- Department of Physiology and Pathophysiology, Petrozavodsk State University, Petrozavodsk, Russia
The existing literature suggests that temperature and gravity may have much in common as regulators of physiological functions. Cold, according to the existing literature, shares with gravity common effects on the neuromuscular system, while heat produces effects similar to those of microgravity. In addition, there are studies evidencing unidirectional modification of the motor system to heat and hypoxia. Such agonistic relationship in a triad of “microgravity, heat, and hypoxia” and in a pair of “cold and gravity” in their effect on the neuromuscular system may have evolutionary origins. To address this problem, ten years ago, we came up with a concept with the working name Baby Astronaut hypothesis, which posed that “Synergetic adaptation of the motor system to different environments comes from their ontogenetic synchronicity.” More specifically, the synchronicity of microgravity (actually, the “wet immersion” model of microgravity), higher temperature, and hypoxia are the characteristics of the intrauterine environment of the fetus. After childbirth, this group of factors is rapidly replaced by the “extrauterine,” routine environment characterized by Earth gravity (1 G), normoxia, and lower ambient temperature. The physiological effect of cold and gravity on the motor system may well be additive (synergistic). We earlier estimated a “gravity-substitution” potential of cold-induced activity and adaptation to cold as 15–20% of G, which needs further validation and correction. In this study, we sought to critically analyze the interaction of temperature and gravity, based on the concept of Baby Astronaut, using data from the new academic literature. We have come to the conclusion that the concept of Baby Astronaut can be regarded as valid only for species such as a rat (immature, altricial species), but not for a human fetus. Several confirmatory experiments were suggested to verify (or falsify) the concept, which would allow us to consider it as empirical. In addition, the interaction of temperature and gravity may be of practical interest in the fields of neurorehabilitation and habilitation in childhood for constructing a physical environment, which would help strengthen or weaken muscle tone in specific muscles.
1 Introduction
Like gravity, temperature is omnipresent and eternal. In a similar way as gravity works, temperature, as a physical factor, accompanies living beings, thereby forming the thermal conditions of the environment—either colder, warmer, or thermoneutral. The origin and evolution of life on Earth were controlled by temperature (Islas, 2014), and this statement is fully applicable to gravity (Morey-Holton, 2003; Goswami, 2017). This suggests that temperature and gravity may have much in common as regulators of physiological functions.
An idea on the similarity of the reactions of the motor system of homeothermic species (mammals and birds) to the cold and to gravitational postural stimuli looks plausible and is regularly discussed (Lupandin Iu., 1980; Meigal and Lupandin, 2005; Lømo et al., 2020). Still, the commonality between cold-induced (thermoregulatory) and gravity-induced (antigravity postural) activity of the motor system is not yet clearly understood and evidenced (Lømo et al., 2020). On the other hand, such a modality of temperature as heating exerts effects on the organism similar to the effect of microgravity (for a review, see Meigal, 2012; Meigal, 2013). Altogether, this may be indicative of co-evolution of physiological reactions on temperature and gravity. Accordingly, temperature and gravity may be equivalent in terms of their physiological action on the homeothermic organism.
To explore mechanisms of probable physiologic commonality between gravity and cold, as well as between microgravity and heat, about 10 years ago, we came up with a concept with the provisional title “Baby Astronaut.” This concept suggested that the similarity of physiological effects of temperature (cold/heat) and gravity (Earth gravity/microgravity) modalities may stem from their temporary coupling (synchronization) in ontogenesis (Meigal, 2012, 2013). More specifically, the synchronization of heat and microgravity may have taken place in utero during the antenatal period. After birth, in the postnatal period, a cooler environment and normal gravity should be synchronized. In general, the concept of the Baby Astronaut assumes the following statements:
1. Muscle tone caused by cold and gravity is strikingly similar, which may reflect the commonality, not to say identity, of their physiological mechanisms.
2. Heat and microgravity exert similar effects on the neuromuscular system and motor activity. These effects are the opposite of those provoked on the neuromuscular system by cold and gravity.
3. Hypoxia, small body size, hypokinesia, and the postnatal period of development all exert a parallel microgravity effect on the neuromuscular system.
4. “Synergetic adaptations of the motor system to different environments come from their ontogenetic synchronicity” (Meigal, 2013).
5. Several specific consequences (predictions) of the effects of microgravity on various physiological parameters can be drawn from the concept of Baby Astronaut.
6. Some practical considerations were formulated on how to apply cold in prophylactic countermeasures in space.
The interaction of temperature and gravity and, in a broader sense, the concept of Baby Astronaut, may be of practical interest in the fields of neurorehabilitation and habilitation in childhood for constructing a physical environment, which would help strengthen or weaken muscle tone in specific muscles. In addition, despite varied exercise countermeasures, neuromuscular deconditioning still takes place during long-duration spaceflight (Comfort et al., 2021). Our earlier prudent quotes attributed the cold to the ability to surrogate for as much as 20% of gravity loss (Meigal, 2013). Therefore, we suggested that ambient temperature, namely the cold, can serve as a basis for novel methods to prevent deconditioning of the human motor system in long-duration space missions.
Over the past 10 years, many studies have been conducted in the field of comparing of separate and combined physiological effects of various environmental physical factors, their cross-tolerance and cross-adaptation. In addition, several valuable studies, for example by Stanojevic et al. (2011), Stanojevic et al. (2012), and by Sekulić et al. (2005), were earlier overlooked by us and, therefore, not used in the original version of the concept of the Baby Astronaut. In the present review, we considered the ideas of these studies. Also, we were unaware of one earlier study devoted to a similar idea (Wood, 1970), and we give full credit to this study.
Thus, the purpose of this study was dual—first, to critically review the entire idea of similarity or identity of physiological reactions to temperature and gravity and, second, to seek more evidence from the extant academic literature that can refute or validate the concept of temporal synchronicity of gravity and temperature (the concept of Baby Astronaut) as a possible mechanism of similarity of their effect on the organism. In addition, we proposed possible confirmatory experiments for this concept.
2 Methods
The study was conducted in accordance with the criteria of a theoretical review supplemented by elements of a critical review (Paré et al., 2015). As a theoretical review, the study was aimed at “explanation building” (Paré et al., 2015) and “theory evaluation” (Baumeister and Leary, 1997). More specifically, we aimed at the explanation of the supposed similarity/identity of the physiological mechanisms of the effect of gravity and temperature with the help of extant literature sources. The nature of the primary sources was conceptual and empirical, and the search strategy was comprehensive (Paré et al., 2015). In addition, all theoretical statements were critically evaluated. Altogether, the type and, correspondingly, methodology of this study fit best corresponding to the hybrid type of the review (Paré et al., 2015).
3 Results
3.1 Statement 1: Muscle tone caused by cold and gravity is strikingly similar, which may reflect the commonality, not to say identity, of their physiological mechanisms
Muscle tone (MT) is appreciated as one of the critical phenomena in physiology and pathophysiology of motion and in neurology. MT has varied mechanisms, definitions, and components. For example, it can be defined as a “fluid state of readiness to movement” (Bernstein, 1947), a state of “prolonged weak muscle contraction,” “tension in the relaxed muscle,” and the phenomenon of “resistance to stretching” (Ward, 2000; Needle et al., 2014; Masi and Hannon, 2008; Ganguly et al., 2021). MT is evaluated using various physiological and neurologic techniques. In addition, MT has the visco-elastic, non-reflex component, evaluated using “digital palpation” (Masi and Hannon, 2008; Ganguly et al., 2021; Amirova et al., 2021). Finally, MT can be elicited by heterogeneous physical stimuli and maneuvers, for example, gravity, cold, vibration, and postcontraction. Gravity induces the antigravity (postural) MT, which allows actively holding a posture on Earth at 1 G, while cold provokes specific heat-productive muscle activity, which comprises “cold shivering” and the “pre-shivering” or “thermoregulatory” muscle tone (Göpfert and Stuffer, 1952; Golenhofen, 1963; IUPS Thermal Commission, 1987; Lømo et al., 2020).
Several lines of evidence can be identified for the probable interrelationship and interaction between the gravity-induced (postural, antigravity) and cold-induced (thermoregulatory) MT.
3.1.1 Gravity- and cold-induced muscle tone are mediated by the same motor units
Indeed, on the level of MUs, thermoregulatory MT is produced by the asynchronous activity of low-threshold, presumably slow-type motor units (MU), either in humans (Petajan and Williams, 1972; Meigal, 2002) or animal species (Lupandin, 1983). Just the same pattern of MU activity, that is, asynchronous activity of low-threshold MUs, is the characteristic of MT during weak isometric muscle contraction (Freund, 1983). By eye, the patterns of low-amplitude stationary interference electromyogram (EMG) during thermoregulatory and postural MT are practically indistinguishable. In animal species, gamma motoneurons and muscle afferents were shown to be active during thermoregulatory MT just like they were during postural MT (Lupandin, 1979). These findings allowed Lupandin (1983) to formulate the concept of hypothetical “thermoregulatory-postural” tone, which served as one of the starting points for the concept of Baby Astronaut.
Still, this issue can be criticized. No direct evidence has been found from the literature that the same MU is recruited both in postural and thermoregulatory MT. As it was stated by Lømo et al. (2020), “ ... it is difficult to distinguish between tonic activity in the service of either posture or body temperature regulation ....”
The experimentum crucis for the decisive determination of whether the aforementioned “thermoregulatory-postural” tone actually exists or not, would be to evidence, by means of EMG, activity of one and the same MU recruited, sequentially, into postural and then into thermoregulatory MT, or in the opposite order. To our knowledge, this has not been performed yet, although it seems technically feasible, especially in the man.
3.1.2 Thermoregulatory muscle tone is modifiable by postural stimuli, and postural tone—by thermal stimuli
In support of that, in laboratory animals, thermoregulatory MT was experimentally modified by the vestibular stimuli (Lupandin and Kuz'mina, 1985). More specifically, in cats, thermoregulatory MT increases in flexor muscles after destruction of the labyrinths, but it decreases during caloric or electrical stimulation of the labyrinths (Kuz'mina, 1980). In humans, thermoregulatory MT is modifiable by the position of the head (Meigal et al., 1996a). In addition, such a specific type of MT as the “after-contraction effect” (the Kohnstamm phenomenon), in human biceps brachii muscle was found to be enhanced under cold ambient conditions (Meigal et al., 1996b).
3.1.3 Thermoregulatory muscle tone is characterized by specific “postural topography” in skeletal musculature
To illustrate, in anaesthetized cats, cold-induced muscle activity emerges strictly in the flexor musculature (Lupandin Iu., 1980; Lupandin, 1983), which may be indicative of a heat loss lessening (or “embryonic”) posture.
Several critical comments could be suggested for this issue. First of all, a retrospective analysis of the study by Lupandin Iu., 1980 allowed us identifying the cold-induced muscle activity as cold shivering due to explicit mechanical tremor. Cold shivering cannot be considered as a kind of MT, since thermoregulatory MT and cold shivering have distinctly different neuronal and metabolic mechanisms (Meigal, 2002; Haman et al., 2004; Lømo et al., 2020). Moreover, recently, Lømo et al. (2020), in contrast to Lupandin (1983), showed that in small animals, tonic activity is limited to deeper rather than superficial muscles. In addition, thermoregulatory MT does not cause a real, even tiny, change in the position of the limbs (Lømo et al., 2020).
Then, in humans, the “flexion” principle of the topography of thermoregulatory MT or cold shivering has not yet been evidenced. Instead, cold shivering in humans is the characteristic of “proximal” musculature, that is, the muscles of the upper girdle, abdomen, chest, and thighs (Golenhofen, 1963; Israel and Pozos, 1989; Meigal et al., 1998). This suggests that the “topography” of cold shivering is determined by the principle of a shorter, that is, “heat-saving,” rout from shivering muscles to internal organs and the brain (Xu et al., 2005). As for the thermoregulatory MT itself, Golenhofen (1963) and Göpfert and Stuffer (1952) suggested that it is the most distinctive characteristic of distal muscles of the limbs.
Finally, in order to determine decisively whether thermoregulatory MT in humans or animal species has a specific “flexor topography” or not, it would be reasonable to conduct such a study in the weightlessness, where gravity does not interfere with thermoregulatory MT, and therefore even weak thermoregulatory (cold-induced) MT would manifest itself as true postural activity.
3.1.4 Thermoregulatory muscle tone and weak voluntary isometric contraction can be simultaneously present in the same skeletal muscle
In our earlier study, in low-intensity isometric contraction (at 10% of a maximal voluntary contraction), the surface EMG in biceps brachii muscle demonstrated 20% greater amplitude in cold compared to the thermoneutral condition, thereby prompting the “co-existence” of weak voluntary postural and involuntary thermoregulatory MT in muscles (Meigal et al., 1998). With an increase in the strength of contraction, such additive cold-induced muscle activity gradually decreased and eventually disappeared at 80% of a maximal voluntary contraction, which probably indicated a competing relationship between postural and thermoregulatory MT (Meigal et al., 2003). Recently, a similar result was presented by Renberg et al. (2020) and Mallette et al. (2021). According to Sherrington’s “common final pathway”, during weak muscle contractions, thermoregulatory MT should be “competing” for a share of the motoneuronal pool, but with stronger efforts, it can be inhibited by a “dominant” stronger voluntary muscle contraction.
Still, this issue, to some extent, looks controversial. In a cooling environment, it may well be so that voluntary muscle contraction is enhanced by a feeling of cold, that is, behaviorally conditioned. Therefore, the increased amplitude of surface EMG does not necessarily indicate the “co-existence” of cold-induced MT and weak voluntary isometric muscle contraction. In addition, muscle contraction is heat-productive, which makes it difficult to distinguish between the “pure” thermoregulatory and postural activity of skeletal muscles.
In addition, a mere “additive” relationship between postural and thermoregulatory MT does not necessarily always take place. For example, an increase in such kind of MT as the Kohnstamm phenomenon in the cold was the characteristic of biceps brachii (Meigal et al., 1996b), but not of deltoid muscles (Meigal and Pis’mennyi, 2009). Presumably, this was due to the confounding effect of antigravity function of the deltoid muscle, namely, the arm abduction, which may contribute to heat loss by increasing the area of the skin exposed to cooling. This probably led to behavioral suppression of the Kohnstamm phenomenon in the deltoid muscle.
The experimentum crucis to decisively determine whether the ambient temperature actually enhances the Kohnstamm phenomenon would be to conduct such a study in zero G, when gravity does not affect the distribution of MT among muscles. This has not yet been performed, although technically feasible during spaceflight.
3.1.5 Motor unit firing rate is modifiable by the ambient temperature and acclimation
In humans, the firing rate of voluntarily recruited MUs decreased by 20% after 30 min exposure to 10°C (Meigal et al., 1997). In rats, after 2 weeks of acclimation to the cold, the firing rate of MUs was decreased by 30% at the same ambient temperature (Sorokina et al., 1984). Similarly, the maximum firing rate of MUs of “winter pigeons,” that is, studied in the winter season, was lower by 20% than that of “summer” pigeons (Sorokina and Medvedev, 1986). A decrease in the MU firing rate would probably transfer the muscle contraction from fused to unfused tetanus, which is known as the most heat-producing regime of contraction (Gurfinkel et al., 1981). This statement is indirectly supported by an increase in the firing rate of MU in humans under heat stress (Meigal et al., 1999), and in rats—after acclimation to heat (Kuokkanen, 1989).
This issue can be also criticized. The phenomenon of decreasing the MU firing rate in humans in the cold has been so far demonstrated in phasic and not in tonic contractions (Meigal et al., 1997). Also, the firing rate of MU could reduce due to direct cooling of the skeletal muscle. However, the study by Mallette et al. (2018) showed that it is not the case.
Lømo et al. (2020) have recently shown that the firing rate of the tonic MUs in rat soleus muscle increases linearly with a decrease in ambient temperature, which is in contrast to our result (Meigal et al., 1997). Such a discrepancy may occur due to differences in the motor task (phasic vs. tonic activity of MUs).
3.1.6 Thermoregulatory and postural muscle tone are similar by fuel selection
Cold shivering corresponds to about 50% of maximal voluntary contraction (Haman, 2006; Renberg et al., 2020), while thermoregulatory MT—only to 5% (Haman et al., 2004), which certainly corresponds to a weak isometric muscle contraction. Bursts of cold shivering co-vary with an increase in carbohydrate oxidation, which, in turn, is associated with the recruitment of type II muscle fibers or faster MUs (Haman et al., 2004; Haman et al., 2010). On the contrary, thermoregulatory MT is specifically associated with lipid oxidation (Vallerand and Jacobs, 1990; Haman, 2006), which applies to low-intensity exercise (Romijn et al., 1993) and resting conditions (van Loon et al., 2001). In addition, similarity between thermoregulatory and postural MT is indirectly supported by the fact that slow running, which requires recruitment of slow muscle fibers, is fueled by fat, while fast running by carbohydrates (Roberts et al., 1996).
Nonetheless, there is no direct evidence that thermoregulatory MT is analogous to postural MT by fuel selection. Cold shivering and exercise are not exact analogs by fuel selection (Tipton et al., 1997; Haman et al., 2004). In addition, fuel selection during cold shivering and thermoregulatory MT is highly individual (Haman et al., 2004).
3.1.7 Cold drives the shift of muscle fiber characteristics in the direction of slower (type I) fibers (“fast-to-slow” modeling)
Several studies support the fact that cold can really cause such a shift (Suzuki et al., 1997; Hirabayashi et al., 2005). Nomura et al. (2002), Lefaucheur et al. (2001), and Herpin et al. (2002) demonstrated that the maturation of type I muscle fibers accelerates under cold conditions. In their recent study, Lømo et al. (2020:19) stated that “In the cold, muscles undergo a certain form of training, as they become redder, acquire more slowly contracting fibers and capillaries around the fibers ...”
3.1.8 Conclusion on statement 1
In sum, our own experimental studies and existing literature suggest that, in terms of a physical factor, temperature does indeed have much in common with gravity, since both cold and gravity 1) induce MT, which is 2) similar by the pattern of activity and type of MUs, and by 3) fuel selection, 4) they interact with each other, and 5) probably “co-exist” in the same motoneuronal pool. In addition, it is likely that the cold decreases MU firing activity and modifies muscle fiber type composition in the direction of slower type. Therefore, cold, similar to gravity, can be considered as a “pro-tonogenic” and, therefore, a “pro-gravity” factor. As such, cold and gravity exert a unidirectional ( convergent or agonistic) effect on the motor system. Nevertheless, by now, most of the evidence of the agonistic relationship between cold and gravity looks as only indirect, though strong. Indirectly, this conclusion is supported by the fact that under extreme modality of gravity (hypergravity), slow muscles shift their features in the direction of even slower ones (slow-to-slower shift) (Bozzo et al., 2004).
Thermoregulatory MT does not produce mechanical postural work, which makes it difficult to compare it with postural MT. In a sense, thermoregulatory MT manifests itself as a “posture without posture”, while cold shivering as a “movement without movement” (Meigal and Lupandin, 2005). It is likely that the postural potential of thermoregulatory MT can be visualized only when gravity does not interfere. Thus, the real “cold-controlled” topography of thermoregulatory MT and cold shivering can probably be observed in orbit, where thermoregulation-related contractions of skeletal muscles would become detectable. Similarly, the effect of cold can be observed on such kind of MT, as the Kohnstamm phenomenon can be reliably tested only under real space conditions. As for various ground-based models of microgravity, such as “dry” immersion or vertical unloading, they are unreliable for testing the interaction of cold and gravity, since gravity is still present in these models.
3.2 Statement 2: Heat and microgravity exert similar effects on the neuromuscular system and motor activity. These effects are the opposite of those caused by cold and gravity
3.2.1 Muscle tone is reduced in both microgravity and heating stress
Under microgravity conditions, MT indeed noticeably weakens, either due to a specific decrease in afferent flow from the receptors of the sole skin (the concept of “support unloading”) (Tomilovskaya et al., 2019; Amirova et al., 2021), or a general decrease in afferent flow from proprioceptors and the vestibular apparatus (the concept of “deafferentation”) (Demangel et al., 2017). As for the heating conditions, from the existing literature, we did not find direct evidence of MT reduction under heat stress or passive hyperthermia. On the other hand, during active, exercise-induced hyperthermia, human muscle activity is inhibited due to the “central fatigue” mechanism, decreased motor drive to muscles, and, as a consequence, weaker recruitment of muscles to sustained isometric contraction (Tucker et al., 2004; Todd et al., 2005; Périard et al., 2019). Recently, this was supported in the study by Ball (2021) who stated that neuromuscular performance under an elevated core temperature is attenuated due to a reduced voluntary drive in motor unit recruitment or/and a failure in muscle afferent feedback.
That conclusion has much in common with the concept of deafferentation during microgravity. The “central fatigue” is likely induced by the brainstem serotoninergic mechanism (Nybo, 2008), although other neurotransmitter circuits may also be involved. In addition, such involuntarily induced MT as the Kohnstamm phenomenon in human biceps brachii muscle is clearly weaker in heat than that under thermoneutral conditions (Meigal et al., 1996b). From a thermoregulatory point of view, the loss (or attenuation) of MT under heat stress has obvious advantages: 1) it provides a decrease in heat production, which prevents further increase in the body temperature, and 2) it allows blood to be pumped to the skin for heat removal, rather than to skeletal muscles (Nybo, 2008; Ball, 2021).
Still, there are two drawbacks in this statement. First, direct studies on the evaluation of MT in either passive or active hyperthermia, or at moderate warming, are still missing. Masiero et al. (2019) reported that in subjects with parkinsonism, short-term immersion in water with a temperature up to 40°C caused the so-called warm water effect in the form of mental and muscular relaxation. However, the MT relaxation effect in this study can be equally attributed to the conditions of heat and immersion. Second, in experiments with modeled microgravity, MT is usually measured using the method of “digital palpation” (myotonometry) (Amirova et al., 2021), which means an assessment of the peripheral passive, or visco-elastic, aspect of MT. On the contrary, in heat stress, muscle activity is studied using electrophysiological methods, which means an assessment of its central neurophysiologic aspect. This makes it impossible to reliably compare MT between these two conditions. In our studies, we encountered the problem of poor compatibility between neurologic (clinimetric) and neurophysiologic methods of assessing MT in a form of muscle rigidity in individuals with Parkinson's disease under the condition of microgravity modeled with “dry” immersion (Miroshnichenko et al., 2018).
3.2.2 Motor units increase their firing rate under microgravity and environmental heating
Todd et al. (2005) hypothesized that exercise under heating conditions would require higher MU rates to allow for the fusion of contracting fast muscle fibers. In humans, hot thermal conditions indeed provoked MU firing at higher rates (Meigal et al., 1999). Moreover, heat stress led to the appearance of such peculiar patterns of MU discharges as “doublets” (Meigal et al., 1999). An increase in the firing rate of MUs, along with the appearance of “doublets”, can be considered as a relevant neuromuscular adjustment which contributes to the rapid increase in the strength of muscle contraction (Mrówczyński et al., 2015) without an increase of heat production in skeletal muscles (Gurfinkel et al., 1981). In rats, after two-week acclimation to heat, the firing rate of MU also increased (Kuokkanen, 1989). Similarly, in the models of microgravity, MUs modified their activity in direction of shorter interspike intervals (bigger firing rate), for example, after 30 days of bed rest (Kozlovskaya and Kirenskaya, 2004).
However, the type of MUs in the studies by Kuokkanen (1989), Kozlovskaya and Kirenskaya (2004), and Meigal et al. (1999) was not verified. In addition, the firing rate and pattern of a MU cannot be regarded as a reliable criterion for determining its type. Therefore, we consider the statement 2 as insufficiently substantiated, although plausible.
3.2.3 Both under heating conditions and microgravity, muscle fibers undergo “slow-to-fast” modeling
A plenty of studies have demonstrated clear “slow-to-fast” modeling of muscle fibers either in ground-based models of microgravity or in real spaceflight (Roy et al., 1996; Ishihara et al., 1997; Shenkman et al., 1997; Trappe et al., 2004; Mulder et al., 2008). For the hot environment, Ball (2021) has stated that higher muscle temperatures resemble a shift in contractile characteristics to a faster phenotype.
As for heat acclimation, in the existing literature, we did not find direct evidence of “slow-to-fast” muscle fiber modification, though some studies indirectly point to a shift in muscle fibers toward a faster type during heat stress. For example, in the study by O'Neill et al. (2006), the phenotypic transformation of muscle fibers toward a slower type, that is, “fast-to-slow” modeling, in the overloaded muscle was effectively suppressed by administration of the T3 hormone which maintained the increasing body temperature. Similarly, according to the study by Baldwin and Haddad, (2001), either hyperthyroidism or unloading (limb suspension) induces muscle transformation to a faster phenotype. In contrast, combined hypothyroidism and muscle overloading presented synergistic, that is, more than additive, effect in a form of fast-to-slow modeling of muscle fibers (Baldwin and Haddad, 2001).
Despite convincing, although indirect evidence, there are still no studies demonstrating the direct modeling effect of heat on muscle fiber composition. In addition, the signaling pathway of T3 action on muscle fiber gene expression differs from that of temperature stressor (Baldwin and Haddad, 2001).
3.2.4 Conclusion on statement 2
Although cold and gravity are highly likely unidirectional (agonistic, parallel, convergent) in their action on the motor system, one cannot just as confidently assert that with respect to the pair “heat-microgravity.” Still, as cold and heat are clearly opposite (antagonistic, divergent) in their action on the motor system and muscle tone, while microgravity—with normal gravity, the overall interaction between these four physical conditions is schematized in Figure 1. In that scheme, cold and gravity are shown as pro-tonogenic factors (favoring muscle tone), while heat and microgravity as the contra-tonogenic ones.
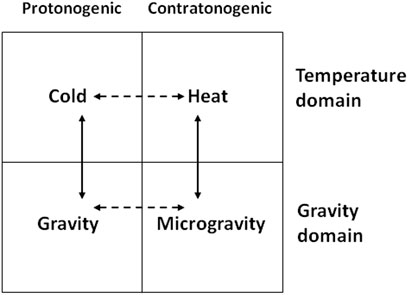
FIGURE 1. Interaction of cold, heat, gravity, and microgravity. Solid lines: the agonistic (convergent) effect; dashed lines: the antagonistic (divergent) effect.
3.3 Statement 3: Hypoxia, body size, hypokinesia, and the postnatal period of development exert parallel effects with the microgravity effect of neuromuscular activity and muscle fiber morphology
The aforementioned schematization of agonistic/antagonistic relations between gravity and temperature with respect to MT can be complemented by other physical factors, which act in utero or right after birth. Among them, hypoxia, body size, inactivity, and also the period of postnatal development look the most deserving of attention.
3.3.1 Hypoxia
In physiology, hypoxia is considered as one of the most potent and studied physical factors, and it does exert the modeling effect on skeletal muscles. For example, in hypoxia-adapted rats, the percentage of fast-type muscle fibers in such typically “slow” muscle as soleus is significantly increased (Ishihara et al., 2000), which is convergent with the effect of microgravity.
Similarly, in patients with chronic obstructive pulmonary disease (COPD), which is characterized by chronic hypoxemia, there is an increase in the proportion of type II fibers (faster, less oxidative) in skeletal muscles and, accordingly, a decrease in the proportion of type I fibers (slower, more oxidative) (Rabinovitch and Vilaro, 2010; Debevec et al., 2018). In addition, the microgravity-like effect of hypoxia is indirectly confirmed by the fact that osteoporosis, characteristic of spaceflight, is more common in patients with COPD than in those without COPD (Graat-Verboom et al., 2009).
Thus, the modeling effect of hypoxia on the neuromuscular system as a whole should be the opposite of the effect of cold and gravity. Indeed, a study by Sorokina et al. (1984) showed that acclimation to cold and hypoxia provokes strictly opposite effects on MU activity. More specifically, in rats under barometric hypoxia (3 h in a barometric chamber, daily, 6 times a week, 7,000 m above sea level, 5 weeks), the mean firing rate of MU was 18–25 imp per sec, while in control rats (at sea level in the thermoneutral conditions), it was 14–18 imp per sec, and for those who acclimated to cold (5 weeks of exposure to air at Ta < 15°C), it was 10–12 imp per sec. In addition, the antagonism of hypoxia and cold is supported by the fact that hypoxia probably inhibits cold-induced thermogenesis (Gautier et al., 1991; Tattersall and Milsom, 2009).
Still, this issue is controversial. Chaillou (2018) has stated that the fiber-type composition of limb skeletal muscles in both adult animals and humans is affected only when combined with intense physical activity. In the same review by Chaillou (2018), it has been stressed that an alteration of the fast-to-slow shift in the fiber type of the soleus muscle during postnatal development in growing rats exposed to severe altitude most likely results from the reduced locomotion, or hypokinesia, consecutive to hypoxia exposure. Similarly, the slow-to-fast shift in the muscle fiber type is common in patients with COPD, which is characterized by a sedentary lifestyle (Chaillou, 2018). This prompts that the modeling effect of hypoxia is better manifested when it interacts with an agonistic concomitant factor, for example, early postnatal period or hypokinesia (Chaillou, 2018).
3.3.2 Postnatal development
In rats, in the early postnatal period, fast-type muscle fibers predominate in skeletal muscles. For example, the soleus, which is a typical slow muscle in adult rats (Novák et al., 2010), in the rat pup contains 55% of fast-type fibers (Thompson et al., 1984). As an organism grows, faster-type muscle fibers transform into slow-type fibers owing to changing properties of their motoneurones from phasic to tonic (Kugelberg, 1976). The “fast-to-slow” shift in muscle fiber composition in growing rats is retarded or even abolished by such a model of microgravity as hind limb suspension (Morey-Holton and Globus, 1998; Huckstorf et al., 2000), which agrees with the statement of Chaillou (2018) that the modeling effect on muscle fiber composition is better manifested in early postnatal development. In rats, only the phasic (oscillating) EMG pattern is present in the early postnatal period, and tonic (stationary) EMG activity emerges between the 11th and 16th days of life (Westerga and Gramsbergen, 1994; Eken et al., 2008).
In human term neonates and guinea pigs, unlike rats, EMG is represented by both phasic and tonic patterns (Gutmann et al., 1975; Schloon et al., 1976; Meigal et al., 1995). In addition, two different patterns of motor unit (MU) activity in newborns were reported by Meigal et al. (1995). One of these patterns represented 75% of all MUs, which persistently discharged at rates 7–15 imp/s. Another pattern (the other 25% of MUs) manifested itself in a form of short (1–2 s) periods of MU action potential with a firing rate of 20–50 imp/s and uniform interspike intervals. Such a “periodic” pattern of high-frequency MU was not evidenced in 1-year-old children (Meigal et al., 1995). In human neonates, such a phasic EMG pattern probably coincides with increased synchronization of MU activity (Del Vecchio et al., 2020). As for the muscle fiber content, in the human fetus, during delivery, the stage of muscle development in the human fetus corresponded to phase III, which means '...with an approximate equal distribution of the two types’ (slow and fast non-differentiated fibers) (Dubowitz, 1966). Thus, slower muscle fibers are already present in newborn's muscles before delivery. Therefore, during the postnatal period, the muscle fiber content in humans, most probably, is not driven by gravity.
3.3.3 Body size
In addition to environmental physical and developmental factors, the body size per se can serve as a modeling factor of the neuromuscular system. Indeed, in animals with a small body size, there is an increased proportion of type II muscle fibers (faster) because “... muscular adaptations to a small body size were met primarily by increased type IIA fiber proportions” (Hesse et al., 2010:446). More specifically, “... smaller animals ... have a smaller postural problem than larger ones because as linear dimensions increase, the weight of an animal increases by the power of three” (Hesse et al., 2010:446).
Human newborns are, indeed, small in size. Consequently, the size factor could contribute to the predominance of faster-type fibers in newborns. However, this is not the case because according to a study by Tambovtseva and Kornienko (1986b) and Kumagai et al. (1984), newborn's muscles (mm. quadriceps, psoas) are characterized by a nearly equal content of slow-type muscle fibers and undifferentiated type fibers. Therefore, the body size factor is not applicable to the concept of the Baby Astronaut.
3.3.4 Inactivity
Inactivity, modeled with bed confinement (bed rest), limb suspension or caused by chronic diseases or injuries provokes a slow-to-fast shift in muscle fiber composition (Oishi et al., 1998; Borina et al., 2010). The intrauterine environment is partially similar to the conditions of inactivity, as it does not allow a fetus to freely move (Sekulic et al., 2005; Stanojević et al., 2011; Stanojević et al., 2012). Still, inactivity in the fetus is not absolute as the fetus produces spontaneous (from weeks 10–11 of gestation) and writhing movements (starting from weeks 36–38) (Hart, 2006). Therefore, the factor of inactivity can well contribute to the effect of microgravity, heat, and hypoxia. However, the factor of inactivity in the fetus cannot be regarded as actual for muscle modifications.
3.4 Statement 4: “Synergistic adaptations of the motor system to different environments come from their ontogenetic synchronicity” (Meigal, 2013)
Thus, heat, microgravity, and hypoxia have much in common in their effect on the neuromuscular system. Similarly, there is much in common in the effect of cold, gravity, and normal oxygenation.
Then comes the question: What are the evolutionary origins of such a commonality?
Logically speaking, such a unidirectional (agonistic, parallel, and convergent) effect of several apparently different environmental factors would have arisen because of their synchronicity. In other words, they once acted together to gain convergent physiological functionality.
There is a good example of how several different factors can simultaneously cause almost the same effect. Namely, 1) a decrease in pH (acidosis), 2) an increase in the partial pressure of CO2 (hypercapnia), and 3) an increase in muscle tissue temperature (hyperthermia) are synchronously represented in exercising muscle and all of them reduce the affinity of O2 to Hb (the right shift of O2–Hb affinity curve, or the Bohr effect) (Weber and Campbell, 2011; Dash et al., 2016). As for heterogeneous factors such as heat, microgravity, and hypoxia (as well as small size and hypokinesia), we assumed that their convergent effect on the motor system stems from their synchronicity in the prenatal intrauterine state (Meigal, 2012, 2013). Indeed, the intrauterine habitat synchronously provides 1) microgravity due to a condition of “wet” immersion in amniotic fluid, 2) heat (up to 38°C), and 3) hypoxia (pO2 = 40 mm Hg in fetal arterial blood). Accordingly, after birth, this triad of environmental factors converts into a subsequent triad represented by 1) normal gravity, 2) lower ambient temperature, and 3) normoxia. In addition, the intrauterine environment is characterized by darkness and relative silence, which after birth turns into an illuminated and noisier extrauterine environment.
Thus, the “intrauterine triad” (microgravity, heat, hypoxia), as was shown afore, favors a “faster” state of the neuromuscular system. Earlier, this was nominated by us as a FM strategy (with allusion to such terms as “faster muscle fibers,” “fetal,” “microgravity,” or “Moon”), and the “extrauterine triad” as GE (with allusion to “gravity,” “Geo,” or “Earth”). We also speculatively suggested that there may be n additional hypothetic triad of environmental conditions characterized by 1) hypergravity, 2) strong cold, and 3) hyperoxia (provisionally nominated as SL, with allusion to “slow” and “Sun”) (Meigal, 2013).
Also, we speculatively suggested that the intrauterine environment could simulate a spaceflight followed by a return to Earth (birth). This assumption constituted the essence of the conception of the Baby Astronaut. Accordingly, the vaginal delivery can be analogous to uncontrolled landing of a space vehicle and Caesar's section to the controlled one.
3.4.1 Criticism with respect to other possibilities for synchronization of temperature and gravity
Some other possible synchronicities can be suggested to explain common reactions between varied gravity and temperature modalities.
1. One can argue that cold may coincide with gravity due to a geographical factor, since the polar regions that presumably should be cold are also characterized by a slightly (mere 0.5%) higher G due to the smaller radius of the Earth there. However, such a difference is negligible (0,05 G) to explain the unidirectional effect of cold and gravity on the neuromuscular system.
2. According to the Weber's silver Thaler illusion, colder objects of the same weight appear as twice heavier (Cahusac and Noyce, 2007), which neither can be considered as a good explanation of the convergent effect of temperature and gravity.
3. As the thermal grill illusion suggests, the integration of tactile, cold, warm, and pain stimuli takes place on the supraspinal level (Ferrè et al., 2018). However, that illusion does not consider gravity.
4. Hypoxia and cold usually coexist at high altitude (Wang et al., 2012). Therefore, they must be agonistic with respect to acclimation, which is not the case (Wang et al., 2012).
3.4.2 Criticism with respect to the intrauterine buoyant force and G value
Over the past years, we found several studies that provided additional experimental data for the revision of the concept of the Baby Astronaut. First, we learned that actually there is no such abrupt increase in the G value after birth as we originally assumed. Starting from the 26th week, the fetus experiences substantial reduction in buoyancy, and, respectively, microgravity because he has significant contact with the inner wall, which reduces the submersed part of the fetus (Sekulić et al., 2005). After the 26th gestation week, the fetus has 60–80% apparent weight (Sekulić et al., 2005), which corresponds to 0.6–0.8G. In addition to the atmospheric pressure (760 mm Hg or 101.325 kPa), the fetus is exposed to 5 mm Hg (0.66 kPa) of the intrauterine pressure (Sideris and Nicolaides, 1990). Thus, in the second half of gestation, the fetus is not an “astronaut” actually (Sekulić et al., 2005). By its effect, such a mechanical contact (stress) must be analogous to the afferent flow from the sole proprioceptors (“support” receptors), which is essential for maintaining the antigravity muscle tone (Kozlovskaya and Kirenskaya, 2004; Amirova et al., 2021). In addition, the kick force of the fetus in utero and stress and strain on the skeleton of the fetus increase significantly right after the week 20 of gestation (Verbruggen et al., 2018). Thus, the buoyant force starting from the 20th week of gestation is actually decreased, which appears as an argument, which is not in favor of the Baby Astronaut hypothesis. Still, before 20 weeks’ gestation, the analogy of intrauterine immersion and microgravity can be regarded as valid.
Thus, for a full-term baby, the gradient of actual gravity between the intra- and extrauterine condition must be only 0.2 G, and for a premature baby—0.4 G. As such, the fetus during the last few weeks of the intrauterine environment is “preparing” for living extrauterine at 1 G. Accordingly, for a premature baby, transition to extrauterine condition must be more stressful. Second, we found that the idea of the “intrauterine conditions as microgravity” and “extrauterine conditions as gravity” have been proposed also by Stanojevic et al. (2011), Stanojevic et al. (2012) in a paradigm of continuity of neurobehavioral development and discontinuity of environmental conditions.
Thus, the in utero fetus is subjected to decreased gravity due to the immersion (buoyancy) effect, higher temperature (around 37°C), and hypoxia (around 40 mm PO2). Then, after birth, the neonate finds him/herself exposed to colder, normoxic atmosphere, at 1 G (Figure 2). The revisited conception of the “Baby Astronaut” is schematized in Figure 2, using the original scheme from the study by Meigal (2013), and the studies by Sekulić et al. (2005) and Zaripova and Meigal (2018).
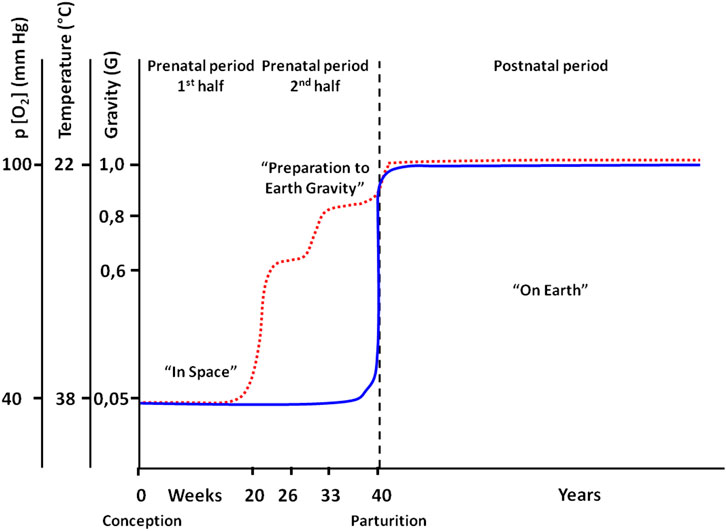
FIGURE 2. Conceptual scheme of gravity-related events and confounding environmental factors in pre- and postnatal periods of a human’s life, with analogs of spaceflight events. Blue solid line: the original; red dotted line: modified imagination of the perceived gravity (based on the study by Sekulić et al. (2005).
3.4.3 Criticism with respect to the models of cross-adaptation and cross-tolerance between environmental factors
Thus, microgravity, heat, and hypoxia have much in common in their effect on the motor system. These can be considered as parallel (convergent) adaptations, which individually can lead to similar results. In addition to parallelism, acclimation to one environmental stressor can enhance adaptation to other stressors, which has been described as the phenomenon of cross-tolerance (White et al., 2014) or cross-adaptation. Cross-tolerance (cross-adaptation) between various environmental stressors is widely studied, for example, between temperature (heat, cold) and hypoxia (Gibson et al., 2017; Rendell et al., 2017), heat and modeled microgravity (Debevec et al., 2018). In general, it has been shown that acclimation to heat increases acclimation to hypoxia (White et al., 2014), though there are studies, which present contradictory results (Rendell et al., 2017). The cross-relationship in acclimation to hypoxia and cold was shown to be negative (Fregly, 1954; Sorokina et al., 1984; Wang et al., 2012). On the other hand, there is a study, which does not evidence cross-tolerance between heat and hypoxia (Rendell et al., 2017).
We sought for studies that would have evidenced the phenomenon of cross-adaptation or cross-tolerance between microgravity and heat and microgravity and hypoxia, in their modeling action on the skeletal muscle. However, we did not find such studies. Thus, we can only state that microgravity, heat, and hypoxia are rather agonistic or convergent than synergistic in their effect on the motor system.
3.4.4 Criticism with respect to maturation by birth (altricial and precocial species)
There are two clearly different categories of homeothermic species. First, those who were born immature and were relatively immobile (altricial species, for example, rat, mouse, and golden hamster); and second, mature, those who were capable of moving right after birth (precocial, for example, guinea pig and sheep). In altricial species (rats), slow-type muscle fibers emerge only a few weeks after birth (Tambovtseva and Kornienko, 1986a). Therefore, in rats, the fiber type content can be modified by lower extrauterine temperature and gravity (microgravity). In humans, although they are classified as altricial species, slow muscle fibers are already present long before birth (Kumagai et al., 1984; Tambovtseva and Kornienko, 1986b), and, therefore, gravity and lower temperature cannot be considered as the major factors of postnatal muscle modification in human infants.
3.5 Statement 5: Several specific predictions of the effects of microgravity on various physiological parameters were drawn from the concept of Baby Astronaut
“Useful theories make predictions” (Howes A., 2012). In the original study (Meigal, 2012; Meigal, 2013), we have presented several predictions based on the concept of Baby Astronaut.
3.5.1 Sound and light might modulate muscle tone
“According to the concept of Baby Astronaut, the effect of darkness and silence, as inherent factors of the intrauterine habitat, on the neuromuscular system should correspond to the fetal strategy (FM strategy) due to their temporary coincidence with microgravity, heat, and hypoxia. Nevertheless, the pre-existing academic literature provides studies that demonstrate that the fetus is actually exposed to relatively bright illumination under intrauterine conditions (from 10 to 250 lx, which corresponds to a dimly or artificially lit room), depending on the external luminance and abdominal thickness (Del Giudice, 2011). Starting from weeks 30 to 32, the vision system allows the fetus to see (Del Giudice, 2011). Therefore, light can well contribute to motor development of the fetus during the last 10 weeks of gestation. The fetus does not experiences silence because intrauterine noise ranges between 72 and 96 db, which can be considered loud (Walker et al., 1971; Smith et al., 1990). Consequently, we have to refute the prediction that darkness would have contributed to muscle development in the fetus during the second half of gestation.
Still, it sounds quite reasonable that darkness or silence contributes to a decrease in muscle tone, for example, during sleeping, while bright light or noise increases it. Also, it is well-known that sound and light stimuli provoke postural and behavioral reactions. Nonetheless, in the academic literature, we found no direct evidence that long-lasting darkness, light, silence, or sound/noise can modulate muscle tone or muscle fiber composition either separately or along with other concomitant factors (microgravity, heat, and hypoxia). This issue can be addressed by exploring muscle fiber composition or the intensity of muscle tone in people with long-term deprivation of hearing (people with deafness or bad hearing) or vision (people with blindness, or bad sighted people). So far, we did not find such studies in the academic literature."
3.5.2 The affinity of O2 to Hb in space should be reduced because microgravity is cooperative with the joint effect of hypoxia and heat on it (the Bohr effect)
In the academic literature, we still did not find the studies, which present data on modification oxygen affinity to Hb under the conditions of weightlessness.
3.5.3 Skeletal muscle must have remodeled in women during pregnancy and postpartum
The state of gravidity, which adds some extra 10 kg to women’s weight, simulates hypergravity, and thus might also appear as a “pro-gravity” factor. We presumed that in pregnant women, a fast-to-slow shift in muscle fiber composition and corresponding modification in MU activity would be observed. We did not find corresponding studies to support this assumption.
3.5.4 The Baby Astronaut concept and parkinsonism
Parkinsonism is characterized by enhanced muscle tone (rigidity), rest tremor, brady-/akinesia, gait, and posture disorders (spontaneous falls, “freezing” of gait). These are strikingly similar to those provoked by cold (thermoregulatory muscle tone, cold shivering, and slowed movements) (Meigal and Lupandin, 2005). In individuals with parkinsonism, the slower fiber type predominate in skeletal muscles in comparison to young and older healthy subjects (Rossi et al., 1996; Lavin et al., 2020), probably due to persistent muscle rigidity. Usually, subjects with parkinsonism have elevated rest energy expenditure and lowered body weight (Capecci et al., 2013). However, we did not find evidence from the literature that this is specifically caused by selection of lipids as a fuel.
Thus, the concept of Baby Astronaut parkinsonism is similar to an enhanced variant of GE strategy. This allows parkinsonism as a “pro-gravity” factor. In support of that, in several past years, we found that muscle rigidity indeed is decreased under a program of short sessions of microgravity modeled with “dry” immersion (Meigal et al., 2018). However, there is no possibility to explore the effect of long-duration, either real or modeled, weightlessness on muscle fiber composition in people with parkinsonism, though older people already travel to space for some tens of minutes as tourists.
3.6 Statement 6: Some practical considerations were formulated on how to apply cold in prophylactic countermeasures in space
We suggested that the studied factors, namely, temperature and gravity, may be “equivalent” (or “isodynamic”) to each other. Since the amplitude of surface EMG of weakly contracting skeletal muscle is increased by some 20%, the MU firing rate in humans is decreased by 20% in the cold, and the MU firing rate is decreased by 30% under acclimation to cold, we suggested that in real weightlessness in space, flight cold could well “substitute” up to 20% of Earth's gravity. Therefore, from the physiological point of view, cold can have a kind of “gravity” value of up to 0,2 G (Meigal, 2013). Having in the mind that cold and gravity are convergent in the action on the neuromuscular system, cold could well have been applied as “surrogate gravity” (“Ersatzgravitation”).
From the pre-existing literature, we found two studies by Arshavskii et al. (1980, 1987) which evidenced that in developing rats, cold favored growth of body weight and skeletal muscle mass.
We fully realize that the “mainstream” research in the field of prophylactic measures on board a space vehicle during long-duration space missions (interplanetary spaceflights, e.g., to Mars) lies entirely within the concept of artificial gravity, modeled with, for example, centrifuge construction. In addition, cold is potentially a hazardous condition because of cold-induced infection, which should not have occurred on board of a space vehicle.
Nonetheless, we believe that cold as a partially equivalent factor for gravity can be interesting per se, as a curious and potentially productive idea that could contribute to the fields of gravitational physiology, cross-adaptation, rehabilitation, and physiology in general.
4 Conclusion
The aim of the present study was dual—1) critically review the idea of unidirectional physiological effects of cold and gravity, as well as heat and microgravity on the neuromuscular system, and 2) explain the convergent physiological effect of temperature and gravity modalities using the concept of the ontogenetic synchronicity (or, the Baby Astronaut concept) of these factors before (immersion-based microgravity, heat, and hypoxia) and after (Earth gravity, lower air temperature, and normoxia) the birth of a child.
We can conclude that the idea of physiological analogy between gravity and cold is justified by numerous, albeit indirect, evidence from the academic literature. However, studies have also been found that do not support this concept. We consider the idea of unidirectional physiological effects of temperature and gravity modalities plausible, although not yet confirmed experimentally. We have proposed several experiments which could have helped verifying that concept. In general, we consider statements 1 and 2 as experimentally provable, hence empirical. Of these two statements, statement 1 seems more valid than statement 2.
As for statements 3 and 4, we conclude that currently there is no convincing evidence from the academic literature to support them. There are two major problematic issues. First, there are two clearly different categories of homeothermic species—those that are born immature (altricial species, for example, rat), and mature (precocial, for example, guinea pig). In rats, slow-type muscle fibers appear a few weeks after birth and, consequently, the fiber type content can be modified by lower extrauterine temperature and lowered gravity (microgravity). In humans, slow muscle fibers are already present before birth and, therefore, gravity and lower temperature cannot be regarded as main drivers of muscle modification. Second, in utero, in the second half of gestation, the fetus body experiences substantial reduction in buoyancy, and, respectively, microgravity so that the fetus has 60–80% apparent weight (Sekulić et al., 2005), which corresponds to 0.6–0.8G. In addition, the fetus is not inactive, as it produces movements. Third, we did not find convincing empirical evidence on cross-adaptation between microgravity, high temperature, and hypoxia. Therefore, the intrauterine environment, especially in the second half of gestation, cannot be regarded as a relevant model of microgravity.
In general, the concept of Baby Astronaut can be regarded as valid only for species such as a rat (immature, altricial), but not for a human fetus. With respect to the aforementioned conclusions, as of now, statement 4 should be considered invalid.
5 Prospective
For a more profound substantiation of the concept of Baby Astronaut, more physiological systems can be taken into consideration, for example, hemodynamics and blood. There are studies, which provide evidence that the effect of exposure to heat (“warm water” effect, “passive heat therapy”) and microgravity, modeled with either water or “dry” immersion, exert a similar relaxing effect on smooth musculature of vessels (Brunt et al., 2016; Sugawara and Tomoto, 2020; Gerasimova-Meigal et al., 2021). Then, Lossec et al. (1999) evidenced rapid postnatal improvement of cardiovascular adjustments favoring blood perfusion and probably heat production during cold-induced shivering in the most oxidative muscles studied.
In addition, data on the neuromuscular development of infants under such specific conditions as multifetal gestation (twin, triplet, and other higher-order pregnancy) (Yudin et al., 2001) and hydramnios could be of potential value because these two conditions specifically contribute to intrauterine buoyancy of the fetus.
Next, there are specific “visceral-motor” functions of the motor system, for example, breathing, defecation, delivery, vomiting, and swallowing. These functions require activity of the skeletal musculature (respiratory muscles, the upper portion of esophagus, m. sphincter ani ext., m. levator ani, etc.). The study by Gerasimova and Kuz'mina (1996) reported that the muscles of the pelvic floor of the cat are involved in thermoregulatory muscle tone under cooling conditions. Similarly, respiratory muscles are involved in cold shivering (Burachevskaia, 1981). This points on a potentially informative approach to study interaction and interrelationship between gravity and temperature.
Finally, in this study, we did not review potential expansion of the studied concept to the field of extreme modalities of gravity and cold, such as hypergravity and strong cold. We earlier considered potential parallelism of hypergravity, cold, and hyperoxia (Meigal, 2012, 2013), but it looks too much speculative.
Data availability statement
The original contributions presented in the study are included in the article; further inquiries can be directed to the corresponding author.
Author contributions
AM contributed to the basic concept of the study, study design, the interpretation of results, writing of the manuscript, and approval of the final draft. LG contributed to study design, search for literature, the interpretation of results, writing of the manuscript, and approval of the final draft.
Funding
This research was financially supported by the Ministry of Science and Higher Education of the Russian Federation (Theme No. 0752-2020-0007).
Acknowledgments
The authors are grateful to their scientific supervisor and teacher in physiology, Professor Yuri Lupandin (1938-2020, Petrozavodsk State University), who introduced them to the fields of thermal physiology, electromyography, and physiology of the motor system.
Conflict of interest
The authors declare that the research was conducted in the absence of any commercial or financial relationships that could be construed as a potential conflict of interest.
Publisher’s note
All claims expressed in this article are solely those of the authors and do not necessarily represent those of their affiliated organizations, or those of the publisher, the editors, and the reviewers. Any product that may be evaluated in this article, or claim that may be made by its manufacturer, is not guaranteed or endorsed by the publisher.
References
Amirova, L. E., Plehuna, A., Rukavishnikov, I. V., Saveko, A. A., Peipsi, A., and Tomilovskaya, E. S. (2021). Sharp changes in muscle tone in humans under simulated microgravity. Front. Physiol. 12, 661922. doi:10.3389/fphys.2021.661922
Arshavskii, I. A., Rozanova, V. D., and Surovtseva, Z. F. (1987). Role of motor activity in the development of hypothyroid rat pups. Zh. Evol. Biokhim. Fiziol. 23, 514–518.
Arshavskii, I. A., Rozanova, V. D., Surovtseva, Z. F., and Gaĭlish, L. G. (1980). EFfect of exposure to cold on growth characteristics and anabolic processes developing rats. Zh. Evol. Biokhim. Fiziol. 16, 137–142.
Baldwin, K. M., and Haddad, F. (2001). Invited Review: Effects of different activity and inactivity paradigms on myosin heavy chain gene expression in striated muscle. J. Appl. Physiol. (1985). 90, 345–357. doi:10.1152/jappl.2001.90.1.345
Ball, D. (2021). Contrasting effects of heat stress on neuromuscular performance. Exp. Physiol. 106, 2328–2334. doi:10.1113/EP088191
Baumeister, R. F., and Leary, M. R. (1997). Writing narrative literature reviews. Rev. Gen. Psychol. 1, 311–320. doi:10.1037/1089-2680.1.3.311
Bernstein, N. A. (1947). O postroenii dvizhenii [On the construction of movements]. Soviet Union, Russia, China: Medgiz.
Borina, E., Pellegrino, M. A., D'Antona, G., and Bottinelli, R. (2010). Myosin and actin content of human skeletal muscle fibers following 35 days bed rest. Scand. J. Med. Sci. Sports 20, 65–73. doi:10.1111/j.1600-0838.2009.01029.x
Bozzo, C., Stevens, L., Bouet, V., Montel, V., Picquet, F., Falempin, M., et al. (2004). Hypergravity from conception to adult stage: Effects on contractile properties and skeletal muscle phenotype. J. Exp. Biol. 207, 2793–2802. doi:10.1242/jeb.01076
Brunt, V. E., Howard, M. J., Francisco, M. A., Ely, B. R., and Minson, C. T. (2016). Passive heat therapy improves endothelial function, arterial stiffness and blood pressure in sedentary humans. J. Physiol. 594 (18), 5329–5342. doi:10.1113/JP272453
Burachevskaia, L. E. (1981). Intercostal muscle motor unit activity during shivering. Fiziol. Zh. SSSR Im. I. M. Sechenova 67, 306–312.
Cahusac, P. M., and Noyce, R. A. (2007). A pharmacological study of slowly adapting mechanoreceptors responsive to cold thermal stimulation. Neuroscience 148, 489–500. doi:10.1016/j.neuroscience.2007.06.018
Capecci, M., Petrelli, M., Emanuelli, B., Millevolte, M., Nicolai, A., Provinciali, L., et al. (2013). Rest energy expenditure in Parkinson's disease: Role of disease progression and dopaminergic therapy. Park. Relat. Disord. 19, 238–241. doi:10.1016/j.parkreldis.2012.10.016
Chaillou, T. (2018). Skeletal muscle fiber type in hypoxia: Adaptation to high-altitude exposure and under conditions of pathological hypoxia. Front. Physiol. 9, 1450. doi:10.3389/fphys.2018.01450
Comfort, P., McMahon, J. J., Jones, P. A., Cuthbert, M., Kendall, K., Lake, J. P., et al. (2021). Effects of spaceflight on musculoskeletal health: A systematic review and meta-analysis, considerations for interplanetary travel. Sports Med. 51, 2097–2114. doi:10.1007/s40279-021-01496-9
Dash, R. K., Korman, B., and Bassingthwaighte, J. B. (2016). Simple accurate mathematical models of blood HbO2 and HbCO2 dissociation curves at varied physiological conditions: Evaluation and comparison with other models. Eur. J. Appl. Physiol. 116, 97–113. doi:10.1007/s00421-015-3228-3
Debevec, T., Ganse, B., Mittag, U., Eiken, O., Mekjavic, I. B., and Rittweger, J. (2018). Hypoxia aggravates inactivity-related muscle wasting. Front. Physiol. 9, 494. doi:10.3389/fphys.2018.00494
Del Giudice, M. (2011). Alone in the dark? Modeling the conditions for visual experience in human fetuses. Dev. Psychobiol. 53, 214–219. doi:10.1002/dev.20506
Del Vecchio, A., Sylos-Labini, F., Mondì, V., Paolillo, P., Ivanenko, Y., Lacquaniti, F., et al. (2020). Spinal motoneurons of the human newborn are highly synchronized during leg movements. Sci. Adv. 6 (47), eabc3916. doi:10.1126/sciadv.abc3916
Demangel, R., Treffel, L., Py, G., Brioche, T., Pagano, A. F., Bareille, M. P., et al. (2017). Early structural and functional signature of 3-day human skeletal muscle disuse using the dry immersion model. J. Physiol. 595, 4301–4315. doi:10.1113/JP273895
Dubowitz, V. (1966). Enzyme histochemistry of developing human muscle. Nature 211 (5051), 884–885. doi:10.1038/211884a0
Eken, T., Elder, G. C., and Lømo, T. (2008). Development of tonic firing behavior in rat soleus muscle. J. Neurophysiol. 99, 1899–1905. doi:10.1152/jn.00834.2007
Ferrè, E. R., Iannetti, G. D., van Dijk, J. A., and Haggard, P. (2018). Ineffectiveness of tactile gating shows cortical basis of nociceptive signaling in the Thermal Grill Illusion. Sci. Rep. 8, 6584. doi:10.1038/s41598-018-24635-1
Fregly, M. J. (1954). Cross-acclimatization between cold and altitude in rats. Am. J. Physiology-Legacy Content 176, 267–274. doi:10.1152/ajplegacy.1954.176.2.267
Freund, H. J. (1983). Motor unit and muscle activity in voluntary motor control. Physiol. Rev. 63, 387–436. doi:10.1152/physrev.1983.63.2.387
Ganguly, J., Kulshreshtha, D., Almotiri, M., and Jog, M. (2021). Muscle tone physiology and abnormalities. Toxins (Basel). 13, 282. doi:10.3390/toxins13040282
Gautier, H., Bonora, M., M'Barek, S. B., and Sinclair, J. D. (1991). Effects of hypoxia and cold acclimation on thermoregulation in the rat. J. Appl. Physiol. (1985). 71, 1355–1363. doi:10.1152/jappl.1991.71.4.1355
Gerasimova, L. I., and Kuz'mina, G. I. (1996). The thermoregulating function of the motor units in the muscles of the pelvic floor of the cat. Fiziol. Zh. Im. I. M. Sechenova 82, 98–103.
Gerasimova-Meigal, L., Meigal, A., Sireneva, N., and Saenko, I. (2021). Autonomic function in Parkinson's disease subjects across repeated short-term dry immersion: Evidence from linear and non-linear hrv parameters. Front. Physiol. 12, 712365. doi:10.3389/fphys.2021.712365
Gibson, O. R., Taylor, L., Watt, P. W., and Maxwell, N. S. (2017). Cross-adaptation: Heat and cold adaptation to improve physiological and cellular responses to hypoxia. Sports Med. 47, 1751–1768. doi:10.1007/s40279-017-0717-z
Golenhofen, K. (1963). Zur topographie der muskelaktivitaet bei kaeltebelastung des menschen [On the topography of muscle activity during cold stress in man]. Arch. Phys. Ther. 15, 435–437.
Göpfert, H., and Stuffer, R. (1952). Die Vorstadien des Kaltezitterns bei geringer Abkuhlung des Menschen [The forestages of shivering in slight chilling in man]. Pflugers. Arch. 256, 161–180. doi:10.1007/bf00369639
Goswami, N. (2017). Falls and fall-prevention in older persons: Geriatrics meets spaceflight. Front. Physiol. 118, 603. doi:10.3389/fphys.2017.00603
Graat-Verboom, L., Wouters, E. F., Smeenk, F. W., van den Borne, B. E., Lunde, R., and Spruit, M. A. (2009). Current status of research on osteoporosis in COPD: A systematic review. Eur. Respir. J. 34, 209–218. doi:10.1183/09031936.50130408
Gurfinkel', V. S., Levik, YuS., Poleshchuk, N. K., and Korovin, YuV. (1981). Dependence of heat production by skeletal muscle on its pattern of contraction. Hum. Physiol. 7, 22–29.
Gutmann, E., Melichna, J., and Syrový, I. (1975). Developmental changes in contraction time and muscle fibre pattern of fast and slow muscles. Experientia 29, 435–436. doi:10.1007/BF01926765
Haman, F., Blondin, D. P., Imbeault, M. A., and Maneshi, A. (2010). Metabolic requirements of shivering humans. Front. Biosci. 2, 124–1168. doi:10.2741/s124
Haman, F., Legault, S. R., Rakobowchuk, M., Ducharme, M. B., and Weber, J. M. (2004). Effects of carbohydrate availability on sustained shivering II. Relating muscle recruitment to fuel selection. J. Appl. Physiol. (1985). 96, 41–49. doi:10.1152/japplphysiol.00428.2003
Haman, F. (2006). Shivering in the cold: From mechanisms of fuel selection to survival. J. Appl. Physiol. (1985) 100, 1702–1708. doi:10.1152/japplphysiol.01088.2005
Hart, H. (2006). Fetal and infant movements and the young nervous system. Dev. Med. Child. Neurol. 48, 547. doi:10.1017/S0012162206001149
Herpin, P., Lossec, G., Schmidt, I., Cohen-Adad, F., Duchamp, C., Lefaucheur, L., et al. (2002). Effect of age and cold exposure on morphofunctional characteristics of skeletal muscle in neonatal pigs. Pflügers. Archiv Eur. J. Physiology 444, 610–618. doi:10.1007/s00424-002-0867-0
Hesse, B., Fischer, M. S., and Schilling, N. (2010). Distribution pattern of muscle fiber types in the perivertebral musculature of two different sized species of mice. Anat. Rec. 293, 446–463. doi:10.1002/ar.21090
Hirabayashi, M., Ijiri, D., Kamei, Y., Tajima, A., and Kanai, Y. (2005). Transformation of skeletal muscle from fast- to slow-twitch during acquisition of cold tolerance in the chick. Endocrinol 146, 399–405. doi:10.1210/en.2004-0723
Howes, A. (2012). Useful theories make predictions. Top. Cogn. Sci. 4, 84–86. doi:10.1111/j.1756-8765.2011.01174.x
Huckstorf, B. L., Slocum, G. R., Bain, J. L., Reiser, P. M., Sedlak, F. R., Wong-Riley, M. T., et al. (2000). Effects of hindlimb unloading on neuromuscular development of neonatal rats. Dev. Brain Res. 119, 169–178. doi:10.1016/s0165-3806(99)00167-4
Ishihara, A., Itoh, K., Itoh, M., and Hirofuji, C. (2000). Effect of hypobaric hypoxia on rat soleus muscle fibers and their innervating motoneurons: A review. Jpn. J. Physiol. 50, 561–568. doi:10.2170/jjphysiol.50.561
Ishihara, A., Oishi, Y., Roy, R. R., and Edgerton, V. R. (1997). Influence of two weeks of non-weight bearing on rat soleus motoneurons and muscle fibers. Aviat. Space Environ. Med. 68, 421–425.
Islas, L. D. (2014). Thermal effects and sensitivity of biological membranes. Curr. Top. Membr. 74, 1–17. doi:10.1016/B978-0-12-800181-3.00001-4
Israel, D. J., and Pozos, R. S. (1989). Synchronized slow-amplitude modulations in the electromyograms of shivering muscles. J. Appl. Physiol. (1985). 66, 2358–2363. doi:10.1152/jappl.1989.66.5.2358
IUPS Thermal Commission (1987). Glossary of terms for thermal physiology. Pflugers Arch. 410, 567–587. doi:10.1007/BF00586542
Kozlovskaya, I. B., and Kirenskaya, A. V. (2004). Mechanisms of disorders of the characteristics of fine movements in long-term hypokinesia. Neurosci. Behav. Physiol. 34, 747–754. doi:10.1023/b:neab.0000036017.46801.5c
Kugelberg, E. (1976). Adaptive transformation of rat soleus motor units during growth. J. Neurol. Sci. 27, 269–289. doi:10.1016/0022-510x(76)90001-0
Kumagai, T., Hakamada, S., Hara, K., Takeuchi, T., Miyazaki, S., Watanabe, K., et al. (1984). Development of human fetal muscles: A comparative histochemical analysis of the psoas and the quadriceps muscles. Neuropediatrics 15, 198–202. doi:10.1055/s-2008-1052366
Kuokkanen, L. P. (1989). The effect of adaptation to heat and increased motor activity on the thermoregulatory function of the motor neuron pool. Fiziol. Zh. SSSR Im. I. M. Sechenova 75, 1063–1068.
Kuz'mina, G. I. (1980). [Bioelectrical activity of limb muscles during shivering while stimulating the vestibular apparatus]. Fiziol. Zh. SSSR Im. I. M. Sechenova 66, 702–708.
Lavin, K. M., Sealfon, S. C., McDonald, M. N., Roberts, B. M., Wilk, K., Nair, V. D., et al. (2020). Skeletal muscle transcriptional networks linked to type I myofiber grouping in Parkinson's disease. J. Appl. Physiol. (1985). 128, 229–240. doi:10.1152/japplphysiol.00702.2019
Lefaucheur, L., Ecolan, P., Lossec, G., Gabillard, J. C., Butler-Browne, G. S., and Herpin, P. (2001). Influence of early postnatal cold exposure on myofiber maturation in pig skeletal muscle. J. Muscle Res. Cell Motil. 22, 439–452. doi:10.1023/a:1014591818870
Lømo, T., Eken, T., Bekkestad Rein, E., and Njå, A. (2020). Body temperature control in rats by muscle tone during rest or sleep. Acta Physiol. 228, e13348. doi:10.1111/apha.13348
Lossec, G., Duchamp, C., Lebreton, Y., and Herpin, P. (1999). Postnatal changes in regional blood flow during cold-induced shivering in sow-reared piglets. Can. J. Physiol. Pharmacol. 77, 414–421. doi:10.1139/y99-034
Lupandin, I. (1983). Regulation of the function of gamma- and alpha-motor neurons of antagonist muscles during cold tremor in the cat. Neirofiziol 15, 242–248.
Lupandin Iu., V. (1980). [Characteristics of motor neuron pool function in cold tremor]. Fiziol. Zh. SSSR Im. I. M. Sechenova 66, 1772–1777.
Lupandin, Iu. V., and Kuz'mina, G. I. (1985). Vzaimodeĭstvie termoretseptivnoĭ i vestibuliarnoĭ signalizatsii v reguliatsii aktivnosti fleksornogo i ékstenzornogo dvigatel'nykh iader vo vremia kholodovogo tremora [Interaction of thermoreceptive and vestibular signalling in regulating the activity of flexor and extensor motor nuclei during cold tremor]. Fiziol. Zhurn. SSSR Im. I. M. Sechenova 71, 1433–1438.
Lupandin, IuV. (1979). Role of the fusimotor system in regulating shivering. Fiziol. Zh. SSSR Im. I. M. Sechenova 65, 1661–1670.
Mallette, M. M., Cheung, S. S., Kumar, R. I., Hodges, G. J., Holmes, M. W. R., and Gabriel, D. A. (2021). The effects of local forearm heating and cooling on motor unit properties during submaximal contractions. Exp. Physiol. 106, 200–211. doi:10.1113/EP088256
Mallette, M. M., Green, L. A., Gabriel, D. A., and Cheung, S. S. (2018). The effects of local forearm muscle cooling on motor unit properties. Eur. J. Appl. Physiol. 118, 401–410. doi:10.1007/s00421-017-3782-y
Masi, A. T., and Hannon, J. C. (2008). Human resting muscle tone (HRMT): Narrative introduction and modern concepts. J. Bodyw. Mov. Ther. 12, 320–332. doi:10.1016/j.jbmt.2008.05.007
Masiero, S., Maghini, I., Mantovani, M. E., Bakdounes, L., Koutsikos, K., Felice, D., et al. (2019). Is the aquatic thermal environment a suitable place for providing rehabilitative treatment for person with Parkinson's disease? A retrospective study. Int. J. Biometeorol. 63, 13–18. doi:10.1007/s00484-018-1632-1
Meigal, A. Y., Lupandin, Y. V., and Hänninen, O. (1996a). Head and body positions affect thermoregulatory tonus in deltoid muscles. J. Appl. Physiol. (1985). 80, 1397–1400. doi:10.1152/jappl.1996.80.4.1397
Meigal, A., Gerasimova-Meigal, L., Saenko, I., and Subbotina, N. (2018). Dry immersion as a novel physical therapeutic intervention for rehabilitation of Parkinson’s disease patients: A feasibility study. Phys. Med. Rehab. Kuror. 28, 275–281. doi:10.1055/a-0577-5139
Meigal, A. (2002). Gross and fine neuromuscular performance at cold shivering. Int. J. Circumpolar Health 61, 163–172. doi:10.3402/ijch.v61i2.17449
Meigal, A. (2012). Interplanetary space flight compared with fetal/neonatal motor strategy: Theoretical and practical implications. Pathophysiology 19, 269–276. doi:10.1016/j.pathophys.2012.08.001
Meigal, A., and Lupandin, Y. (2005). Thermoregulation-dependent component" in pathophysiology of motor disorders in Parkinson's disease? Pathophysiology 11 (4), 187–196. doi:10.1016/j.pathophys.2005.02.001
Meigal, A., Pavlova, I., Lupandin, Y., Sokolov, A., Antonen, E., and Sokolov, A. (1995). Thermoregulatory activity of motor units during human development. Arct. Med. Res. 54, 192–200.
Meigal, A. Y., Lupandin, Y. V., and Hänninen, O. (1996b). Influence of cold and hot conditions on postactivation in human skeletal muscles. Pflugers Arch. - Eur. J. Physiol. 432, 121–125. doi:10.1007/s004240050113
Meigal, A. Y., Oksa, J., Gerasimova, L. I., Hohtola, E., Lupandin, Y. V., and Rintamäki, H. (2003). Force control of isometric elbow flexion with visual feedback in cold with and without shivering. Aviat. Space Environ. Med. 74, 816–821.
Meigal, A. Y., Oksa, J., Hohtola, E., Lupandin, Y. V., and Rintamäki, H. (1998). Influence of cold shivering on fine motor control in the upper limb. Acta Physiol. Scand. 163, 41–47. doi:10.1046/j.1365-201x.1998.00333.x
Meigal, A. Y., and Pis’mennyi, K. N. (2009). The influence of whole body heating and cooling on the aftercontraction effect in the upper limb muscles. Hum. Physiol. 35, 51–57. doi:10.1134/S0362119709010095
Meigal, A. Y. (2013). Synergistic action of gravity and temperature on the motor system within the lifespan: A "baby astronaut" hypothesis. Med. Hypotheses 80, 275–283. doi:10.1016/j.mehy.2012.12.004
Meigal, A. Yu., Gerasimova, L. I., and Lupandin, Iu. V. (1999). Proizvol'noe rekrutirovanie dvigatel'nykh edinits v usloviiakh peregevaniia organizma [Voluntary recruitment of the motor units during body overheating]. Fiziol. Chel. 25, 111–116.
Meigal, A. Yu., Gerasimova, L. I., Zolotova, E. V., and Lupandin, Yu.V. (1997). Voluntary recruiting of motor units under conditions of cold shivering. Fiziol. Chel 23, 64–69.
Miroshnichenko, G. G., Meigal, A. Y., Saenko, I. V., Gerasimova-Meigal, L. I., Chernikova, L. A., Subbotina, N. S., et al. (2018). Parameters of surface electromyogram suggest that dry immersion relieves motor symptoms in patients with parkinsonism. Front. Neurosci. 12, 667. doi:10.3389/fnins.2018.00667
Morey-Holton, E. R., and Globus, R. K. (1998). Hindlimb unloading of growing rats: A model for predicting skeletal changes during space flight. Bone 22, 83S–88S. doi:10.1016/s8756-3282(98)00019-2
Morey-Holton, E. R. (2003). “The impact of gravity on life,” in Evolution on planet Earth: The impact of the physical environment. Editors L. Rothschild, and A. Lister (New York, NY: Academic), 143.
Mrówczyński, W., Celichowski, J., Raikova, R., and Krutki, P. (2015). Physiological consequences of doublet discharges on motoneuronal firing and motor unit force. Front. Cell. Neurosci. 9, 81. doi:10.3389/fncel.2015.00081
Mulder, E. R., Gerrits, K. H., Rittweger, J., Felsenberg, D., Stegeman, D. F., and de Haan, A. (2008), Characteristics of fast voluntary and electrically evoked isometric knee extensions during 56 days of bed rest with and without exercise countermeasure, Eur. J. Appl. Physiol. 103, 431–440. doi:10.1007/s00421-008-0724-8
Needle, A. R., Baumeister, J., Kaminski, T. W., Higginson, J. S., Farquhar, W. B., and Swanik, C. B. (2014). Neuromechanical coupling in the regulation of muscle tone and joint stiffness. Scand. J. Med. Sci. Sports 24, 737–748. doi:10.1111/sms.12181
Nomura, T., Kawano, F., Kang, M. S., Lee, J. H., Han, E. Y., Kim, C. K., et al. (2002). Effects of long-term cold exposure on contractile properties in slow- and fast-twitch muscles of rats. Jpn. J. Physiol 52, 85–93. doi:10.2170/jjphysiol.52.85
Novák, P., Zachařová, G., and Soukup, T. (2010). Individual, age and sex differences in fiber type composition of slow and fast muscles of adult lewis rats: Comparison with other rat strains. Physiol. Res. 59, 783–801. doi:10.33549/physiolres.931827
Nybo, L. (2008). Hyperthermia and fatigue. J. Appl. Physiol. (1985). 104, 871–878. doi:10.1152/japplphysiol.00910.2007
O'Neill, D. E., Aubrey, F. K., Zeldin, D. A., Michel, R. N., and Noble, E. G. (2006). Slower skeletal muscle phenotypes are critical for constitutive expression of Hsp70 in overloaded rat plantaris muscle. J. Appl. Physiol. (1985). 100, 981–987. doi:10.1152/japplphysiol.00831.2005
Oishi, Y., Ishihara, A., Yamamoto, H., and Miyamoto, E. (1998). Hindlimb suspension induces the expression of multiple myosin heavy chain isoforms in single fibres of the rat soleus muscle. Acta Physiol. Scand. 162, 127–134. doi:10.1046/j.1365-201X.1998.0284f.x
Paré, G., Trudel, M.-C., Jaana, M., and Kitsiou, S. (2015), Synthesizing information systems knowledge: A typology of literature reviews. Inf. Manag. 52, 183–199. doi:10.1016/j.im.2014.08.008
Périard, J. D., Houtkamp, D., Bright, F., Daanen, H. A. M., Abbiss, C. R., Thompson, K. G., et al. (2019). Hyperoxia enhances self-paced exercise performance to a greater extent in cool than hot conditions. Exp. Physiol. 104, 1398–1407. doi:10.1113/EP087864
Petajan, J. H., and Williams, D. D. (1972). Behavior of single motor units during pre-shivering tone and shivering tremor. Am. J. Phys. Med. 51, 16–22.
Rabinovitch, R., and Vilaro, V. (2010). Structural and functional changes of peripheral muscles in chronic obstructive pulmonary disease patients. Curr. Opin. Pulm. Med. 16, 123–133. doi:10.1097/MCP.0b013e328336438d
Renberg, J., Wiggen, Ø. N., Oksa, J., Dyb, K. B., Reinertsen, R. E., and Roeleveld, K. (2020). The effect of mild whole-body cold stress on isometric force control during hand grip and key pinch tasks. J. Therm. Biol. 89, 102537. doi:10.1016/j.jtherbio.2020.102537
Rendell, R. A., Prout, J., Costello, J. T., Massey, H. C., Tipton, M. J., Young, J. S., et al. (2017). Effects of 10 days of separate heat and hypoxic exposure on heat acclimation and temperate exercise performance. Am. J. Physiology-Regulatory Integr. Comp. Physiology 313, R191–R201. doi:10.1152/ajpregu.00103.2017
Roberts, T. J., Weber, J. M., Hoppeler, H., Weibel, E. R., and Taylor, C. R. (1996). Design of the oxygen and substrate pathways. II. Defining the upper limits of carbohydrate and fat oxidation. J. Exp. Biol. 99, 1651–1658. doi:10.1242/jeb.199.8.1651
Romijn, J. A., Coyle, E. F., Sidossis, L. S., Gastaldelli, A., Horowitz, J. F., Endert, E., et al. (1993). Regulation of endogenous fat and carbohydrate metabolism in relation to exercise intensity and duration. Am. J. Physiology-Endocrinology Metabolism 265, E380–E391. doi:10.1152/ajpendo.1993.265.3.E380
Rossi, B., Siciliano, G., Carboncini, M. C., Manca, M. L., Massetani, R., Viacava, P., et al. (1996). Muscle modifications in Parkinson's disease: Myoelectric manifestations. Electroencephalogr. Clin. Electromyogr. Mot. Control 101, 211–218. doi:10.1016/0924-980x(96)94672-x
Roy, R. R., Baldwin, K. M., and Edgerton, V. R. (1996). Response of the neuromuscular unit to spaceflight: What has been learned from the rat model. Exerc. Sport Sci. Rev. 24, 399–426. doi:10.1249/00003677-199600240-00015
Schloon, H., O'Brien, M. J., Scholten, C. A., and Prechtl, H. F. (1976). Muscle activity and postural behaviour in newborn infants. A polymyographic study. Neuropediatrics 7, 384–415. doi:10.1055/s-0028-1091640
Sekulić, S. R., Lukac, D. D., and Naumović, N. M. (2005). The fetus cannot exercise like an astronaut: Gravity loading is necessary for the physiological development during second half of pregnancy. Med. Hypotheses 64, 221–228. doi:10.1016/j.mehy.2004.08.012
Shenkman, B. S., Kozlovskaya, I. B., Nemirovskaya, T. L., and Tcheglova, I. A. (1997). Human muscle atrophy in supportlessness: Effects of short-term exposure to dry immersion. J. Gravit. Physiol. 4, P137–P138.
Sideris, I. G., and Nicolaides, K. H. (1990). Amniotic fluid pressure during pregnancy. Fetal diagn. Ther. 5, 104–108. doi:10.1159/000263555
Smith, C. V., Satt, B., Phelan, J. P., and Paul, R. H. (1990). Intrauterine sound levels: Intrapartum assessment with an intrauterine microphone. Am. J. Perinatol. 7, 312–315. doi:10.1055/s-2007-999511
Sorokina, L. V., Lupandin, I., and Vlasova, L. P. (1984). Thermoregulatory activity of the motor neuron pool in the rat adapted to cold and hypoxia. Fiziol. Zh. SSSR Im. I. M. Sechenova 70, 75–80.
Sorokina, L. V., and Medvedev, N. V. (1986). [Thermoregulatory activity of the motor units of skeletal muscles in the pigeon]. Fiziol. Zh. SSSR Im. I. M. Sechenova 72, 1571–1574.
Stanojevic, M., Kurjak, A., Salihagić-Kadić, A., Vasilj, O., Miskovic, B., Shaddad, A. N., et al. (2011). Neurobehavioral continuity from fetus to neonate. J. Perinat. Med. 39, 171–177. doi:10.1515/jpm.2011.004
Stanojevic, M., Zaputovic, S., and Bosnjak, A. P. (2012). Continuity between fetal and neonatal neurobehavior. Semin. Fetal Neonatal Med. 17, 324–329. doi:10.1016/j.siny.2012.06.006
Sugawara, J., and Tomoto, T. (2020). Effects of short-term warm water immersion on cardiac baroreflex sensitivity in healthy men. J. Physiol. Sci. 70 (1), 34. doi:10.1186/s12576-020-00762-1
Suzuki, J., Gao, M., Ohinata, H., Kuroshima, A., and Koyama, T. (1997). Chronic cold exposure stimulates microvascular remodeling preferentially in oxidative muscles in rats. Jpn. J. Physiol. 47, 513–520. doi:10.2170/jjphysiol.47.513
Tambovtseva, R. V., and Kornienko, I. A. (1986b). Development of various types of muscle fibers in the quadriceps femoris and the soleus during human ontogenesis. Arkh. Anat. Gistol. Embriol. 91, 96–99.
Tambovtseva, R. V., and Kornienko, I. A. (1986a). Growth of different types of muscle fibers of the soleus muscle during postnatal ontogeny in the rat. Arkh. Anat. Gistol. Embriol. 90, 77–81.
Tattersall, G. J., and Milsom, W. K. (2009). Hypoxia reduces the hypothalamic thermogenic threshold and thermosensitivity. J. Physiol. 587, 5259–5274. doi:10.1113/jphysiol.2009.175828
Thompson, W. J., Sutton, L. A., and Riley, D. A. (1984). Fibre type composition of single motor units during synapse elimination in neonatal rat soleus muscle. Nature 309, 709–711. doi:10.1038/309709a0
Tipton, M. J., Franks, G. M., Meneilly, G. S., and Mekjavic, I. B. (1997). Substrate utilisation during exercise and shivering. Eur. J. Appl. Physiol. 76, 103–108. doi:10.1007/s004210050220
Todd, G., Butler, J. E., Taylor, J. L., and Gandevia, S. C. (2005). Hyperthermia: A failure of the motor cortex and the muscle. J. Physiol. 563, 621–631. doi:10.1113/jphysiol.2004.077115
Tomilovskaya, E., Shigueva, T., Sayenko, D., Rukavishnikov, I., and Kozlovskaya, I. (2019). Dry immersion as a ground-based model of microgravity physiological effects. Front. Physiol. 10, 284. doi:10.3389/fphys.2019.00284
Trappe, S., Trappe, T., Gallagher, P., Harber, M., Alkner, B., and Tesch, P. (2004). Human single muscle fibre function with 84 day bed-rest and resistance exercise. J. Physiol. 557, 501–513. doi:10.1113/jphysiol.2004.062166
Tucker, R., Rauch, L., Harley, Y. X., and Noakes, T. D. (2004). Impaired exercise performance in the heat is associated with an anticipatory reduction in skeletal muscle recruitment. Pflugers Arch. 448, 422–430. doi:10.1007/s00424-004-1267-4
Vallerand, A. L., and Jacobs, I. (1990). Influence of cold exposure on plasma triglyceride clearance in humans. Metabolism 39, 1211–1218. doi:10.1016/0026-0495(90)90097-v
van Loon, L. J., Greenhaff, P. L., Constantin-Teodosiu, D., Saris, W. H., and Wagenmakers, A. J. (2001). The effects of increasing exercise intensity on muscle fuel utilisation in humans. J. Physiology 536, 295–304. doi:10.1111/j.1469-7793.2001.00295.x
Verbruggen, S. W., Kainz, B., Shelmerdine, S. C., Hajnal, J. V., Rutherford, M. A., Arthurs, O. J., et al. (2018). Stresses and strains on the human fetal skeleton during development. J. R. Soc. Interface 15 (138), 20170593. doi:10.1098/rsif.2017.0593
Walker, D., Grimwade, J., and Wood, C. (1971). Intrauterine noise: A component of the fetal environment. Am. J. Obstet. Gynecol. 109, 91–95. doi:10.1016/0002-9378(71)90840-4
Wang, H., Liu, W., Yang, D. F., Zhao, X. L., Long, C. L., Yin, Z. Y., et al. (2012). The acclimatization to extreme environments and its physiological mechanisms. Zhongguo Ying Yong Sheng Li Xue Za Zhi 28, 494–503.
Ward, A. B. (2000). Editorial. Assessment of muscle tone. Age Ageing 29, 385–386. doi:10.1093/ageing/29.5.385
Weber, R. E., and Campbell, K. L. (2011). Temperature dependence of haemoglobin-oxygen affinity in heterothermic vertebrates: Mechanisms and biological significance. Acta Physiol. (Oxf). 202, 549–562. doi:10.1111/j.1748-1716.2010.02204.x
Westerga, J., and Gramsbergen, A. (1994). Development of the EMG of the soleus muscle in the rat. Dev. Brain Res. 80, 233–243. doi:10.1016/0165-3806(94)90108-2
White, A. C., Salgado, R. M., Schneider, S., Loeppky, J. A., Astorino, T. A., and Mermier, C. M. (2014). Does heat acclimation improve exercise capacity at altitude? A cross-tolerance model. Int. J. Sports Med. 35, 975–981. doi:10.1055/s-0034-1368724
Wood, C. (1970). Weightlessness: Its implications for the human fetus. BJOG:An. Int. J. O&G. 77, 333–336. doi:10.1111/j.1471-0528.1970.tb03528.x
Xu, X., Tikuisis, P., Gonzalez, R., and Giesbrecht, G. (2005). Thermoregulatory model for prediction of long-term cold exposure. Comput. Biol. Med. 35, 287–298. doi:10.1016/j.compbiomed.2004.01.004
Yudin, M. H., Asztalos, E. V., Jefferies, A., and Barrett, J. F. (2001). The management and outcome of higher order multifetal pregnancies: Obstetric, neonatal and follow-up data. twin Res. 4, 4–11. doi:10.1375/1369052012083
Keywords: temperature, gravity, microgravity, skeletal muscle, muscle tone, neuromuscular system, postnatal period
Citation: Meigal A and Gerasimova-Meigal L (2022) Cold for gravity, heat for microgravity: A critical analysis of the “Baby Astronaut” concept. Front. Space Technol. 3:981668. doi: 10.3389/frspt.2022.981668
Received: 29 June 2022; Accepted: 02 November 2022;
Published: 22 November 2022.
Edited by:
Jean-Philippe Hainaut, Université de Lorraine, FranceReviewed by:
Slobodan Sekulic, University of Novi Sad,SerbiaTimo Frett, German Aerospace Center (DLR), Germany
Copyright © 2022 Meigal and Gerasimova-Meigal. This is an open-access article distributed under the terms of the Creative Commons Attribution License (CC BY). The use, distribution or reproduction in other forums is permitted, provided the original author(s) and the copyright owner(s) are credited and that the original publication in this journal is cited, in accordance with accepted academic practice. No use, distribution or reproduction is permitted which does not comply with these terms.
*Correspondence: Alexander Meigal, bWVpZ2FsQHBldHJzdS5ydQ==