onset of severe side effects that could arise after a double dosing. Without understanding the precise PK/PD of drugs in space, a double dosage could lead to overdosing without knowledge. Nonetheless, asking for a pharmacist’s advice regarding this matter could warn the astronaut about the potential risks of using two medications–such as Zolpidem and Zaleplon - on the same night. Zolpidem and zaleplon are short-acting non-benzodiazepine sleep aids (Rang et al., 2016; Lemke et al., 2013), better known as Z-agents; they act binding to GABA-A, but in a more specific fashion as compared to benzodiazepines. Zolpidem and zaleplon are recommended for the short-term management of insomnia (Rang et al., 2016; Lemke et al., 2013): it has been demonstrated that both medications reduce sleep latency, and enhance the duration and quality of sleep, maintaining its physiology, while causing minimal impact on performance in the morning following their usage (Wotring, 2011; Rang et al., 2016; Lemke et al., 2013). A concern regarding sleep aids could be their simultaneous use during an onboard emergency. Certainly, emergencies can arise unexpectedly; thus, there’s a possibility of having an astronaut exhibiting signs of drowsiness after using these medications. The same scenario could occur with the utilization of promethazine or other antihistamines - drugs frequently used by astronauts in case of space motion sickness (SMS) symptoms. SMS medications are among the most used drugs, especially in the initial days of the flight (Wotring, 2011): “[…] The usual drug that we use (for SMS), also before the launch, is meclizine […] Promethazine is available on board as oral suppositories and injectables, in case things go very bad and it’s considered a rescue medication […]” (I.1 Q. 4). Promethazine – first generation histamine H1 antagonist – has been highlighted as the historically most used drug on board the ISS (Wotring, 2011; Wotring, 2015). In contrast, meclizine – histamine H1 antagonist – is preferentially utilized as an SMS treatment. Indeed, as reported by the flight surgeon (I.1), the use of promethazine at the lowest therapeutic dose is limited to cases of severe nausea and vomiting. The reason could lie in the fact that promethazine, as compared to meclizine, has a more pronounced sedative side effect (Southard and Al Khalili, 2024). Nonetheless, the use of promethazine or meclizine concomitant with sleep aids could lead to dangerous pharmacodynamic interactions. As first-generation antihistamine drugs, promethazine and meclizine can cross the blood-brain barrier, leading to sedative effects and drowsiness. Specifically, these two SMS medications have the potential to enhance, amplify, or extend the sedative effects of other central nervous system (CNS) depressants, including sedatives/hypnotics, tricyclic antidepressants, narcotics, general anesthetics, and alcohol (Rang et al., 2016; Lemke et al., 2013; Southard and Al Khalili, 2024). Therefore, the concomitant use of these drugs should be avoided.
An interesting and positive aspect is that astronauts have a weekly video conference with their flight surgeon. They also test all the medications available on board the ISS before departure (I.1, Q. 4 and I.2, Q. 7). Weekly video conferences are beneficial for tracking the astronaut’s medication usage, and the medication testing could be useful for assessing any allergies/side effects that may occur after medication administration. Nonetheless, is important to recognize that the pre-testing is well outside good clinical practices where a drug should be administered only if indicated. It could also lead to sensitization (first administration well tolerated and subsequent anaphylaxis). Lastly, the predictive value of a previous administration for subsequent intolerance is not validated.
Introducing pharmacokinetic tests during these pre-flight procedures would be crucial to assess the drug’s actual metabolism in each astronaut, thus facilitating a personalized treatment approach. At this point, we understand the pivotal role of personalized medicine in initiating the correct management and use of drugs in a space setting. In particular, the consistent collection of omics data is a valuable opportunity to personalize astronauts’ therapy. The exploration of astronauts’ genomic profiles before space missions and the introduction of protocols capable of evaluating the drug’s PK – for instance, the DUS or DBS method – in a microgravity setting have the potential to enhance personalized medicine approaches. These measures can assess risks and develop effective pharmacological countermeasures for future deep-space missions. Moreover, optimizing cargo is possible by including the most effective medications customized for each crew member, which reduces cost and minimizes the risks associated with adverse drug reactions or therapy failures. As also highlighted by I.4 (Q. 6) and I.6/7 (Q. 12), the implementation of personalized pharmacology and a tailored approach to prevent (or counteract) SIC in upcoming space missions is essential.
Taking a closer look at the storage protocol for medications on board the ISS, the flight surgeon (I.1) confirmed the presence of an open pharmacy onboard, where astronauts can access medications without direct authorization from their flight surgeons. A section needs permission usage, but nothing is locked under a code (Q. 2). At the same time, a noteworthy point that emerged during the interview with the astronaut is the medications’ dispensing: “[…] According to US regulations, only a certified pharmacist can give you restricted medication. So, what happens for the period when you are in the Station, there is somebody on the crew that is, temporarily certified as a pharmacist. […]” (Q. 3). Normally, the dispensation and supply of any medication on Earth are exclusively the pharmacist’s responsibility, who carries out this professional obligation and assumes the related responsibility. In this scenario, the temporary certification of a crew member as a pharmacist is a unique circumstance. At this point, we can already highlight two important aspects: 1) free access to drugs could lead to an increased risk of potential drug-drug, drug-food interaction, or incorrect drug usage. Consequently, this results in a higher likelihood of therapy failure and an increased risk of adverse effects. 2) The absence of a fully qualified pharmacist within the medical team. The temporary certification of a crew member as a pharmacist is not optimal. Consequently, we recommend the presence of pharmacists either on board or at least on the ground. In this respect, a pharmacist could potentially offer personalized guidance to each astronaut regarding the correct use of drugs, both for prescribed medications and for OTC (over-the-counter) drugs. As highlighted by I.4 “[…] All healthcare professionals should have some kind of representation. […] Our voices (pharmacists) are not there. […]” (Q. 9). Moreover, only a few studies have been conducted regarding astronauts’ drug assumptions (VirginiaE and Smith, 2020; Macias et al., 2020), not due to a lack of willingness on the part of researchers, but rather due to a lack of funding, and limited interest in the field of space pharmacology from the various space agencies. In this regard, data on tracking medication use, indication, efficacy, and side effects are almost entirely lacking. On this matter, having a pharmacist as part of the astronauts’ medical team could help in the collection and analysis of data on the frequency of drug administration and provide also crucial information regarding administration techniques, timing, and any precautions necessary before usage or particular side effects that may occur. Further, a pharmacist’s team could develop space-related clinical guidelines, including determining the most appropriate formulations for spaceflight medication and analyzing and developing correct drug storage and transportation. As pointed out by I.4 in Q. 9, we should also bear in mind the forthcoming tourist space travels. Civilian astronauts, including tourists and workers, may lack the rigorous training and vetting of ordinary astronauts. Instead, these civilians represent ordinary members of society with diverse patient-focused characteristics, potentially leading to a need for more considerations in optimizing medication compared to traditional astronauts. In this scenario, the pharmacist’s role becomes even more crucial, for instance, in carefully assessing the pharmacological aspect before the civilian’s departure.
Another crucial aspect of the storage protocol was highlighted by I.3 in Q.3: the JSC pharmacists have considerably cut back on the practice of repackaging. In the past, NASA pharmacists often repackaged pharmaceuticals to deal with limitations in mass and volume (Blue et al., 2019a). But “[…] They’re now using the original manufacturing packaging as much as possible […]” (Q. 3). The process of repackaging could potentially impact the shelf life or stability of stored medication. I.3 mentioned the use of plastic containers without specific protection and storage in fabric bags, offering protection from light, but not from humidity, oxygen, and radiation. Space radiation is a significant hazard for astronauts and pharmaceuticals. During forthcoming space missions beyond LEO, astronauts and all items stocked aboard the spacecraft will be exposed to Galactic Cosmic Rays (GCRs), which consist of very high energy (GigaeV) protons, helium ions, and occasionally, highly energetic heavier nuclei (Fogtman et al., 2023). Additionally, astronauts may encounter sporadic radiation storms caused by solar eruptions, known as Solar Particle Events (SPEs) (Fogtman et al., 2023). Heightened exposure to GCRs and SPEs will affect astronauts and all items stocked aboard the spacecraft, including pharmaceuticals, leading to higher cumulative radiation exposure and an elevated risk of radiation-induced changes in pharmaceutical stability, structure, potency, and potential toxicity (Fogtman et al., 2023; Mehta and Bhayani, 2017; Du et al., 2011; Wotring, 2016). In this context, the idea of using a 3D printer in space has been considered. NASA has started integrating additive manufacturing technologies into missions through the In-Space Manufacturing project. This integration aims to facilitate on-demand manufacturing and repair capabilities during transit and on the surface for logistics and maintenance (Seoane-Viaño et al., 2022). In 2014, the first 3D printer sent to space was a fused deposition modeling printer used to produce spare parts. By employing the right software, 3D-printed tablets can be generated within minutes, ensuring the exact dosage is tailored to the specific needs of the crewmember (Seoane-Viaño et al., 2022). Nonetheless, the process of drug manufacturing in space requires a thorough evaluation, and numerous questions remain unanswered (e.g., How can you ensure its quality? How do you regulate the process? How will quality control be implemented? What machinery will be utilized?). Furthermore, active ingredients would still need to be stored aboard the spacecraft. Thus, the space-saving benefits for future space missions with cargo limitations are limited.
It’s also crucial to emphasize that stability properties and industry standards for shelf life are clearly defined for applications in terrestrial conditions. However, while the temperature and humidity conditions on the ISS have been proven suitable for storing medication, the effects of other unique environmental factors associated with space travel, such as microgravity and increased radiation exposure, have not yet been evaluated (Wotring, 2016; Blue et al., 2019b). The impact of space radiation on drugs of any formulation is unclear, as little research has been conducted to investigate these responses thoroughly (Du et al., 2011; Wotring, 2016; Blue et al., 2019b). Lastly, potential changes in the physical or chemical properties (that may involve variations in appearance or consistency, as well as modification of excipients, toxic degradation, or interactions between excipients and active ingredients) could jeopardize the drug’s stability. Special shielding may present a viable option, but “[…] Any shield made of metal is more dangerous than no shielding […]” (I.3 Q. 12). Alternative shields (water, iron, concrete, or plastics) could be an option, but, as emphasized by I.3, they require significant thickness to provide adequate protection. However, added weight, making it infeasible for launching. “If we consider the ISS, drug stability is not a big problem because the resupply is easy” (I.4 Q.7), but in the context of upcoming missions to the Moon and Mars, developing new materials and pharmaceuticals to shield and safeguard astronauts will be crucial. A new approach is required, especially for liquid formulations (e.g., Promethazine is available as a liquid formulation onboard the ISS), and it is the responsibility of radiation scientists to address this matter.
In fact, most biotechnological pharmaceuticals are provided in liquid forms and frequently need refrigeration. Denosumab, for instance, might be an effective drug for mitigating bone mass loss experienced in microgravity. The primary method to address space bone demineralization is by employing loading techniques, and that is why exercise serves as a significant means of counteracting this issue, with ARED being particularly notable as a loading device that allows crewmembers to perform bone-preserving weight-lifting exercises aboard the ISS (LeBlanc et al., 2013; LeBlanc et al., 2002). Without countermeasures, astronauts are projected to experience a decline in bone mineral density, estimated at approximately 1.5% at the trochanter and roughly 1% at the femoral neck and spine per month (Tran et al., 2022). In situations where exercise is not feasible for injuries, sprains, equipment malfunction, sickness, or other crew or environmental constraints, additional alternatives will be notably crucial (LeBlanc et al., 2013; LeBlanc et al., 2002). Is physical exercise sufficient to prevent loss of bone mass and muscle mass? This query was posed to numerous interviewees, who highlighted that physical activity is mandatory but not entirely sufficient (I.1 Q. 13 and I.3 Q. 8/10). In this regard, to address the problem of bone mass loss, LeBlanc and colleagues proposed using alendronate in combination with physical activity (LeBlanc et al., 2002). However, it’s imperative to emphasize that bisphosphonates have numerous side effects, including gastrointestinal issues. Specifically, alendronate can cause local irritation in the upper gastrointestinal mucosa. From a technical standpoint, Denosumab’s usage could be a potential solution for short-term missions (up to 6 months maximum). Nonetheless, this period is not sufficient for longer-duration missions, and carrying an injection would be very problematic, both for refrigeration storage and drug stability. In this regard, “[…] A long-acting depot injection or some kind of implant that releases the drug over time […]” (I.3 Q. 8) could be a potential solution.
A crucial point to highlight from both I.1 and I.3 is that none of the medications from the personal medical kit (expired or not expired) are left on board or returned to Earth: they are disposed of. This approach represents a lost chance for research. Bringing back on Earth samples of medications (expired or not) for chemical and pharmaceutical analysis would help to understand any changes in the active ingredient or its excipients, as well as any unusual or toxic degradation products. Realistic shelf life could be determined. This would facilitate the design of effective countermeasures for proper drug storage, not only aboard the ISS but also for future missions beyond LEO. It is also possible that some information concerning drug management and use are classified by space agencies.
The last aspect to be discussed is the preeminence of immediate health issues over long-term health issues. The mission of the flight medical team is to take care of immediate medical problems, to allow completion of the mission, and safe return of the crew, which is indeed mandatory. As stated by the ESA flight surgeon, “You can consider the use of drugs for this issue when you start doing very long-duration flights or if you start finding certain cardiovascular events. That would be to treat a minority of crewmembers because the majority do not have it. Why do I have to give a drug for something that does not cause me a problem?” (Q.8/9). This is in contrast with the longer-term views of I.6 who considers that SIC in major physiological systems may compromise the future health of astronauts, notably beyond LEO and for longer missions. For instance, lunar astronauts had shorter life-spans than reference US populations, despite being selected for excellent health, mainly because of cardiovascular complications (Delp et al., 2016). Further, a critical insight regarding cardiovascular health is that: “[…] the longer you stay in microgravity, the worse it is […]” and “[…] This increase in stiffness is equivalent to 20 years of aging and we don’t know about the reversibility of this phenomenon” (I. 6/7 Q. 1) (Hughson et al., 2016). Given this alarming data, a critical question emerges: how can we halt or at least decelerate the vascular aging process in astronauts? The answer is not straightforward. Firstly, the countermeasures presently employed on board the ISS, namely, exercise and a healthy diet, are mandatory but only partially effective. Some pharmaceutical countermeasures could be helpful, particularly in the context of upcoming space missions, especially in addressing arterial stiffness, a cardiovascular risk factor of considerable importance. Additionally, as highlighted by I.5, the space environment significantly affects endothelial cells: “[…] I could say that many of the health problems reported by astronauts could be associated with such malfunctioning of the endothelium, the molecular basis of which was unknown” (Q. 1). In particular, microgravity and space radiation have an opposite effect on the endothelial cells: “[…] the net effect was that autophagy was inhibited. Thus, during spaceflight in endothelial cells, autophagy is not working properly” (Q. 3). At this stage, it is essential to underscore the interconnection of these elements: endothelial stress and resulting endothelial damage, combined with cardiovascular deconditioning, may result in severe cardiovascular health outcomes for astronauts during prolonged space missions and/or deep space endeavors (such as lunar and Martian missions). The use of drugs and/or supplements in this context becomes mandatory, aimed not at treating a pathology but at preventing potential complications. For instance, protective cardiovascular drugs and antioxidant supplements capable of preventing ROS-induced damage should be explored as potential pharmacological countermeasures.
Within the context of cardiovascular health, a significant issue requiring attention is orthostatic intolerance (OI) upon return to Earth. After short-duration missions (4–18 days), the occurrence of postflight OI is estimated to be around 20%–40%, while for long-duration missions, the incidence of postflight OI increases significantly to 80% (Baran et al., 2021). In this regard, the astronaut stated: “I didn’t experience anything that had to do with pressure drops, but I did have a serious … let’s call it instability problem, with vomiting and nausea. […]” (Q. 8). In this case, the astronaut did not experience any issues with OI, but upon returning to planet Earth, stability problems emerged, likely linked to vestibular SIC. Nonetheless, OI and stability issues upon re-entry to Earth pose many difficulties. Currently, a medical team is in place to assist astronauts upon landing. However, the first astronauts that will land on the “Red Planet” will necessarily be alone, and, similar to Antarctica, they will rely on the resources at their disposal. In this scenario, an orthostatic intolerance-induced fall can jeopardize both the mission and the lives of the crewmembers. Several pharmacological countermeasures have been explored to address this issue, for instance, fludrocortisone at a single 0.3 mg dose has been administrated in astronauts before the landing. Fludrocortisone is a synthetic steroid possessing mineralocorticoid activity, promoting the reabsorption of sodium from renal tubules and enhancing the urinary excretion of potassium in exchange for hydrogen (Freeman et al., 2018). However, fludrocortisone was dropped from further studies because of its glucocorticoid side effects (Wotring, 2011; Hargens and Watenpaugh, 1996). The effect of midodrine has also been investigated as a countermeasure (Wotring, 2011) and I.6/7 also mentioned it as a potential countermeasure for OI. Midodrine elevates standing systolic blood pressure (SBP) in a dose-dependent manner, reaching the peak efficacy 1 hour after oral administration (Freeman et al., 2018). However, it is crucial to consider the hypertensive effect associated with the administration of a vasoconstrictor drug, like midodrine. Thus, these drugs can only be used in astronauts, who are in excellent physical health, and only in the context of future planet landing. Nonetheless, prior testing of pharmacokinetics and pharmacodynamic response is mandatory to mitigate the risk of severe hypertension in astronauts.
Thus, we should generally view drugs as viable countermeasures to mitigate vascular issues (and other SIC) resulting from extended space travel. However, these countermeasures should only be considered after an in-depth assessment and study of the PK of these drugs in the space environment (data that are currently lacking). Drug administration in space, with the assumption of similar action and PK to those on Earth, poses significant risks of adverse effects, toxicity, or ineffective therapeutic outcomes. Lastly, physical countermeasures are insufficient to prevent SIC, and many SIC are not fully reversible. This is also a cultural issue, acute, curative medicine contrasting with longer-term, preventive medicine. Both should be considered in the context of new space: longer-term missions, deep space, and less selected crews, to guarantee both operational crews and healthy astronauts after return.
In conclusion, it is essential to acknowledge that future space missions will encounter substantial constraints regarding cargo capacity: “[…] the problem is space: the ISS is a luxury boat. […] The missions to the Moon will be in a tiny spacecraft that may get a few kilos of medical supplies […]” (I.1 Q. 15/16). There won’t be enough space to bring all the needed medications during this type of journey. Thus, it is necessary to carefully consider which pharmaceuticals and medical devices to take along because “[…] we don’t have the technology to put sufficient mass and volume on the Lunar Gateway to treat everything that could happen […]” (I.1 Q. 15/16). In this regard, NASA and ESA have two different approaches. The NASA drug kit relies on a probabilistic methodology based on a risk-based-drug approach. Thus, medications for the Lunar Gateway program will be selected according to the balance of the following criteria: 1) the drugs usually taken by astronauts’ everyday life on the ground (i.e., the preferred antipain medication); 2) the drugs more frequently utilized by astronauts during previous ISS flights; 3) a thorough predictive analysis of the new scenario astronauts will encounter.
In a different direction, ESA is trying to draw up a medical kit that grants the chance to manage any health issues, with a preference for those drugs that could treat various pathological conditions regardless of their etiology (i.e., broad-spectrum antibiotics are more eligible than narrow-spectrum ones). Once decided which medications to carry, the next crucial consideration regards how much drug/how many pills should be embarked on, a parameter that can be determined according to the duration of the routinary therapeutic cycles. Both approaches entail risks due to the lack of data, thus representing a complex unsolvable dilemma. Considering the limitations of mass and volume, the astronaut’s drug susceptibility and any genetic polymorphisms should be verified before the launch. Genotyping, phenotyping, and determining any genetic polymorphism of each astronaut to obtain tailor-made countermeasures is thus mandatory. Therefore, pre-flight genomic screening could provide a more comprehensive and detailed view of biological processes, contributing to understanding the disease, the development of new therapies, and personalizing medicine.
Furthermore, it is crucial to highlight the contrast between the tasks performed by astronauts aboard the ISS during extravehicular activities (EVAs) and the activities that astronauts will perform during the upcoming Artemis missions on the lunar surface (I.3 Q. 15). EVAs aboard the ISS consist mainly of manual tasks using hand tools. On the lunar surface, EVAs may be much more physically demanding. Hence, astronauts will use many more tools and be exposed to rocks and lunar dust. The Apollo missions highlighted a significant challenge faced by astronauts in “walking” on the lunar surface. Indeed, the Apollo crew hopped, skipped, jumped, and fell on the Lunar surface during EVAs. In particular, 21 near falls and 27 falls occurred throughout all the Apollo missions (Thuro and Stirling, 2021). Thus, there might be an increased risk of fractures and/or traumatic injuries. In this context, the use of analgesic medications, including stronger ones such as opioids, might be taken into account, along with specific bandages and splints for limb immobilization.
Lastly, one exciting approach in mitigating biological space alterations (such as impaired state of tiny blood vessel endothelium, inhibited autophagy, heightened DNA damage, and significant alterations in cytoskeletal structure (Barravecchia et al., 2022), which could lead to several SIC, could be hibernation. The concept of using hibernation for space travel has been discussed for many years. However, it has recently become more feasible due to the advent of technologies to produce hibernation-like circumstances (synthetic torpor) in non-hibernating species. As mentioned by I.5 evidence from animal studies indicates that radiation effects are mitigated during hibernation (Cerri et al., 2016).
An emerging and attractive topic is the role that the astronauts’ microbiome modifications can play during space flights, either in altering the effectiveness of drugs, or in causing health issues, particularly with regard to long-term missions (Tesei et al., 2022; Turroni et al., 2020; Voorhies et al., 2019). Hence in the immediate future, it will be important to develop strategies to preserve a healthy microbiome and/or to enrich astronauts diet with specific probiotics and prebiotics to positively impact on inflammation status, bone integrity, and other parameters/functions (Tesei et al., 2022; Turroni et al., 2020).
5 Conclusion and future prospectives
This research highlights the critical need for more intense exploration of space pharmacology, underlining the various research gaps and proposed potential pharmacological strategies for future space missions. Despite insights from various researchers and experts in the field, significant gaps persist in our understanding of drugs PK/PD in space.
A potential strategy to adopt to bridge the lack of research regarding possible alterations of drug metabolism in space could be the use of DBS and DUS. These techniques have undergone feasibility testing during parabolic flights and have proven to fulfill the necessary standards for space pharmacology in terms of energy efficiency, reliability, safety, and ease of use (Derobertmasure et al., 2023; Derobertmasure and Boutouyrie, 2024). As a result, DBS and DUS represent a promising solution for conducting pharmacokinetic studies in remote environments where conventional sampling and storage methods may not be practical (Derobertmasure et al., 2023; Derobertmasure and Boutouyrie, 2024). Their minimal biological sample requirements, lightweight, and extended stability make these two techniques particularly suitable for use aboard the ISS.
In addition, animal models may present a logistically easier and more cost-effective way to assess PK and PD under microgravity conditions. Human analogs, such as bed rest or dry immersion, could also be considered as alternatives. Nonetheless, human analog studies in simulated microgravity are not validated with the in-flight model. Comparative research on medications PK between actual and simulated microgravity is, in fact, notably lacking. The ideal approach could be conducting the same study both in microgravity and bed rest.
Further, one promising direction could be the implementation of personalized medicine through the application of pharmacogenomic testing. In particular, personalized medicine aims to offer tailored healthcare to an individual’s specific physical, genetic, clinical, and sociodemographic attributes. The aim is to foresee disease patterns and responses to treatment at an individual level (Pavez et al., 2021). Additionally, genomic studies have identified individual differences linked to spaceflight-related aspects such as bone mineral density and sensitivity to radiation (Cope et al., 2022). Thus, instead of an empirical pre-testing before departure (I.1 Q4 and I.2 Q.7), the exploration of astronauts’ genomic profiles before space missions could have the potential to enhance personalized medicine approaches by assessing risks and developing countermeasures. Moreover, optimizing cargo for future space missions would be possible by including the most effective medications customized for individual crew members.
6 Limitations
The authors emphasize that a specific protocol for the selection of interviewees could not be applied, as there are very few experts in the field of space pharmacology. In addition, not all of them responded positively to our request for an interview, and some of them were not allowed to release interviews. It should be noted that the paucity of experts, and the small sample size of interviewees, is intrinsically due to the limited literature and research in this particular field. For this reason, the methodology of the present study could be considered as a collection of separate interviews.
Further, it should be underlined that the information and answers reported by the interviewees are entirely subjective and based on the general experience of the interviewees. The information reported does not represent the official positions of the various Space Agencies. Moreover, certain questions were not addressed, and some details were withheld to maintain confidentiality. For instance, no psychiatric issues were examined due to the classified nature of the information.
Finally, the authors also acknowledge that the regulatory aspect of drugs onboard the ISS has not been addressed, as the present work aims to focus on pharmacological aspects rather than drug legislation. The presence of an international crew is certainly a factor to consider, as each country has unique drug regulatory agencies, resulting in different regulatory practices. This may involve different drug dosages or formulations, with some medications being available exclusively in certain countries.
Data availability statement
The raw data supporting the conclusions of this article will be made available by the authors, without undue reservation.
Author contributions
KB: Writing–review and editing, Writing–original draft, Conceptualization, Investigation, Data curation, Methodology, Formal Analysis, Project Administration. AD: Writing–review and editing, Conceptualization, Investigation, Methodology, Supervision. PB: Writing–review and editing, Conceptualization, Investigation, Methodology, Supervision. PS: Writing–review and editing, Conceptualization, Investigation, Methodology, Supervision, Funding acquisition.
Funding
The author(s) declare that no financial support was received for the research, authorship, and/or publication of this article.
Acknowledgments
We would like to express our heartfelt gratitude to all the interviewees: thank you for your time, invaluable support, and availability during this project. Your contributions have been truly appreciated.
Conflict of interest
The authors declare that the research was conducted in the absence of any commercial or financial relationships that could be construed as a potential conflict of interest.
Publisher’s note
All claims expressed in this article are solely those of the authors and do not necessarily represent those of their affiliated organizations, or those of the publisher, the editors and the reviewers. Any product that may be evaluated in this article, or claim that may be made by its manufacturer, is not guaranteed or endorsed by the publisher.
Supplementary material
The Supplementary Material for this article can be found online at: https://www.frontiersin.org/articles/10.3389/frspt.2024.1456614/full#supplementary-material
References
Ax, T., Ganse, B., Fries, F. N., Szentmáry, N., De Paiva, C. S., March De Ribot, F., et al. (2023). Dry eye disease in astronauts: a narrative review. Front. Physiol. 14, 1281327. doi:10.3389/fphys.2023.1281327
Baran, R., Marchal, S., Garcia Campos, S., Rehnberg, E., Tabury, K., Baselet, B., et al. (2021). The cardiovascular system in space: focus on in vivo and in vitro studies. Biomedicines 10 (1), 59. doi:10.3390/biomedicines10010059
Barchetti, K. (2024). Space travels, astronauts, and drugs the state of the art on pharmacological aspects through interview analysis with experts in the field. Italy: University of Urbino Carlo Bo. dissertation/master’s thesis.
Barger, L. K., Flynn-Evans, E. E., Kubey, A., Walsh, L., Ronda, J. M., Wang, W., et al. (2014). Prevalence of sleep deficiency and use of hypnotic drugs in astronauts before, during, and after spaceflight: an observational study. Lancet Neurol. 13 (9), 904–912. doi:10.1016/s1474-4422(14)70122-x
Barravecchia, I., De Cesari, C., Forcato, M., Scebba, F., Pyankova, O. V., Bridger, J. M., et al. (2022). Microgravity and space radiation inhibit autophagy in human capillary endothelial cells, through either opposite or synergistic effects on specific molecular pathways. Cell. Mol. Life Sci. 79 (1), 28. doi:10.1007/s00018-021-04025-z
Blue, R. S., Bayuse, T. M., Daniels, V. R., Wotring, V. E., Suresh, R., Mulcahy, R. A., et al. (2019a). Supplying a pharmacy for NASA exploration spaceflight: challenges and current understanding. Npj Microgravity 5 (1), 14. doi:10.1038/s41526-019-0075-2
Blue, R. S., Chancellor, J. C., Antonsen, E. L., Bayuse, T. M., Daniels, V. R., and Wotring, V. E. (2019b). Limitations in predicting radiation-induced pharmaceutical instability during long-duration spaceflight. Npj Microgravity 5 (1), 15. doi:10.1038/s41526-019-0076-1
Boyd, J., Wang, Z., and Putcha, L. (2009). Bioavailability of promethazine during spaceflight. Available at: https://ntrs.nasa.gov/citations/20090001322.
Cerri, M., Tinganelli, W., Negrini, M., Helm, A., Scifoni, E., Tommasino, F., et al. (2016). Hibernation for space travel: impact on radioprotection. Life Sci. Space Res. 11, 1–9. doi:10.1016/j.lssr.2016.09.001
Cintrón, N. M., Putcha, L., and Vanderploeg, J. M. (1987a). “Inflight pharmacokinetics of acetaminophen in saliva,” In Results of the life science DSOs conducted aboard the space shuttle 1981–1986. Editors M. W. Bungo, T. M. Bagian, M. A. Bowman, and B. M. Levitan (Houston, Texas: Space Biomedical Research Institute, Johnson Space Center), 19–23. https://ntrs.nasa.gov/api/citations/19870017063/downloads/19870017063.pdf.
Cintrón, N. M., Putcha, L., and Vanderploeg, J. M. (1987b). “Inflight salivary pharmacokinetics of scopolamine and dextroamphetamine,” In Results of the life science DSOs conducted aboard the space shuttle 1981–1986. Editors M. W. Bungo, T. M. Bagian, M. A. Bowman, and B. M. Levitan (Houston, Texas: Space Biomedical Research Institute, Johnson Space Center), 25–29. https://ntrs.nasa.gov/api/citations/19870017063/downloads/19870017063.pdf.
Cope, H., Willis, C. R. G., MacKay, M. J., Rutter, L. A., Toh, L. S., Williams, P. M., et al. (2022). Routine omics collection is a golden opportunity for European human research in space and analog environments. Patterns 3 (10), 100550. doi:10.1016/j.patter.2022.100550
Dello, R. C., Bandiera, T., Monici, M., Surdo, L., Yip, V. L. M., Wotring, V., et al. (2022). Physiological adaptations affecting drug pharmacokinetics in space: what do we really know? A critical review of the literature. Br. J. Pharmacol. 179 (11), 2538–2557. doi:10.1111/bph.15822
Delp, M. D., Charvat, J. M., Limoli, C. L., Globus, R. K., and Ghosh, P. (2016). Apollo lunar astronauts show higher cardiovascular disease mortality: possible deep space radiation effects on the vascular endothelium. Sci. Rep. 6 (1), 29901. doi:10.1038/srep29901
Demontis, G. C., Germani, M. M., Caiani, E. G., Barravecchia, I., Passino, C., and Angeloni, D. (2017). Human pathophysiological adaptations to the space environment. Front. Physiol. 8, 547. doi:10.3389/fphys.2017.00547
Derobertmasure, A., Toh, L. S., Verstuyft, C., De Sousa Carvalho, C., Couronné, R., Beauvalet, M., et al. (2024). Feasibility of dried blood spot collection for caffeine pharmacokinetic studies in microgravity: insights from parabolic flight campaigns. Br. J. Clin. Pharmacol. under review. doi:10.22541/au.172498722.28068605/v1
Derobertmasure, A., Kably, B., Justin, J., De Sousa Carvalho, C., Billaud, E. M., and Boutouyrie, P. (2023). Dried Urine Spot Analysis for assessing cardiovascular drugs exposure applicable in spaceflight conditions. J. Chromatogr. B 1219, 123539. doi:10.1016/j.jchromb.2022.123539
Du, B., Daniels, V. R., Vaksman, Z., Boyd, J. L., Crady, C., and Putcha, L. (2011). Evaluation of physical and chemical changes in pharmaceuticals flown on space missions. AAPS J. 13 (2), 299–308. doi:10.1208/s12248-011-9270-0
European Space Agency - Bedrest Studies (2024). ESA website. Available at: https://www.esa.int/Science_Exploration/Human_and_Robotic_Exploration/Bedrest_studies/.
Eyal, S. (2020). How do the pharmacokinetics of drugs change in astronauts in space? Expert Opin. Drug Metab. Toxicol. 16 (5), 353–356. doi:10.1080/17425255.2020.1746763
Eyal, S., and Derendorf, H. (2019). Medications in space: in search of a pharmacologist’s guide to the galaxy. Pharm. Res. 36 (10), 148. doi:10.1007/s11095-019-2679-3
Fogtman, A., Baatout, S., Baselet, B., Berger, T., Hellweg, C. E., Jiggens, P., et al. (2023). Towards sustainable human space exploration—priorities for radiation research to quantify and mitigate radiation risks. Npj Microgravity. 9 (1), 8. doi:10.1038/s41526-023-00262-7
Freeman, R., Abuzinadah, A. R., Gibbons, C., Jones, P., Miglis, M. G., and Sinn, D. I. (2018). Orthostatic hypotension. J. Am. Coll. Cardiol. 72 (11), 1294–1309. doi:10.1016/j.jacc.2018.05.079
Gandia, P., Bareille, M., Saivin, S., Le-Traon, A. P., Lavit, M., Guell, A., et al. (2003). Influence of simulated weightlessness on the oral pharmacokinetics of acetaminophen as a gastric emptying probe in man: a plasma and a saliva study. J. Clin. Pharmacol. 43 (11), 1235–1243. doi:10.1177/0091270003257229
Gandia, P., Saivin, S., Le-Traon, A. P., Guell, A., and Houin, G. (2006). Influence of simulated weightlessness on the intramuscular and oral pharmacokinetics of promethazine in 12 human volunteers. J. Clin. Pharmacol. 46 (9), 1008–1016. doi:10.1177/0091270006291032
Hargens, A. R., and Watenpaugh, D. E. (1996). Cardiovascular adaptation to spaceflight. Med. Sci. Sports Exerc 28, 977–982. doi:10.1097/00005768-199608000-00007
Hollander, J., Gore, M., Fiebig, R., Mazzeo, R., Ohishi, S., Ohno, H., et al. (1998). Spaceflight downregulates antioxidant defense systems in rat liver. Free Radic. Biol. Med. 24 (2), 385–390. doi:10.1016/s0891-5849(97)00278-5
Hughson, R. L., Robertson, A. D., Arbeille, P., Shoemaker, J. K., Rush, J. W. E., Fraser, K. S., et al. (2016). Increased postflight carotid artery stiffness and inflight insulin resistance resulting from 6-mo spaceflight in male and female astronauts. Am. J. Physiol-Heart Circ. Physiol. 310 (5), H628–H638. doi:10.1152/ajpheart.00802.2015
Kast, J., Yu, Y., Seubert, C. N., Wotring, V. E., and Derendorf, H. (2017). Drugs in space: pharmacokinetics and pharmacodynamics in astronauts. Eur. J. Pharm. Sci. 109, S2–S8. doi:10.1016/j.ejps.2017.05.025
Kovachevich, I. V., Kondratenko, S. N., Starodubtsev, A. K., and Repenkova, L. G. (2009). Pharmacokinetics of acetaminophen administered in tablets and capsules under long-term space flight conditions. Pharm. Chem. J. 43 (3), 130–133. doi:10.1007/s11094-009-0255-6
LeBlanc, A., Matsumoto, T., Jones, J., Shapiro, J., Lang, T., Shackelford, L., et al. (2013). Bisphosphonates as a supplement to exercise to protect bone during long-duration spaceflight. Osteoporos. Int. 24 (7), 2105–2114. doi:10.1007/s00198-012-2243-z
LeBlanc, A. D., Driscol, T. B., Shackelford, L. C., Evans, H. J., Rianon, N. J., Smith, S. M., et al. (2002). Alendronate as an effective countermeasure to disuse induced bone loss. J. Musculoskelet. Neuronal Interact. 2 (4), 335–343.
Lee, A. G., Mader, T. H., Gibson, C. R., Tarver, W., Rabiei, P., Riascos, R. F., et al. (2020). Spaceflight associated neuro-ocular syndrome (SANS) and the neuro-ophthalmologic effects of microgravity: a review and an update. Npj Microgravity 6 (1), 7. doi:10.1038/s41526-020-0097-9
Lemke, T. M., Williams, D. A., Roche, V. F., and Zito, W. S. (2013). Foye’s Principles of medicinal chemistry. 7th Edition.
Macias, B. R., Patel, N. B., Gibson, C. R., Samuels, B. C., Laurie, S. S., Otto, C., et al. (2020). Association of long-duration spaceflight with anterior and posterior ocular structure changes in astronauts and their recovery. JAMA Ophthalmol. 138 (5), 553. doi:10.1001/jamaophthalmol.2020.0673
Mehta, P., and Bhayani, D. (2017). Impact of space environment on stability of medicines: challenges and prospects. J. Pharm. Biomed. Anal. 136, 111–119. doi:10.1016/j.jpba.2016.12.040
Pavez, L. E., Baatout, S., Choukér, A., Buchheim, J. I., Baselet, B., Dello, R. C., et al. (2021). The future of personalized medicine in space: from observations to countermeasures. Front. Bioeng. Biotechnol. 9, 739747. doi:10.3389/fbioe.2021.739747
Polyakov, A. V., Svistunov, A. A., Kondratenko, S. N., Kovachevich, I. V., Repenkova, L. G., Savelyeva, M. I., et al. (2022). Study of the pharmacokinetics of various drugs under conditions of antiorthostatic hypokinesia and the pharmacokinetics of acetaminophen under long-term spaceflight conditions. Drug Metab. Pers. Ther. 37 (2), 163–175. doi:10.1515/dmpt-2021-0159
Rang, H. P., Ritter, J. M., Flower, R. J., and Henderson, G. (2016). RANG and DALE farmacologia ottava edizione. Eighth edition. Milano (MI), Italy: Edra SPA.
Saivin, S., Pavy-Le Traon, A., Cornac, A., Güell, A., and Houin, G. (1995). Impact of a four-day head-down tilt (–6°) on lidocaine pharmacokinetics used as probe to evaluate hepatic blood flow. J. Clin. Pharmacol. 35 (7), 697–704. doi:10.1002/j.1552-4604.1995.tb04110.x
Schuck, E. L., Grant, M., and Derendorf, H. (2005). Effect of simulated microgravity on the disposition and tissue penetration of ciprofloxacin in healthy volunteers. J. Clin. Pharmacol. 45 (7), 822–831. doi:10.1177/0091270005276620
Seoane-Viaño, I., Ong, J. J., Basit, A. W., and Goyanes, A. (2022). To infinity and beyond: strategies for fabricating medicines in outer space. Int. J. Pharm. X 4, 100121. doi:10.1016/j.ijpx.2022.100121
Southard, B. T., and Al Khalili, Y. (2024). “Promethazine,” in StatPearls (Treasure Island (FL): StatPearls Publishing). Available at: http://www.ncbi.nlm.nih.gov/books/NBK544361/(Accessed June 12, 2024).
Tesei, D., Jewczynko, A., Lynch, A. M., and Urbaniak, C. (2022). Understanding the complexities and changes of the astronaut microbiome for successful long-duration space missions. Life 12 (4), 495. doi:10.3390/life12040495
Thuro, A., and Stirling, L. (2021). “Characterization of the Apollo astronaut lunar extravehicular activity falls and near-falls,” in 2021 IEEE aerospace conference (50100). Big sky (MT, USA: IEEE), 1–6. Available at: https://ieeexplore.ieee.org/document/9438263/.
Tran, Q. D., Tran, V., Toh, L. S., Williams, P. M., Tran, N. N., and Hessel, V. (2022). Space medicines for space health. ACS Med. Chem. Lett. 13 (8), 1231–1247. doi:10.1021/acsmedchemlett.1c00681
Turroni, S., Magnani, M., Kc, P., Lesnik, P., Vidal, H., and Heer, M. (2020). Gut microbiome and space travelers’ health: state of the art and possible pro/prebiotic strategies for long-term space missions. Front. Physiol. 11, 553929. doi:10.3389/fphys.2020.553929
VirginiaE, W., and Smith, L. K. (2020). Dose tracker application for collecting medication use data from international space station crew. Aerosp. Med. Hum. Perform. 91 (1), 41–45. doi:10.3357/amhp.5392.2020
Voorhies, A. A., Mark Ott, C., Mehta, S., Pierson, D. L., Crucian, B. E., Feiveson, A., et al. (2019). Study of the impact of long-duration space missions at the International Space Station on the astronaut microbiome. Sci. Rep. 9 (1), 9911. doi:10.1038/s41598-019-46303-8
White, R. J. (1998). Weightlessness and the human body. Sci. Am. 279 (3), 58–63. doi:10.1038/scientificamerican0998-58
Wotring, V. (2011). Evidence report: risk of therapeutic failure due to ineffectiveness of medication. Houston, TX: Universities Space Research Association. Available at: https://humanresearchroadmap.nasa.gov/evidence/reports/pharm.pdf.
Wotring, V. E. (2015). Medication use by U.S. Crewmembers on the international space station. FASEB J. 29 (11), 4417–4423. doi:10.1096/fj.14-264838
Keywords: space environment, astronauts, microgravity, metabolism, pharmacokinetics, pharmacodynamics, pharmacogenetics
Citation: Barchetti K, Derobertmasure A, Boutouyrie P and Sestili P (2024) Redefining space pharmacology: bridging knowledge gaps in drug efficacy and safety for deep space missions. Front. Space Technol. 5:1456614. doi: 10.3389/frspt.2024.1456614
Received: 28 June 2024; Accepted: 27 September 2024;
Published: 18 October 2024.
Edited by:
Ilaria Cinelli, Aerospace Medical Association, Alexandria, United StatesReviewed by:
Octavian Vasiliu, Dr. Carol Davila University Emergency Military Central Hospital, RomaniaKimia Seyedmadani, National Aeronautics and Space Administration, United States
Adel B. Elmoselhi, University of Sharjah, United Arab Emirates
Copyright © 2024 Barchetti, Derobertmasure, Boutouyrie and Sestili. This is an open-access article distributed under the terms of the Creative Commons Attribution License (CC BY). The use, distribution or reproduction in other forums is permitted, provided the original author(s) and the copyright owner(s) are credited and that the original publication in this journal is cited, in accordance with accepted academic practice. No use, distribution or reproduction is permitted which does not comply with these terms.
*Correspondence: Audrey Derobertmasure, YXVkcmV5LmRlcm9iZXJ0bWFzdXJlQGFwaHAuZnI=
†These authors have contributed equally to this work and share senior authorship