- 1Dirección de Supervisión y Monitoreo en las Estaciones Experimentales Agrarias, Instituto Nacional de Innovación Agraria (INIA), Lima, Peru
- 2Universidad César Vallejo, Lima, Peru
- 3Dirección de Supervisión y Monitoreo en las Estaciones Experimentales Agrarias, Estación Experimental Agraria Santa Ana, Instituto Nacional de Innovación Agraria (INIA), Huancayo, Peru
- 4Dirección de Supervisión y Monitoreo en las Estaciones Experimentales, Estación Experimental Agraria de Baños del Inca, Instituto Nacional de Innovación Agraria (INIA), Cajamarca, Peru
Introduction: Monoculture is a significant concern due to its negative impact on soil quality, resource productivity, and agricultural sustainability, particularly in vulnerable communities. This research aims to evaluate high Andean soil management for maca monoculture.
Materials and methods: To this end, interviews were conducted with maca farmers adjacent to Lake Chinchaycocha. The effect on soil quality was evaluated based on principal component analysis (PCA), weighted soil quality index (SQIw), and physico-chemical characteristics.
Results: The results indicated differences between farmers in agronomic management, monoculture period (from 5 to 9 years), and fallow time (up to 10 years in the best cases). Regarding soil quality, the PCA highlighted boron and total nitrogen locations in the same quadrant, with the highest contribution to the analysis. Finally, the SQIw showed that soils without maca cultivation presented better quality.
Conclusion: This research’s results indicate a need to optimize soil management practices, especially for small farmers, who are the most vulnerable group. In addition, further studies on boron and nitrogen availability in soils cultivated with maca are required, emphasizing areas that exceed 10 years of continuous use.
1 Introduction
Soil is an essential resource in hydrological and biogeochemical cycles, as it stores carbon and acts as a natural filter to purify water (1). Soil management to increase agricultural productivity must not jeopardize the ecosystem services it provides (2). In this sense, soil quality indicators use is of great importance for agricultural production sustainability (2).
Extensive monoculture agriculture can negatively alter soil fertility and even contaminate the soil (3). Continuous monoculture is an agronomic problem in extensive crops such as rice, maize, cotton, and potato; in ornamentals such as chrysanthemum and lilies; in horticultural crops such as tomato and watermelon; and mainly in medicinal crops (4). The main cause of soil fertility deterioration is nutrient imbalance since each plant has different requirements and absorption rates showing certain identifiable symptoms (5). In addition, continuous cultivation can significantly decrease essential nutrient content, such as available phosphorus and potassium, while balanced fertilizer use can be an alternative that enhances crop development (6).
It has been shown that soil physical fertility loss is due to water erosion of bare soils without vegetation and in most cases steep, during the fallow period (7). However, rotation with natural grasses, gramineae, leguminous, and grass residues incorporation with sheep manure, improves soil characteristics by increasing nitrogen mineralization and increasing available phosphorus and potassium (8).
Maca is the only Brassicaceae that can grow between 4000 and 4500 m altitude, where extremely low temperatures and intense solar radiation prevail and where mostly grasses survive (9). Maca’s main adaptive feature is that it can grow in areas with mean temperatures between 1.5 - 12°C. In addition, this crop is best adapted to slightly acidic soils with a pH between 6.5 and 7, and sensitive to strongly acidic pH values. It is known that soil pH values decrease with continuous years of cultivation (10). The maca production system requires long cultivation and fallow periods. However, it is important to further study the effect of continuous maca cultivation on the physical and chemical characteristics of the soil and to identify optimal management strategies to mitigate monoculture’s negative effects.
Continuous maca cultivation causes a reduction in its yield and quality (4). Custodio et al. (11) found that maca cultivation significantly reduced soil pH from 7.28 to 4.93 in fields with two successive maca campaigns. Wang et al. (4) report the same pH reduction effect from 6.89 to 6.79 by continuous maca cultivation, with significant differences, in soils with three successive campaigns. Likewise, in both studies, soil nitrogen, phosphorus, and potassium are reduced by the continuous maca cultivation effect. Lozano et al. (12) describe that soil acidity, exchangeable Al+3 and H+ high concentrations, and available phosphorus low levels are the cause of lower seed survival, reduced vegetative growth, and reduced maca yields in high Andean areas. In addition, acetic, propionic, butyric, and valeric acid root exudates, which are characteristic in maca cultivation, are the cause of acidity and increased populations of acid bacteria in the soil (4).
The Peruvian Andes has a great diversity of fragile ecosystems (scrublands, bogs, wetlands, grasslands, etc.), which can be negatively impacted by the intensive monoculture of maca (13). It is mainly cultivated in the “Chinchaycocha” area of the “Bombón” plateau in the central Andes of Peru. Its nutritional and medicinal properties, high price, and national and international demand have significantly increased its cultivation in the central Andes of Peru (14).
In 2018, the National Institute for Agrarian Innovation (INIA) of the Ministry of Agriculture and Irrigation (MINAGRI) promoted the application of new cultivation techniques to safeguard the quality of the soils that produce maca in Junín. This project was aimed at small farmers in the rural areas of Junin as part of the national project for the “Recovery of degraded soils of high Andean grasslands caused by the intensive cultivation of maca in this region of Peru” (15). For example, the Chinchaycocha Agricultural Producers’ Association in the department of Junin has been producing yellow, black, and red maca for more than 10 years, to meet domestic and international demand. While maca is a Peruvian traditional crop, Peru and China are the current leading consumers and producers of maca in the world. Between January-August 2023, Peruvian exports of maca reached 1830 tons, worth US$ 14 million, being Brazil (26.9%), United States (26.2%), Netherlands (7.3%), South Korea (7.1%) and Germany (5.4%) the main importing countries (16). The land area involved 8,000 hectares of maca cultivation in the national territory, with more than 10,000 producers dedicated to this crop (17). At the same time, more than 480 hectares of maca have achieved organic certification under EU organic regulations 834/2007 and 889/2008, USDA NOP, and National RTPO-Peru, for more than 480 hectares of maca. For this reason, it is necessary to find a sustainability balance, with special emphasis on agricultural production, environmental protection, and fragile ecosystems.
This research aims to evaluate soil management destined for the maca monoculture through structured interviews related to the production system by small farmers near Lake Chinchaycocha (Junin) and to evaluate the monoculture effect on soil quality as evidenced by physical-chemical characteristics and soil quality indexes, in cultivated and not cultivated fields with maca, to determine the trends and latent challenges to achieve sustainability, especially in these vulnerable high Andean communities of Peru.
2 Materials and methods
2.1 Study area characterization
The study was conducted in the region between 11°01’24” LS - 75°59’37” LW and 11°13’48” LS - 75°58’34” LW, at an elevation of 4136 - 4248 masl, within the Lake Chinchaycocha Commonwealth, Junín Department. The area experiences an average annual temperature ranging from 5 to 7°C, relative humidity between 60 and 70%, and cumulative yearly rainfall between 600 and 900 mm, with precipitation concentrated from November to April (18). According to the SoilGrids global digital soil mapping system (19), which follows the FAO-WRB (World Reference Base for Soil Resources) international soil classification system, the soils sampled in the Chinchaycocha community are classified as Cambisols. These soils exhibit early stages of development, characterized by moderate profile development where limited physical and chemical weathering of the parent material has occurred. Cambisols are typically found in mountainous regions or areas with significant geological activity (20). They are distinguished by the presence of a cambic subsurface horizon (Bw), marked by color differences compared to the parent material, due to the accumulation of clays, iron, and aluminum oxides, with minimal eluviation and illuviation processes (21). Figure 1 shows the cultivation sites examined in the study.
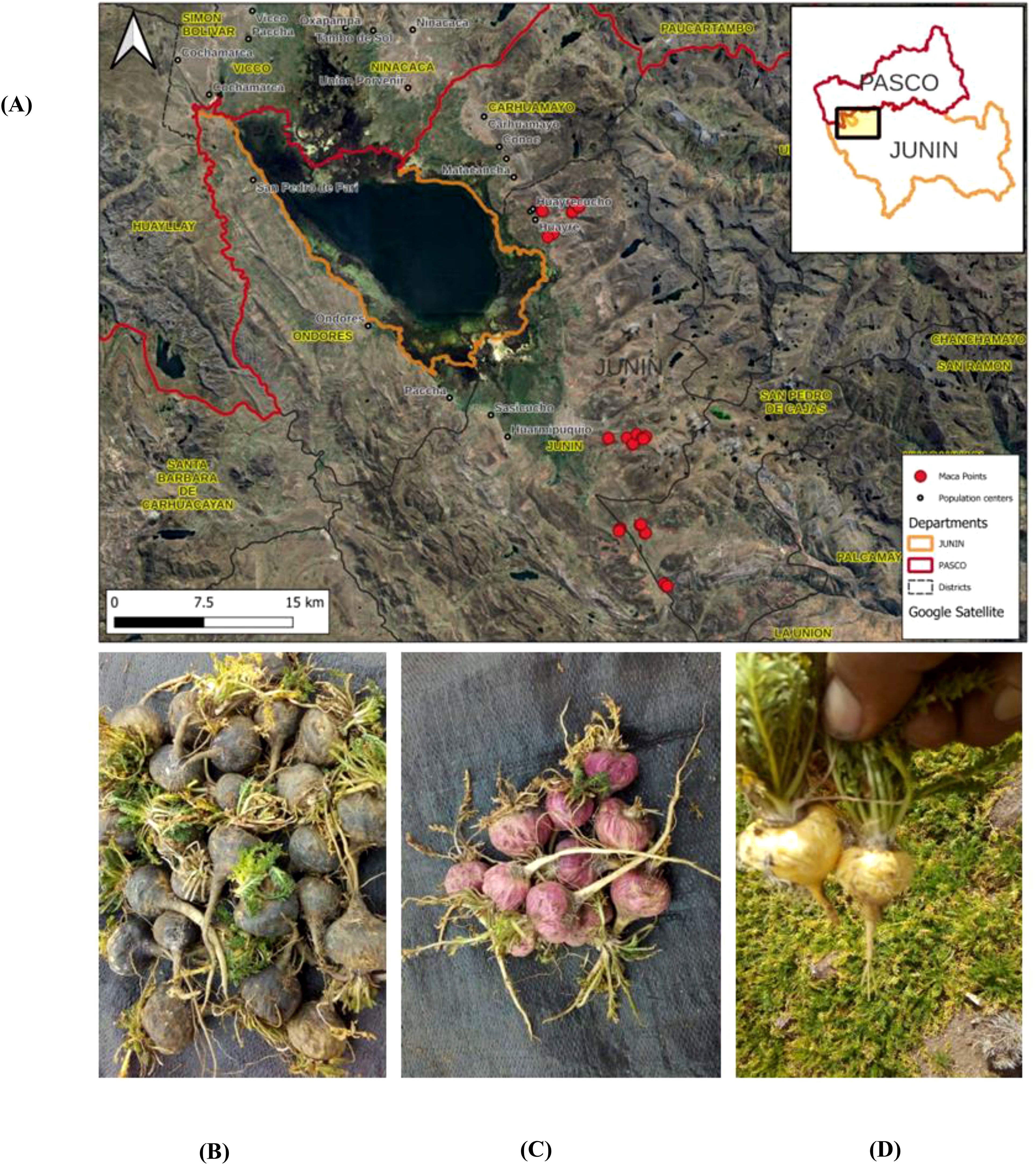
Figure 1. Location map showing soil sampling points in the Chinchaycocha Lake Commonwealth (A). Types of maca cultivated in the Chinchaycocha Lake Commonwealth: black maca (B), red maca (C), and yellow maca (D).
2.2 Soil sampling
The plots were randomly selected, ensuring that they had similar characteristics in terms of land use and agricultural management. For soil sampling choice, random plots of large and small farmers were used. The soil samples taken were from the districts of Junin and San Pedro de Cajas, in a very humid subalpine tropical paramo life zone, with slightly steep slopes, where the predominant vegetation is puna grassland and puna lawn.
The sampling process consisted of digging an approximately 20 cm deep hole, avoiding vegetation or stone areas that could alter the results. Once the sample was extracted, it was placed in the collection bucket and labeled respectively. This process was repeated in all the selected plots, making sure to follow the same steps and maintain consistency in the used technique. Once the extraction and collection of all samples were completed, they were stored in a container and subsequently sent to the Soil, Water, and Foliar Laboratory of the National Institute for Agrarian Innovation (INIA) for further analysis. Figure 2 shows part of the field visit.
2.3 Physico-chemical analysis of soils
Soil samples were analyzed at INIA’s Soil, Water, and Foliar Laboratories Network (LABSAF). The following methodologies were employed for soil characterization:
a. Percentage of sand, silt, and clay: According to the USDA international system, sand particle size distribution was determined by sieving. The proportions of silt and clay were measured using the Bouyoucos hydrometer method (22). A 40 g sample of air-dried soil, was passed through a 2 mm sieve, and 60 mL of sodium hexametaphosphate dispersant solution was used.
b. pH: Soil pH was determined by the potentiometric method using two electrodes in a suspension of 20 g of soil and 20 ml of distilled water (1:1 ratio) (23).
c. EC (dS·m-1): Soluble anion and cation concentrations were determined by measuring electrical conductivity in the saturation extract (24).
d. Organic Matter (%): Organic matter content was determined using the Walkley and Black method with potassium dichromate (K2Cr2O7) as the oxidizing agent (22). One gram of soil and 10 mL of 1 N potassium dichromate were used.
e. Calcium Carbonate (%): The carbonate content was measured by the acid neutralization method (25). A 1 g soil sample and 10 mL of 1 N HCl were used.
f. Available Phosphorus (mg·kg-1): Available phosphorus was determined using the Bray and Kurtz method, suitable for acid and neutral soils (22). A 2 g soil sample and 20 mL of acid extractant solution (0.03 N NH4F in 0.025 N HCl) were used.
g. Available Potassium (mg·kg-1): Available potassium was measured using a 1 N ammonium acetate (NH4CH3COO) extractant (26). A 5 g soil sample and 33 mL of ammonium acetate extractant solution were used.
h. Exchangeable Cations (mEq·100 g-1): The concentrations of Ca2+, Mg2+, K+, and Na+ were determined using the saturation method with ammonium acetate and atomic absorption spectrometry (22). A 5 g soil sample and 100 mL of 1 N ammonium acetate were used.
i. Effective CEC (mEq·100 g-1): Effective CEC was calculated as the sum of exchangeable bases: Ca2+, Mg2+, K+, and Na+.
j. Total Metals (mg·kg-1): The total contents of Be, V, Co, Ni, Cu, As, Se, Sr, Mo, Ag, Cd, Ti, Pb, Ca, B, Al, Cr, Mn, Zn, Ba, Hg, Na, Mg, K, and Fe were determined using EPA Method 3050B via inductively coupled plasma mass spectrometry (ICP-MS). A 0.5 g dry soil sample was used, and acid digestion was performed with 10 mL of concentrated nitric acid.
2.4 Interviews with maca farmers from the Chinchaycocha Lake Commonwealth
A structured questionnaire was designed with open-ended questions related to cultivation techniques, impact on the soil, conservation measures, and soil degradation perceptions in the maca production system. The proposed methodology by Castillo-Valdez et al. (27) was used, under ethical and free-will conditions, through the informed consent of 30 farmers dedicated to maca cultivation. The questions considered social, economic, and normative perceptions. Likewise, farmers with and without maca cultivation were also divided to record the crop’s empirical perception of soil degradation.
2.5 Weighted soil quality index calculation
A correlation matrix was constructed to establish a minimum data set (MDS) with indicators that had no redundancy between them. The stats (28) and ggcorrplot (29) packages were used, the first to obtain Pearson correlation coefficient values and the second to plot the matrix. Subsequently, a PCA was carried out, taking into account all principal components (PC). The “prcomp” function of the stats package (28) was used to obtain the eigenvalues and variables contributions, and the “fviz_pca_var” function of the factoextra package (29) was used to plot these contributions. The indicators were transformed by linear methods so a score was assigned to each indicator according to the “more is better” (Equation 1) and “less is better” (Equation 2) methods used by (30). Shown values in Table 1 were used in this study. To calculate the specific weightings (Sw) for each component we divided the variance percentage of each PC by the accumulated variance percentage of the last PC. Then the weightings variable (W) was obtained by multiplying it by the contribution values of each PC (Equation 3). Finally, the weighted soil quality index (SQIw) was obtained by running Equation 4 (30). All of these processes were executed in code supported with functions from the Tidyverse package (31).
Where, Vn = normalized value; Imin = minimum indicator value; Imax = maximum indicator value; lm = mean indicator value; W = variable weighting; Sw = PC specific weighting; C = contribution of the variable in the PC; n = number of selected PCs and SQIw = weighted soil quality index.
2.6 Statistical analysis
Two main data groups have been obtained, the first consisting of the results of measuring soil physicochemical properties and the second product of the interviews applied to the Lake Chinchaycocha Commonwealth farmers dedicated to maca cultivation. Descriptive statistics were developed using the Excel program and inferred using R studio software. The T-Student’s test was applied to compare the soil’s physicochemical characteristics results, between the group of soils with maca cultivation versus soils without cultivation.
3 Results and discussion
3.1 Land use patterns and maca production in Lake Junin adjacent area
Maca cultivation in the Peruvian Andean highlands extends from the Bombón plateau, also known as the “pampas of Junín”, to the districts of San Pedro de Cajas and Palcamayo in the province of Tarma, as well as the districts of Ondores, Junín, and Carhuamayo in the Junín province, of the department of Junín. The results made it possible to distinguish three levels of producers; the large producer sows more than 70 hectares per season with the capacity to export and transform the raw material. In addition, these producers receive technical support and use organic fertilizers and manure. There is also the medium-sized producer, who sows between 20 and 60 hectares per season and supplies the large producer and other traders who process the product; they rarely use organic fertilizers and amendments. Thirdly, there is the small producer, who sows less than 20 hectares and is generally a whole family dedicated to this activity. This producer does not use fertilizers or organic amendments to produce maca.
In terms of soil-use management, maca is often sown on meadow-type grass and puna lawns. Large farmers sow for up to maximum years continuously, using organic and mineral fertilizers. After that, they set an 8-to 10-years fallow period. On the other hand, the small producer sows a maximum period of 2 years continuously, followed by a fallow period. Large and medium-sized farmers lease plots of land to other farmers for sowing maca, which lasts on average 8-to 10-months. Random seed varieties (yellow, red, zamba, black, and purple maca, or others) are used for sowing, and few farmers select the maca seeds by color. Instead, after harvesting and drying, maca is selected by color and size to obtain better sale prices.
According to interviews with farmers, 60% use fertilizers, while the others only apply organic amendments (Figure 3A). Although chemical fertilizer application increases larger-scale production, proper management of its use is necessary to increase soil fertility and reduce pollution (32). The possibility of integrating inorganic fertilizers and organic manure use, as a strategy for integrated soil management and maca (Lepidium meyenii Walp.) crop nutrition, will contribute to the sustainability of the production system. These strategies make it possible to reduce nitrogenous inorganic fertilizer use, which has economic and ecological benefits for farmers (33). Shrestha et al. (34) reported that the balanced and routinary use of organic and inorganic fertilizers can reverse, within a few years, soil degradation produced and sustain crop productivity. Thus, Junín province producers must develop organic and inorganic fertilization techniques for their agricultural properties.
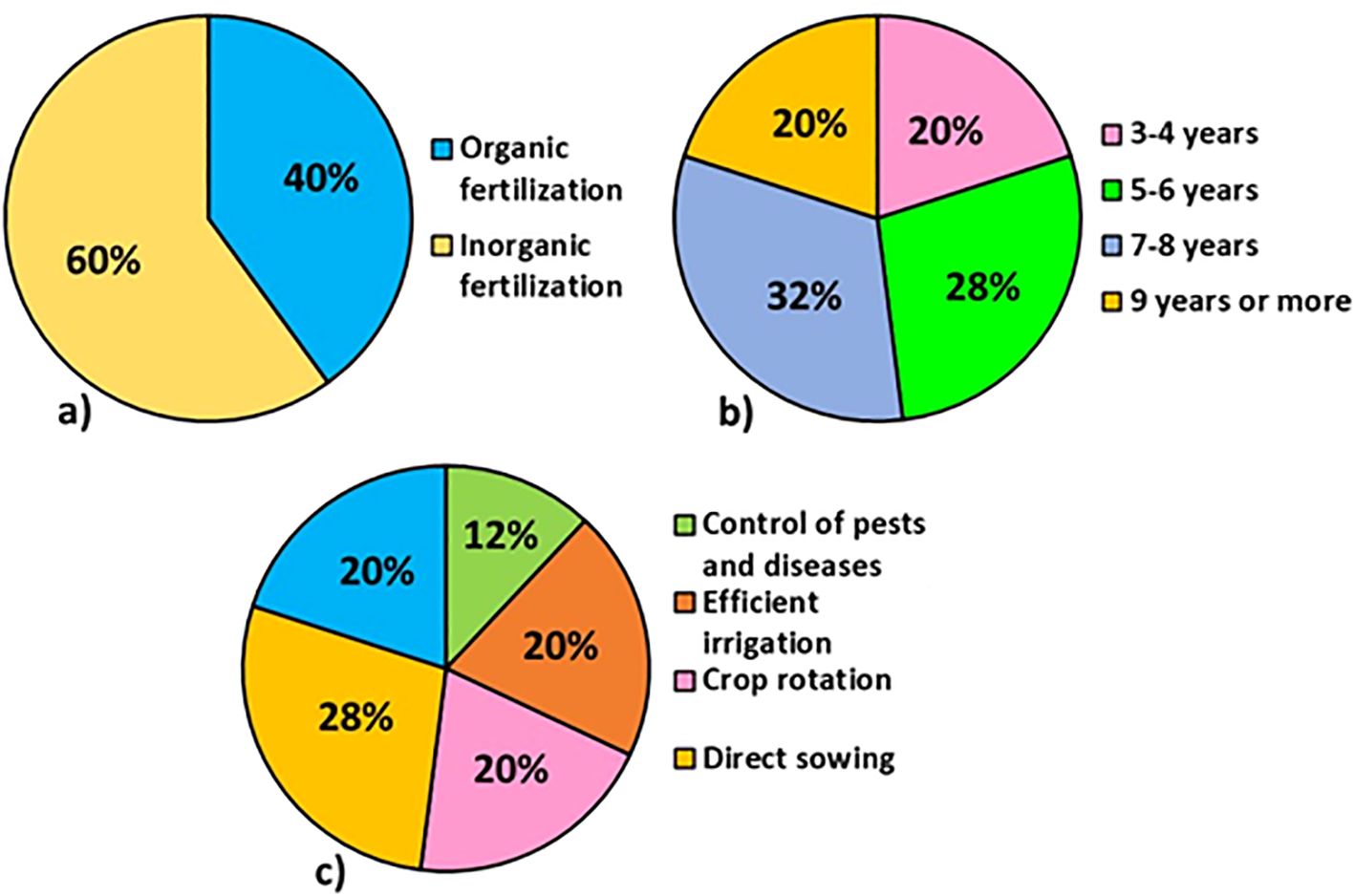
Figure 3. Pie chart of fertilization type used in maca cultivation in the Lake Chinchaycocha Commonwealth during the last 10 years (A), maca cultivation campaigns during the last 10 years (B), and used techniques to mitigate soil degradation during the last 10 years (C).
Monoculture proved to be the most prominent feature of the maca production system in the high Andean areas, where 100% of the farmers continuously sow for 3 to more than 9 years (Figure 3B). The survey showed that 20% of the farmers cultivate maca continuously for 3 to 4 years. 60% of the farmers carry out continuous maca production campaigns for 5 to 8 years. This practice can extend to more than 9 years in 20% of farmers, which is detrimental, as it causes crop yield and quality loss over the years, as well as reduction of soil organic matter, available phosphorus and potassium, and soil acidification (4, 11).
Maca growers do not commonly use mitigation techniques for soil degradation (Figure 3C). 80% of the farmers do not use crop rotation and only 20% use direct sowing and organic fertilizers application. Continuous soil conservation techniques such as rotation with gramineae and leguminous, and sheep or green manure use, have been shown to increase the available nitrogen, phosphorus, and potassium mineralization in maca production systems (8). Undoubtedly, diversified cropping systems would favor soil quality improvement by optimizing maca productivity. In addition, crop rotation mitigates climate change’s negative impact on agricultural systems, allowing soil physicochemical properties to change in a slow process (35). On the other hand, 20% of producers use efficient irrigation, and only 12% use pesticides for disease and pest control, an indicator of the crop’s low technological level.
3.2 Evaluation of the physicochemical characteristics of the soil change due to the effect of maca cultivation
The majority of the evaluated soils exhibit a loam texture (80%), followed by sandy loam (13%) and clay loam (7%), indicating that most of the soils fall into the medium-texture category (Table 2). The mean percentage composition of sand, silt, and clay is 45.46 ± 9.36%, 34.19 ± 5.48%, and 20.35 ± 7.87%, respectively. These characteristics suggest a high water infiltration capacity throughout the soil profile, as well as medium moisture retention (36). However, only 20% of farmers adequately irrigate the soil using furrow irrigation methods.
The maca monoculture system did not significantly alter soil pH. An average pH of 6.91 ± 0.5 was measured in soils cultivated with maca, compared to 6.87 ± 0.42 in uncultivated soils. This pH range is associated with a high nutritional potential due to nutrient solubility (36). Similar soil pH ranges have been observed in maca-producing areas at 3000 masl in Yunnan Province, China (4). However, the results indicated a slight decrease in pH from 6.89 ± 0.02 to 6.79 ± 0.01 after three years of continuous maca cultivation. Custodio et al. (11) reported more pronounced soil acidification, with pH dropping from 7.28 to 4.93 after two successive growing seasons. It is likely that the pH of soils cultivated with maca in the high Andean zones of Junín Province will remain stable, attributable to a significant increase in organic matter content from 5.20 ± 0.58% to 5.80 ± 0.75% (Figures 4, 5). These findings contrast with those of Wang et al. (4), who documented a decline in soil organic matter from 5.57 ± 0.08% to 3.03 ± 0.05%. Additionally, 60% of growers exclusively utilize organic fertilization to meet the crop’s nutritional requirements, which may contribute to the increase in soil organic matter. This aligns with the observations of Rolando et al. (8), who noted that the incorporation of native grass residues and sheep manure enhances soil organic matter content in maca cropping systems in the high Andes of Peru. Consequently, the increase in soil organic matter may create a buffering effect on changes in soil pH, mitigating the acidification induced by maca root exudates (37).
Among the chemical characteristics of the soil, there was a significant increase in electrical conductivity (EC) and exchangeable sodium, which could pose future challenges if agronomic practices are not implemented to mitigate the levels of exchangeable sodium in soils cultivated with maca. The EC of soils under maca monoculture is measured at 0.38 ± 0.14 dS·m-1, while in non-monoculture soils, the EC is 0.11 ± 0.04 dS·m-1. Similarly, soils with monoculture exhibit exchangeable Na+ values of 0.11 ± 0.03 mEq·100 g-1, compared to 0.03 ± 0.05 mEq·100 g-1 in non-monoculture soils. A comparative analysis of total sodium in the soil and the ratio of exchangeable sodium to total sodium was conducted (Figures 6, 7). The results indicated that both soil types have similar total sodium storage capacities, ranging from 51.74 to 55.45 mg· kg-1. However, in soils cultivated with maca, there is a 61.3% extraction of total sodium into the exchangeable form, whereas in non-cultivated soils, this value is only 16.4%. It is important to note that, in soils cultivated with maca, exchangeable Na+ demonstrates a high negative correlation with the exchangeable calcium percentage (ECP) (R = -0.67). Additionally, it shows a high positive correlation with the percentage of sand (R = 0.70), total magnesium (R = 0.51), and exchangeable Mg2+ (R = 0.61). These findings suggest that the slight increases in soil salinity may be attributed to enhanced sodium solubility, a characteristic of these sandy soils (with a sand content of 45.46 ± 9.36%), where magnesium-rich minerals such as vermiculite are prevalent in Cambisol-type soils, further facilitating sodium extraction (19, 38, 39).
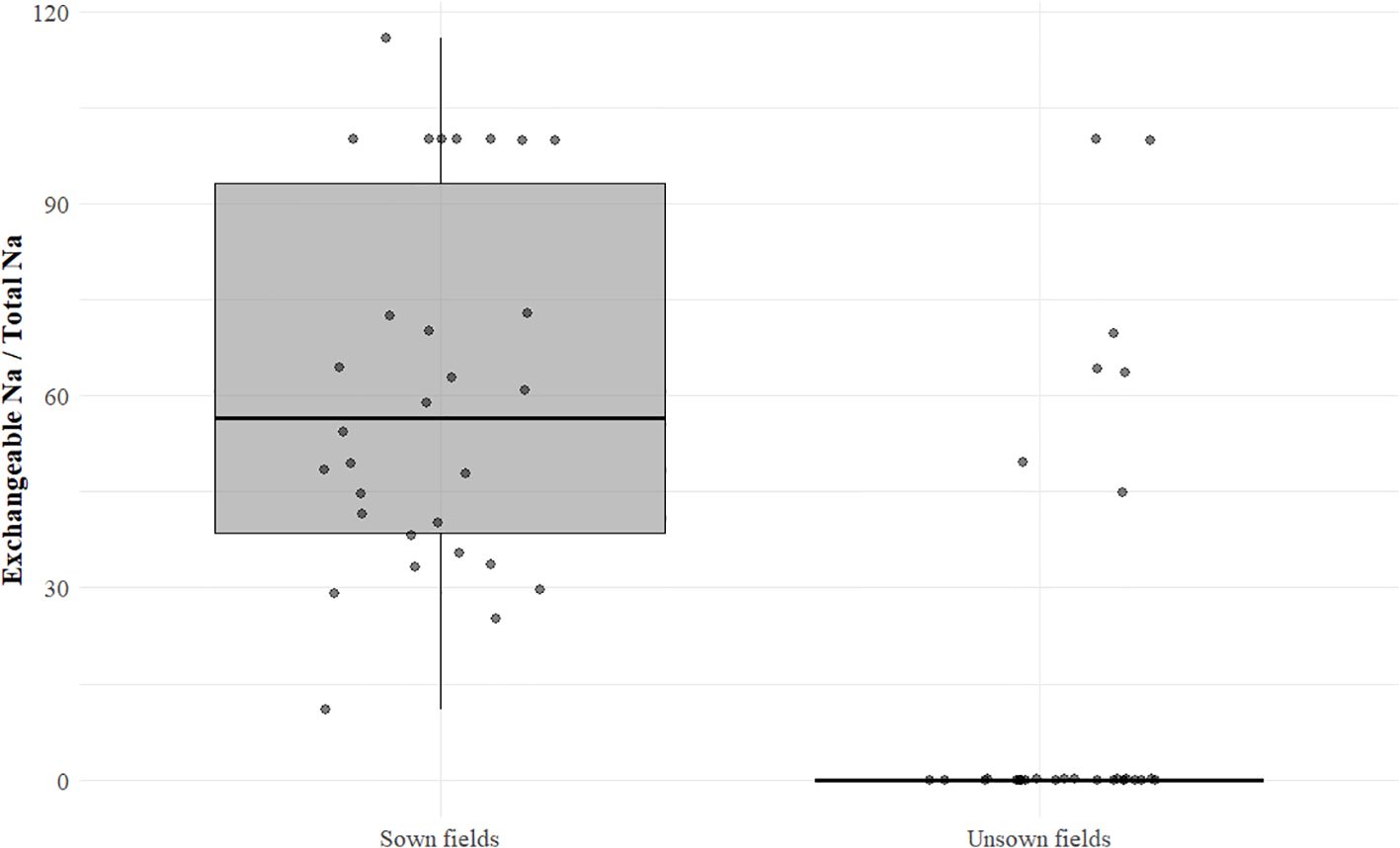
Figure 7. Relationship between exchangeable Na and total Na in the soil as a result of maca cultivation.
3.3 Effect of maca cultivation on soil fertility
Soil available phosphorus in fields cultivated with maca increases from 59.15 ± 36.36 mg·kg-1 to 70.27 ± 37.75 mg·kg-1. These findings suggest that current fertilization practices for maca are enhancing the available phosphorus reserves in the soil, primarily from organic sources. This outcome contrasts with the majority of studies that report a negative impact of maca cultivation on soil phosphorus availability (4, 8, 11, 12). Additionally, while soil available potassium does not decline due to maca cultivation, the percentage of potassium saturation decreases from 8.85 ± 8.26% to 5.94 ± 3.96%. This reduction is attributed to a significant increase in sodium saturation, as sodium competes with potassium for exchange sites on soil colloids (40). Continuous cultivation of maca for three or more seasons may lead to potassium deficiencies due to this competitive effect of sodium.
The t-test for paired two-sample means (p = 0.0045) revealed a significant difference in total nitrogen content between soils cultivated with maca and those without. Specifically, the percentage of total nitrogen decreased from 0.73 ± 0.11% to 0.65 ± 0.11% as a result of maca cultivation. This decline can be attributed to the crop’s high nitrogen requirements (8). Nitrogen plays a crucial role in the metabolism of glucosinolates (GLs), which are the most significant secondary metabolites in maca (41). The accumulation of GLs increases in relation to the growth of the maca hypocotyl and nitrogen uptake (42). Furthermore, a substantial accumulation of GLs occurs at the foliar level, with these compounds being translocated to the hypocotyl, thus enhancing their concentration even after the harvest. The biosynthesis of GLs is closely linked to nitrogen metabolism and the production of amino acids, primarily leucine, tryptophan, and tyrosine (41). This study identified a significant reduction in soil nitrogen percentage in fields cultivated with maca (Figure 8).
3.4 Maca cultivation in high Andean areas decreases the total boron content of the soil
In this study, total soil boron was assessed using microwave-assisted nitric acid digestion. The results indicated an average concentration of 6.95 ± 1.15 mg·kg-1 for soils cultivated with maca and 8.89 ± 0.77 mg·kg-1 for uncultivated soils, with statistically significant differences observed (p < 0.05) (Figure 9). Total soil boron comprises five fractions: readily available boron, boron adsorbed to clay, boron bound to oxides, boron bound to organic compounds, and residual boron (43). Available soil boron includes both readily usable boron and boron adsorbed to clay, which together are estimated to constitute 1-2% of the total soil boron (43). Specifically, the available boron in soils cultivated with maca ranged from 0.07 ± 0.01 to 0.14 ± 0.02 mg·kg-1, while in uncultivated soils, it ranged from 0.09 ± 0.18 to 0.18 ± 0.02 mg·kg-1.
Research has indicated that the critical threshold for available boron concentration necessary for crop growth ranges between 0.51 and 0.52 mg·kg-1 (44). Furthermore, different optimal boron levels have been identified for various soil types: in entisols, the range is between 0.53 and 0.54 mg·kg-1, while in inceptisols, it is between 0.57 and 0.58 mg·kg-1 (45, 46). For Brassicaceae species, the threshold varies from 0.50 to 1 mg·kg-1 (45). These findings suggest that in the high Andean cambisols of Junín province, where maca is cultivated, the available boron levels in the soil are significantly below what is required for optimal crop development.
However, soils cultivated with maca exhibited significantly lower boron levels compared to uncultivated soils, suggesting that maca cultivation may have a high extraction capacity for boron adsorbed onto clay, boron bound to oxides, and boron associated with organic compounds. Furthermore, the cultivation practices in high Andean regions often neglect corrective or maintenance fertilization for soil boron, with only 40% of producers implementing mineral fertilization with macronutrients. Consequently, the intensification of maca cultivation contributes to the depletion of this essential nutrient, resulting in its reduced availability for subsequent crops.
Boron is an element that maca readily utilizes, as it is a nutrient that moves easily through the phloem (47). In contrast, for many other plant species, boron is considered less mobile, with its movement primarily occurring through the xylem via transpiratory flow. This difference arises because maca synthesizes polyols as its primary mobile carbohydrates, which are transported alongside boron, whereas other species typically rely on sucrose for this function (47, 48). In Brassica species, mannitol-type sugar alcohols have been shown to form complexes with boron, facilitating its transport to growing structures such as young leaves and hypocotyls (47).
Another evidence of maca’s high boron requirement is the accumulation of phenolic compounds in the hypocotyls at the time of harvest. Boron is a crucial nutrient for the enzyme phenylalanine ammonium lyase (PAL), which plays a key role in the synthesis of phenolic compounds within the shikimic acid pathway (49). The biochemical composition of maca is characterized by a significant proportion of phenolic compounds, with flavanols being the most prominent. Yabar (41) reports that between 90 days prior to harvest and at the time of harvest, the total flavanol content increases from 18.17 to 55.48 mg·100 g-1 of dry matter. Thus, maca demonstrates a substantial boron requirement for phenolic metabolism.
3.5 Principal component analysis
In the first PCA, 30 important variables were selected and filtered. The results of the final PCA can be seen in Figure 10. The PCA showed that there is a diversity in soil management and soil status in terms of pH, which is the parameter that governs the ionic availability of elements and sand that shapes soil texture. The first two components of the PCA explained 34% of the total variance (first component: 20%; second component: 14%). The CaCO3 content, used by farmers to regulate the soil, was found to be associated with pH, while sand was not related since the direction between the two loads was almost perpendicular. All cationic parameters and salts were positively related to soil pH and negatively related to sand (p< 0.05). The sand was more strongly associated with organic matter (organic carbon) and total magnesium (Mg) content.
The analysis revealed that the centroid of the sites without maca cultivation was located in the third quadrant, and its direction coincides with the variables B and N. This suggests that these sites may have a higher availability of these elements. Additionally, it was observed that certain areas without maca cultivation are associated with high clay and silt contents. On the other hand, the centroid of the sites with maca cultivation is arranged towards the variables P_av (available phosphorus), Na, K_av (available potassium), K_ex (exchangeable potassium), and heavy metals such as Hg, and Mn, as well as EC and longitude (east).
Boron and nitrogen were associated with a loamy texture that provides a larger specific area to compete with cationic forms and salts present in the soil, including P forms.
3.6 Weighted soil quality index calculation: selection of soil quality indicators
Pearson’s statistic indicated a high positive correlation between soil CEC and pH (Table 3). In addition, soil CEC is also negatively correlated with the exchangeable potassium percentage (EPP). Soil pH is negatively correlated with EPP. Likewise, there is a high negative correlation between soil total boron and EC. Thus, the minimum data set (MDS) for the quality index construction was established as pH, available P, available K, OM percentage, ESP, total boron, and total nitrogen.
3.7 Soil quality index in land use systems with and without maca cultivation
Soil evaluation after maca cultivation showed that the soil quality was significantly lower compared to uncultivated soils. The soil quality values without maca cultivation were found to be twice as high as those derived from maca cultivation, and this difference was statistically significant (p-value <0.05). This research also revealed that soils with maca cultivation had the highest total B values (average = 8.89 mg·kg-1), while soils without maca cultivation had the lowest (average = 6.95 mg·kg-1). Total nitrogen percentage in soils without maca cultivation was also higher, with an average of 0.73% compared to 0.65% in soils with maca cultivation. In addition, the ESP in soils without maca cultivation was found to be lower (1.24%) compared to the soil with maca cultivation, which had the highest values (3.25%). The pH of both soil types was in the neutral range. This research found that B and N were the variables that contributed the most to the PCA and therefore to the SQIw. Understandably, the soils without maca cultivation had the highest soil quality compared to the areas with maca cultivation, as shown in Figure 11.
3.8 Trends and challenges
This study highlights the importance of assessing the impact of soil management on maca monoculture. It aims to alert not only users, such as the associations of small Andean farmers, but also decision-makers about the need to optimize and strengthen soil management programs, recovery, and care. In the future, the authors hope to develop studies to improve the indicators analyzed in this research in the medium and long term (10-20 years) following what was stated by Ortiz et al. (50). Further research is needed to determine the critical thresholds of available boron concentration in the soil and its extraction by maca. Additionally, aspects such as the total nitrogen consumption and pH, as parameters that allow elements mobility, deserve special attention. Boron is essential for soil nutrition, and its absorption kinetics by plant roots is a problem that arises due to nutrient content deficiency or excesses, like nitrogen, which can cause soil and crop toxicity (46). It is crucial to study the chemical forms of elements present in the soil based on their solubility and availability to the plant and soil conditions, as well as applied land use practices. The intensity of land use has increased in high Andean smallholder farming communities, and innovative ideas are needed to maintain soil fertility and productivity. Fallowing and crop rotation, in addition to ground rest, is a healthy option as long as appropriate evaluation criteria are determined to improve soil quality. It is essential to look for multifunctional options to maintain soil quality reflected in soil productivity and the generation of additional sources of income. In the sustainability context, further research should look forward at nutrient balance and climate change influence aspects.
4 Conclusions
High Andean soil management used for monoculture maca cultivation has been studied and their quality status has been established based on four relevant aspects: interviews with local farmers, soil physico-chemical characteristics analysis, principal component analysis, and the quality index in the sustainability context. It has become evident that there is a big difference between large and small farmers who make use of the land, which is why a better practice is developed for those farmers who use an average of 70 ha, through organic fertilizers use and crop rotation, which only reaches 20% of the farming community. Although small farmers reduce their cultivation period, continuous cultivation periods of more than 9 years have been recorded. The fallow land application has counteracted monoculture effects; however, it is already detrimental to crop quality. Boron and nitrogen presence was associated with a silty texture that provides a larger specific area to compete with the cationic forms and salts present in the soil, including P forms. In addition, the SQIw provided relevant information on the degradation process that has been occurring in these maca-growing soils, as well as fallow use weakness and extended cultivation periods without adequate practices. This is a problem that suggests an imbalance in nutrient supply or availability such as nitrogen and phosphorus in the presence of boron, which could cause soil toxicity.
Data availability statement
The raw data supporting the conclusions of this article will be made available by the authors, without undue reservation.
Ethics statement
Written informed consent was obtained from the individual(s) for the publication of any identifiable images or data included in this article.
Author contributions
RS-A: Conceptualization, Formal analysis, Funding acquisition, Methodology, Project administration, Validation, Writing – original draft. MC: Data curation, Investigation, Validation, Writing – review & editing. MS: Data curation, Investigation, Writing – review & editing. RC-T: Conceptualization, Formal analysis, Methodology, Writing – original draft. VC: Writing – review & editing. SL: Data curation, Formal analysis, Software, Visualization, Writing – review & editing. AA: Investigation, Resources, Writing – review & editing. PV: Investigation, Resources, Writing – review & editing. KQ: Formal analysis, Visualization, Writing – review & editing. CC-L: Software, Visualization, Writing – review & editing. JPC: Formal analysis, Writing – original draft. JCC: Funding acquisition, Project administration, Supervision, Writing – review & editing. CT: Project administration, Supervision, Writing – review & editing.
Funding
The author(s) declare financial support was received for the research, authorship, and/or publication of this article. This research was funded by the INIA project CUI 2487112 “Mejoramiento de los servicios de investigación y transferencia tecnológica en el manejo y recuperación de suelos agrícolas degradados y aguas para riego en la pequeña y mediana agricultura en los departamentos de Lima, Áncash, San Martín, Cajamarca, Lambayeque, Junín, Ayacucho, Arequipa, Puno y Ucayali”.
Acknowledgments
To the farmers of the Lake Chinchaycocha area.
Conflict of interest
The authors declare that the research was conducted in the absence of any commercial or financial relationships that could be construed as a potential conflict of interest.
Publisher’s note
All claims expressed in this article are solely those of the authors and do not necessarily represent those of their affiliated organizations, or those of the publisher, the editors and the reviewers. Any product that may be evaluated in this article, or claim that may be made by its manufacturer, is not guaranteed or endorsed by the publisher.
References
1. Andrés-Abellán M, Wic-Baena C, López-Serrano FR, García-Morote FA, Martínez-García E, Picazo MI, et al. A soil-quality index for soil from Mediterranean forests. Eur J Soil Sci. (2019) 70:1001–11. doi: 10.1111/ejss.12798
2. Williams H, Colombi T, Keller T. The influence of soil management on soil health: An on-farm study in southern Sweden. Geoderma. (2020) 360:114010. doi: 10.1016/j.geoderma.2019.114010
3. Zeeshan Ul Haq M, Yu J, Yao G, Yang H, Iqbal HA, Tahir H, et al. A systematic review on the continuous cropping obstacles and control strategies in medicinal plants. Int J Mol Sci. (2023) 24:12470. doi: 10.3390/ijms241512470
4. Wang S, Dong L, Luo Y, Jia W, Qu Y. Characterization of rhizosphere microbial communities in continuous cropping maca (Lepidium meyenii) red soil, Yunnan, China. Arch Agron Soil Sci. (2020) 66:805–18. doi: 10.1080/03650340.2019.1639155
5. Shrivastav P, Prasad M, Singh T, Yadav A, Goyal D, DrA A, et al. Role of nutrients in plant growth and development. Contam Agric. (2020), 43–59. Available online at: https://link.springer.com/chapter/10.1007/978-3-030-41552-5_2.
6. Chen Y, Hu S, Guo Z, Cui T, Zhang L, Lu C, et al. Effect of balanced nutrient fertilizer: A case study in Pinggu District, Beijing, China. Sci Total Environ. (2021) 754:142069. doi: 10.1016/J.SCITOTENV.2020.142069
7. Rolando JL, Dubeux JC, Perez W, Ramirez DA, Turin C, Ruiz-Moreno M, et al. Soil organic carbon stocks and fractionation under different land uses in the Peruvian high-Andean Puna. Geoderma. (2017) 307:65–72. doi: 10.1016/j.geoderma.2017.07.037
8. Rolando JL, Dubeux JCB, Ramirez DA, Ruiz-Moreno M, Turin C, Mares V, et al. Land use effects on soil fertility and nutrient cycling in the Peruvian high-andean puna grasslands. Soil Sci Soc America J. (2018) 82:463–74. doi: 10.2136/sssaj2017.09.0309
9. Chen R, Wei J, Gao Y. A review of the study of active components and their pharmacology value in Lepidium meyenii (Maca). Phytother Res. (2021) 35:6706–19. doi: 10.1002/ptr.7257
10. Huarancca Reyes T, Esparza E, Crestani G, Limonchi F, Cruz R, Salinas N, et al. Physiological responses of maca (Lepidium meyenii Walp.) plants to UV radiation in its high-altitude mountain ecosystem. Sci Rep. (2020) 10:2654. doi: 10.1038/s41598-020-59638-4
11. Custodio M, Huaraca-Meza F, Peñaloza R, Alvarado-Ibañez JC, de la Cruz-Solano H. Composición bacteriana en suelos de cultivo de maca (Lepidium meyenii Walp) analizada mediante metagenómica: un estudio en los Andes centrales del Perú. Sci Agropecuaria. (2021) 12:175–83. doi: 10.17268/sci.agropecu.2021.020
12. Lozano-Canales A, Janampa-Santome M, Clark D, Gonzáles WL. Seed weight predicts seedling emergence, and extremely acid soil and low availability of Phosphorus are associated with poor plant performances in Lepidium meyenii Walpers (maca). Sci Hortic. (2019) 253:341–8. doi: 10.1016/j.scienta.2019.04.059
13. Brack Egg A. Biodiversidad amazónica: potencialidades y riesgos. Global Business Admin J. (2018) 2:100–11. doi: 10.31381/gbaj.v2i2.2203
14. Huaraca-Meza F, Custodio M, Peñaloza R, Alvarado-Ibañez J, Paredes R, de la Cruz H, et al. Bacterial diversity in high Andean grassland soils disturbed with Lepidium meyenii crops evaluated by metagenomics. Braz J Biol. (2021) 82:e240184. doi: 10.1590/1519-6984.240184
15. INIA promueve nuevas técnicas para cuidar calidad de suelos de cultivos de maca en Junín . Instituto Nacional de Innovación Agraria. Available online at: https://www.inia.gob.pe/2018-nota-218/ (Accessed April 7, 2024).
16. Exportaciones Peruanas de maca sumaron 1.830 toneladas por US$ 14 millones entre enero y agosto de 2023 . Available online at: https://www.agraria.pe/noticias/exportaciones-peruanas-de-maca-sumaron-1-830-toneladas-por-u-33608 (Accessed April 8, 2024).
17. Minagri: El Perú es el primer productor y consumidor de maca en el mundo - SENASA al día . Available online at: https://www.senasa.gob.pe/senasacontigo/el-peru-es-el-primer-productor-y-consumidor-de-maca-en-el-mundo/ (Accessed April 7, 2024).
18. SENAMHI. Descarga de datos. Servicio Nacional de Meteorología e Hidrología del Perú. (s.f.). Available online at: https://www.senamhi.gob.pe/site/descarga-datos/.
19. Hengl T, Mendes de Jesus J, Heuvelink GB, Ruiperez Gonzalez M, Kilibarda M, Blagotić A, et al. SoilGrids250m: Global gridded soil information based on machine learning. PloS One (2017) 12(2):e0169748.
20. Saldaña H, Muñoz C, Esenarro D, Rodriguez C, Amaya P. Reference levels for heavy metals in soil in the city of cerro de pasco-Peru-2020. In: Asia conference on environment and sustainable development. Singapore: Springer Singapore (2021). p. 14–28.
21. Mantel S, Dondeyne S, Deckers S. World reference base for soil resources (WRB). In: Michael J, editor. Goss, margaret oliver encyclopedia of soils in the environment, 2nd ed. (2023). p. 206–17.
22. NOM-021-RECNAT-2000 — Norma Oficial Mexicana Que Establece Las Especificaciones de Fertilidad, Salinidad y Clasificación de Suelos. NOM-021-RECNAT-2000 — Norma Oficial Mexicana Que Establece Las Especificaciones de Fertilidad, Salinidad y Clasificación de Suelos. Estudios, Muestreo y Análisis. (2002).
23. USEPA. Method 9045D: Soil and waste pH. Washington, D.C.: U.S. Environmental Protection Agency (2004). Available online at: https://www.epa.gov/sites/default/files/2015-12/documents/9045d.pdf.
24. Standardization ISO. International O for. ISO 11265:1994 Soil quality — Determination of the specific electrical conductivity (1994). Available online at: https://www.iso.org/standard/19243.html (Accessed April 7, 2024).
25. ISO. Soil quality—Determination of organic and total carbon after dry combustion (elementary analysis) (ISO 10694: 1995). Berlin: Beuth. (1995).
26. Bazán Tapia R. Manual de procedimientos de los análisis de suelos y agua con fines de riego. (2017).
27. Castillo-Valdez X, Etchevers-Barra JD, Hidalgo-Moreno CMI, Aguirre-Gómez A. Evaluación de la calidad de suelo: generación e interpretación de indicadores. Rev TERRA LATINOAMERICANA. (2021) 39. doi: 10.28940/terra.v39i0.698
28. Team RC. R: A Language and Environment for Statistical Computing (2023). Available online at: https://www.R-project.org/.
29. Kassambara A, Mundt F. Factoextra: Extract and Visualize the Results of Multivariate Data Analyses (2020). Available online at: https://cran.r-project.org/web/packages/factoextra/index.html (Accessed April 8, 2024).
30. Andrews SS, Karlen DL, Mitchell JP. A comparison of soil quality indexing methods for vegetable production systems in Northern California. Agric Ecosyst Environ. (2002) 90:25–45. doi: 10.1016/S0167-8809(01)00174-8
31. Wickham H, Averick M, Bryan J, Chang W, D’ L, Mcgowan A, et al. Welcome to the tidyverse. J Open Source Softw. (2019) 4:1686. doi: 10.21105/JOSS.01686
32. Quispe SS, Dávalos KM, Sangay-Tucto S, de la Cruz RCC. Uso de coberturas vegetales en el manejo sostenible del suelo asociado al cultivo de maíz amiláceo (Zea mays L.). Sci Agropecuaria. (2021) 12:329–36. doi: 10.17268/sci.agropecu.2021.036
33. Mosquera VHB, Delgado JA, Alwang JR, López LOE, Ayala YEC, Andrade JMD, et al. Conservation agriculture increases yields and economic returns of potato, forage, and grain systems of the andes. Agron J. (2019) 111:2747–53. doi: 10.2134/agronj2019.04.0280
34. Jiban S, Subash S, Prasad TK, Amit C, Manoj K, Subina T. Conservation agriculture as an approach towards sustainable crop production : A Review. Farm Manage. (2020) 5. doi: 10.31830/2456-8724.2020.002
35. Yu T, Mahe L, Li Y, Wei X, Deng X, Zhang D. Benefits of crop rotation on climate resilience and its prospects in China. Agronomy. (2022) 12:436. doi: 10.3390/AGRONOMY12020436
36. Havlin JL, Tisdale SL, Nelson WL, Beaton JD. Soil Fertility and Fertilizers: An Introduction to Nutrient Management. 8th ed. Upper Saddle River (NJ): Pearson Education (2013). p. 528.
37. Dvořáčková H, Dvořáček J, Hueso González P, Vlček V. Effect of different soil amendments on soil buffering capacity. PloS One (2022) 17(2):e0263456.
38. Othaman NNC, Isa MNM, Ismail RC, Ahmad MI, Hui CK. Factors that affect soil electrical conductivity (EC) based system for smart farming application. AIP Conf Proc. (2020) 2203. doi: 10.1063/1.5142147/961411
39. Sá MM, Schaefer CE, Bockheim JG, Loureiro DC, Michel RF, Simas FN. Genesis of a holocene soil chronosequence from the southernmost andes mountains, tierra del fuego. Catena (2018) 162:291–302.
40. Havlin JL, Tisdale SL, Nelson WL, Beaton JD. Soil fertility and fertilizers. Pearson Education India (2016) 8.
41. Yábar Villanueva EF. Evolución de glucosinolatos, compuestos fenólicos y β-sitosterol, en tres ecotipos de maca (Lepidium meyennii Walp.) durante la pre y post-cosecha. Lima: Universidad Nacional Agraria La Molina (2017). Available online at: http://repositorio.lamolina.edu.pe/handle/20.500.12996/3128.
42. Miękus N, Marszałek K, Podlacha M, Iqbal A, Puchalski C, Świergiel AH. Health benefits of plant-derived sulfur compounds, glucosinolates, and organosulfur compounds. Molecules (2020) 25(17):3804.
43. Padbhushan R, Kumar D. Fractions of soil boron: a review. J Agric Sci. (2017) 155:1023–32. doi: 10.1017/S0021859617000181
44. Mal S, Sarkar D, Mandal B, Basak P, Kundu R, Ghosh D, et al. Determination of Critical Concentrations of Boron in Soils and Leaves of Tomato (Lycopersicon esculentum L.) using Polynomial Equation. J Soil Sci Plant Nutr. (2023) 23:4055–65. doi: 10.1007/s42729-023-01323-2
45. Basak P, Sarkar D, Mandal B, Mal S, Adhikary S, Kundu R, et al. Determination of Critical Concentrations of Boron in Soils and Plants of Cauliflower (Brassica oleracea var. botrytis L.) Using a Polynomial Equation. Commun Soil Sci Plant Anal. (2022) 53:2388–99. doi: 10.1080/00103624.2022.2071444
46. Khalid D, Saad D, Kacem M, Fatima Ezzahra N, Fouad A, Abdelhadi AH. Sunflower response to Boron supply when grown in a silty clay soil. J Saudi Soc Agric Sci. Amsterdam: Elsevier. (2020) 19:81–6. doi: 10.1016/j.jssas.2018.06.004
47. Minich DM, Ross K, Frame J, Fahoum M, Warner W, Meissner HO. Not all maca is created equal: A review of colors, nutrition, phytochemicals, and clinical uses. Nutrients. (2024) 16:530. doi: 10.3390/nu16040530
48. Cakmak I, Brown P, Colmenero-Flores JM, Husted S, Kutman BY, Nikolic M, et al. Micronutrients. In: Marschner's mineral nutrition of plants. Academic Press (2023). p. 283–385.
49. Yan L, Li S, Cheng J, Zhang Y, Jiang C. Boron-mediated lignin metabolism in response to aluminum toxicity in citrus (Poncirus trifoliata (L.) Raf.) root. Plant Physiol Biochem. (2022) 185:1–12. doi: 10.1016/j.plaphy.2022.05.018
Keywords: maca, high Andean soils, monoculture, fallows, soil quality index
Citation: Solórzano-Acosta R, Chanco M, Seminario M, Cabello-Torres R, Camel V, Lastra S, Arias A, Verástegui P, Quispe K, Carbajal-Llosa C, Cuevas JP, Cruz J and Turín C (2025) Soil management in Lepidium meyenii (maca) monoculture: trends and challenges for small farmers around Lake Chinchaycocha in the Andean highlands of Junin (Peru). Front. Soil Sci. 4:1419745. doi: 10.3389/fsoil.2024.1419745
Received: 18 April 2024; Accepted: 06 November 2024;
Published: 17 January 2025.
Edited by:
Sunita K. Meena, Dr. Rajendra Prasad Central Agricultural University, IndiaReviewed by:
Serenella Nardi, University of Padua, ItalyEric G. Cosio, Pontifical Catholic University of Peru, Peru
Copyright © 2025 Solórzano-Acosta, Chanco, Seminario, Cabello-Torres, Camel, Lastra, Arias, Verástegui, Quispe, Carbajal-Llosa, Cuevas, Cruz and Turín. This is an open-access article distributed under the terms of the Creative Commons Attribution License (CC BY). The use, distribution or reproduction in other forums is permitted, provided the original author(s) and the copyright owner(s) are credited and that the original publication in this journal is cited, in accordance with accepted academic practice. No use, distribution or reproduction is permitted which does not comply with these terms.
*Correspondence: Richard Solórzano-Acosta, aW52ZXN0aWdhY2lvbl9sYWJzYWZAaW5pYS5nb2IucGU=
†ORCID: Richard Solórzano-Acosta, orcid.org/0000-0003-1629-2549
Mirella Chanco, https://orcid.org/0000-0001-7437-7722
Martín Seminario, https://orcid.org/0000-0002-1102-3182
Rita J. Cabello-Torres, https://orcid.org/0000-0002-9965-9678
Vladimir Camel, https://orcid.org/0000-0002-3618-8215
Sphyros Lastra, https://orcid.org/0000-0001-9017-0852
Alberto Arias, https://orcid.org/0000-0002-6055-8722
Patricia Verástegui, https://orcid.org/0000-0002-2610-2713
Kenyi Quispe, https://orcid.org/0009-0002-5748-9292
Carlos Carbajal-Llosa, https://orcid.org/0000-0002-1638-8076
Juan Pablo Cuevas, https://orcid.org/0000-0003-0669-6873
Juancarlos Cruz, https://orcid.org/0000-0003-1169-440X
Cecilia Turín, https://orcid.org/0000-0003-2597-7056