- 1Institute of Applied Ecology, Chinese Academy of Sciences, Shenyang, China
- 2Weifang Academy of Modern Agriculture and Ecological Environment, Weifang, China
- 3Key Laboratory of Stable Isotope Techniques and Applications, Shenyang, China
- 4Shenyang Agro-ecosystem Experimental Station, Chinese Academy of Sciences, Shenyang, China
- 5School of Landscape Architecture, Liaoning Agricultural Vocational and Technical College, Yingkou, China
There is limited knowledge regarding the impact of nitrification inhibitors (NIs) and straw application on fertilizer N retention and in-season release. We conducted a trial to study the transformation of 15N-labeled urea in soils during the growing season of maize. To facilitate multiple destructive samplings throughout the season, we utilized a larger plot (25 m2) and a lower abundance 15N-fertilizer (1.193%) than usual. Soil extractable mineral N, mineral fixed ammonium, and organic N (ON) recovered 20 ± 21% (mean ± standard deviation), 6 ± 5%, and 25 ± 6% of the applied fertilizer N across three sampling stages of the growing season. On average, the bioavailability of fertilizer N in extractable mineral form was four times higher than that of mineral fixed ammonium. In contrast, fertilizer-derived ON represented a relatively stable N pool, maintaining high content throughout the growing period and becoming the major form (82%–93%) in the pool of total soil 15N at the physiological maturity stage of maize. Moreover, the co-application of nitrapyrin (a type of NI) significantly promoted fertilizer N storage in the ON form while the effect of straw was not significant. In conclusion, the NI-induced promotion of fertilizer-derived ON likely plays a critical role in storing fertilizer N for subsequent cultivations, rather than providing N nutrients for crop uptake during the current season.
1 Introduction
Fertilizer nitrogen (N) applied to agricultural soil can be rapidly converted into mineral N forms (ammonium and nitrate), which increases the soil N supply to crops and contributes to yield improvements (1). However, the prematurely accumulated mineral N in the topsoil is easily lost to the environment, failing to synchronize the soil N supply with root N absorption (2, 3). Two measures to conserve fertilizer N in soils are the application of nitrification inhibitors (NIs) and the incorporation of straw. These strategies aim to transform fertilizer-derived mineral N into stable N pools during the early stage of crop growth, ensuring a gradual release that meets the crop’s N demand during the middle and late stages (4, 5). However, due to a lack of experimental methods, it remains unclear whether and to what extent these measures can enhance the release of early-conserved fertilizer N during the middle and late stages of crop growth (6).
The 15N tracer technique is the primary tool used to trace the transformation and fate of fertilizer N in soil-crop systems. Over the last four decades, many studies utilizing this technique have explored the effects of various management practices on fertilizer N fate (7, 8). However, due to limitations in the detection accuracy of 15N, most experiments required the use of high abundance 15N-fertilizer, which increased costs. To reduce expenses, researchers have limited the size of plots (e.g., <2 m2). As a result, most sampling occurred only at harvest, as small plot sizes restricted the frequency of destructive sampling (9). However, with advancements in testing technology over the past decade, the detection accuracy of 15N has significantly improved (10, 11), allowing for the use of lower abundance 15N-fertilizer and enabling larger plot sizes.
We conducted a tracer study using lower abundance 15N-fertilizer and a larger plot size to monitor the seasonal dynamics of fertilizer-derived N in soil under different nutrient management practices (i.e., NI and straw application). Our goal was to compare the bioavailability of various soil N constituents to root uptake during one growing season of maize. We hypothesize that these practices can enhance the transformation of fertilizer N into fixed ammonium and organic N pools, which can be released for crop absorption and utilization during the middle or late stages of maize growth.
2 Materials and methods
2.1 Experimental design
The field 15N tracer trial was conducted in a suburban area of Gongzhuling City, Northeast China (43°30’N, 124°48’E). The site features a semi-humid continental monsoon climate. From 2011 to 2020, the average annual precipitation and temperature at the study site were 666 mm and 6.8°C, respectively. Maize, the region’s primary cereal crop, has been cultivated annually for decades without any rotation with other crops. The soil in the area is classified as a Mollisol according to the US soil taxonomy and is typical black soil in China. Two days prior to the trial, soil samples were collected from a depth of 0-20 cm to assess soil characteristics. The background information of the soil: pH, 6.19; total carbon, 19.1 g/kg; total N, 1.52 g/kg; sand, 15%; silt, 52%; clay, 33%.
Five treatments were established: 1) 100%N, 200 kg urea N/ha; 2) 100%N+S (straw), 200 kg urea N/ha and 2400 kg dry straw/ha; 3) 80%N, 160 kg urea N/ha; 4) 80%N+NI, 160 kg urea N/ha and nitrification inhibitor (Nitrapyrin, C6H3Cl4N, 1.6 kg/ha); 5) 80%N+NI+S, 160 kg urea N/ha, 1.6 kg Nitrapyrin/ha and 2400 kg dry straw/ha. Each treatment consisted of three plots (replicates). To allow for multiple samplings within the growing season, each plot had an area of 25 m2, and all areas was labeled by 15N fertilizer. We arranged a trial for ridge-furrow cultivation similar to the management practices used by local farmers, as described by Quan et al. (9). Specifically, all fertilizers (including 15N-urea), NI, and maize straws were placed on a ridge 5 cm below the ground before sowing, all on the same day (May 6). No topdressing was performed during the growing period of maize. The abundance of applied 15N-urea was 1.193%. After fertilization, a hand-powered hole-drilling machine was used for sowing on the ridge. The local farmers’ agronomic practices were followed during the maize growth period.
The maize variety used in this study was Xianyu 335, with a planting density of 70000 plants per hectare. The row and plant spacings were set at 60 cm and 20 cm, respectively. Other agronomic practices during the maize growth period adhered to local farmers’ procedures. Irrigation and fertilization were not conducted during the growth period. A nearby meteorological station recorded daily mean air temperature and precipitation throughout the trial period (Figure 1).
2.2 Soil and plant sampling
Soil samples were collected three times after fertilization and sowing, on days 42, 82, and 152 (Figure 1). At these three time points, maize growth stages were recorded as V6, VT, and R6, corresponding to the six-leaf, tasseling, and physiological maturity stages, respectively. To account for the uneven distribution of fertilizers in the soil, we used a frame that covered both the ridge and furrow to assist with sampling. In all cases, soil within the frame was excavated from a depth of 0–10 cm, mixed thoroughly by hand on plastic sheeting, and a portion was set aside for sampling.
Plant samples were collected exclusively during the maize harvest. All aboveground plant material within the plot was harvested to quantify the mass of both the straw and maize cobs. Additionally, three maize plants were randomly selected and divided into four parts: stem, leaf, cob, and grain. Each part was weighed separately to determine their relative proportions. Fresh samples were chopped into pieces smaller than 3 cm. Portions of these samples were transported to the laboratory, where they were dried in an oven at 70°C. This process determined their water content and facilitated the calculation of the dry weight of each part.
2.3 Chemical and isotope analysis
Subsamples of fresh soil were extracted with 2 M potassium chloride, shaken for one hour, and then filtered through filter paper to measure the mineral N (NH4+-N, NO3−-N) concentrations. Subsequently, 15N-NH4+ and 15N-NO3− abundances in the extracts were measured using the hypobromite oxidation/hydroxylamine reduction method (10) and the modified azide method (11). Dry soil and plant samples were pulverized and finely ground to analyze the total nitrogen (TN) concentration and 15N abundance using an elemental analyzer and a stable isotope ratio mass spectrometer (EA-IRMS). Mineral fixed NH4+-N concentration and its 15N abundance in residual soils after extraction were determined by the EA-IRMS after organic N was removed by excessive alkaline KOBr solution (12). The fertilizer-derived organic N (ON), mainly soil microbial biomass or necromass N or other non-biologically synthesized organic matter, was calculated by subtracting fertilizer-derived NH4+-N, NO3−-N and mineral fixed NH4+-N from soil fertilizer-derived TN based on the mass-balance principle (13). In fertilizer-derived ON pool, extractable organic N (EON) was considered ignorable owing to its low concentration and 15N recovery in our previous studies (14).
2.4 Statistical analysis
Statistical analysis in this study was performed using SPSS 13.0 (SPSS Inc., Chicago, IL, USA). One-way analysis of variance was used to test differences among the five treatments, and multiple comparisons were performed using the least significant difference (LSD) test with a 95% confidence interval.
3 Results and discussion
Over the course of growth from the V6 to R6 stage, the 15N recoveries of the extractable ammonium (NH4+) and nitrate (NO3−) pools decreased from 21%–52% to 0.1%–4% and from 9%–18% to 1%–4%, respectively, primarily due to soil turnover, crop uptake, and environmental losses (Figure 2). Compared with conventional fertilization (100%N and 80%N), the application of NI (80%N+NI and 80%N+NI+S) slowed the nitrification process, increased the residence time of fertilizer-derived extractable NH4+, enhanced root- or soil microbe-mediated N immobilization, and decreased the accumulation of highly mobile NO3− (15, 16). As a result, NI application can encourage fertilizer N retention and may enhance subsequent soil N supply during the middle and late growth stages (17). However, this study suggests that the remineralization ability of newly formed fertilizer-derived organic N (ON) was lower than expected. For treatments without NI addition, 15N recoveries as soil ON decreased by 8%–27% as the growth stage progressed from V6 to R6 (P>0.05). In contrast, for treatments with NI addition, 15N recoveries as soil ON were maintained and even increased at the VT and R6 stages of growth (Figure 2). The stable 15N recovery in fertilizer-derived ON pool is likely due to its resistant components, as well as the physicochemical protection by minerals and aggregates (18).
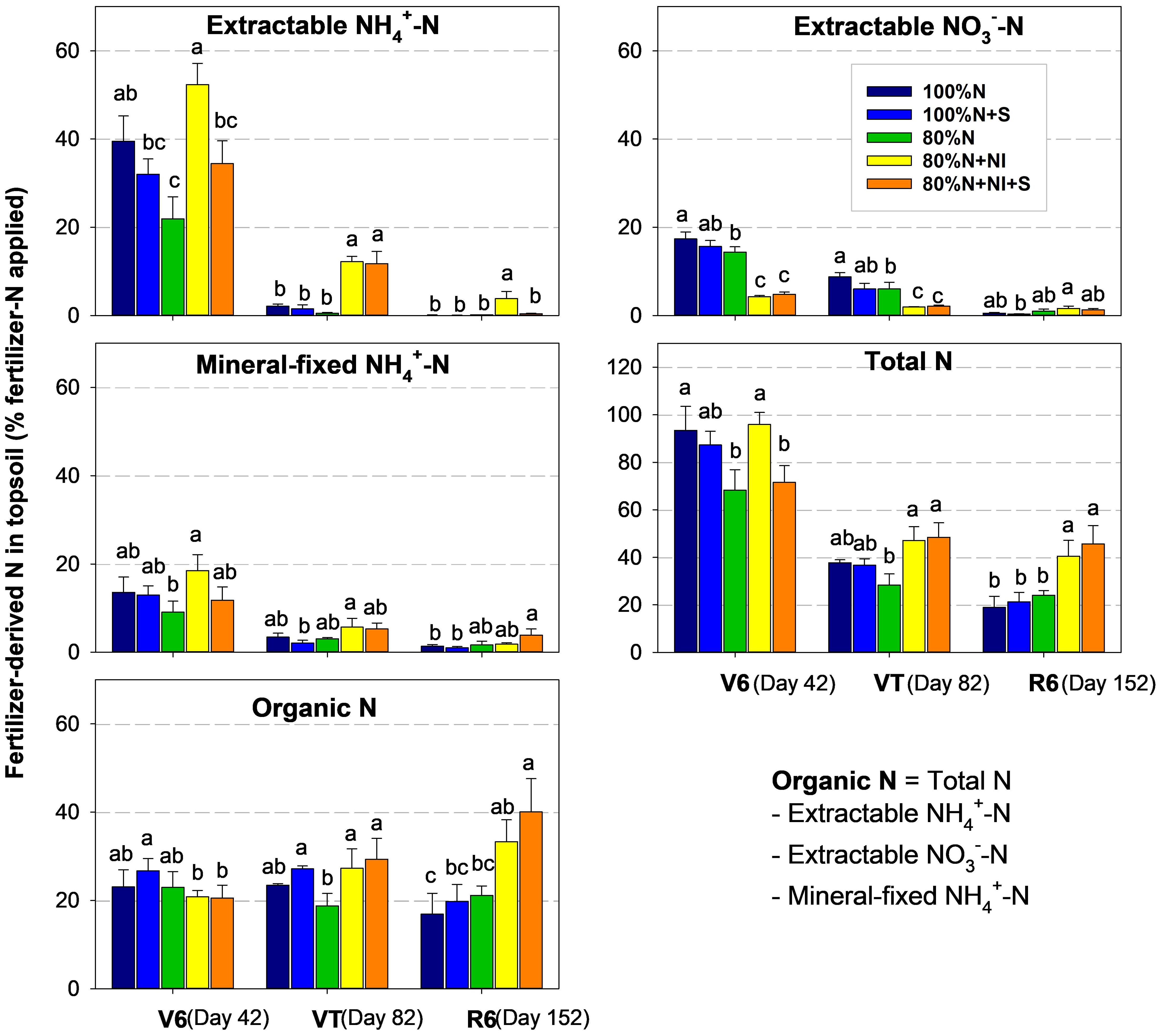
Figure 2. Fertilizer N components in topsoil (0–10 cm) during the maize growing season. The y-axis represents the proportion of different soil N components derived from the applied fertilizer N. Organic N (ON) was calculated as the difference between total N and mineral N (extractable NH4+-N + extractable NO3−-N + mineral fixed NH4+-N). The recovery of 15N was determined using the formula: 15N recovery = (N content in a soil component × %N)/fertilizer N rate. The %N indicates the proportion of fertilizer N in the corresponding soil N component and was calculated based on the 15N abundances of both the soil and the fertilizer. Specifically, %N = (soil 15N abundance in the 15N-fertilized plot - 0.3663%)/(fertilizer 15N abundance - 0.3663%). The fertilizer 15N abundance was 1.193%. Error bars represent standard errors (n = 3). Different lowercase letters above the columns in the same cluster, within the same sampling stage indicate significant differences (LSD, P < 0.05).
When fertilizer N was converted to ON through microbial immobilization or anabolism, its availability decreased not only in the current season but also in subsequent seasons (3, 19). For example, Smith and Chalk (8) conducted a global meta-analysis using published data from in situ 15N tracer trials and found that the legacy N utilization by crops decreased significantly compared to the first season. The low legacy N utilization indicates that the newly retained ON may require a long period before it is released back into the soil, and the related mechanism remains to be further explored. In recent years, an increasing number of studies suggest that soil N assimilated by microorganisms is an important process of soil N stabilization (20, 21). The “microbial carbon pump” theory of Liang et al. (22), and the “mineral carbon pump” theory of Xiao et al. (23) both provide explanations for this phenomenon.
Since our experiment was conducted over only one season, the responses of grain yield and maize N uptake to NI and straw application were not significant. However, the application of NI (80%N+NI and 80%N+NI+S) significantly reduced the proportion of maize N derived from fertilizer (%Ndff) and the recovery of 15N in aboveground biomass (Figure 3). Compared to treatments without NI addition, soil 15N-nitrate availability decreased significantly in the NI addition treatments (Figure 2). Considering that maize is a nitrate-preferring plant, the decreases in %Ndff and crop 15N recovery under NI treatments are understandable. These results are consistent with the findings of Ma et al. (21) from their pot experiments, which indicated that the addition of NI promoted the retention of fertilizer-derived N and its subsequent release, primarily as mineral fixed NH4+-N rather than ON, regardless of whether crop straw was added. Under field conditions, even without the addition of exogenous organic material, crop roots can also provide carbon sources for microbial N immobilization (24). A previous study confirmed our results, finding that the %Ndff of crop N uptake decreased with NI addition, while the fertilizer-derived hydrolyzable N in the soil increased (25). Therefore, we speculate the function of NI application likely shifts from solely regulating fertilizer N release to simultaneously promoting microbial N immobilization, thereby increasing the residence time of fertilizer N and reducing its losses (26).
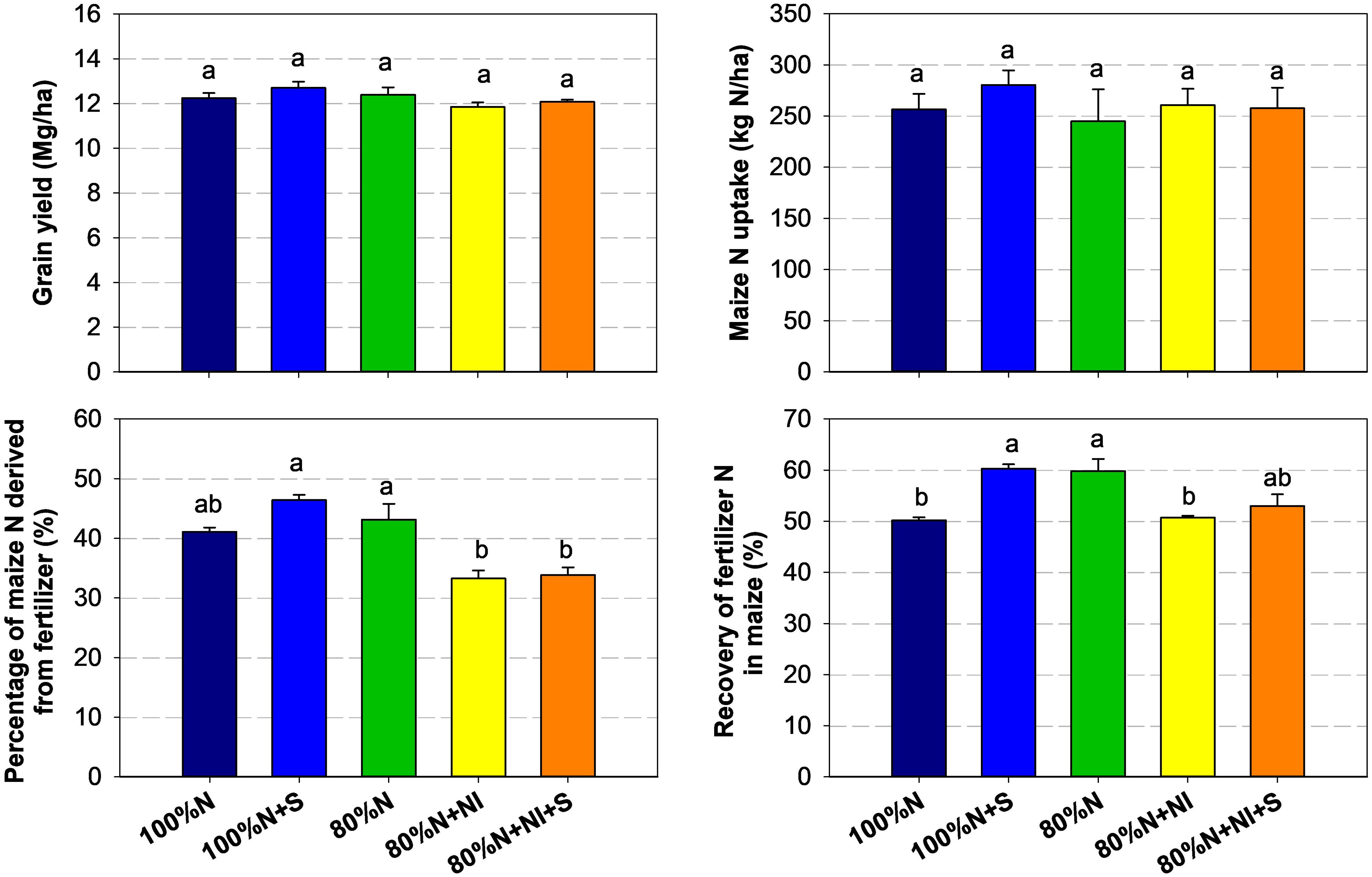
Figure 3. Grain yield, maize N uptake, proportions of maize N derived from fertilizer (N%), and fertilizer 15N recovery at R6 stage of the maize growing season. Maize N uptake includes four organs: stem, leaf, cob, and grain. The recovery of 15N was determined using the formula: 15N recovery = (N content in a plant organ × %N)/fertilizer N rate. The %N indicates the proportion of fertilizer N in the corresponding organ and was calculated based on the 15N abundances of both the plant and the fertilizer. Specifically, %N = (plant 15N abundance in the 15N-fertilized plot - 0.3663%)/(fertilizer 15N abundance - 0.3663%). The fertilizer 15N abundance was 1.193%. Error bars in the figure are standard errors (n = 3). Different lowercase letters above the columns indicate significant differences (LSD, P < 0.05).
In our study, the recovery of 15N in the TN pool decreased with the growth stage due to root N uptake and gaseous or hydrologic losses (Figure 2). The two mineral components—fertilizer-derived extractable mineral N and fertilizer-derived mineral fixed NH4+-N, exhibited strong positive relationships with fertilizer-derived TN during the V6 and VT stages, with slopes of 0.81 and 0.20, respectively (Figure 4). This suggests that mineral fixed NH4+ plays a critical role in buffering and supplying fertilizer N during the maize growing season (17). However, its bioavailability was only approximately a quarter of that of the extractable mineral 15N, although it was significantly higher than 15N-ON during the maize growing season.
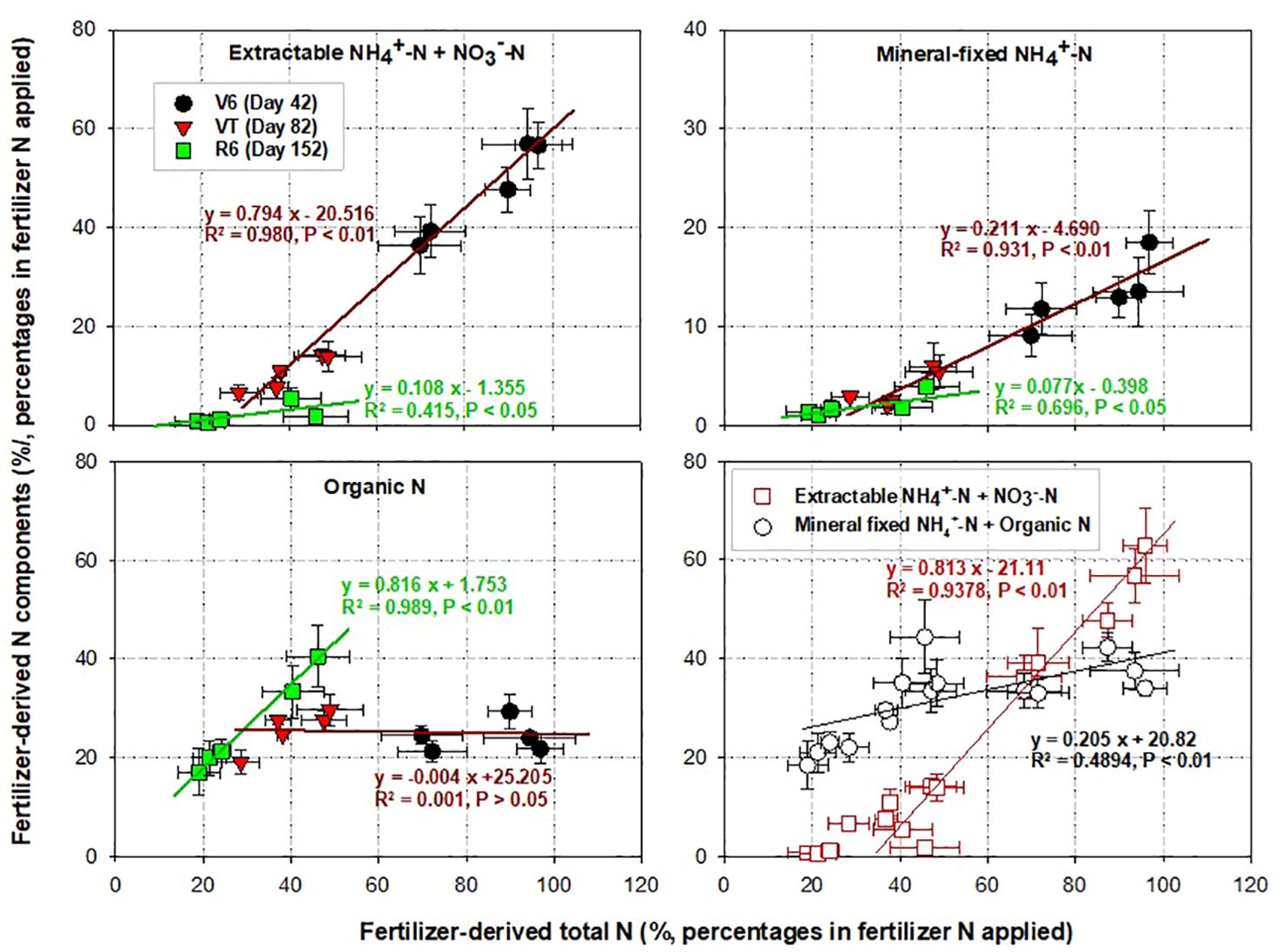
Figure 4. Pearson correlation analysis showing the relationships between fertilizer-derived TN and its components: extractable mineral N (NH4+-N + NO3−-N), mineral fixed NH4+-N, and organic N (ON). Organic N is calculated as the difference between soil TN and soil mineral N. In the figure, error bars represent standard errors (n = 3).
With the rapid depletion of soil extractable mineral N, the release of fertilizer-derived ON and mineral fixed NH4+ has become critical for soil N supply during the middle and late growth stages (27). In this study, the 15N recovery in the ON pool showed a stable or minimal reduction trend during the three stages of maize growth (V6, VT, R6) (Figures 2, 4), indicating that the in-season N supply capacity of fertilizer-derived ON is limited (21). An earlier 15N tracer study by Clay et al. (25) also observed the relatively stable nature of fertilizer-derived soil ON at three sampling times during maize growth. In summary, the addition of NIs promotes the preservation of fertilizer N in the form of soil ON, which could improve crop yields. However, we know little about the underlying mechanisms. Future research needs to explore the extent to which soil preserved fertilizer-derived ON can be released and how efficiently it can provide N to crops in the long term.
4 Conclusions
Our findings demonstrate that the newly retained fertilizer-derived ON during the early growth stage of maize serves as a relatively stable N reservoir. The addition of NI is an effective strategy for stabilizing the applied fertilizer N, primarily in the form of ON. However, the release of ON was unsuccessful during the middle and late growth stages of maize. This suggests that the long-term N supply could be enhanced by regulating the conversion of N fertilizer from mineral N pools to ON pools.
Data availability statement
The raw data supporting the conclusions of this article will be made available by the authors, without undue reservation.
Author contributions
ZQ: Conceptualization, Data curation, Formal analysis, Funding acquisition, Investigation, Methodology, Project administration, Resources, Software, Supervision, Validation, Visualization, Writing – original draft, Writing – review & editing. SL: Data curation, Formal analysis, Investigation, Methodology, Resources, Writing – review & editing. DL: Data curation, Formal analysis, Investigation, Methodology, Writing – review & editing. CL: Data curation, Formal analysis, Investigation, Methodology, Writing – review & editing. ZX: Formal analysis, Investigation, Methodology, Writing – review & editing. XC: Conceptualization, Methodology, Supervision, Validation, Visualization, Writing – review & editing. YF: Conceptualization, Data curation, Funding acquisition, Investigation, Methodology, Resources, Supervision, Visualization, Writing – review & editing.
Funding
The author(s) declare financial support was received for the research, authorship, and/or publication of this article. This research was supported by the National Key Research and Development Program of China, Project No. 2023YFD1501400 and 2023YFD1500802; the Strategic Priority Research Program of the Chinese Academy of Sciences, Project No. XDA28020302; the National Natural Science Foundation of China, Project No. 42177214; the Shandong Provincial Natural Science Foundation, Project No. ZR2023YQ030; the Liaoning Vitalization Talents Program, Project No. XLYC2203058 and XLYC1902016; the Shenyang Science and Technology Talent Program, Project No. RC230102; and the Taishan Scholars Program, Project No. 202211306. We also acknowledge support from the K.C. Wong Edu-cation Foundation (YF) and the Youth Innovation Promotion Association of the Chinese Academy of Sciences (ZQ).
Acknowledgments
We would like to thank Editage (www.editage.cn) for English language editing.
Conflict of interest
The authors declare that the research was conducted in the absence of any commercial or financial relationships that could be construed as a potential conflict of interest.
Generative AI statement
The author(s) declare that no Generative AI was used in the creation of this manuscript.
Publisher’s note
All claims expressed in this article are solely those of the authors and do not necessarily represent those of their affiliated organizations, or those of the publisher, the editors and the reviewers. Any product that may be evaluated in this article, or claim that may be made by its manufacturer, is not guaranteed or endorsed by the publisher.
References
1. Erisman JW, Galloway JN, Dice NB, Sutton MA, Bleeker A, Grizzetti B, et al. Nitrogen too much of a vital resource: Science Brief. Zeist, The Netherlands: WWF Netherlands (2015).
2. Qasim W, Xia L, Shan L, Li W, Zhao Y, Butterbach-Bahl K. Global greenhouse vegetable production systems are hotspots of soil N2O emissions and nitrogen leaching: A meta-analysis. Environ pollut. (2020) 272:116372. doi: 10.1016/j.envpol.2020.116372
3. Zhao X, Wang Y, Cai S, Ladha JK, Castellano MJ, Xia L, et al. Legacy nitrogen fertilizer in a rice-wheat cropping system flows to crops more than the environment. Sci Bull. (2024) 69:1212–6. doi: 10.1016/j.scib.2024.02.027
4. Pan F, Yu W, Ma Q, Zhou H, Jiang C, Xu Y. Do organic amendments improve the synchronism between soil N supply and wheat demand? Appl Soil Ecol. (2018) 125:184–91. doi: 10.1016/j.apsoil.2018.01.006
5. Ding W, Xu X, He P, Ullah S, Zhang J, Cui Z, et al. Improving yield and nitrogen use efficiency through alternative fertilization options for rice in China: a meta-analysis. Field Crops Res. (2019) 227:11–8. doi: 10.1016/j.fcr.2018.08.001
6. Sha Z, Ma X, Wang J, Lv T, Li Q, Misselbrook T, et al. Effect of N stabilizers on fertilizer-N fate in the soil-crop system: A meta-analysis. Agricult Ecosyst Environ. (2020) 290:106763. doi: 10.1016/j.agee.2019.106763
7. Gardner JB, Drinkwater LE. The fate of nitrogen in grain cropping systems: a meta-analysis of 15N field experiments. Ecol Appl. (2009) 19:2167–84. doi: 10.1890/08-1122.1
8. Smith CJ, Chalk PM. The role of 15N in tracing N dynamics in agro-ecosystems under alternative systems of tillage management: A review. Soil Tillage Res. (2020) 197:104496. doi: 10.1016/j.still.2019.104496
9. Quan Z, Li S, Xun Z, Liu C, Liu D, Wang Y, et al. Evaluating the effects of reduced N application, a nitrification inhibitor, and straw incorporation on fertilizer-N fates in the maize growing season: A field 15N tracer study. Nitrogen. (2024) 5:584–97. doi: 10.3390/nitrogen5030039
10. Liu D, Fang Y, Tu Y, Pan Y. Chemical method for nitrogen isotopic analysis of ammonium at natural abundance. Analytical Chem. (2014) 86:3787–92. doi: 10.1021/ac403756u
11. Tu Y, Fang Y, Liu D, Pan Y. Modifications to the azide method for nitrate isotope analysis. Rapid Communication Mass Spectrometry. (2016) 30:1213–22. doi: 10.1002/rcm.v30.10
12. Beuters P, Scherer HW. Modification of the standard method for determination of non-exchangeable NH4-N in soil. Plant Soil Environ. (2012) 58:557–60. doi: 10.17221/575/2012-PSE
13. Rutherford PM, Juma NG. Effect of glucose amendment on microbial biomass, spelling fertilizer 15N-recovery and distribution in a barley-soilsystem. Biol Fertil Soils. (1992) 12:228–32. doi: 10.1007/BF00336037
14. Quan Z, Huang B, Lu C, Shi Y, Chen X, Zhou J, et al. Formation of extractable organic nitrogen in an agricultural soil: A 15N labeling study. Soil Biol Biochem. (2018) 118:161–5. doi: 10.1016/j.soilbio.2017.12.015
15. Ernfors M, Brennan FP, Müller C. The nitrification inhibitor dicyandiamide increases mineralization-immobilization turnover in slurry-amended grassland soil. J Agric Sci. (2014) 152:137–49. doi: 10.1017/S0021859613000907
16. Harty MA, McGeough KL, Carolan R, Müller C, Laughlin RJ, Lanigan GJ, et al. Gross nitrogen transformations in grassland soil react differently to urea stabilizers under laboratory and field conditions. Soil Biol Biochem. (2017) 109:23–34. doi: 10.1016/j.soilbio.2017.01.025
17. Ma Q, Wu Z, Yu W, Zhou C, Ning C, Yuan H, et al. Does the incorporation of dicyandiamide and hydroquinone with straw enhance the nitrogen supplying capacity in soil? Appl Soil Ecol. (2019) 136:158–62. doi: 10.1016/j.apsoil.2018.12.007
18. Schmidt MW, Torn MS, Abiven S, Dittmar T, Guggenberger G, Janssens IA, et al. Persistence of soil organic matter as an ecosystem property. Nature. (2011) 478:49–56. doi: 10.1038/nature10386
19. Sebilo M, Mayer B, Nicolardot B, Pinay G, Mariotti A. Long-term fate of nitrate fertilizer in agricultural soils. Proc Natl Acad Sci United States America. (2013) 110:18185–9. doi: 10.1073/pnas.1305372110
20. Ma Q, Wu Z, Shen S, Zhou H, Jiang C, Xu Y, et al. Responses of biotic and abiotic effects on conservation and supply of fertilizer N to inhibitors and glucose inputs. Soil Biol Biochem. (2015) 89:72–81. doi: 10.1016/j.soilbio.2015.06.024
21. Ma Q, Li S, Xu Z, Fan S, Xia Z, Zhou C, et al. Changes in N supply pathways under different long-term fertilization regimes in Northeast China. Soil Tillage Res. (2020) 201:104609. doi: 10.1016/j.still.2020.104609
22. Liang C, Schimel JP, Jastrow JD. The importance of anabolism in microbial control over soil carbon storage. Nat Microbiol. (2017) 2:17105. doi: 10.1038/nmicrobiol.2017.105
23. Xiao KQ, Liang C, Wang Z, Peng J, Zhao Y, Zhang M, et al. Beyond microbial carbon use efficiency. Natl Sci Rev. (2024) 11:nwae059. doi: 10.1093/nsr/nwae059
24. Zhang F, Cui Z, Chen X, Ju X, Shen J, Chen Q, et al. Integrated nutrient management for food security and environmental quality in China. Adv Agonomy. (2012) 116:1–40. doi: 10.1016/B978-0-12-394277-7.00001-4
25. Clay DE, Malzer GL, Andersonand JL. Tillage and dicyandiamide influence on nitrogen-fertilizer immobilization, remineralization, and utilization by maize (Zea-Mays L.). Biol Fertil Soils. (1990) 9:220–5. doi: 10.1007/BF00336229
26. Mariano E, Boschiero BN, Trivelin PCO. Effects of N application rate and dicyandiamide on the fate of 15N fertilizer and the abundance of microbial genes in a sandy soil amended with sugarcane litter. J Soil Sci Plant Nutr. (2022) 22:359–73. doi: 10.1007/s42729-021-00654-2
Keywords: soil N transformation, microbial N assimilation, fertilizer N fate, immobilization and remineralization, straw returning
Citation: Quan Z, Li S, Liu D, Liu C, Xun Z, Chen X and Fang Y (2025) Nitrification inhibitor promotes fertilizer N stabilization in soil as organic forms during a growing season of maize: a field 15N tracer study. Front. Soil Sci. 5:1520476. doi: 10.3389/fsoil.2025.1520476
Received: 31 October 2024; Accepted: 02 January 2025;
Published: 22 January 2025.
Edited by:
Upendra Kumar, National Rice Research Institute (ICAR), IndiaReviewed by:
Haijun Sun, Nanjing Forestry University, ChinaPengshuai Shao, Shandong University of Aeronautics, China
Copyright © 2025 Quan, Li, Liu, Liu, Xun, Chen and Fang. This is an open-access article distributed under the terms of the Creative Commons Attribution License (CC BY). The use, distribution or reproduction in other forums is permitted, provided the original author(s) and the copyright owner(s) are credited and that the original publication in this journal is cited, in accordance with accepted academic practice. No use, distribution or reproduction is permitted which does not comply with these terms.
*Correspondence: Zhi Quan, cXVhbnpoaUBpYWUuYWMuY24=; Yunting Fang, ZmFuZ3l0QGlhZS5hYy5jbg==