- 1Department of Biological Engineering, Massachusetts Institute of Technology (MIT), Cambridge, MA, United States
- 2Department of Developmental Biology, Harvard School of Dental Medicine, Boston, MA, United States
- 3Javelin Biotech, Woburn, MA, United States
- 4Redwire Space Technologies, Jacksonville, IN, United States
- 5Department of Chemical Engineering, Massachusetts Institute of Technology, Cambridge, MA, United States
- 6Corteva Agriscience, Indianapolis, IN, United States
- 7Departments of Pediatrics, Orthopedic Surgery and Medicine (Section of Rheumatology), Rush University Medical Center, Chicago, IL, United States
- 8Department of Orthopaedic Surgery, Indiana University School of Medicine, Indianapolis, IN, United States
- 9Lund University, Lund, Sweden
- 10Departments of Electrical Engineering and Computer Science, Cambridge, MA, United States
- 11Mechanical Engineering, Massachusetts Institute of Technology (MIT), Cambridge, MA, United States
Post-traumatic Osteoarthritis (PTOA) results from traumatic joint injuries (such as an ACL rupture). Mechanical impact and an immediate synovial inflammatory response can result in joint tissue degradation and longer-term progression to PTOA. Astronauts are susceptible to increased exercise-related joint injuries leading to altered musculoskeletal physiology, further escalated due to microgravity and increased exposure to ionizing radiation. We applied a human Cartilage-Bone-Synovium (CBS) coculture model to test the potential of low-dose dexamethasone (Dex) and IGF-1 in ameliorating PTOA-like degeneration on Earth and the International Space Station-National Laboratory (ISS-NL, ISS for short). CBS cocultures were established using osteochondral plugs (CB) subjected to compressive impact injury (INJ) followed by coculture with synovium (S) explants. Study groups consisted of control (CB); disease [CBS + INJ]; treatment [CBS + INJ + Dex + IGF-1]; and drug-safety [CB + Dex + IGF-1]. Outcome measures included cell viability, altered matrix glycosaminoglycans (GAG) and collagens, multiplex-ELISA quantification of released cytokines, histopathology, and metabolomic and proteomic analyses of spent media. A 21-day study on ISS-NL explored PTOA-like pathogenesis and treatment in microgravity. Tissue cards for study groups were cultured in custom-built culture chambers within multi-use variable-g platforms (MVPs). A marked upregulation in the release of inflammatory cytokines and tissue-GAG loss was observed in CBS + INJ groups in space and ground controls utilizing tissues from the same donors, similar to that reported in a previous multi-donor study on Earth; these changes were partly ameliorated by Dex + IGF-1, but with donor variability. Metabolomic and proteomic analyses revealed an array of distinct differences between metabolites/proteins released to the medium in Space versus on Earth.
Introduction
Post-traumatic osteoarthritis (PTOA) results from joint injuries such as ACL and meniscal tears of the knee (Brown et al., 2006), and has become a major contributor to disability across all ages world-wide (Dilley et al., 2023). Such knee injuries involve pathological damage to multiple tissues including cartilage, bone, synovium, ligaments, meniscus and nerves. The mechanical impact causing injury triggers an intense inflammatory response, originating primarily from the synovial lining, which is characterized by the release of inflammatory cytokines including IL-6, TNFα, IL-1β, IL-8 and others (Struglics et al., 2015; Bradley et al., 2023). In the following days and weeks, increased cell death and matrix degeneration are observed in cartilage, leading to the release of aggrecan fragments and collagen type II cross-linked peptides (Lohmander et al., 2003) into the synovial fluid, along with a host of other degradome components (Rydén et al., 2023). These changes in cartilage are accompanied by alterations in the subchondral bone caused by abnormal cellular remodeling events initiated by changes in levels of sclerostin, RANKL, alkaline phosphatase, and osteocalcin (Robling et al., 2008; Sanchez et al., 2008; Xiong et al., 2011). Taken together, the ensuing degeneration of joint tissues and cell death can eventually lead to PTOA.
Astronauts are susceptible to high rates of musculoskeletal injuries before, during and after their mission periods due in part to the rigorous training required for mission-readiness. A study examining musculoskeletal injuries in astronauts during missions between 1981 and 1998 found a three-fold increase in injury rate within the mission period compared to overall rate of injury, including pre-flight and post-flight knee injuries (NASA, 1999). Musculoskeletal alterations during spaceflight may increase the vulnerability of astronauts to injury, further exacerbated by strenuous daily activities (Kerstman et al., 2012) and the high intensity resistance training exercise regimen (Thudium et al., 2023) followed on the International Space Station. Having flown high-performance jets previously, astronauts may have a pre-existing history of musculoskeletal injuries susceptible to further progression (NASA, 2016). Pre-flight simulated microgravity training in the weightless environment training facility and neutral buoyancy lab have also caused injuries (Viegas et al., 2004; Strauss et al., 2005), as have post-flight musculoskeletal deconditioning and landing impacts (Web.Archive, 2003). Any alterations in crew activities resulting from musculoskeletal injuries could have a serious impact on the mission’s success and timeline. Injuries on the ISS could also severely impact crew performance and mission activities (Scheuring et al., 2009; Ramachandran et al., 2018). Though osteoarthritis significantly impacts the quality of life of individuals on Earth and may adversely impact the crew mission in Space, there are still no FDA-approved disease-modifying osteoarthritic drugs (DMOADs), and knee replacement is not a desirable option especially in younger and otherwise healthy individuals.
To understand the early events involved in the interplay between biomechanical and biological responses to traumatic joint injury, we established an in vitro PTOA-like disease model using cartilage-bone-synovium cocultures derived from cadaveric human knee joints (Dwivedi et al., 2022). This human-derived microphysiological system (MPS) provides an effective way to recapitulate aspects of disease biology and to test the effects of potential therapeutics in a physiologically relevant manner to enable clinical translation. In our MPS, PTOA-like conditions are initiated by subjecting the cartilage surfaces of osteochondral plugs to a single mechanical compressive impact (simulating joint trauma) followed by coculture with synovium explants, the primary source of the inflammatory response to joint injury. Cocultures using 25 knees from 16 human donors revealed release of multiple inflammatory cytokines, primarily from the synovium explants, promoting increased chondrocyte death in the impacted cartilage along with extracellular matrix degradation and a range of metabolomic changes (Dwivedi et al., 2022). These results matched some of the earliest events documented in human patients post-ACL tear (Johnson et al., 1998; Struglics et al., 2015).
In PTOA, the cartilage homeostasis is disrupted due to an imbalance between catabolic and anabolic processes (Mueller and Tuan, 2011). To address this disparity between tissue repair and degradation, we investigated the combined use of dexamethasone (Dex) and IGF-1 on Earth as well as in Space. In a previous study using bovine and human knee cartilage, the combination of IGF-1 and Dex ameliorated cell death and cytokine-mediated matrix degradation, highlighting the potential benefits of combined pro-anabolic and anti-catabolic mediators (Li et al., 2015). Chronic inflammation and the accompanying release of proteolytic enzymes are hallmarks of PTOA disease (Richardson and Dodge, 2003; Huo et al., 2011), motivating the use of Dex, a pleiotropic, synthetic glucocorticoid, used clinically for OA pain (Black and Grodzinsky, 2019). Our preliminary studies showed that Dex exhibited anti-catabolic and chondroprotective properties by preserving chondrocyte viability, decreasing synovium release of inflammatory cytokines, and reducing matrix loss in the cartilage tissue subjected to mechanical impact injury and coculture with synovium explants (Dwivedi, 2019). IGF-1, a potent pro-anabolic (McQuillan et al., 1986) known to prevent injury-induced cell apoptosis (D’Lima et al., 2001) and promote the biosynthetic activity of chondrocytes (Tyler, 1989; Bonassar et al., 2000) was also included in the treatment group.
The overall goal of the present study was to investigate the response of our PTOA-like MPS disease model to spaceflight conditions on the ISS and to treatment with Dex and IGF-1 by comparing effects on cell viability, inflammation, and matrix alterations on Earth and in Space. The specific aims were 1) to adapt the MPS to on-station hardware developed by implementation partner, Redwire Space Technologies (f/k/a Techshot, Inc.), in order to prepare tissue payloads for autonomous operation on the ISS; 2) in collaboration with Redwire, to execute studies on Earth and the ISS with PTOA-like diseased and healthy control tissue groups with and without Dex and IGF-1, and 3) to compare the effects of spaceflight to ground control conditions assessed using proinflammatory multiplex ELISA, biochemical, metabolomic and proteomic readouts.
Materials and methods
Overall summary of mission
Payload preparation for launch on SpaceX-21 was initiated at MIT (Cambridge, MA) and at Redwire (Greenville, IN), and completed at the Space Life Sciences Laboratory (SLSL, FL). Cadaveric knees were received at MIT, and tissue explants were harvested and inserted into specialized tissue cards. These tissue cards were shipped along with nutrient media kit bags to SLSL, where they were assembled into Redwire’s Multi-Use Variable-g Platform (MVP) Modules. MVPs containing all designated tissue treatment conditions were delivered to the cold stowage team at the Kennedy Space Center (KSC) for transfer to the cargo payload of SpaceX’s new Cargo Dragon 2 capsule. Launch to the ISS on 6 December 2020 enabled a 3.5-week experiment on the ISS; return of all tissue and media samples to MIT for analysis was completed by May 2021. Here we focus on the experiments and the results of biochemical, inflammatory, metabolomic and proteomic outcome measures gained during this ISS-Expedition 64 mission. (A preliminary study using a precursor of this MVP system was launched to the ISS on SpaceX-17, 4 May 2019; results and lessons-learned will be described in a separate article.).
Tissue receipt and initial preparations for launch
Due to the unpredictability in procuring cadaveric knees in time for launch, we collaborated with three donor banks (LifeNet Health, Virginia Beach, VA; Gift of Hope Organ and Tissue Donor Network, Itaska, IL; National Disease Research Interchange (NDRI), Philadelphia, PA) to initiate deliveries to MIT 8–9 days before the intended launch date. In anticipation of possible launch delays/scrubs, multiple knees were received during that time; the most recent tissues received were used to prepare the final tissue cards for SLSL. For launch, we received both knees en bloc from each of two donors (male, 31 y.o.; female, 47 y.o.) within 36 h of death [Figures 1A,B]. All four joint surfaces were scored as Grade 0 (i.e., normal, no signs of any degenerative morphological changes) using the modified Collins grading system (Muehleman et al., 1997).
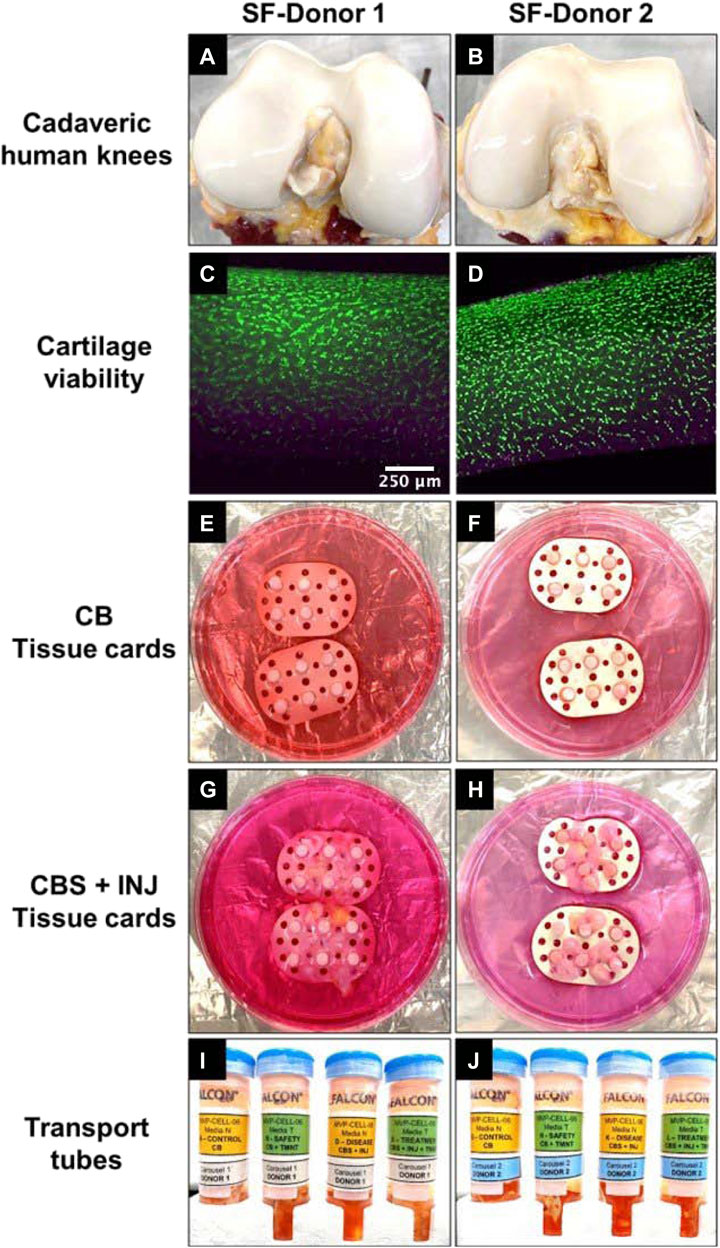
FIGURE 1. (A and B) Cadaveric human knees from SF-Donor 1 (A) and SF-Donor 2 (B) used for extraction of tissues and preparation of tissue cards. (C and D) Viability images following FDA/PI staining on day 0 upon receipt of donor knee joints to show live (green) and dead (pink) cells in the cartilage tissues of donor 1 (C) and donor 2 (D), before preparation of tissue cards for Spaceflight. (E and F) CB tissue cards prepared with non-injured CB plugs in donor 1 (E) and donor 2 (F) used for control and safety groups. (G and H) CBS + INJ tissue cards prepared with mechanically injured CB plugs and sutured synovium tissue for donor 1 (G) and 2 (H) used in disease and treatment groups. (I and J) Tissue cards were placed inside transport tubes containing 50 mL of media N (control, disease) or media T (safety, treatment) for shipping from MIT lab to SLSL.
Cartilage-bone-synovium harvesting, mechanical injury and culture configuration
All procedures were approved by MIT’s Committee on the Use of Humans as Experimental Subjects. Osteochondral plugs (designated CB, having total length 5 mm and diameter 3.5 mm) as well as synovium explants (S, approximately 6 × 6 mm) were harvested from all four femurs using tools and methods recently described in detail (Dwivedi et al., 2022). A total of 48 osteochondral and 24 synovium explant samples from these 2 donors were obtained for spaceflight. Cartilage viability was confirmed within the first day before further preparation [FDA/PI staining of sliced full thickness sections (Li et al., 2013) Figures 1C,D]. Harvested tissues were prepared for 4 treatment conditions on ISS: 1) normal CB plugs alone (non-injured control), 2) mechanically injured CB plugs co-cultured with synovium explants (“disease” group, CBS + INJ), 3) CBS + INJ + therapeutic drugs (“treatment” group), and 4) CB + therapeutic drugs (“drug safety” group). Additional tissues from the same joints were prepared for ground control studies at MIT. Therapeutic drugs for this study included 100 nM dexamethasone (Dex) plus 100 ng/mL (13 nM) insulin-like growth factor-1 (IGF-1). A single injurious compressive injury was applied to individual CB plugs using unconfined compression in a specialized incubator-housed instrument (15 MPa/s to peak stress of 5 MPa, held at 5 MPa for 0.4 s, then unloaded at 15 MPa/s) as previously described (Dwivedi et al., 2022). The resulting media concentrations of cytokines released by the synovial explants (Dwivedi et al., 2022) are markedly higher than those found in synovial fluid aspirates taken from normal human knees (Struglics et al., 2015); hence the use of the cut synovial explants in the ‘disease’ but not the CB control groups.
Since organ culture on the ISS required MPS culture in a closed sterile environment, custom-designed culture chambers and modules were developed [Figures 1, 2] to hold groups of 6 tissues (i.e., 6 technical replicates) from each donor joint pair, inserted into a tissue card for each of the four treatment conditions [Figures 1E–H]. Each culture chamber was primed with 15 mL of media [Figures 2A–D]. The tissue cards were designed to mechanically secure the tissues in order to separate and prevent them from aggregating together within a culture chamber during SpaceX launch and experiments on the ISS. Tissue cards were prepared from food-grade multi-purpose neoprene rubber sheet (1/64” thick), 38 mm long and 22 mm wide, and sterilized before use. Perforations in each card (3 mm diameter) enabled insertion of the 6 CB plugs for all treatment groups [Figures 1E–H] There was also enough space between perforations to allow the suturing of synovium explants for the disease and drug-treatment groups [Figures 1G,H]. Each synovium tissue was secured with a sterile suture (Covidien Surgipro 6–0, Cat No. VP-718-X) by tying three knots using the instrument-tie method. Tissue card perforations also allowed media circulation across and along each card within the culture chamber [Figures 2A,B]. The 8 tissue cards were prepared and placed inside transport tubes containing 50 mL of media [Figures 1I,J] immediately after preparation, and kept at room temperature until shipping that day from MIT to SLSL.
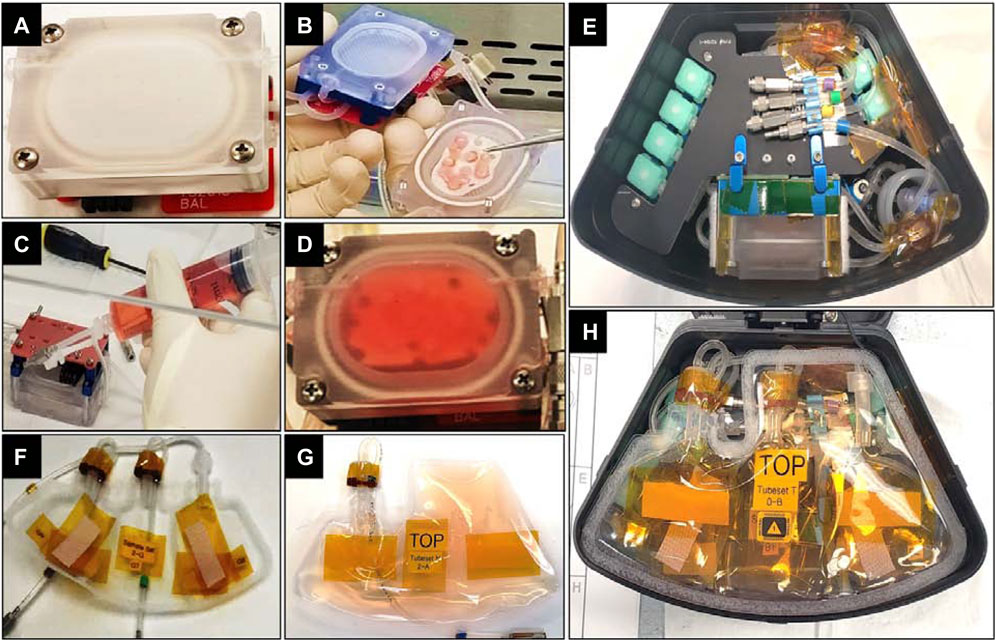
FIGURE 2. (A) Culture chamber capable of holding 15 mL media used for maintaining tissue cultures in a closed environment on ISS-NL. (B) Tissue card placed inside culture chamber. (C) Culture chamber, gas exchange loop and fluid loop were primed after closing of culture chamber to fill with media. (D) Culture chamber after being filled with media. (E) Culture chamber placed inside MVP module. (F) An empty, 3-compartment sample bag meant to collect media samples taken from the of culture chamber every 2 days (G) Nutrient bag with 60 mL of media meant to supply media for 3 media exchanges before being swapped with a fresh kit. (H) Nutrient & Sample Tubesets connected to culture chamber and placed on top of the assembly inside the MVP module.
Nutrient media preparation
Nutrient bags made of Fluorinated Ethylene Propylene (FEP) [Figure 2G] were designed to hold up to 60 mL media, allowing 3 media changes of 15 mL each once assembled into the MVPs [Figure 2H]. Nutrient kit-0 was attached in each module at the time of liftoff and was used to replenish media every 2 days once the study timeline was initiated by astronauts on the ISS. Almost-empty kit-0 was replaced in each of the 8 MVPs by the astronaut crew after 3 media exchanges, with a fresh kit-1 (kit swap on day 5, shown in the OPS timeline of Figure 3). Kits-2 and -3 were used at subsequent swaps (days 11 and 17). Two types of media were needed to prepare the nutrient kits. Media N was comprised of CO2 independent media (Thermo Fisher) containing 1% ITS (insulin-transferrin-selenium at 10 μg/mL, 5.5 μg/mL, and 5 ng/mL, respectively; Sigma #I2521), 20 mM MOPS buffer, 0.1 mM nonessential amino acids, 0.4 mM proline, 20 μg/mL ascorbic acid, 100 U/mL penicillin G, 100 μg/mL streptomycin, and 0.25 μg/mL amphotericin. Media T was identical in composition to media N but additionally contained treatment therapeutics, 100 nM Dex and 100 ng/mL IGF-1. Media N and T were prepared at MIT and shipped to SLSL 10–15 days before launch. Nutrient kits were filled and frozen at SLSL 10 days before launch and turned over to the cold stowage team 4–5 days before launch. In addition to nutrient kits, empty labeled sample bags [e.g., Figure 2F], sterilization, and disposal supplies meant for service events on ISS were also handed over to be stowed for launch. (See Supplementary Appendix A [and associated Supp Figs. 1a-h] for the need, validation, and use of CO2 independent media.)
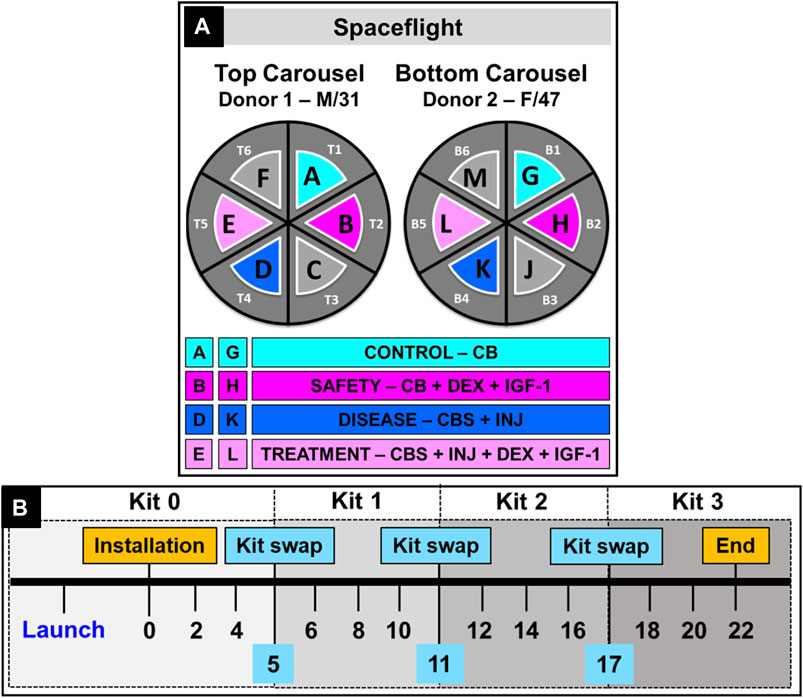
FIGURE 3. (A) Carousel utilization by modules in Techshot’s MVP facility in an EXPRESS rack on ISS-NL. Four groups from 2 donors (SF-Donor 1 and SF-Donor 2) were used to prepare 8 groups studied. (B) Experiment timeline of a typical study on ISS-NL showing time points for launch, installation, media sample collection and kit swaps.
Tissue card loading into culture chamber and MVP assembly
Tissue cards were shipped from MIT to SLSL within a few hours of preparation in a Biocontainer (World Courier shipping) that maintained temperatures between 15°C to ambient. Upon receipt at SLSL, the tissue cards were kept at 16°C until assembly into MVP modules. [See Supplementary Appendix A; and Supplementary Figures 1A–X for need and use of lower temperature storage before initiating experiments on ISS]. The process to load into MVP modules started 2 days before launch attempt 1 and was turned over to the cold stowage team 1 day before launch attempt 1, to be used for launch attempts 1 and 2, typically on 2 consecutive days. (In case launch attempts 1 and 2 were unsuccessful, a stand down period would follow, during which MVP modules would be re-loaded with new tissues in cards sent by the MIT team to SLSL as needed for subsequent launch attempts. In our case, launch attempt 2 was successful.).
The assembly of tissue cards into culture chambers and then MVPs at SLSL was performed using aseptic technique. After being taken out of the sterilization pouch, the unique serial number of each culture chamber was recorded for QA purposes. The transport tube containing the tissue card was photo-documented and transferred inside the biosafety cabinet. The tissue card was taken out and placed carefully inside the culture chamber [Figure 2B]. After closing the culture chamber, the process of media-priming was carried out during which the culture chamber, gas exchange loop, and fluid loop were filled with respective media [Figures 2C,D]. For operation with minimal crew involvement, this culture chamber was placed inside an MVP module, a single locker replacement payload with a microfluidic system that performed autonomous media change every 2 days [Figure 2E]. To accomplish this, the culture chamber was connected to the 3-compartment sample bag [Figure 2F] for spent media sample collection at the first 3 time points on ISS. The nutrient kit containing fresh media [Figure 2G] was connected next and placed on the top of the assembly [Figure 2H]. The module was then closed, and the same process was repeated for all modules. The modules were placed at 16°C until handover to cold stowage at KSC (approximately 1 day before launch attempt 1), and then stowed in the Dragon cargo hold at 16°C until launch.
Upon launch, the Dragon capsule reached ISS about 2 days later, and the modules were unloaded within 24 h after docking. The modules were installed in carousels (Figure 3A) inside Redwire‘s MVP facility, which was already present on ISS inside an EXPRESS rack (EXpediate the Processing of Experiments to the ISS), followed by a gradual increase of temperature to 37°C. This was accompanied by the first media change and collection of day 0 media samples on the ISS. In the following days, an autonomous media swap occurred every 2 days when 15 mL of fresh media moved from the nutrient kit into the culture chamber, replacing spent media which was pumped out and collected into a sample bag. After 3 media swaps in each module, the nutrient kits were replaced with fresh media: the crew removed the MVPs from the carousels, opened the lids, removed the used nutrient kits and the filled sample bags, and new nutrient kits and empty sample bags were connected before closing the modules and placing them back into the carousels. The timeline for all crew service events (SE) is detailed in Supplementary Table S1.
Post spaceflight inflammation and biochemical analyses
Immediately after removal from MVP modules while on the ISS, the crew froze the sample bags (−80°C), which remained frozen until received at MIT. At the end of the experiment, the culture chambers with the tissue cards inside them were also frozen. Complete return of all tissue and media samples occurred by May 2021. Following receipt at MIT, the sample bags and culture chambers were thawed overnight at 4°C and then photo-documented [e.g., Supplementary Figures 2A, B]. The thawed media from each bag was then transferred into a 15 mL tube and the volume was recorded. At this time, aliquots were prepared for subsequent spent media-based assays to prevent repeated freeze-thaw. The thawed culture chambers were carefully opened, and media was collected into a 15 mL tube. The tissue card was taken out, photo-documented [Supplementary Figures 2C, D] and wet weights of each tissue were recorded to normalize biochemical data. Upon examination of chamber “B” [Figure 3] post-flight, housing the drug-safety condition for Donor 1 (CB + DEX + IGF-1), contamination was discovered; thus, data from this one condition are not included; all 7 other MVP chambers were contamination-free.
Since 6 tissue sets were cultured together in each tissue card in the 15 mL-culture chamber for SF, each media sample collected represented a pooled sample from these n = 6 tissues. Tissue-based assays were performed separately on each tissue (n = 6) and normalized using respective tissue wet weight. Spent media assays (120 in total) were performed on pooled samples and results are reported as concentrations here. Tissue release of cytokines to the medium was assessed for all time points using the Human V-Plex Plus proinflammatory 10 spot panel kit from Meso Scale Discovery (Rockville, MD) following the manufacturer’s directions. Ten cytokines relevant to human joint injury (Struglics et al., 2015), including 7 pro-inflammatory (IL-1β, 1L-2, IL-6, IL-8, IL-12p70, IFN-γ, TNF-α) and 3 anti-inflammatory (IL-4, IL-10, IL-13) cytokines were analyzed. Tissue samples from days 0, 2, 6, and 14 were digested and used to measure the amount of remaining intra-tissue sGAG, collagen, and DNA using the methods previously described (Dwivedi et al., 2022). Briefly, tissues were digested in 500ul proteinase K solution (5 mg/mL in 50 mM Tris-HCl, 1 mM CaCl2, pH8) (Roche, Indianapolis, MN) at 56°C for 16 h sGAG content of cartilage digests and spent media was determined using the dimethyl methylene blue assay (DMMB assay) (Farndale et al., 1986). Total collagen was assessed in tissue digests that were hydrolyzed and dried using the hydroxyproline (OHP) assay (Sierra et al., 2015) and normalized to wet weights. The concentration of CTX-II fragments, a known biomarker of cartilage collagen degradation, was determined using a Sandwich-ELISA assay following manufacturer’s instructions (AC-08F1, IDS, Gaithersburg, MD, for CTX-II) as previously described (Dwivedi et al., 2022).
Measurement and analysis of untargeted metabolomics
Metabolomics studies focused on circulating metabolites and were performed using spent media samples from tissue cultures of donors 1 and 2 (both ground control (GC) and spaceflight (SF)). To focus on early alterations in circulating metabolites, spent media were pooled from multiple wells on each culture day 2 and 7 of GCs, and were collected on culture days 0, 2, and 6 of SF experiments. Frozen spent media samples were shipped to Human Metabolome Technologies America, Inc. (HMT; Boston, MA, United States) for global metabolite profiling. Sample preparation was carried out using the previously described protocol (Mina et al., 2019). Metabolome analysis was performed using capillary electrophoresis Fourier transform mass spectrometry (CE-FTMS) in two modes for cationic and anionic metabolites. Over 320 metabolites were detected on the basis of HMT’s standard library. Prior to analyses, data were cleaned by removing the metabolites that were not detected (N.D.) in the majority (>80%) of the samples. For metabolites that were N.D. in some samples, relative abundance values were assigned as 5-fold lower than the lowest value measured in the whole dataset. Log transformation and pare-to scaling were then performed on the dataset before analyses. Statistical significance was assessed using two-way ANOVA with FDR correction for multiple testing. All computation was performed using MetaboAnalyst and in R.
Mass spectrometry and analysis of media proteomes
Protein isolation and trypsinization from pooled week 1 and week 2 media samples, discovery MS, and protein quantification were performed as previously described (Black et al., 2022). Ground samples were first searched using n = 10 donors, the 2 donors used in the present space flight study along with 8 additional donors processed in our MIT lab as described previously (Black, 2022; Dwivedi et al., 2022). Based on this n = 10 ground study, we selected proteins having a significant effect of [CBS + INJ] vs. control (using the R package limma and a linear mixed effects model with donor as a random variable, and least squares means test for p values performed on all 10 ground donors). We then separately looked at the behavior of those proteins (selected as significant for [CBS + INJ]) in the two space donors compared specifically to their two corresponding ground donors 1 and 2. MS data were searched in two batches: all n = 10 ground samples, and then the space flight samples with their corresponding ground controls (donors 1 and 2) separately. For both the ground and space data sets, proteins were filtered out if they were exogenous (e.g., from ITS media supplement) or not identified and quantified in at least 70% of samples, and missing values were imputed using the k-nearest neighbor method (Lazar et al., 2016; Black et al., 2021). Individual peptide abundances for collagen I (COL1A1) and collagen II (COL2A1) were filtered and imputed via the same methods. Pairwise comparisons between treatments were performed using limma and MATLAB (MathWorks) (Ritchie et al., 2015). Protein abundance data were log2-transformed and scaled, and principal component analysis (PCA) was performed using the “prcomp” function (Black et al., 2020). PCA protein loadings were ranked by their dot product and analyzed using Gene Set Enrichment Analysis (GSEA) c5 gene sets with the Human UniProt IDs chip and 1,000 repeats for enrichment score distributions (Mootha et al., 2003; Subramanian et al., 2005). For comparison of space and ground effects on the same proteins, proteins with a significant (adjusted p-value <0.05) effect of CBS + INJ treatment across all ground samples were selected. Within Donor 1 and Donor 2, the log2 fold change of CBS + INJ versus CB samples were found for the proteins both identified in space samples and with a significant effect across all samples. Differences in space and ground responses were assessed by taking the square of the difference between the space fold change and ground fold change. Enrichment analysis for biological processes was performed using STRING analysis as previously described (Szklarczyk et al., 2019; Black et al., 2020).
Ground studies
Ground studies were set up using tissues from the same donors studied on ISS (donors 1 and 2) and performed at MIT under conditions similar to those described from our recently published studies (Dwivedi et al., 2022). Tissues were cultured in 24 well plates in DMEM complete culture medium in a 5% CO2 incubator for 14 days. CB plugs were used to set up control (CB) and safety groups, where the latter was cultured in the presence of therapeutics 100 nM Dex and 100 ng/mL IGF-1 (CB + Dex + IGF-1) to ensure the safe application of therapeutic drugs on healthy tissue. CBS + INJ cultures were performed as previously described (Dwivedi et al., 2022), both in the absence and presence of therapeutics (i.e., treatment, CBS + INJ + DEX + IGF-1). The viability of the cartilage tissue was verified on day 14 using FDA/PI staining as described. Spent media samples were collected on days 2, 4, 7, 9, 11, and 14 (n = 6 technical replicates at each day for each treatment condition). Tissue samples (also n = 6) were frozen on day 14 for biochemical analyses. Spent media samples from 6 replicates were pooled for MSD (inflammation) and CTX-II assays. Biochemical, metabolomic and proteomic assays were performed as described above for space flight samples.
Results
In the subsections below, we compare observations and data from spaceflight with those of the associated ground controls for each outcome measure (inflammatory, biochemical, metabolomic and proteomic). In general, the qualitative trends of the ground control data reported here are very similar to those reported in our recently published laboratory study involving 25 knees from 16 donors, using this same newly developed in vitro microphysiological system. Along with this large background dataset, we further suggest similarities and differences associated with spaceflight in the Discussion.
Cell viability
Tests using initial donor joints showed that cartilage and bone explant cells remained viable for the DMEM, and CO2 independent media used at the various temperatures needed for launch-preparation and culture on ISS [Supplementary Figures 1A–H]. Cartilage tissue specifically from Donors 1 and 2 used for spaceflight was assessed at the time of donor receipt [Figures 1C,D] and at the end of 14 days in ground controls. By day 14, minimal cell death was observed in the osteochondral-alone (CB) groups, mostly limited to superficial zone of the cartilage [Figures 4A,B]. However, as observed previously (Dwivedi et al., 2022), mechanical impact injury and coculture with synovium (CBS + INJ) caused an increase in cell death in all the tissue sections in this group [Figures 4C,D]. Importantly, the combination of Dex + IGF-1 greatly reduced cell death caused by CBS + INJ, as indicated by the mostly green cells in [Figures 4E,F]. Comparably little cell death was observed in the healthy CB-alone tissue cultured with Dex + IGF-1 [Figures 4G,H], confirming the safety and efficacy of this combination of drugs used in the present study. While cell viability on the ISS could not be directly confirmed at the end of spaceflight experiments (since tissues were then immediately frozen on ISS), the additional outcomes reported below strongly suggest that explants/cell did not differ substantially from that of ground controls.
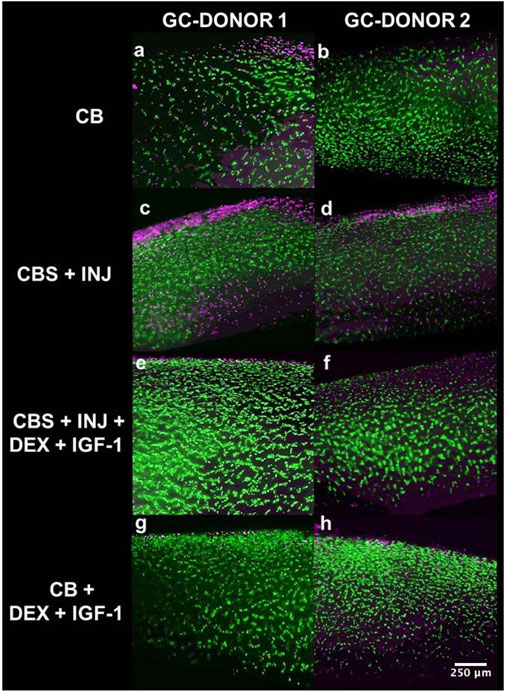
FIGURE 4. (A–H): FDA/PI stained cartilage sections from ground control (GC) studies of Donors 1 and 2 at the end of 14 days. Chondrocyte viability was maintained in CB group (A and B). Increased cell death was observed in the disease group (CBS + INJ) as a result of mechanical injury and synovial inflammation (C and D). Treatment with Dex + IGF-1 ameliorated cell death in PTOA-like (CBS + INJ) conditions, and most of the tissue was viable with few dead cells, primarily in superficial zone (E and F). Uninjured osteochondral plugs (CB) treated with Dex + IGF-1, i.e., the drug-safety group, showed comparable viability to uninjured CB control plugs (G and H), suggesting that there were no adverse effects of Dex + IGF-1 drug treatment on chondrocyte viability. GC: Ground control samples.
Cytokine release increased by CBS + INJ, reduced by Dex + IGF-1 with donor variability
Levels of 7 pro-inflammatory (IL-1, TNFα, IL-6, IL-8, IL-2, IL-12, IFNγ) and 3 anti-inflammatory (IL-4, IL-10, IL-13) cytokines were analyzed to assess the extent of inflammatory initiation of PTOA-like disease and the response to treatment with Dex and IGF-1. Previous studies showed donor-dependent variability in absolute concentrations but similar trends in the release of inflammatory cytokines (Dwivedi et al., 2022). The ground control studies here showed an immediate increase in the release of all 10 cytokines analyzed 2–4 days after initiation of CBS + INJ, followed by a gradual decrease though, in some cases, concentrations remain elevated over 14 days [Figure 5, Supplementary Figure 3]. In contrast, control (CB) and safety (CB + Dex + IGF-1) groups were typically associated with lower levels of released inflammatory cytokines, consistent with previous studies (Dwivedi et al., 2022) showing that synovium is the primary source of inflammation in this model system.
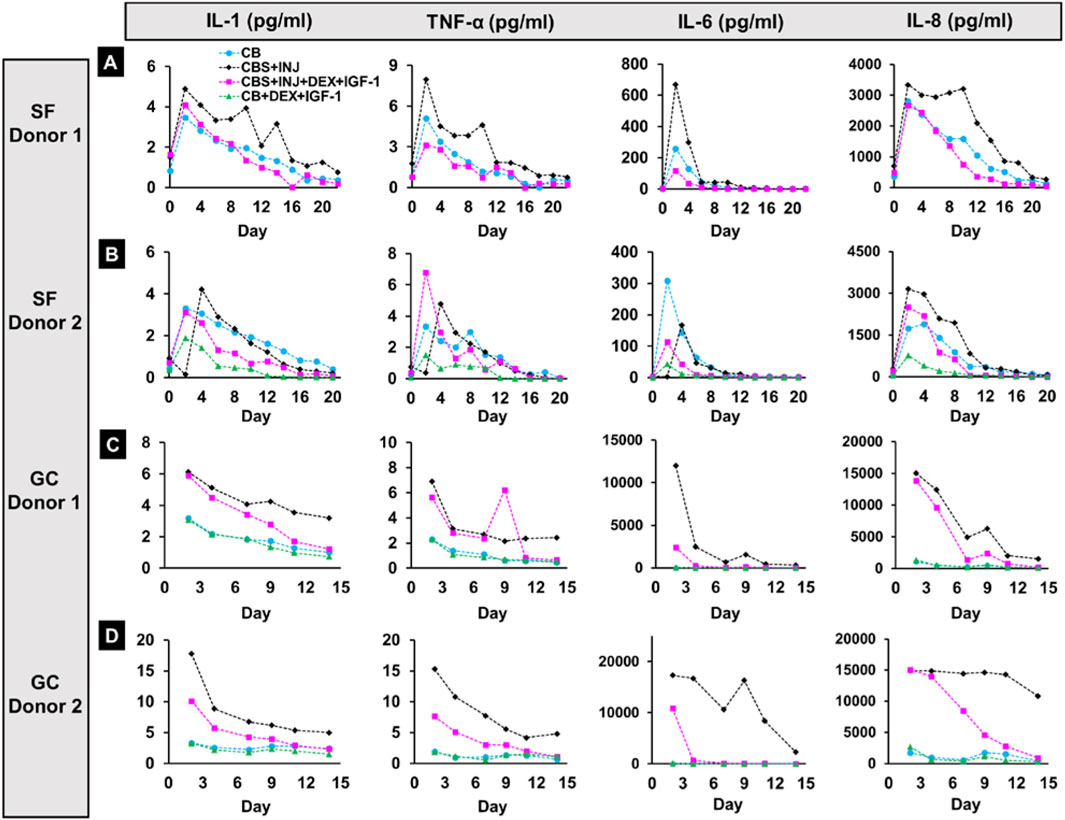
FIGURE 5. Panels showing levels of 4 pro-inflammatory cytokines, IL-1, TNF-α, IL-6, and IL-8, released in Space and on Earth. Rows (A and B) 2 donors from Spaceflight (SF-Donor 1 (A) and SF-Donor 2 (B)). Rows (C and D) Cytokine concentrations were also measured in corresponding ground studies from donors sent into Space (GC-Donor 1 (C) and GC-Donor 2 (D)). SF: Spaceflight samples. GC: Ground control samples.
With donor 1 in space, increased release of inflammatory cytokines was observed on day 2 in all treatment groups for all cytokines studied, with the maximum elevation most often in the CBS + INJ disease group [Figure 5 and Supplementary Figure 3]. Although levels were higher in the first week, treatment with Dex + IGF-1 tended to reduce the inflammatory response to levels comparable with the CB group starting on day 4 or 6. In the respective ground study from the same donor 1, a strong inflammatory response was observed for CBS + INJ with or without drug treatment in the first week of culture. While treatment with Dex + IGF-1 tended to reduce the release starting week 2 comparable to the CB group, levels of inflammatory cytokines were considerably elevated in the CBS + INJ group.
With SF donor 2, an increase in the levels was observed on day 4 instead of day 2 for most cytokines in response to CBS + INJ (except for IL-8 on day 2 and IL-12 on day 6). Surprisingly, however, cytokine release levels also tended to be high even in the control CB group and the drug treatment group, especially in the early phase of the culture [Figure 5, Supplementary Figure 3]. Dex + IGF-1 treatment generally reduced the inflammatory response by week 2 for most cytokines. In the corresponding ground study from the same donor 2, release of cytokines in the disease (CBS + INJ) group was 2–5 times higher at day 2 compared to that for donor 1, except for IL-6 and IL-8 which were similar for both donors. Dex + IGF-1 treatment caused a marked reduction in the release of cytokines from day 2 onwards (except IL-8, which decreased at later times), reducing the severity of inflammatory response from the early stage of culture. Unlike spaceflight studies from the same donors, low release was observed for CB control and safety-treatment groups in both GC-donor 1 and 2 groups. Taken together, donor variability was evident even between the 2 donors studied on Earth. Moreover, treatment with Dex and IGF-1 was more effective in reducing the inflammatory response in GC-donor 2 from the earliest time in the culture. But importantly, many cytokines showed elevated release from SF tissues for most if not all treatment conditions from both donors, in contrast to GC tissues.
Biochemical analysis reveals increased GAG loss in disease group in space and ground
During 3 weeks in space and 2 weeks in ground controls, release of sGAG to the media was consistently higher in the disease (CBS + INJ) group compared to CB control treatment for both donor tissues [Figure 6]. For donor 1, addition of Dex + IGF-1 reduced sGAG loss in the CBS + INJ + Dex + IGF-1 group down to levels of CB control on Earth [Figure 6B], and partially reduced sGAG loss in space as well [Figure 6A]. However, Donor 2 tissues did not similarly respond to Dex + IGF-1 on earth or in space by the 2–3 weeks time point in culture [Figures 6C,D]. This human donor variability to such therapeutic treatment has been seen previously using this same microphysiological system with multiple laboratory studies (Dwivedi et al., 2022) as well as with cultures of osteochondral plugs with added exogenous cytokines (Black et al., 2022) No differences were found between treatment groups on Earth or in Space for total collagen content or release of CTX-II fragments, for both donor tissues [Supplementary Figure 4]. Very low levels of CTX-II were detected in SF and GC samples, as expected at this early stage of culture (see Discussion).
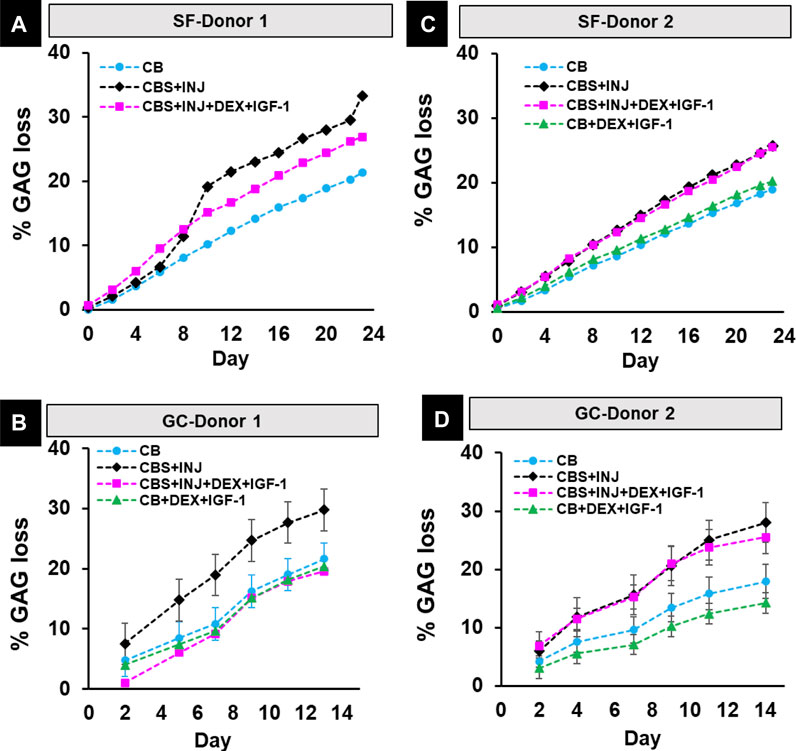
FIGURE 6. (A–D): % GAG loss in spaceflight study in SF-Donor 1 (A) and SF-Donor 2 (B) and their corresponding ground studies in GC-Donor 1 (C) and GC-Donor 2 (D). Data are means ± SEM. SF: Spaceflight samples. GC: Ground control samples.
Metabolomics shows increased oxidative stress and inflammation under spaceflight conditions
Metabolic signatures and group differences were studied using the spent media samples collected from SF Donor 1 and 2, and GC Donor 1 and 2. Principal component analysis (PCA) performed on all samples showed that 69% variance was explained in the first 2 principal components [Figure 7A]. More importantly, samples formed two separate clusters on PC1 for ground control and spaceflight, showing that spaceflight conditions had a more pronounced effect on metabolic signatures compared to groups differences such as disease-associated or drug-induced effects. PC2, on the other hand, showed those group differences, both in the ground control cluster and the spaceflight cluster, where disease (CBS + INJ) samples were grouped closer to drug treatment (CBS + INJ + DEX + IGF-1) samples, and control (CB) samples were grouped closer to safety (CB + DEX + IGF-1) samples [Figure 7A].
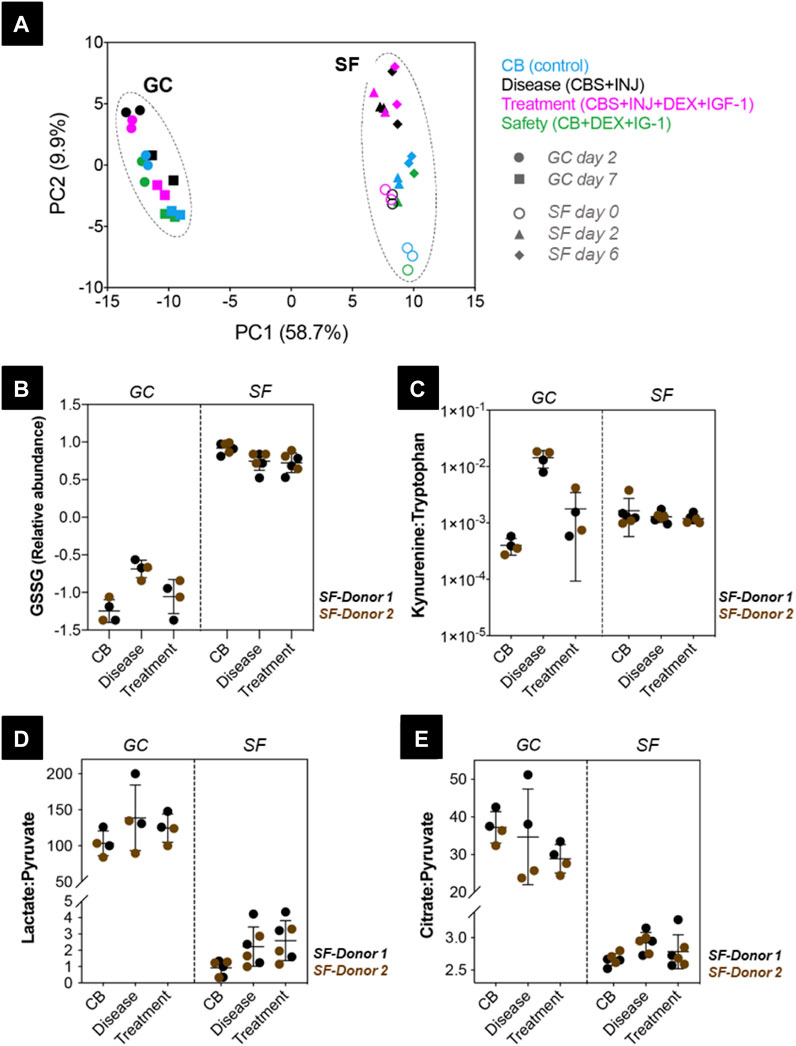
FIGURE 7. Circulating metabolites measured in ground control (GC) and spaceflight (SF) samples from Donor 1 and 2. (A) Principal components analysis scores plot showing GC samples on culture day 2 (circles) and day 7 (squares), and SF samples on day 0 (open circles), day 2 (triangles) and day 6 (diamonds) clustering separately. In both GC and SF samples, CB (control, blue), disease (CBS + INJ, black), treatment (CBS + INJ + DEX + IGF-1, magenta), and safety (CB + DEX + IGF-1, green) groups are shown. (B) Relative abundance of oxidized glutathione (GSSG) compared in GC and SF samples for both Donor 1 (black) and 2 (brown). (C) Kynurenine: Tryptophan (Kyn:Trp), (D) Lactate:Pyruvate (L:P), and (E) Citrate:Pyruvate (Cit:Pyr) ratios measured in GC and SF samples are compared between CB, disease, and treatment groups for Donor 1 (black) and 2 (brown). SF: Spaceflight samples. GC: Ground control samples.
Since PCA analysis showed that the greatest variance was between ground vs. space and between treatment conditions, but not between time points, we then grouped days 2 and 7 for ground, and days 0, 2, and 6 together for space, and statistical tests (see Methods above, and (Dwivedi et al., 2022)) were performed based on these groupings. To assess the regulation of cellular functions under ground control and spaceflight conditions, metabolic markers of redox status, central carbon metabolism, and inflammation-activated metabolic pathways were studied. Redox status, mostly informed using ratios of glutathiones (GSH: GSSG) and pyridine nucleotides (NADH: NAD+, NADPH: NADP+), was assessed comparing measured oxidized glutathione (GSSG) abundance between groups. In GC samples, GSSG was increased under disease conditions (CB vs. disease; padj < 0.0001) and reduced with drug treatment (disease vs. treatment; padj = 0.0101) [Figure 7B]. On the contrary, in space, GSSG was slightly higher in control samples and comparable in disease and drug treatment samples (padj > 0.05 for CB, disease, treatment group comparisons). Overall, GSSG abundance under spaceflight conditions was very high compared to GC, suggesting increased oxidative stress for all groups in spaceflight conditions.
Kynurenine (Kyn) pathway activation has been associated with multiple inflammatory diseases and was observed in our previous study (Dwivedi et al., 2022) as measured kynurenine in CBS spent media, which was not detected in control (CB) groups. This metabolic pathway is regulated by inflammatory cytokines, and the activation can be informed using the Kyn:Trp ratio (Dwivedi et al., 2022). In GC samples, Kyn:Trp ratio was higher under disease conditions (CB vs. disease; padj < 0.0001) and reduced with drug treatment (disease vs. treatment; padj < 0.0001) [Figure 7C], in agreement with the measured inflammatory cytokine profiles [Figure 5]. On the contrary, in space, the Kyn:Trp ratio was comparable in control, disease and drug treatment groups (padj > 0.05 for CB, disease, treatment group comparisons), and ratios in SF groups were higher than GC control (CB) samples. Considering that Kyn pathway was not activated in GC control (CB) groups, these results suggest that all groups in SF show inflammation induced Kyn pathway activation to a similar degree.
Two metabolic markers, Lactate: Pyruvate (L:P) ratio and Citrate: Pyruvate (Cit:Pyr) ratio, were used to evaluate differences in central carbon metabolism under spaceflight conditions. In GC samples, L:P ratio was comparable in control, disease, and drug treatment groups [Figure 7D], In space, the ratio for disease and drug treatment groups was higher (padj > 0.05 for CB, disease, treatment group comparisons). Overall, the L:P ratio was almost 100-fold lower in the spaceflight samples (any GC vs. any SF group comparison; padj < 0.0001). This decrease resulted from a ∼3-fold decrease in lactate relative abundance, and ∼10-fold increase in pyruvate relative abundance in SF samples compared to the GC samples [Supplementary Figure 6]. Similarly, the Cit: Pyr ratio was found to be ∼10-fold lower in the SF samples compared to GC samples. These results show that central carbon metabolism signatures were distinct between GC and SF experiments, and possibly suggest higher glycolysis and suppressed TCA cycle activity [Figure 7 and Supplementary Figure 6] under spaceflight conditions.
Comparison of media proteome changes on the ground versus in space shows differences in metabolic and bone biomarker release
Principal component analysis [PCA, Figure 8] was performed on the ground and space samples for week 1 [Figures 8A,C] and week 2 [Figures 8B,D] for donors 1 [Figures 8A,B] and 2 [Figures 8C,D]. Across all four analyses, the most significant effect on sample variability was the separation of space versus ground samples, with the second largest contribution from CBS + INJ versus CB controls or only CB + Dex + IGF-1. Dex + IGF-1 treated samples did not separate much from their untreated counterparts, particularly for donor 2. GSEA was performed on the ranked protein loadings from the first principal component for each data set to find which proteins were more associated with the SF samples versus the GC samples. SF, but not GC, was consistently associated with proteins related to desmosomes such as cystatin A, desmocolins, desmoplakins, and plakophilin 1. GC samples were broadly associated with intracellular metabolic proteins such as protein disulfide isomerases, glucose-processing proteins, and those involved in endoplasmic reticulum function.
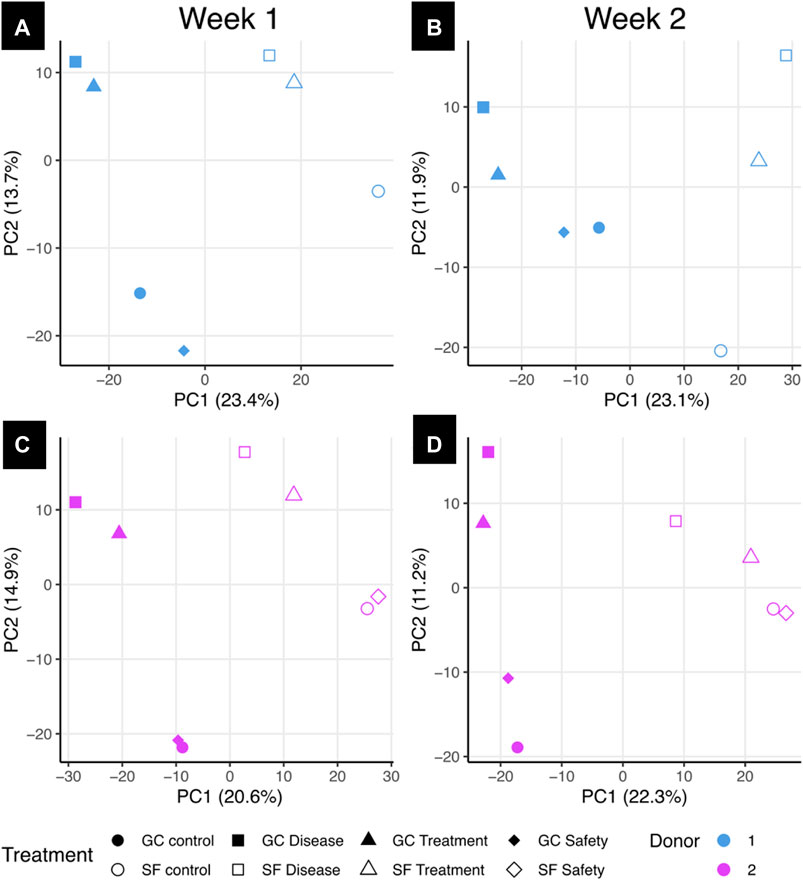
FIGURE 8. Principal component analysis of space and ground samples using proteomics data. Principal component analysis (PCA) was performed using protein abundance values from donors 1 (A and B) and 2 (C and D) for treatment conditions on both ground and space from the first or second week of treatment. Percentages on axes represent percent variance explained by that principal component. GC: Ground control samples, SF: Spaceflight samples, Control: osteochondral plug alone; Disease: CBS + INJ (osteochondral plug with mechanical injury cocultured with synovial explant; Treatment: CBS + INJ + Dex + IGF-1; safety: CB + Dex + IGF-1.
To compare whether changes on proteins in the ground control samples were recapitulated in space, we selected proteins with a significant effect of CBS + INJ on GC and filtered for proteins also identified in the SF samples. The log2 fold change of CBS versus control within each of donor 1 and donor 2 for week 1 and week 2 were calculated for the ground and space samples, and ground and space fold changes plotted against each other [Figure 9]. Proteins were highlighted if the mean squared difference between the two was greater than 5, and the absolute value of the fold changes for both space and ground were greater than 1 (i.e., a two-fold increase or decrease). Highlighted proteins in Figure 9 are summarized in Supplementary Tables S2, S3. Notable proteins for donor 1 that were not highlighted include IL-6, which had no change in the release in SF in week 1 but a large increase in the GC samples; collagen II, which had a decrease with CBS + INJ treatment in the GC samples and a slight increase in SF; and collagen VI (A1 and A2 chains), with a greater increase in SF compared to GC. Donor 2 also had higher amounts of collagen II and collagen IV release in space for week 1 compared to decreases in their release in the ground samples. For both donors across both weeks, there were greater increases in sclerostin (SOST), osteomodulin (OMD), and osteonectin (also known as SPARC) in SF media compared to GC.
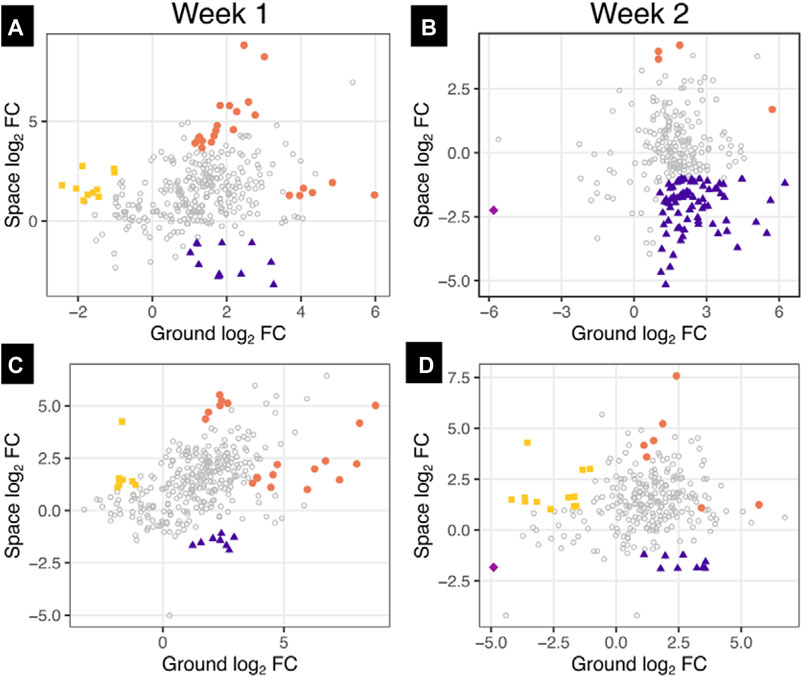
FIGURE 9. Differences between space and ground culture on media abundances of proteins affected by disease progression in comparison to control. Proteins with a significant effect of CBS + INJ versus osteochondral controls (CB) on the ground were selected and filtered for proteins also identified in space samples. The log2 fold changes (FC) between CBS + INJ and CB were determined within just donors 1 (A and B) and 2 (C and D) on the ground and in space for each of week 1 (A and C) and week 2 (B and D). Each point represents an individual protein, and proteins are colored if their log2 FC for both ground and space were greater than 1 or less than −1, and the mean squared difference between space and ground were greater than 5, indicating a large difference between the behavior in ground and space as well as a noticeable change in both ground and space. NOTE: The color key corresponds to notation of Supplementary Tables S2, S3.
Changes in collagen synthesis identified via analysis of peptide abundances
Collagen I and II synthesis was assessed in donors 1 and 2 via analysis of the individual peptides identified corresponding to those collagens. Comparing CBS + INJ treatments to CB alone, collagen II synthesis (assessed by changes in the N- and C-terminal peptides) was only increased in SF and not in GC tissues over the first 2 weeks: in the first week for donor 1, and the second week for donor 2 [Supplementary Figure 5]. Collagen I synthesis followed the same trend, with increases in N- and C- terminal peptides indicative of new synthesis only in space with CBS + INJ treatment.
Discussion
Space exploration has been an exciting journey for the human race, but possible adverse effects on astronaut health have long been recognized. In addition to microgravity, the crew is also exposed to radiation, high g-forces, the effects of intensive exercise on musculoskeletal tissues while on the ISS, and impact upon landing (Jones et al., 2000) It is no surprise that musculoskeletal complaints are one of the most reported in-flight problems (Scheuring, 2010). To prevent risk to future crew missions, timely therapeutic intervention is critical. Post-traumatic osteoarthritis provides an excellent focus to explore the origin of joint tissue breakdown after injury and potential pharmacological treatments to protect astronauts’ present and future joint health. Microphysiological systems are an effective method to recapitulate the human physiology of PTOA and to advance understanding of disease biology and therapeutic target (Reardon, 2015; Hinman et al., 2020) Our microphysiological system (Dwivedi et al., 2022) incorporates coculture and crosstalk between native human cartilage, bone, and synovium, three critically important knee tissues currently under intense study as principal sites for initiation and progression of OA/PTOA (Oo and Hunter, 2022).
Cell death in the cartilage tissue is one of the most detrimental outcomes of mechanical trauma which may be a contributing factor in the development of PTOA (Kim et al., 2002; Murray et al., 2004). Since SF tissues were frozen on ISS before return to Earth, it was not possible to assess cell viability. However, we can still compare cell/tissue viability in space to that after similar treatment conditions in GC and our lab studies (Dwivedi et al., 2022). Those results showed that CBS + INJ caused dramatic increases in chondrocyte death, while Dex and IGF-1 rescued cell viability (e.g., Figure 4). Cartilage tissue showed negligible cell death in the CB + Dex + IGF-1 safety group, suggesting the safety of this treatment combination. Further insight into cell behavior in space is derived from our proteomic results, which showed a significant effect of spaceflight on media proteome composition. Here, proteins associated with desmosomes were prominent in SF media samples compared to GC across all treatment conditions. This finding could be linked to the proliferation of lacunar chondrocytes identified in previous studies of OA progression (Lotz et al., 2010; Brink et al., 2019). The increase in desmosomal proteins is likely not only due to changes in the cartilage tissue: in cases of traumatic arthritis, more desmosomes were observed from synovial cells (Ghadially et al., 1978). In addition, GC samples were associated with higher release of intracellular metabolic proteins, which are considered to be markers of necrotic cell death, particularly early in culture (Roth and Adelman, 1975). This suggests that early cell death events are not occurring in space. However, exposure to the conditions of space may cause proliferation and aggregation of cells together with corresponding increases in the amount of desmosomal junctions, which may replicate the behavior seen during OA progression. Future studies are needed to validate this hypothesis.
Regarding cytokine release, we first note that the levels of cytokines were low on day 0 in the Spaceflight samples. This is to be expected since the samples were being held at 16°C prior to MVP installation at the ISS where the temperature was raised to 37°C. Lower temperature likely helped to maintain tissue viability, but in a state of low metabolic activity. The motivation for this experimental design was to ensure that the study started upon reaching the ISS. After the tissue cards were prepared at MIT and assembled at SLSL in Florida, the synovium would have continued to release inflammatory cytokines if maintained at 37°C during the time they were held in cargo in preparation for launch. This would have been undesirable since the goal of the study was to assess the progress of disease and response to treatment in microgravity. Thus, the low cytokine levels in media collected on day 0 indicated that synovium was likely in a state of low metabolic activity until the temperature was raised to 37°C on the ISS. This is consistent with the increase in the cytokine levels observed on days 2, 4 and onwards. Donor-related variability in absolute concentrations of released cytokines is consistent with our earlier laboratory findings utilizing a large donor set (Dwivedi et al., 2022). Regardless, a few general trends were evident. In the SF as well as GC, the highest levels of released pro-inflammatory cytokines were mainly from the disease (CBS + INJ) group. A considerable spike was typically observed during week 1 followed by a gradual reduction during the rest of the experiment, also consistent with previous studies (Swärd et al., 2012; Bigoni et al., 2013; Dwivedi et al., 2022). Importantly, in comparing SF with GC results for cytokine release, it is notable that many cytokines showed elevated release from SF tissues for many if not most experimental conditions from both donors, in contrast to GC tissues where cytokine release was generally highest for the disease (CBS + INJ) compared to other conditions (especially GC donor 2 compared to SF donor 2).
Degradation of extracellular matrix, such as the release of aggrecan-GAG fragments, is an important hallmark of PTOA disease and often one of the earliest noted changes in cartilage. We observed loss of GAG to the medium to highest for disease (CBS + INJ) compared to CB controls in both donors in SF and GC cultures [Figure 6]. This important observation is consistent with the results of many previous in vitro studies as well as clinical observations of patient synovial fluids shortly after ACL-rupture (i.e., acute knee injury) (Swärd et al., 2012). Proteomic analyses have further highlighted additionally important observations that distinguish SF from CG conditions. Over 2 weeks of culture, CBS + INJ caused increases in the release of many additional proteins associated with extracellular matrix composition and organization, though the extent of such changes was milder compared to published in vitro disease models that used much higher concentrations of exogenous cytokines to represent the effects of inflammation (Black et al., 2021) (compared the cytokine concentrations released by synovium explants in our MPS). In addition to the changes in collagen synthesis and abundance described above, collagens VI, XV, and XVIII, all pericellular matrix collagens, had a higher release in space compared to GC samples in the second week for donor 2, suggesting either more of an anabolic response with new deposition of these proteins in response to disease progression in space, or early catabolic breakdown events (Pufe et al., 2004; Smeriglio et al., 2015; Bretaud et al., 2020). An increased anabolic response is supported by collagen II synthesis [Supplementary Figure 5], an important part of the cartilage reparative response to OA progression, not being decreased with CBS + INJ treatment in space as it was for ground for either donor. Donor 1 (though not donor 2) in space showed higher levels of peptides from the propeptide regions of collagen I, indicative of collagen I synthesis and bone osteoblast activity. The biomarkers of bone health OMD, SPARC, and SOST, all also had greater increases to the media under disease stress in space than ground (for both donors), perhaps a result of bone reacting to being in space and striving for healthier bone metabolism (Eastell and Lobo, 2007; Chang et al., 2018; Lin et al., 2021).
Donor-dependent variability was also found in the effects of treatment with Dex + IGF-1, exacerbated by the SF instrumentation limit of using at most 2 donors. While drug treatment reduced GAG loss to CB control levels for GC-donor-1 and moderately reduced GAG loss for SF-donor-1 as well, treatment was ineffective at preventing GAG loss for donor 2 under both SF and GC conditions [Figure 6]. On earth, using a much larger donor pool (25 knees from 16 donors), preliminary studies have revealed a statistically significant decrease in GAG loss under CBS + INJ + Dex + IGF-1 compared to CBS + INJ with no drugs (Dwivedi, 2019); however, with large donor-to-donor variations in cytokine release and response to such therapeutics, it is difficult to identify effectiveness when looking at any two donors alone. Dex + IGF-1 had little effect on attenuating changes to the media proteome caused by CBS + INJ disease progression (Black, 2022), and PCA of space samples also showed little deviation between CBS + INJ versus CBS + INJ + Dex + IGF-1 treatments. Dex and IGF-1 did increase the release of metallothioneins, which are known to be protective against oxidative stress (Ruttkay-Nedecky et al., 2013). It is well-established that Dex treatment drives synthesis of metallothioneins, indicating a potential mechanism for therapeutic benefit, especially in the high-radiation environment of space (Karin et al., 1980). These results suggest that the impact of early intervention with Dex + IGF-1 is to prevent matrix catabolism and cytotoxic events, without many off-target effects detectable in what was released to the media. It is notable that Dex increased the release of IGF binding protein 6, which could bind to IGF and decrease its activity (Tanaka et al., 2021). In general, donor-to-donor variability could be related to donor age, sex, and a variety of other variables. An age-related decline in response to IGF-1 has also been reported before (Loeser et al., 2000), and current approaches to delivery of IGF-1 (and other proteins) could mitigate such effects (Geiger et al., 2018). This is an important consideration for the flight physician to ensure more personalized pharmacological intervention based on an individual’s physiological characteristics, medical history, fitness level and inherent pre-existing propensities.
Finally, we note that the literature suggests that it is highly unlikely that a single compound will exist that can reduce tissue degeneration and, simultaneously, promote tissue regeneration following joint injuries that cause OA/PTOA (Wieland et al., 2005). Hence our approach to combine the use of an anti-catabolic, anti-inflammatory corticosteroid (Dex) to reduce degeneration, and a pro-anabolic growth factor (IGF-1) to promote regeneration. The concentrations we used were motivated by dose-response studies in the literature for IGF-1 (Tyler, 1989; Bonassar et al., 2000) and Dex (Lu et al., 2011). In terms of drug administration, we note that, like most in vitro studies, the concentrations of Dex and IGF-1 in all our GC and SF experiments are maintained constant. Following joint injuries, intra-articular injection of drugs is currently the primary clinical approach; however, injected drugs are known to be cleared from the joint within hours, well before they can penetrate into cartilage or other tissue targets. Therefore, we have separately focused on studies to develop drug delivery techniques to achieve more sustained, intratissue delivery using cationic nanoparticles conjugated to either IGF-1 (Geiger et al., 2018) or Dex (Bajpayee et al., 2016; Bajpayee and Grodzinsky, 2017), to deliver them into cartilage. In addition, we have developed a predictive mathematical model to predict the transport of IGF-1-based fusion proteins into human knee joints upon intra-articular injection (Krishnan et al., 2022), including penetration into cartilage and escape into systemic circulation through the joint capsule. Together, the hope is to set the stage for translation of such in vitro culture studies to in vivo preclinical studies and potentially clinical application.
Conclusion
We have used our recently published native human osteochondral-synovium explant co-culture system (Dwivedi et al., 2022), confirmed to reveal early events associated with initiation and progression of PTOA, to investigate effects of microgravity in low earth orbit. We were able to adapt our CBS MPS to Redwire’s hardware; while practical facilities constraints on the ISS restricted our experiments to the use of tissues from 2 human donor, our results can be placed in context with recently published laboratory results using 25 knees from 16 donors (Dwivedi et al., 2022). However, we were able to perform both spaceflight and ground control experiments using tissues from these same 2 donors for comparison. Of particular note, both metabolomic and proteomic analyses revealed that the overall behavior of these tissues in Space was different overall than on Earth. Metabolomics showed increased oxidative stress and activation of inflammatory pathways under all treatment conditions in Space, consistent with the observed trend of higher cytokine release for all conditions in Space, and not just the disease condition (CBS + INJ) which was more pronounced on Earth. Proteomic analyses revealed increased release of many extracellular proteins caused by CBS + INJ in Space (consistent with the observed increase in GAG release), as well as marked increases in the release of pericellular collagens. Additional studies are needed to further confirm these observations, including their relationship to the effects of the physical demands and rigorous exercise regimen required of the ISS astronauts.
Data availability statement
The original contributions presented in the study are included in the article/Supplementary Material, further inquiries can be directed to the corresponding author.
Ethics statement
The studies involving humans were approved by the MIT Committee on the Use of Humans as Experimental Subjects. The studies were conducted in accordance with the local legislation and institutional requirements. The human samples used in this study were acquired from Tissues were collected from cadavers by donor banks and sent to us. Written informed consent for participation was not required from the participants or the participants’ legal guardians/next of kin in accordance with the national legislation and institutional requirements.
Author contributions
GD: Conceptualization, Data curation, Formal Analysis, Investigation, Methodology, Project administration, Writing–original draft, Writing–review and editing. LF: Data curation, Methodology, Writing–review and editing. BA: Methodology, Formal Analysis, Writing–original draft, Writing–review and editing. EF: Conceptualization, Data curation, Formal Analysis, Methodology, Writing–review and editing, Software. RB: Conceptualization, Data curation, Methodology, Writing–review and editing, Formal Analysis, Writing–original draft. JF: Conceptualization, Data curation, Investigation, Methodology, Writing–review and editing. CS: Data curation, Methodology, Project administration, Writing–review and editing, Conceptualization, Formal Analysis, Investigation. KB: Data curation, Methodology, Writing–review and editing. EL: Writing–review and editing, Data curation, Methodology. NT: Project administration, Supervision, Writing–review and editing, Resources. EB: Data curation, Writing–review and editing, Conceptualization, Formal Analysis, Methodology, Project administration, Supervision. YK: Data curation, Writing–review and editing. H-HH: Writing–review and editing, Data curation. SC: Writing–review and editing, Resources, Supervision, Investigation. ST: Writing–review and editing, Supervision. EG: Project administration, Writing–review and editing. VR: Writing–review and editing, Methodology, Resources, Supervision, Conceptualization. PO: Writing–review and editing, Data curation, Formal Analysis, Investigation, Methodology, Supervision, Conceptualization. MC: Writing–review and editing, Data curation, Formal Analysis, Investigation, Methodology, Supervision, Conceptualization, Resources. AG: Conceptualization, Data curation, Formal Analysis, Funding acquisition, Methodology, Supervision, Writing–original draft, Writing–review and editing.
Funding
The author(s) declare financial support was received for the research, authorship, and/or publication of this article. Funded by NIH-NCATS Grant UG3/UH3 TR002186 (AG); Rush Klaus Kuettner Endowed Chair (SC); United States Department of Veterans Affairs and Indiana University School of Medicine (ST).
Acknowledgments
The authors thank the donor banks and donors’ families who made this study possible: Gift of Hope Organ & Tissue Donor Network (Itasca, IL); the National Disease Research Interchange (NDRI), Philadelphia, PA; and LifeNet Health, Virginia Beach, VA. We also thank Dr. Dan Tagle of NIH-NCATS and Drs. Lucie Low and Marc Giulianotti who were then our program managers at NIH-NCATS and CASIS-ISS-NL, respectively, for their encouragement and guidance throughout the project.
Conflict of interest
Authors BA, EG, and MC were employed by the Javelin Biotech. Authors JF, CS, KB, NT, and EB were employed by the Redwire Space Technologies. YK was employed by the Corteva Agriscience.
The remaining authors declare that the research was conducted in the absence of any commercial or financial relationships that could be construed as a potential conflict of interest.
Publisher’s note
All claims expressed in this article are solely those of the authors and do not necessarily represent those of their affiliated organizations, or those of the publisher, the editors and the reviewers. Any product that may be evaluated in this article, or claim that may be made by its manufacturer, is not guaranteed or endorsed by the publisher.
Supplementary material
The Supplementary Material for this article can be found online at: https://www.frontiersin.org/articles/10.3389/frspt.2024.1358412/full#supplementary-material
References
Bajpayee, A. G., and Grodzinsky, A. J. (2017). Cartilage-targeting drug delivery: can electrostatic interactions help? Nat. Rev. Rheumatol. 13 (3), 183–193. doi:10.1038/nrrheum.2016.210
Bajpayee, A. G., Quadir, M. A., Hammond, P. T., and Grodzinsky, A. J. (2016). Charge based intra-cartilage delivery of single dose dexamethasone using Avidin nano-carriers suppresses cytokine-induced catabolism long term. Osteoarthr. Cartil. 24 (1), 71–81. doi:10.1016/j.joca.2015.07.010
Bigoni, M., Sacerdote, P., Turati, M., Franchi, S., Gandolla, M., Gaddi, D., et al. (2013). Acute and late changes in intraarticular cytokine levels following anterior cruciate ligament injury. J. Orthop. Res. 31 (2), 315–321. doi:10.1002/jor.22208
Black, R. (2022). Understanding the differential effects of Dexamethasone on the metabolism of healthy and diseased articular cartilage. Cambridge, MA, United States: Massachusetts Institute of Technology. Available at: https://dspace.mit.edu/handle/1721.1/153473
Black, R., and Grodzinsky, A. J. (2019). Dexamethasone: chondroprotective corticosteroid or catabolic killer? Eur. Cell Mater 38, 246–263. doi:10.22203/ecm.v038a17
Black, R. M., Flaman, L. L., Lindblom, K., Chubinskaya, S., Grodzinsky, A. J., and Önnerfjord, P. (2022). Tissue catabolism and donor-specific dexamethasone response in a human osteochondral model of post-traumatic osteoarthritis. Arthritis Res. Ther. 24 (1), 137. doi:10.1186/s13075-022-02828-4
Black, R. M., Wang, Y., Struglics, A., Lorenzo, P., Chubinskaya, S., Grodzinsky, A. J., et al. (2021). Proteomic clustering reveals the kinetics of disease biomarkers in bovine and human models of post-traumatic osteoarthritis. Osteoarthr. Cartil. Open 3 (4), 100191. doi:10.1016/j.ocarto.2021.100191
Black, R. M., Wang, Y., Struglics, A., Lorenzo, P., Tillgren, V., Rydén, M., et al. (2020). Proteomic analysis reveals dexamethasone rescues matrix breakdown but not anabolic dysregulation in a cartilage injury model. Osteoarthr. Cartil. Open 2 (4), 100099. doi:10.1016/j.ocarto.2020.100099
Bonassar, L. J., Grodzinsky, A. J., Srinivasan, A., Davila, S. G., and Trippel, S. B. (2000). Mechanical and physicochemical regulation of the action of insulin-like growth factor-I on articular cartilage. Archives Biochem. Biophysics 379 (1), 57–63. doi:10.1006/abbi.2000.1820
Bradley, P. X., Thomas, K. N., Kratzer, A. L., Robinson, A. C., Wittstein, J. R., DeFrate, L. E., et al. (2023). The interplay of biomechanical and biological changes following meniscus injury. Curr. Rheumatol. Rep. 25 (2), 35–46. doi:10.1007/s11926-022-01093-3
Bretaud, S., Guillon, E., Karppinen, S. M., Pihlajaniemi, T., and Ruggiero, F. (2020). Collagen XV, a multifaceted multiplexin present across tissues and species. Matrix Biol. Plus 6-7, 100023. doi:10.1016/j.mbplus.2020.100023
Brink, M., Lundquist, A., Alexeyenko, A., Lejon, K., and Rantapää-Dahlqvist, S. (2019). Protein profiling and network enrichment analysis in individuals before and after the onset of rheumatoid arthritis. Arthritis Res. Ther. 21 (1), 288. doi:10.1186/s13075-019-2066-9
Brown, T. D., Johnston, R. C., Saltzman, C. L., Marsh, J. L., and Buckwalter, J. A. (2006). Posttraumatic osteoarthritis: a first estimate of incidence, prevalence, and burden of disease. J. Orthop. Trauma 20 (10), 739–744. doi:10.1097/01.bot.0000246468.80635.ef
Chang, J. C., Christiansen, B. A., Murugesh, D. K., Sebastian, A., Hum, N. R., Collette, N. M., et al. (2018). SOST/Sclerostin improves posttraumatic osteoarthritis and inhibits MMP2/3 expression after injury. J. Bone Min. Res. 33 (6), 1105–1113. doi:10.1002/jbmr.3397
Dilley, J. E., Bello, M. A., Roman, N., McKinley, T., and Sankar, U. (2023). Post-traumatic osteoarthritis: a review of pathogenic mechanisms and novel targets for mitigation. Bone Rep. 18, 101658. doi:10.1016/j.bonr.2023.101658
D’Lima, D. D., Hashimoto, S., Chen, P. C., Lotz, M. K., and Colwell, C. W. (2001). Prevention of chondrocyte apoptosis. J. Bone Jt. Surg. Am. 83 (1), 25–26. doi:10.2106/00004623-200100021-00006
Dwivedi, G., Flaman, L., Alaybeyoglu, B., Struglics, A., Frank, E. H., Chubinskya, S., et al. (2022). Inflammatory cytokines and mechanical injury induce post-traumatic osteoarthritis-like changes in a human cartilage-bone-synovium microphysiological system. Arthritis Res. Ther. 24 (1), 198. doi:10.1186/s13075-022-02881-z
Dwivedi, G. F. L. (2019). Dexamethasone rescues chondrocyte viability and matrix degradation induced by injurious compression and exposure to inflammatory cytokines in a novel human cartilage-bone-synovium co-culture microphysiological system in 65th Orthopedic Research Society conference. Austin, TX, USA.
Eastell, R. H. R. (2007). “Biochemical markers of bone turnover,” in Treatment of the postmenopausal woman. Editor R. Lobo Third Edition (Academic Press), 337–349.
Farndale, R. W., Buttle, D. J., and Barrett, A. J. (1986). Improved quantitation and discrimination of sulphated glycosaminoglycans by use of dimethylmethylene blue. Biochim. Biophys. Acta 883 (2), 173–177. doi:10.1016/0304-4165(86)90306-5
Geiger, B. C., Wang, S., Padera, R. F., Grodzinsky, A. J., and Hammond, P. T. (2018). Cartilage-penetrating nanocarriers improve delivery and efficacy of growth factor treatment of osteoarthritis. Sci. Transl. Med. 10 (469), eaat8800. doi:10.1126/scitranslmed.aat8800
Ghadially, F. N., Lalonde, J. M., and Dick, C. E. (1978). A mechanism of formation of desmosome-like structures between synovial intimal cells. Experientia 34 (9), 1212–1213. doi:10.1007/bf01922963
Hinman, S. S., Kim, R., Wang, Y., Phillips, K. S., Attayek, P. J., and Allbritton, N. L. (2020). Microphysiological system design: simplicity is elegance. Curr. Opin. Biomed. Eng. 13, 94–102. doi:10.1016/j.cobme.2019.12.010
Huo, Y., Rangarajan, P., Ling, E. A., and Dheen, S. T. (2011). Dexamethasone inhibits the Nox-dependent ROS production via suppression of MKP-1-dependent MAPK pathways in activated microglia. BMC Neurosci. 12, 49. doi:10.1186/1471-2202-12-49
Johnson, D. L., Urban, W. P., Caborn, D. N., Vanarthos, W. J., and Carlson, C. S. (1998). Articular cartilage changes seen with magnetic resonance imaging-detected bone bruises associated with acute anterior cruciate ligament rupture. Am. J. Sports Med. 26 (3), 409–414. doi:10.1177/03635465980260031101
Jones, J. A., Hart, S. F., Baskin, D. S., Effenhauser, R., Johnson, S. L., Novas, M. A., et al. (2000). Human and behavioral factors contributing to spine-based neurological cockpit injuries in pilots of high-performance aircraft: recommendations for management and prevention. Mil. Med. 165 (1), 6–12. doi:10.1093/milmed/165.1.6
Karin, M., Andersen, R. D., Slater, E., Smith, K., and Herschman, H. R. (1980). Metallothionein mRNA induction in HeLa cells in response to zinc or dexamethasone is a primary induction response. Nature 286 (5770), 295–297. doi:10.1038/286295a0
Kerstman, E. L., Scheuring, R. A., Barnes, M. G., DeKorse, T. B., and Saile, L. G. (2012). Space adaptation back pain: a retrospective study. Aviat. Space Environ. Med. 83 (1), 2–7. doi:10.3357/asem.2876.2012
Kim, H. T., Lo, M. Y., and Pillarisetty, R. (2002). Chondrocyte apoptosis following intraarticular fracture in humans. Osteoarthr. Cartil. 10 (9), 747–749. doi:10.1053/joca.2002.0828
Krishnan, Y., Yang, Y. J., Barnes, S. K., Hung, H. K., Olsen, B. D., Hammond, P. T., et al. (2022). Predicting transport of intra-articularly injected growth factor fusion proteins into human knee joint cartilage. Acta Biomater. 153, 243–259. doi:10.1016/j.actbio.2022.09.032
Lazar, C., Gatto, L., Ferro, M., Bruley, C., and Burger, T. (2016). Accounting for the multiple natures of missing values in label-free quantitative proteomics data sets to compare imputation strategies. J. Proteome Res. 15 (4), 1116–1125. doi:10.1021/acs.jproteome.5b00981
Li, Y., Frank, E. H., Wang, Y., Chubinskaya, S., Huang, H. H., and Grodzinsky, A. J. (2013). Moderate dynamic compression inhibits pro-catabolic response of cartilage to mechanical injury, tumor necrosis factor-α and interleukin-6, but accentuates degradation above a strain threshold. Osteoarthr. Cartil. 21 (12), 1933–1941. doi:10.1016/j.joca.2013.08.021
Li, Y., Wang, Y., Chubinskaya, S., Schoeberl, B., Florine, E., Kopesky, P., et al. (2015). Effects of insulin-like growth factor-1 and dexamethasone on cytokine-challenged cartilage: relevance to post-traumatic osteoarthritis. Osteoarthr. Cartil. 23 (2), 266–274. doi:10.1016/j.joca.2014.11.006
Lin, W., Zhu, X., Gao, L., Mao, M., Gao, D., and Huang, Z. (2021). Osteomodulin positively regulates osteogenesis through interaction with BMP2. Cell Death Dis. 12 (2), 147. doi:10.1038/s41419-021-03404-5
Loeser, R. F., Shanker, G., Carlson, C. S., Gardin, J. F., Shelton, B. J., and Sonntag, W. E. (2000). Reduction in the chondrocyte response to insulin-like growth factor 1 in aging and osteoarthritis: studies in a non-human primate model of naturally occurring disease. Arthritis Rheum. 43 (9), 2110–2120. doi:10.1002/1529-0131(200009)43:9<2110::aid-anr23>3.0.co;2-u
Lohmander, L. S., Atley, L. M., Pietka, T. A., and Eyre, D. R. (2003). The release of crosslinked peptides from type II collagen into human synovial fluid is increased soon after joint injury and in osteoarthritis. Arthritis Rheum. 48 (11), 3130–3139. doi:10.1002/art.11326
Lotz, M. K., Otsuki, S., Grogan, S. P., Sah, R., Terkeltaub, R., and D'Lima, D. (2010). Cartilage cell clusters. Arthritis Rheum. 62 (8), 2206–2218. doi:10.1002/art.27528
Lu, Y. C., Evans, C. H., and Grodzinsky, A. J. (2011). Effects of short-term glucocorticoid treatment on changes in cartilage matrix degradation and chondrocyte gene expression induced by mechanical injury and inflammatory cytokines. Arthritis Res. Ther. 13 (5), R142. doi:10.1186/ar3456
McQuillan, D. J., Handley, C. J., Campbell, M. A., Bolis, S., Milway, V. E., and Herington, A. C. (1986). Stimulation of proteoglycan biosynthesis by serum and insulin-like growth factor-I in cultured bovine articular cartilage. Biochem. J. 240 (2), 423–430. doi:10.1042/bj2400423
Mina, S. G., Alaybeyoglu, B., Murphy, W. L., Thomson, J. A., Stokes, C. L., and Cirit, M. (2019). Assessment of drug-induced toxicity biomarkers in the brain microphysiological system (MPS) using targeted and untargeted molecular profiling. Front. Big Data 2, 23. doi:10.3389/fdata.2019.00023
Mootha, V. K., Lindgren, C. M., Eriksson, K. F., Subramanian, A., Sihag, S., Lehar, J., et al. (2003). PGC-1α-responsive genes involved in oxidative phosphorylation are coordinately downregulated in human diabetes. Nat. Genet. 34 (3), 267–273. doi:10.1038/ng1180
Muehleman, C., Bareither, D., Huch, K., Cole, A. A., and Kuettner, K. E. (1997). Prevalence of degenerative morphological changes in the joints of the lower extremity. Osteoarthr. Cartil. 5 (1), 23–37. doi:10.1016/s1063-4584(97)80029-5
Mueller, M. B., and Tuan, R. S. (2011). Anabolic/Catabolic balance in pathogenesis of osteoarthritis: identifying molecular targets. Pm R. 3 (6), S3–S11. doi:10.1016/j.pmrj.2011.05.009
Murray, M. M., Zurakowski, D., and Vrahas, M. S. (2004). The death of articular chondrocytes after intra-articular fracture in humans. J. Trauma 56 (1), 128–131. doi:10.1097/01.ta.0000051934.96670.37
NASA (1999). Injury rate of shuttle astronauts - longitudnal study of astronaut health. Longitudnal study Astronaut. Heal. Newsl. 8, 1.
Oo, W. M., and Hunter, D. J. (2022). Repurposed and investigational disease-modifying drugs in osteoarthritis (DMOADs). Ther. Adv. Musculoskelet. Dis. 14, 1759720X2210902. doi:10.1177/1759720x221090297
Pufe, T., Petersen, W. J., Miosge, N., Goldring, M. B., Mentlein, R., Varoga, D. J., et al. (2004). Endostatin/collagen XVIII--an inhibitor of angiogenesis--is expressed in cartilage and fibrocartilage. Matrix Biol. 23 (5), 267–276. doi:10.1016/j.matbio.2004.06.003
Ramachandran, V., Dalal, S., Scheuring, R., and Jones, J. (2018). Musculoskeletal injuries in astronauts: review of pre-flight, in-flight, post-flight, and extravehicular activity injuries. Curr. Pathobiol. Rep. 6, 149–158. doi:10.1007/s40139-018-0172-z
Richardson, D. W., and Dodge, G. R. (2003). Dose-dependent effects of corticosteroids on the expression of matrix-related genes in normal and cytokine-treated articular chondrocytes. Inflamm. Res. 52 (1), 39–49. doi:10.1007/s000110300012
Ritchie, M. E., Phipson, B., Wu, D., Hu, Y., Law, C. W., Shi, W., et al. (2015). Limma powers differential expression analyses for RNA-sequencing and microarray studies. Nucleic Acids Res. 43 (7), e47. doi:10.1093/nar/gkv007
Robling, A. G., Niziolek, P. J., Baldridge, L. A., Condon, K. W., Allen, M. R., Alam, I., et al. (2008). Mechanical stimulation of bone in vivo reduces osteocyte expression of Sost/sclerostin. J. Biol. Chem. 283 (9), 5866–5875. doi:10.1074/jbc.m705092200
Roth, G. S., and Adelman, R. C. (1975). Age related changes in hormone binding by target cells and tissues; possible role in altered adaptive responsiveness. Exp. Gerontol. 10 (1), 1–11. doi:10.1016/0531-5565(75)90009-1
Ruttkay-Nedecky, B., Nejdl, L., Gumulec, J., Zitka, O., Masarik, M., Eckschlager, T., et al. (2013). The role of metallothionein in oxidative stress. Int. J. Mol. Sci. 14 (3), 6044–6066. doi:10.3390/ijms14036044
Rydén, M., Turkiewicz, A., Önnerfjord, P., Tjörnstrand, J., Englund, M., and Ali, N. (2023). Identification and quantification of degradome components in human synovial fluid reveals an increased proteolytic activity in knee osteoarthritis patients vs controls. Proteomics 23 (15), e2300040. doi:10.1002/pmic.202300040
Sanchez, C., Deberg, M. A., Bellahcène, A., Castronovo, V., Msika, P., Delcour, J. P., et al. (2008). Phenotypic characterization of osteoblasts from the sclerotic zones of osteoarthritic subchondral bone. Arthritis Rheum. 58 (2), 442–455. doi:10.1002/art.23159
Scheuring, R. A. (2010). “Musculoskeletal changes, injuries and rehabilitation associated with Spaceflight,” in American Osteopathic Academy of Sports Medicine.
Scheuring, R. A., Mathers, C. H., Jones, J. A., and Wear, M. L. (2009). Musculoskeletal injuries and minor trauma in space: incidence and injury mechanisms in U.S. astronauts. Aviat. Space Environ. Med. 80 (2), 117–124. doi:10.3357/asem.2270.2009
Sierra, H., Cordova, M., Chen, C. J., and Rajadhyaksha, M. (2015). Confocal imaging-guided laser ablation of basal cell carcinomas: an ex vivo study. J. Invest. Dermatol 135 (2), 612–615. doi:10.1038/jid.2014.371
Smeriglio, P., Dhulipala, L., Lai, J. H., Goodman, S. B., Dragoo, J. L., Smith, R. L., et al. (2015). Collagen VI enhances cartilage tissue generation by stimulating chondrocyte proliferation. Tissue Eng. Part A 21 (3-4), 840–849. doi:10.1089/ten.tea.2014.0375
Strauss, S., Krog, R. L., and Feiveson, A. H. (2005). Extravehicular mobility unit training and astronaut injuries. Aviat. Space Environ. Med. 76 (5), 469–474.
Struglics, A., Larsson, S., Kumahashi, N., Frobell, R., and Lohmander, L. S. (2015). Changes in cytokines and aggrecan ARGS neoepitope in synovial fluid and serum and in C-terminal crosslinking telopeptide of type II collagen and N-terminal crosslinking telopeptide of type I collagen in urine over five years after anterior cruciate ligament rupture: an exploratory analysis in the knee anterior cruciate ligament, nonsurgical versus surgical treatment trial. Arthritis Rheumatol. 67 (7), 1816–1825. doi:10.1002/art.39146
Subramanian, A., Tamayo, P., Mootha, V. K., Mukherjee, S., Ebert, B. L., Gillette, M. A., et al. (2005). Gene set enrichment analysis: a knowledge-based approach for interpreting genome-wide expression profiles. Proc. Natl. Acad. Sci. U. S. A. 102 (43), 15545–15550. doi:10.1073/pnas.0506580102
Swärd, P., Frobell, R., Englund, M., Roos, H., and Struglics, A. (2012). Cartilage and bone markers and inflammatory cytokines are increased in synovial fluid in the acute phase of knee injury (hemarthrosis)--a cross-sectional analysis. Osteoarthr. Cartil. 20 (11), 1302–1308. doi:10.1016/j.joca.2012.07.021
Szklarczyk, D., Gable, A. L., Lyon, D., Junge, A., Wyder, S., Huerta-Cepas, J., et al. (2019). STRING v11: protein-protein association networks with increased coverage, supporting functional discovery in genome-wide experimental datasets. Nucleic Acids Res. 47 (D1), D607–d613. doi:10.1093/nar/gky1131
Tanaka, N., Tsuno, H., Ohashi, S., Iwasawa, M., Furukawa, H., Kato, T., et al. (2021). The attenuation of insulin-like growth factor signaling may be responsible for relative reduction in matrix synthesis in degenerated areas of osteoarthritic cartilage. BMC Musculoskelet. Disord. 22 (1), 231. doi:10.1186/s12891-021-04096-w
Thudium, C. S., Engstrøm, A., Bay-Jensen, A.-C., Frederiksen, P., Jansen, N., De Zwart, A., et al. (2023). Cartilage tissue turnover increases with high-compared to low-intensity resistance training in patients with knee OA. Arthritis Res. Ther. 25 (1), 22. doi:10.1186/s13075-023-03000-2
Tyler, J. A. (1989). Insulin-like growth factor 1 can decrease degradation and promote synthesis of proteoglycan in cartilage exposed to cytokines. Biochem. J. 260 (2), 543–548. doi:10.1042/bj2600543
Viegas, S. F., Williams, D., Jones, J., Strauss, S., and Clark, J. (2004). Physical demands and injuries to the upper extremity associated with the space program. J. Hand Surg. Am. 29 (3), 359–366. doi:10.1016/j.jhsa.2004.01.015
Web.Archive (2003). A brief history of Space accidents. Available at: https://web.archive.org/web/20030204073904/.
Wieland, H. A., Michaelis, M., Kirschbaum, B. J., and Rudolphi, K. A. (2005). Osteoarthritis - an untreatable disease? Nat. Rev. Drug Discov. 4 (4), 331–344. doi:10.1038/nrd1693
Keywords: post-traumatic osteoarthritis, ISS-NL, osteochondral-synovium coculture in space, dexamethasone, IGF-1, proteomic/metabolomic studies of PTOA
Citation: Dwivedi G, Flaman L, Alaybeyoglu B, Frank EH, Black RM, Fite J, Scherzer C, Barton K, Luyster E, Thomas N, Boland E, Krishnan Y, Hung H-H, Chubinskaya S, Trippel SB, Geishecker E, Rosen V, Önnerfjord P, Cirit M and Grodzinsky AJ (2024) Effects of dexamethasone and IGF-1 on post-traumatic osteoarthritis-like catabolic changes in a human cartilage-bone-synovium microphysiological system in space and ground control tissues on earth. Front. Space Technol. 5:1358412. doi: 10.3389/frspt.2024.1358412
Received: 19 December 2023; Accepted: 20 February 2024;
Published: 14 March 2024.
Edited by:
Lorenzo Moroni, Maastricht University, NetherlandsReviewed by:
Yupeng Chen, University of Connecticut, United StatesGuohui Zhong, China Astronaut Research and Training Center, China
Copyright © 2024 Dwivedi, Flaman, Alaybeyoglu, Frank, Black, Fite, Scherzer, Barton, Luyster, Thomas, Boland, Krishnan, Hung, Chubinskaya, Trippel, Geishecker, Rosen, Önnerfjord, Cirit and Grodzinsky. This is an open-access article distributed under the terms of the Creative Commons Attribution License (CC BY). The use, distribution or reproduction in other forums is permitted, provided the original author(s) and the copyright owner(s) are credited and that the original publication in this journal is cited, in accordance with accepted academic practice. No use, distribution or reproduction is permitted which does not comply with these terms.
*Correspondence: Alan J. Grodzinsky, alg@mit.edu