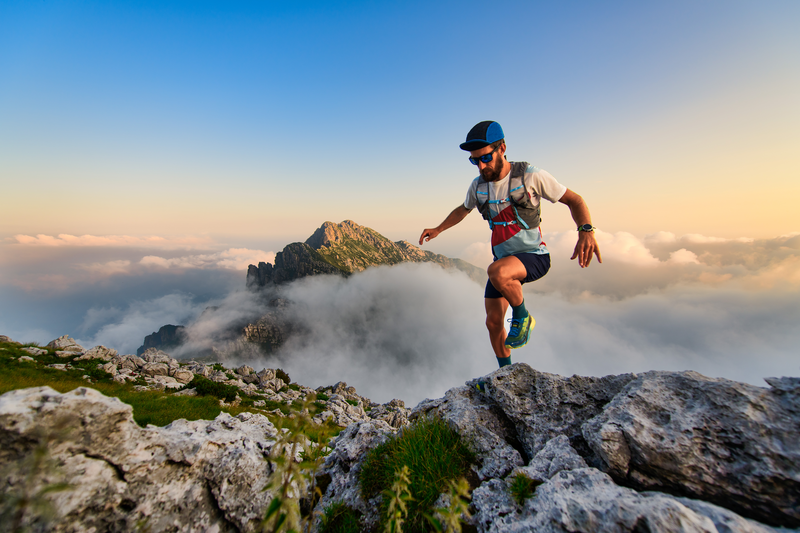
94% of researchers rate our articles as excellent or good
Learn more about the work of our research integrity team to safeguard the quality of each article we publish.
Find out more
EDITORIAL article
Front. Space Technol. , 26 October 2022
Sec. Space Propulsion
Volume 3 - 2022 | https://doi.org/10.3389/frspt.2022.1063163
This article is part of the Research Topic Astrodynamics, Guidance, Navigation and Control (GNC) in Chaotic Multi-Body Environments View all 5 articles
Editorial on the Research Topic
Astrodynamics, guidance, navigation and control (GNC) in chaotic multi-body environments
Modern space missions are frequently targeted towards new and unexplored regions of space, such as the region between the Earth and the Moon, which is denoted as the Cislunar space (NASA, 2020; International Space Exploration Coordination Group – ISECG, 2018); binary asteroid systems (Rivkin et al., 2021); comets and other irregularly shaped celestial objects; satellites of other Solar system planets. In all of these mission scenarios, the spacecraft dynamics is governed by an intriguing, yet complex and chaotic dynamical environment that is driven by the presence of multiple and/or non-spherical massive bodies. The gravitational influence of these objects shall be addressed with methods and techniques that are different from the standard Keplerian tools available in the classic Two-Body Problem. In recent years, the space community has shown a renovated scientific and technological interest in mastering the multi-body non-Keplerian astrodynamics for practical applications. Immediately, new technological and engineering challenges emerged in order to cope with this uninvestigated portion of outer space. In particular, the Guidance, Navigation and Control (GNC) and the Propulsive subsystems developments have been strongly supportive of this endeavor.
Future lunar and solar system exploration missions will be supported by a complex infrastructure of space systems orbiting in multi-body regions and influenced by chaotic dynamics (Whitley and Martinez, 2016). They will guarantee continuous human and robotic presence well beyond Earth orbits, broadcast of communication relay networks, Solar system exploration and advanced navigation systems. This new space race demands the upgrade of consolidated space technologies to handle the unique features of multi-body environments, by exploiting the chaotic dynamics of the n-body problem and its different flavors (Howell, 2001; Belbruno, 2018). For these reasons, this Research Topic is dedicated to articles focused on astrodynamics, guidance, navigation, and control specifically leveraging multi-body dynamics, with the purpose to highlight the most innovative methods, tools, technologies and solutions about trajectory design and spacecraft control in chaotic multi-body environments, such as the Earth-Moon system. Indeed, the latter is one of the most relevant under an applicative point of view for near-future missions, and most of the submitted research works took this setting into account. The topic was open to works about orbital and rotational dynamics, GNC of spacecraft, formations, and constellations. Moreover, contributions discussing system-specific aspects, such as instrumentation, GNC architectures and computing requirements were also encouraged (or welcomed).
One of the most complex challenges in future spacecraft missions is the autonomous navigation in deep space. In these regards, the article of Turan et al. proposes a method for the “Autonomous Crosslink Radionavigation for a Lunar CubeSat Mission,” which is based on crosslink radiometric measurements, and it is based on the Linked Autonomous Interplanetary Satellite Orbit Navigation (LiAISON) method (Hill et al., 2006). In this case, the non-Keplerian cislunar environment is beneficial to achieve the ambitious goal owing to the asymmetric gravity field. The analysis is settled on an Earth-Moon L2 Halo orbit, with an inter-satellite link between a lunar elliptical frozen orbiter. The authors prove that the true state can be estimated on the Lagrangian point orbit with an error in the order of 500 m for position, and 2 mm/s for velocity, respectively.
Formation flying in space is another interesting area of active research, which is addressed by Bando et al. in their work on “Formation Flying Along Libration Point Orbits Using Chattering Attenuation Sliding Mode Control.” The research presents a control law to achieve accurate formation flying in cislunar space, which exploits the mathematical methods that are closely related with the chaotic multi-body environment analyses. Indeed, the authors use the eigenstructure of the linearized flow around a libration point of the Earth-Moon system to implement a fuel-efficient formation flying controller based on a chattering attenuation sliding mode control law. Their results show good performance and robustness, even in the presence of unmodelled non-linearities of a simplified Circular Restricted 3-body Problem (CR3BP). Moreover, they are feasible with existing low-thrust propulsion capabilities (Rayman et al., 2000; Russell and Raymond, 2012), showing maximum accelerations in the order of 10−6–10−7 m/s2.
One of the most fascinating problems in chaotic multi-body environments is the optimization of orbital transfers. Many consolidated tools exist for Keplerian orbits, but the research area is very prolific in the case of non-Keplerian settings. Martens and Bucci proposes a method exploiting “Double Tisserand Graphs for Low Energy Lunar Transfer Design.” Indeed, despite Tisserand graphs are a widespread tool for interplanetary trajectory design, they do not include the perturbation of bodies other than the fly-by ones. The authors propose a novel double Tisserand Graph, where the level lines of both the Earth-Moon and the Sun-Earth systems are superimposed, portraying the complex 4-body dynamics into a single plot. The results discussed in this paper show how this method can be applied to efficiently design Weak Stability Boundary (WSB) transfers, lunar resonance transfers or lunar fly-bys. The resulting ∆v-s are in the order of few meters per second, requiring an accurate thrust control (Parrish et al., 2020).
The cislunar space will be deeply exploited to host complex systems and infrastructures. Thus, the on-board assembly of such systems will require a sound improvement of proximity operations capabilities. In these regards, the work of Innocenti et al. presents a “Dynamics and Control Analysis during Rendezvous in non-Keplerian Earth-Moon Orbits.” The authors describe the development of a framework to deal with rendezvous operations in cislunar space. Their results address both the dynamics and the system related issues, such as the influence of actuators and sensors on the GNC performance, and the impact of failures on the safety of the mission. A close-range closed-loop guidance method is shown and discussed with respect to other existing literature works, which already addressed the topic (Bucci and Colagrossi, 2018; Colagrossi et al., 2021). The required control effort demands for a great throttleability of the propulsion subsystem, and it shall be addressed at system level during the design of future cislunar space missions.
In summary, the articles in this Research Topic provide an overview of significant methods that are relevant to the field of modern astrodynamics and GNC in chaotic multi-body environments, with particular focus on the cislunar space. The propulsion systems used in this context will have to take particular care to the small control actions and to the accuracy of the thrust output. As evident from the results discussed in this Research Topic, accurate low-thrust propulsion systems will be necessary to successfully explore the Earth-Moon system, and they will play a key role in the future robotic and human expeditions in the solar system (Crusan et al., 2018).
AC organized and wrote the manuscript. All authors listed have made a substantial, direct, and intellectual contribution to the work and approved it for publication.
The authors declare that the research was conducted in the absence of any commercial or financial relationships that could be construed as a potential conflict of interest.
All claims expressed in this article are solely those of the authors and do not necessarily represent those of their affiliated organizations, or those of the publisher, the editors and the reviewers. Any product that may be evaluated in this article, or claim that may be made by its manufacturer, is not guaranteed or endorsed by the publisher.
Belbruno, E. (2018). “Capture dynamics and chaotic motions in celestial mechanics,” in Capture dynamics and chaotic motions in celestial mechanics (Princeton, New Jersey, United States: Princeton University Press).
Bucci, L., and Colagrossi, A. (2018). Rendezvous in lunar near rectilinear Halo orbits. Adv. Astronaut. Sci. Technol. 1, 39–43. doi:10.1007/s42423-018-0012-6s42423-018-0012-6
Colagrossi, A., Pesce, V., Bucci, L., Colombi, F., and Lavagna, M. (2021). Guidance, navigation, and control for 6DOF rendezvous in Cislunar multi-body environment. Aerosp. Sci. Technol. 114, 106751. doi:10.1016/j.ast.2021.106751
Crusan, J. C., Smith, R. M., Craig, D. A., Caram, J. M., Guidi, J., Gates, M., et al. (2018). “Deep space gateway concept: Extending human presence into cislunar space,” in 2018 IEEE Aerospace Conference, Big Sky, MT, 03-10 March 2018 (New York, NY: IEEE), 1–10.
Hill, K., Born, G. H., and Lo, M. W. (2006). “Linked, autonomous, interplanetary satellite orbit navigation (LiAISON) in lunar Halo orbits,” in 2005 AAS/AIAA Astrodynamics Specialists Conference, Lake Tahoe, California, USA, 7–11 August, 2005.
Howell, K. (2001). Families of orbits in the vicinity of the collinear libration points. J. Astronaut. Sci. 49, 107–125. doi:10.1007/BF03546339
International Space Exploration Coordination Group – ISECG (2018). The global exploration roadmap. Tech. rep., ISECG. Washington, DC: National Aeronautics and Space Administration Headquarters.
NASA (2020). NASA’s lunar exploration program overview. Washington, DC: National Aeronautics and Space Administration Headquarters.
Parrish, N. L., Kayser, E., Udupa, S., Parker, J. S., Cheetham, B. W., and Davis, D. C. (2020). “Ballistic lunar transfers to near rectilinear Halo orbit: Operational considerations,” in AIAA scitech 2020 forum, Orlando, FL, January 6–10, 2020, 1466.
Rayman, M. D., Varghese, P., Lehman, D. H., and Livesay, L. L. (2000). Results from the Deep Space 1 technology validation mission. Acta Astronaut. 47, 475–487. doi:10.1016/s0094-5765(00)00087-4
Rivkin, A. S., Chabot, N. L., Stickle, A. M., Thomas, C. A., Richardson, D. C., Barnouin, O., et al. (2021). The double asteroid redirection test (DART): Planetary defense investigations and requirements. Planet. Sci. J. 25, 173. doi:10.3847/psj/ac063e
Russell, C., and Raymond, C. (2012). The dawn mission to minor planets 4 vesta and 1 ceres. New York, NY: Springer Science & Business Media.
Keywords: astrodynamics, multi-body chaotic environment, non-Keplerian dynamics, cislunar space, trajectory design, GNC
Citation: Colagrossi A, Lizy-Destrez S, Baresi N, Masdemont J and Bucci L (2022) Editorial: Astrodynamics, guidance, navigation and control in chaotic multi-body environments. Front. Space Technol. 3:1063163. doi: 10.3389/frspt.2022.1063163
Received: 06 October 2022; Accepted: 13 October 2022;
Published: 26 October 2022.
Edited and reviewed by:
Ioannis G. Mikellides, NASA Jet Propulsion Laboratory (JPL), United StatesCopyright © 2022 Colagrossi, Lizy-Destrez, Baresi, Masdemont and Bucci. This is an open-access article distributed under the terms of the Creative Commons Attribution License (CC BY). The use, distribution or reproduction in other forums is permitted, provided the original author(s) and the copyright owner(s) are credited and that the original publication in this journal is cited, in accordance with accepted academic practice. No use, distribution or reproduction is permitted which does not comply with these terms.
*Correspondence: Andrea Colagrossi, YW5kcmVhLmNvbGFncm9zc2lAcG9saW1pLml0
Disclaimer: All claims expressed in this article are solely those of the authors and do not necessarily represent those of their affiliated organizations, or those of the publisher, the editors and the reviewers. Any product that may be evaluated in this article or claim that may be made by its manufacturer is not guaranteed or endorsed by the publisher.
Research integrity at Frontiers
Learn more about the work of our research integrity team to safeguard the quality of each article we publish.