- 1Department of Agronomy and Ecological Management, Faculty of Agriculture and Natural Resources Management, Enugu State University of Science and Technology, Enugu, Nigeria
- 2Economic Community of West African States (ECOWAS) Commission on Private Sector Development, Abuja, Nigeria
The concept of a circular bioeconomy focuses on the sustainable use of biological resources, minimizing waste and negative environmental impacts. Soil ecosystem services are crucial in this context as they support agricultural production, biodiversity conservation, and nutrient recycling. The circular bioeconomy offers benefits like resource efficiency, reduced waste, lower environmental impacts, and economic opportunities, with soil ecosystem services playing a significant role in achieving these benefits. Soil provides various services for human well-being, including security, protection from ecological shocks, access to balanced diets, clean water, clean air, and energy for temperature control. This review highlights the importance of soil ecosystem services in circular resource management and bio-based sustainable production systems. These services encompass provisioning, regulating, cultural, and supporting roles, providing resources like food, fibre, and fuel, controlling erosion and temperature, offering aesthetic value, and sustaining plant and animal diversity. The bioeconomy comprises knowledge, research, technology, and innovation related to biological resource production, use, conservation, and regeneration. The application of circular bioeconomy strategies benefits from the ecological services soil provides to bio-based industries. The policy that converts farming, grazing, and woodland systems into renewable operations is bound to protect soil functions while relieving pressure on other critical ecosystem functions. Overall, a holistic understanding of soil ecosystem services is crucial for successfully implementing circular practices across different bioeconomy sectors. Soil conservation, sustainable management, and the protection of soil resources are vital for maintaining the services that support a circular bioeconomy.
1 Introduction
Soils are diverse ecosystems comprising living and nonliving elements that interact in various ways (1) It is greatly concerned with nutrient cycling, pore creation, and pore structure stabilization. Organisms in the soil congregate at the upper soil horizon, generally the top 2-3 cm, which contains most organic matter. Other creatures may be found many kilometres under the soil’s surface (2) Some earthworms (Lumbricus terrestris) have been observed to burrow as deep as 3 meters (300 cm) in some cases (3). Soil organisms are vital in soil fertility, structure, drainage, and aeration. They vary in size from minuscule cells that consume organic matter in decomposition to small animals that predominantly eat other soil creatures. They also break down tissues from animals and plants, liberating essential elements and transforming them into forms needed by plants (4). Soils provide an environment for seeds to grow. They make heat, nutrients, and water available to help plants grow to maturity. These plants get together with other plants and organisms to create an ecosystem. Ecosystems offer a diverse range of resources and processes that benefit society. These advantages are known as ecosystem services, and they emerge from the interactions of microorganisms, animals and plants with the environment (5). The variety of species and functional groupings in soil determines its multifunctionality (6). Soil ecosystems comprise intricate connections between living things with their surroundings (7). They are distinguished by the diversity of their vegetation, interactions between biotic and abiotic processes, and climatic and soil conditions. The advantages people gain from soil ecological systems are known as “soil ecosystem services”. A human-centred perspective on nature distinguishes an ecosystem service approach to land management and public policy decision-making. It comprehensively covers all of the advantages people derive from the soil ecosystem. It is set up so that market-oriented and non-market ecosystem services can be given a monetary or non-monetary value. To understand the historical significance of soil to people, consider how the loss of soil fertility led to the downfall of past civilizations (8).
1.1 Soils provide ecosystem services
The ecosystem services (ES) idea is a helpful communication tool emphasizing how much ecosystems depend on humans for their well-being. Sustainable resource management can close the gaps between ecology, economics, and society (9). According to Braat and de Groot (9), the most current definition of ecosystem services identifies ecosystem services as the direct and indirect benefits of ecosystems to human well-being. The idea of ecosystem services was introduced in the early 1980s to increase public awareness of the negative consequences of biological diversity loss on human well-being Ehrlich and Ehrlich (10); Mooney et al. (11). Soil ecosystem services are fundamental to human well-being. The soil is the primary source of material for a comfortable existence and societal relationships. The essential component of pleasant life and social connections are facilitated by soil. Additionally, it promotes security, freedom of activity, health, and safety (12). The qualities of the soil govern its functions, which in turn regulate ecosystem services and human well-being. The soil organic matter content, the distribution of soil particle sizes, coarse soil fragments, soil acidity, soil densification, characteristics of soil water retention, CEC, soil water conductivity, distribution of soil pores and air permeability, soil water transmissivity, soil organisms, the structure of the soil and soil heat, soil clay minerals, and soil compatibility, among others, regulate soil functions. The MEA (13) defined ecosystem services as the rewards that ecosystems bring to people, which help make human existence feasible and worthwhile.
1.2 Components of human well-being facilitated by soils
Human well-being is partially, but not entirely, dependent on soil ecosystem services. Although the top-level categories are broad, the sub-elements are specific to the role of soil ecosystem services (14). Soil ecosystem services define the activities and functions offered by nature that humans rely on for their well-being. Soil ecosystem services provide protection, a secure environment, and resilience to ecological shocks like droughts, floods, and pests. They enable humans to recover from changes and recover from pedological time (15). Short-term human soil management approaches are unsustainable, threatening human well-being and potentially armed conflict. Environmental demands impact soil processes and functions, potentially affecting well-being (16). Ecosystem impairments, including wetland depletion, biodiversity loss, freshwater pollution, gentrification, and climate change, directly or indirectly impact human and animal health through land degradation and desertification (17). Floods, heat waves, water shortages, landslides, pollution impact human well-being, ecosystems, and affect infectious diseases, agricultural yields, and creative taste (18). The varied effects of lost livelihoods, population displacement, conflicts, and improper adaptation and mitigation are additional indirect, postponed, and displaced health impacts (19). Essential ecological services, such as sustainable livelihood resources and purchasing ability, are crucial for human well-being, with perceptions of insecurity and risk influencing decisions (20). Soil is essential for human well-being, providing food, fuel, energy, water, and other necessities. It preserves healthy ecosystems, ensuring balanced food, illness prevention, clean water, air, and energy. Soil’s state determines its ecosystem’s function (21). Soils facilitate social interactions, promoting artistic and leisure ethics, expressing cultural importance, building social capital, and avoiding stress and conflict, promoting human well-being. Ecosystem services impact liberty and choice, with socioeconomic conditions influencing ecosystem transformation and human welfare (22).
1.3 Soil functions and ecosystem services
The essential ecological services that soil provides enable life on Earth (Figure 1). Soils sustain human life through agriculture, medicine, water filtration, and the provision of basic building materials. Through the cycle of soil carbon, soils control climate. Soils enrich culture through landscapes and a sense of location in our daily life (24). Soil functions are directly related to ecosystem services (25). Soils house a biodiversity pool, which includes ecosystems, species, and genes. Soils directly facilitate the production of biomass, including that from agriculture and forestry. The soil stores, filter and transform nutrients, substances, and water. Soils are raw material sources, serve as a carbon sink, and are the repository of prehistoric and geological artefacts. For human life, soils are essential because they deliver and regulate various ecosystem services (ES). Cleaner air, freshwater, and agricultural production are only a few of the benefits of soil; they are also essential for reducing poverty and combating climate change. These represent some of the most urgent problems facing our civilization. The same environmental factors that will define soil properties and functions determine the type, quantity, and quality of soil ES (26). The distinct categories of soil services provided by ecosystems were recognized by the MEA (13): provisioning services, i.e., material goods including fibre, fuel, food, feed and maintenance services. Regulating services (such as moderating temperature, controlling flooding, and filtering water); The basis of all ecosystems and their services is cultural services, which include recreational, spiritual, and aesthetic benefits, as well as supporting services, which include habitat for living things and preserving a variety of them (23, 27) (Figure 2).
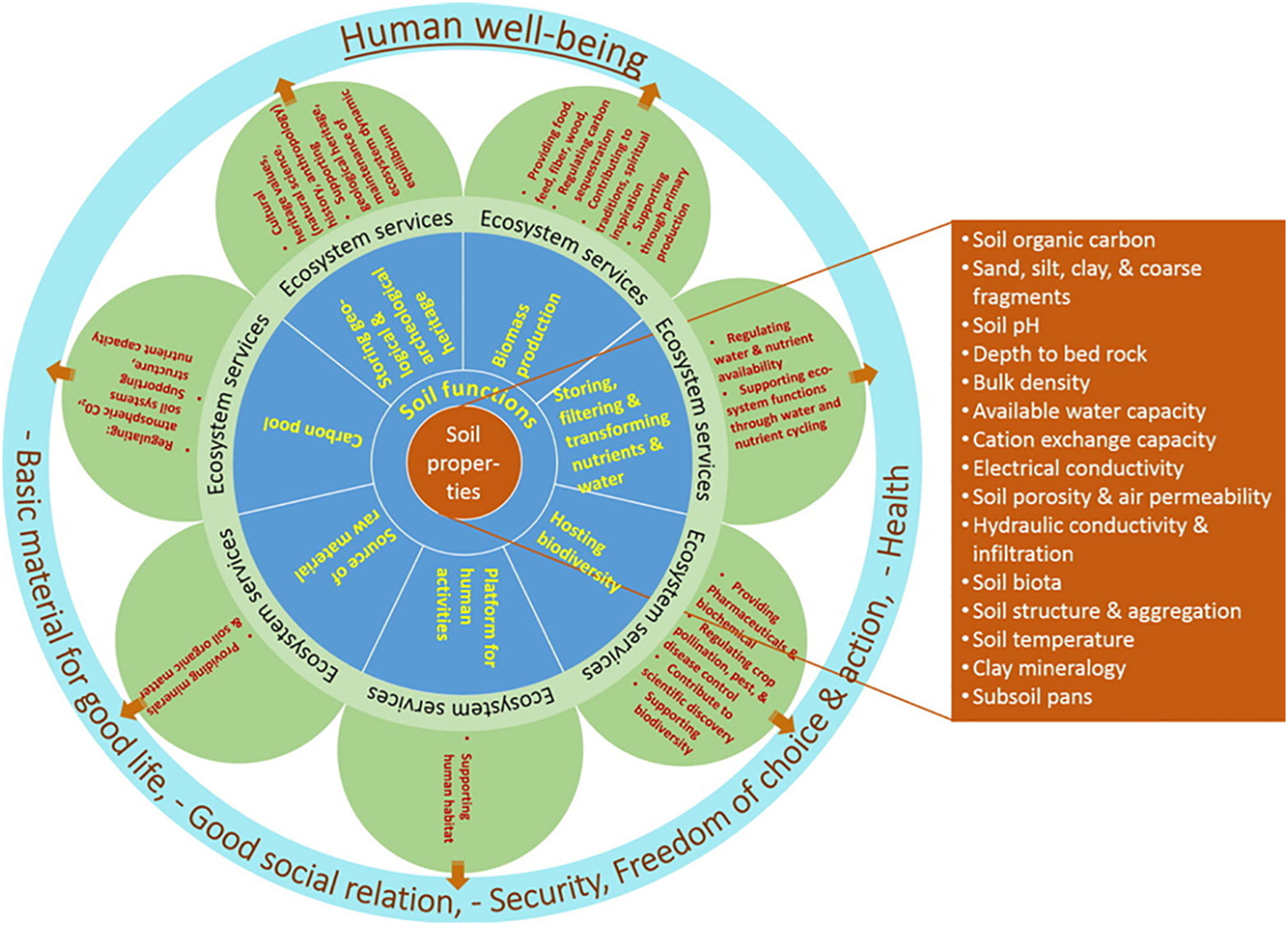
Figure 1 Linking soils to ecosystem services- Source (23).
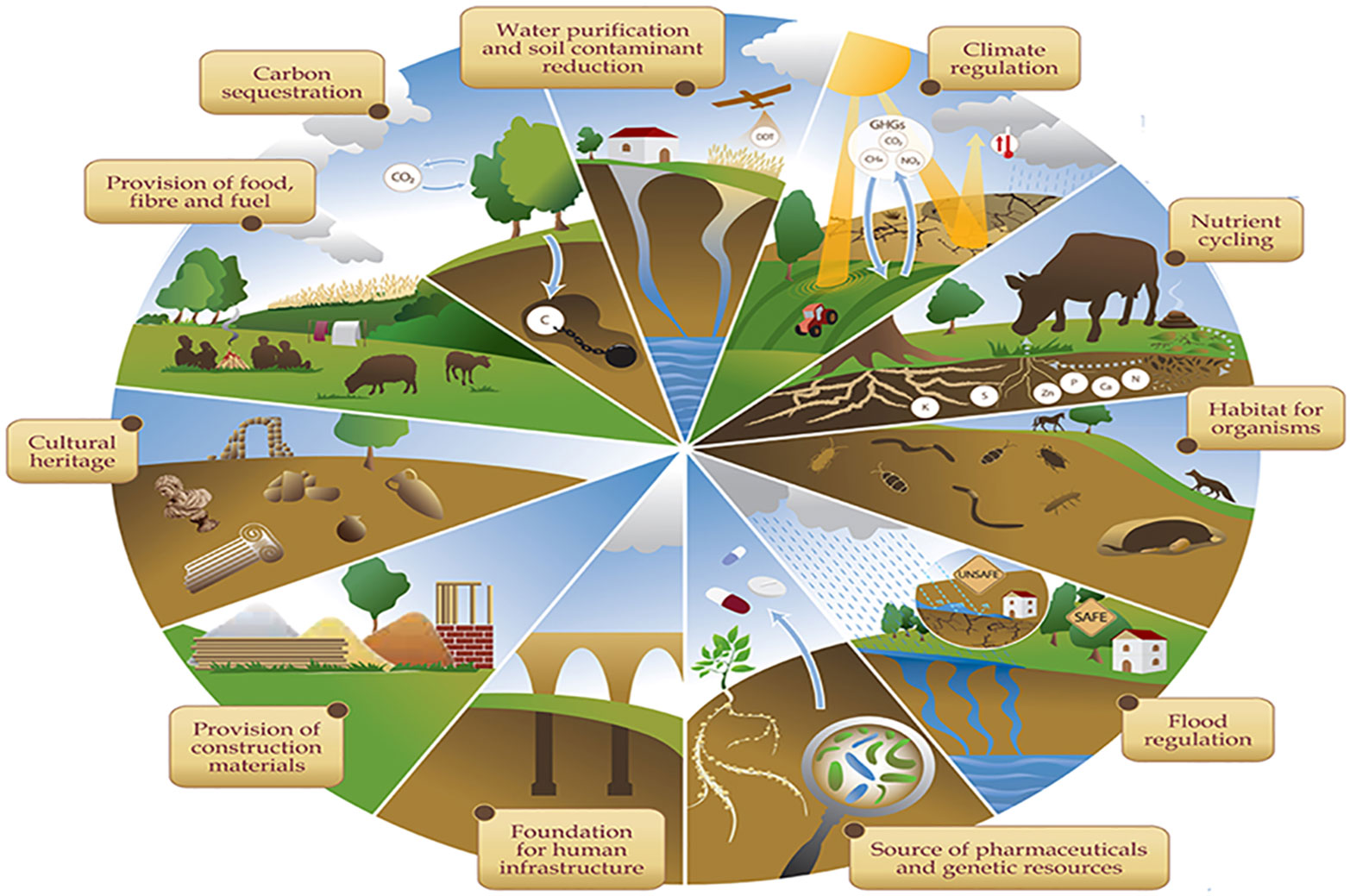
Figure 2 Ecosystem services provided by soils. (Adapted from http://www.fao.org/resources/infographics/infographics-details/en/c/284478/) (27).
1.3.1 Provisioning services
The soil ecosystem provides the conditions for growing, collecting, hunting and harvesting food. Provisioning services are goods (products) people use and are derived directly from soil ecosystems (28, 29). Soil ecological services provide essential goods like food, fodder, fiber, energy, biochemical compounds, genomic resources, fresh water, and medicines. These tangible goods can be purchased or taken from soil ecosystems, which are limited resources that can be regenerated (30). Soil is essential for all living things, providing food, water, shelter, and building materials for plants and animals (31). Since many animals eat plants as their primary food source, soil also serves as a source of sustenance for animals. Plants purify air, essential for animal existence, and soil provides essential nutrients for human nutrition, enabling poor people to create nutrient-rich animal food (32). The soil hosts ruminant animals that could breakdown the roughage in plants and change it to proteins eaten by humans as one way to produce food on vast rangelands unsuitable for growing crops (33, 34). Soil is crucial for aquaculture, providing aquatic conditions for half of human fish consumption. Carbon particles and humic and fulvic acids protect fish and shrimp, while humates break down minerals, improving water penetration and root zone health. Improved root development enhances soil’s nutrient holding capacity, supporting healthy ecosystems, providing economic diversification and safety nets (35). Medicinal plants treat various diseases, aid in drought, and boost income for poorer populations by selling wild fruits, firewood, and charcoal, and consuming them directly (36, 37). Freshwater ecosystems, including wetlands, rivers, mangroves, and aquifers, play a crucial role in reducing floods and droughts (38). Soil ecosystems supply, purify, and protect freshwater resources, managing water flows and storing water. Irrigation accounts for 60% of withdrawals, and cropping technologies help retain water. Livestock and grasslands contribute to soil ecology (39). The provision of freshwater by aquatic ecosystems can be supported through sustainable fisheries management and aquaculture development (40). Sustainable freshwater management requires incorporating fisheries and fish management, while soils support forests and provide physical support (41, 42). The soils sustain forests, maintaining healthy aquatic ecologies and reliably providing contaminant-free freshwater (43). Furthermore, to filtering and purifying water, forests also assist in preventing soil erosion, lessen reservoir sedimentation, and reduce the likelihood of mudslides, floods, and other issues that could endanger downstream water supplies (44, 45).
1.3.2 Regulating services
Soil ecosystem services involve regulating processes that impact environmental matrices, promoting plant growth, controlling pests, purifying water, degrading pollutants, regulating climate, and controlling erosion, affecting various aspects of ecosystems (45). Soil ecosystem services regulate water, energy, nutrient uptake, carbon transfer, and environmental conditions, impacting human health, safety, and comfort (46, 47). Soil ecosystem services involve water storage and recycling, enabling plants and organisms to utilize conserved water, enhancing land productivity and soil health (48, 49). Soil regulates water infiltration, storage, and control, with recharge occurring through groundwater recharge, adding to the groundwater pool. The quantity and quality of water that reaches aquifers are governed by groundwater recharge zones (50). Therefore, the main focus of pollution prevention and supply maintenance for drinking water and ecosystems is recharging zones (51). Recharge can assist in transporting extra salts that build up in the root zone into the groundwater system or deeper soil layers. Tree roots can reduce water runoff by increasing groundwater saturation (52). Nutrient cycling is crucial for soil ecosystem function, as it involves the metabolism of nutrients, storage, and release. This process allows nutrients to be stored, converted, or released into the atmosphere or water (53). The direct conversion of various sulphur, phosphorus, and nitrogen forms, which are linked to the cycling of carbon, maintains the flux and availability of nutrients required for plant development (54). The breakdown of organic materials, that regulates the release of essential elements and is crucial for soil structural preservation and the maintenance of the quality of the soil for plant development, is mediated by microorganisms (55). Microorganisms aid in remediation by decomposing contaminants, immobilizing heavy metals, and improving soil quality, while also reducing environmental carbon emissions through respiration and methane synthesis (56). Soil absorption capacity refers to its ability to hold dissolved substances in solution and interact with solid phase. It can be classified into mechanical, physical, physical-chemical, chemical, and biological categories based on absorption mechanism (57). It preserves aluminosilicate and organic particle suspensions, colloidally dispersed compounds, colloidal fractions, and weakly soluble nutrients and ameliorants. The degree of mechanical absorption is affected by soil porosity, pore size, and material dispersion (58). Organic materials and organisms aid water absorption and dispersion (59). Clayey and loamy soils have higher mechanical absorption capacity, promote plant growth, and maintain ecosystem balance through increased forest and plant community density (60). Soil filters, buffers, and hosts diverse biodiversity, promoting plant species’ flourishing and reducing pollutants.
1.3.3 Cultural services
Soil is a diverse environment crucial for terrestrial ecosystems, providing numerous non-material benefits and services (61). Soil biodiversity offers cultural and ecological services, including leisure, interactions, belonging, intellectual advancement, introspection, and artistic enjoyment. These services highlight the value of nature in cultures and health and include activities like harvesting herbs and watching wildlife (62). Outdoor activity offers health benefits and contributes to nature tourism. Cultural and environmental benefits are challenging to estimate and evaluate, but academics believe they are essential for preserving ecosystems (61). Emphasis on social indicators like artistic endeavours, design, clothing, nature tourism, and outdoor recreation for health benefits makes these concepts marketable (63, 64). Soil ecosystems preserve and prevent the degradation of artefacts and built structures from past human use, serving as a natural and cultural archive (65). Soil can be a natural phenomenon with unique structures or protection from destruction by humans or processes (66). Soils reliably perform many essential ecological functions when they are in good condition. The preservation of antiquity provides numerous examples Costantini (67) highlights the importance of a cultural or natural heritage inventory, including paleosols, earth sciences, soil classification, pedological development models, ecological significance, historical and cultural significance, and social and economic importance. Paleosols represent past environments, earth sciences, soil classification, and pedological development models. The inventory could include preservation methods for pedosites (68). Soil archive function depends on soil characteristics such as soil depth, acidity, texture, moisture content, water saturation, permeability, base saturation, nutrients, etc. These factors protect cultural heritage from degradation/mineralization (67). Soil features preserve historical legacy and act as cultural and natural archives. However, land use change challenges these services, promoting degradation and oxidation. Humans indirectly benefit from learning new knowledge and admiring cultural artefacts (69).
1.3.4 Supporting services
Soils provide supporting services for ecosystems, including habitation areas, preserving plant and animal diversity, and providing nutrients, cycles, primary production, soil formation, and habitat provision. They also offer additional amenities like food, flood mitigation, and water filtration. Soil aids in primary production through photosynthesis, which produces atmospheric oxygen through plants, algae, and cyanobacteria (70). The soil mediates these and other activities, such as chemosynthesis, which employs the oxidation of chemical compounds as an energy source (71). Soils play a crucial role in global warming by managing the CO2 budget, primarily through net primary production for food, fuel, and feed (72). Soils impact air quality, plant growth, and soil pollution; human activity and global warming impact discharge patterns; reducing erosion and nutrient losses is crucial (73). Soil formation and retention are also critical natural and manmade processes. Microorganisms and physical processes break down organic matter into tiny particles to make soil (74). Soils mediate the recycling of nitrogen and phosphate, while water circulates between oceans, the atmosphere, and the land. Soils support biodiversity, ecosystem services, and habitats, influencing ecosystem functions and promoting mutual advantages or compromises (75). Biodiversity hotspots are habitats with high species diversity, providing biologically diverse environments for agroecosystems. These systems replicate natural ecosystems, ensuring large yields and long-term production and establishing regionally suitable genotypes (76). Soils preserve ecosystem variety, but land use, climate, and natural disturbances impact soil organisms. Human activities like mining, agriculture, and wildfires can harm soil biodiversity, affecting decomposition, nutrient cycling, and water penetration capacity (77). Climate change may alter soil ecosystems, affecting plant and organic matter degradation, food, water, flood mitigation, and climate management. Understanding negative impacts and changing behaviour is crucial for future sustainability (78).
The concept of a circular bioeconomy has emerged as a promising framework for addressing the challenges associated with resource depletion, waste generation, and environmental degradation (79). It centres around the sustainable use of biological resources to produce goods and services while minimizing negative impacts. By closing resource loops, reducing reliance on non-renewable resources, and adopting a holistic and regenerative approach, the circular bioeconomy offers immense potential to transition towards a more sustainable and resilient economy. The circular bioeconomy recognizes the fundamental importance of soil ecosystems in achieving its objectives. As a complex and dynamic ecosystem, soil is the foundation for agricultural production, biodiversity conservation, and nutrient recycling. It supports the growth of plants, which in turn provide essential resources such as food, fibre, and energy for human societies. Understanding the significance of soil ecosystem services in the context of the circular bioeconomy is pivotal for developing effective strategies and policies for sustainable resource management. By highlighting the interconnectedness between soil ecosystem services and the circular bioeconomy, this review aims to contribute to the body of knowledge on sustainable production and consumption systems.
Based on the detailed literature survey, some of the most critical questions relating to the role of soil ecosystem services in the circular bioeconomy are 1. What are the specific soil ecosystem services that contribute to the functioning of a circular bioeconomy? 2. What potential benefits can be derived from integrating soil ecosystem services within the circular bioeconomy, such as resource efficiency, waste reduction, environmental sustainability, and economic opportunities? 3. How do soil ecosystem services contribute to achieving these benefits, and what are the mechanisms and processes involved? 4. What are the implications of soil ecosystem services for bio-based industries in the circular bioeconomy? By addressing these research questions, this review aims to provide an understanding of the role of soil ecosystem services within the circular bioeconomy framework. It seeks to contribute to the body of knowledge in developing sustainable and regenerative production and consumption systems. The other objectives of the review are to provide an understanding of the circular bioeconomy concept and its significance in resource management; To explore the role of soil ecosystem services in supporting and advancing the goals of the circular bioeconomy. To identify the potential benefits of the circular bioeconomy regarding resource efficiency, waste reduction, environmental impacts, and economic opportunities. To examine the specific contributions of soil ecosystem services to achieving these benefits within the circular bioeconomy framework. To evaluate the implications of soil ecosystem services for bio-based industries in the context of the circular bioeconomy. To contribute to the body of knowledge on sustainable production and consumption systems by elucidating the relationship between soil ecosystem services and the circular bioeconomy.
2 Circular bioeconomy
The bioeconomy refers to creating, exploiting, preserving, and rejuvenating natural assets and accompanying knowledge, technological advances, and creative thinking (80). This allows for the creation of sustainable solutions (information, goods, and services) in all economic sectors and the transition to a maintainable economy (81). A circular biobased economy offers a theoretical context for reorganizing and controlling our soil resources, food, well-being, and manufacturing industries to attain sustainable well-being in harmony with nature (82). Customer requirements, technical improvements, environmental challenges, responses, and changes in access to resources and situations are driving the economy’s growth. International discussions, business and science and technology efforts, and the confluence of nanotechnology, biological technology, technological innovation, and cognition drive these elements (83). While the circular bioeconomy requires advanced technology, innovation, and traditional wisdom, its primary engine is biodiversity (Figure 3). Because biodiversity impacts biological systems’ ability to adapt and change in a changing environment, it is critical to guarantee our biological resources’ resilience and sustainability (84). We must recognize this critical role through appropriate conservation policies and locally customized market-based instruments that incentivize farmers, forest owners, and biobased businesses to engage in biodiversity (85). The economy’s cross-cutting nature provides a once-in-a-lifetime chance to address interconnected societal concerns such as food and nutrition security, fossil fuel reliance, natural resource scarcity, climate change etc., while attaining sustainable economic development. The critical need to transform the global economy into an increasingly ecologically sound, environmentally friendly system that relies on natural assets that require fewer resources or do not utilize fossil fuels, known as the bioeconomy, has been acknowledged worldwide. The bioeconomy entails harnessing natural assets, methods, and values to produce goods and offer solutions across every facet of the economy. Agriculture, forests, fishing, food, paper, textiles, engineering and construction, science, information and communication technology, and medicines are all economic areas. Biological materials, bioplastics, implantation devices, organic materials for construction, sweetener replacements, natural medicinal products, and biofuels are some everyday goods made from microbes.
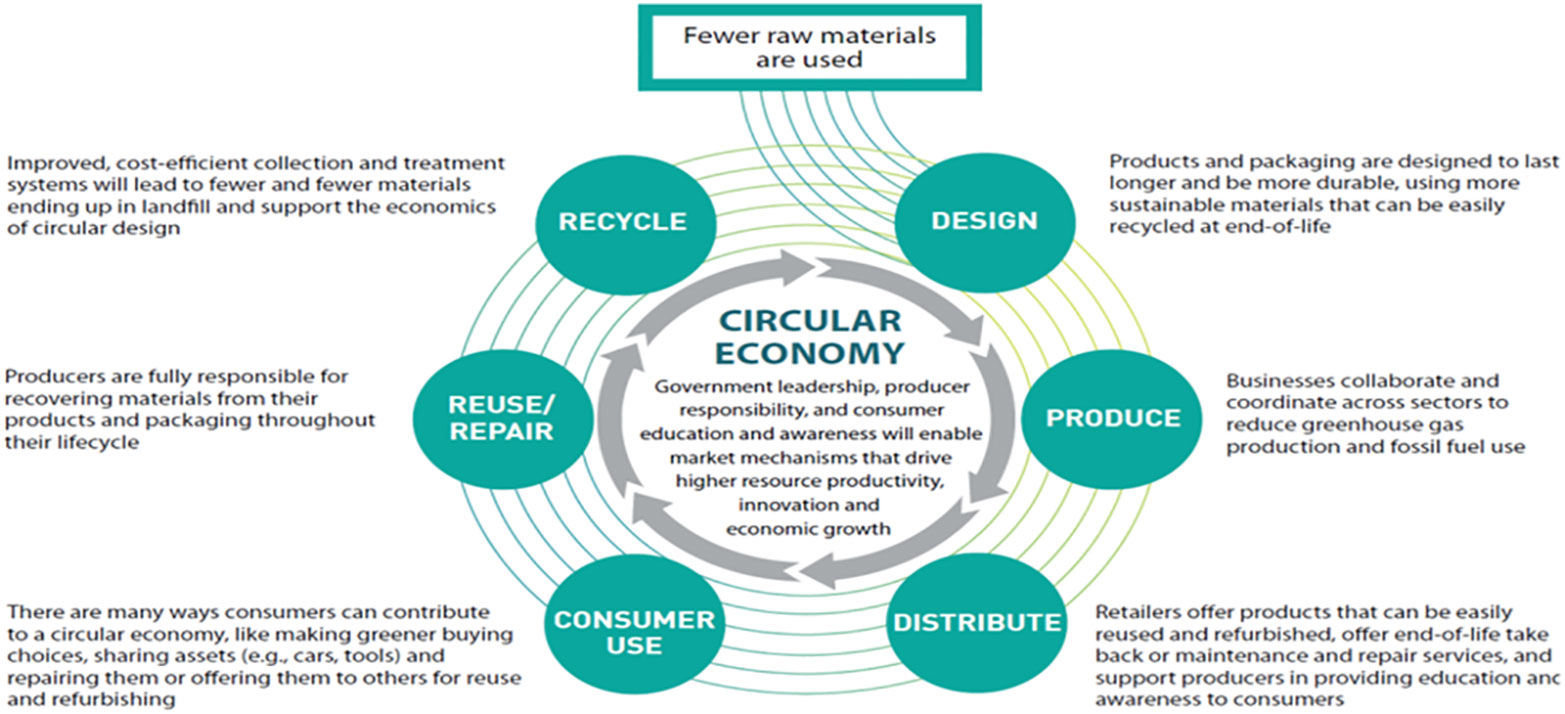
Figure 3 Material Flow in a Zero-Waste City. Source: Ontario’s Strategy for a Waste-Free Ontario: Building the Circular Economy (80).
Bio-refineries (which convert biomass into chemicals, precision farming, regulated-environment agribusiness, renewable aquatic biological resources, and carbon dioxide as the manufacturing substrate of the modern era and the recovery of phosphor from wastewater are a few examples of industrial operations. Seaweed panelling for electricity, hardwood skyscrapers, and environmentally friendly and reusable facades are examples of biobased technologies for urban transformation. Bio-based batteries and DNA-based computing devices are examples of technologically advanced bioeconomic approaches. Circular bioeconomics aims to eradicate poverty and hunger while improving quality of life, equality between the sexes, access to sanitation and clean water, energy production, employment opportunities, and economic development. Other focus areas include industry, innovation, infrastructure, lowered inequality, ecologically sound cities, conscientious consumption, and environmental protection. More than fifty nations have already made promoting a bioeconomy a top priority. Several countries have developed specific bioeconomy plans and programmes. The transformation of productive sectors is frequently credited to the interaction of the bioeconomy and digitization. In several nations throughout the globe, bioeconomy plans have been embraced. Care of soils which preserves their properties and prevents soil deterioration is necessary to ensure their sustained use. The maintenance of ecological services and practical resource usage is only possible after that (86).
The idea of ecosystem services has garnered more attention in recent years, yet, its relationship to soil functions and management methods is still poorly defined. The roles of soil are essential to produce food, reduce greenhouse gas emissions, and provide additional ecological benefits (87). Agricultural management prioritizes productivity above other roles performed by soils, such as water refinement, carbon storage, biodiversity, nutrient and (agro)chemical reprocessing (88). Many factors contribute to the imbalance in soil functions. In addition to food, bioeconomy plans aim to increase the agricultural output of nonfood items such as energy and materials to replace fossil resources. Long-term fertility and other social goals need a healthy balance of soil services while reducing degradation. To avoid endangering resource-saving aims, biomass production must comply with resource utilization efficiency principles (89).
2.1 Soil ecosystem services and circular bioeconomy
In the past few years, the circular economy concept has acquired prominence as an entrepreneurial model. It solves many of the world’s problems with sustainability by separating economic growth and spending. In a circular economy, value chains constitute closed cycles whereby goods intended to be discarded are repurposed and reprocessed.
The circular economy is founded on the fundamental principles of reusing products and materials, eliminating waste and pollution from the system, and recreating natural systems (90). This reduces resource consumption, waste, and pollution while generating new value from items previously considered garbage. Developing a circular economy can also add value by creating new industries and jobs. The ecosystem provides services that keep our civilizations running. The environment controls the climatic conditions and stores carbon, manages to flood then generates water stored underground while similarly serving as a significant source of happiness and enjoyment (91). Nature must be diversified to function correctly. Soil ecosystem services, or the structures and functions of soil ecosystems, are needed to sustain bioeconomy, health, and wellness. Soil ecosystem services provide an opportunity for various businesses in industries such as tourism, culture, and health and wellness. A vital component of the bioeconomy is the development and commercialization of sustainable solutions. Such solutions may include recognizing and obtaining broader access to soil ecosystem services.
Regarding building, decentralized energy production, and water and landscape management, soil ecosystem services present new commercial prospects (for instance, incorporating greenery into environmentally conscious neighbourhood design (92). Soil ecosystem services are valuable for the execution of circular bioeconomy policies. Bioeconomic activities provided by soil ecosystem services are diverse. The economic activities offered by soil ecosystem services include agriculture, aquaculture and fisheries, Industry sectors that could benefit from this technology include the biochemical sector, building, food, pharmaceuticals, forest products, tourist attractions, paper and pulp, clean energy, biodegradable materials, water filtration, and wood-based goods sector.
2.1.1 Circular agriculture, food industry and soil ecosystem services
Soil ecosystem services are essential to all areas of life because they support the creation of ecosystem products and resources like energy supplies, water storage and purification etc. The environmental, social, and economic implications of the modern food production system’s “linear” nature are enormous. Without adjustments associated with the present untenable feeding habits, CO2 emissions might double by 2050. Circular agriculture is a method of farming that is environmentally friendly while utilizing scientific discoveries, innovations, and new technologies (93). Circular agriculture prioritizes plant-livestock diversity, sustainable cultivation, reusing water, as well as effluent recycling. Increasing food demands have restricted agriculture as an ecosystem, leading to the degradation of other services. Ecological services refer to the benefits natural ecosystems regularly give that are appreciated commercially. Small-scale, labour-intensive agriculture is suited to circular agriculture methods, promoting gender-inclusive local economic growth. The main interlinked issues associated with farming methods are water shortages, fertilizer overload, declining biodiversity, marine overfishing, global warming, and ecosystem transformation. Global partnerships and benefits, including water user groups, secure resource access, and property rights, may promote implementing circular farming practices. Daily (94) classified these services into pest control, conservation of biodiversity, protection from UV rays, climate stabilization, taming of extreme weather conditions, cleaning water and air purification, disaster mitigation, soil generation and conservation, controlling pests, safeguarding soil, visual appeal, and stimulation of thought. These categories are related to agriculture and are mediated by the soils, either directly or indirectly. Agriculture depends on the soil for inputs, many of which go unaccounted for and unreported. Capturing shared natural assets, including sunshine, the wind, as well as precipitation and converting them into usable basics like plants is the core goal of plant agribusiness. The agricultural sector has two separate circular cycles: technical and biotic. Agro-processing innovation that increases efficiency in farming and reduces wastage, and saves cost is an excellent example of how the technological loop relates to agriculture-industrial technologies. The natural process retrieves value from waste in the system by utilizing food scraps and waste products, regenerating nutrients, and recycling food. Trash is transformed into new products that help grow crops, produce livestock feed, process food, fuel, and the healthcare and biopharmaceutical sectors (95) Navarre et al., 2021. According to Jurgilevich (93), sustainable economy principles are now effectively implemented in farming operations, such as decreasing waste and increasing productivity. The expenditures and resource requirements for chemical fertilizers may be reduced by turning organic materials into fertilizers, such as crop twigs, foliage, pods, and animal excrement. Sewage from urban areas, villages, and farm operations may also be recycled to grow plants. The creation of bioenergy for use in generating power and fuel for vehicles may be done directly or via modified processes using feedstock from animal and plant matter.
Agriculture currently impacts the ground beneath our feet more than any other industry. A number of the farming concerns we face in this decade, such as those related to global change, the environment, food safety, and clean water, are brought on by declining soil quality. The need for action is growing stronger by the day, yet market-driven innovation has so far lagged behind the science of soil health solutions. A pioneering action via The Nature Conservancy (TNC) is designed to promote support for breakthroughs aimed at a particular problem of soil quality as investor demand for arising technological advances in agriculture merges with the possible use of technological advances for enhancing the long-term viability of world food systems (96). TNC is currently putting resources into various innovative agrotechnology to help accelerate the widespread use of sustainable agricultural practices for the advantage of agriculturalists, soil quality, and the ecosystem. Some critical domains of soil quality — the growing popularity of cover crops, use of zero-till methods, enhanced diversity of plants achieved via crop cycles, and accurate or efficient agricultural feedstock handling — have demonstrated strong scaling possibilities as remedies by some emerging privately-owned businesses. Early investments might include: 1. Growers Edge is a technology-based financial services company that guarantees agricultural business revenue based on information merchandise, remedies, and instruments. 2. Kula Bio is a low-cost biological nitrogen fertilizer that reduces run-off. 3. Pattern Ag analyses soil microbiomes and makes farm input optimization recommendations. 4. Stony Creek Colors produces indigo and other organic dyes for the fabrics, decorative, and culinary industries. 5. Swarm Farm, an autonomous, robotic farming solution, enables the practical precision application of nutrition and crop protection inputs. This programme contributes to the more significant endeavour to speed the transition to a regenerative, nature-positive industrial model. Ventures in partnership with initial-stage agricultural technology firms can help producers improve soil health management’s operational efficiency and profitability. Other manufacturing operations affiliated with ecological soil systems consist of biochemical refineries, waste from energy utilization, precision farming, fish farming, regulated-environment agriculture, and carbon dioxide as a commercial resource of the twenty-first century. These models would increase soil ecosystem functioning, boosting societal well-being and prosperity. Circular agriculture methods prioritize sustainable cultivation, plant-livestock diversity, and the reuse of water and effluent. Soil ecosystem services, such as pest control, biodiversity conservation, climate stabilization, and soil generation and conservation, play a crucial role in supporting circular agriculture.
2.1.2 Circular aquaculture, fisheries and soil ecosystem services
Soil ecosystems support natural fishing and aquaculture production worldwide. As a consequence of both aquaculture and fishing operations, a variety of environments, including forests, reef systems, swamps, and sea beds, have suffered severe damage, resulting in modifications to the habitats and, therefore, to the amenities that that they offer (97). As a result of the widespread deterioration of aquatic environments in the last few decades, attempts are being undertaken to mitigate the adverse effects of aquaculture farming activities and restore natural habitats, resources, and ecosystem functions (98). The circular economic system in fisheries uses garbage and remnants from distribution networks to create nutritious food for people. For instance, approximately 35 per cent of the seafood used in fisheries comes from scraps from the manufacturing process of fish.
Aquaculture provides year-round employment, sustains robust working waterfronts and coastal towns, and has the potential to bring long-term economic growth. Techniques for sustainable aquaculture farming emphasize food production as well as environmental management. Sophisticated technology examines “ocean communities,” such as the free Ocean Reports mapping and planning tool. It gives vital information for making informed decisions that increase sustainable aquaculture prospects while avoiding potential environmental consequences. It also includes thorough information on habitats and species, current activities in the area, and potential aquaculture dangers. The soil system is a sink for some ‘waste products’ of the aquaculture and fish industry. Mangrove habitats’ primary land uses to connect to the soil/water interface, and their support maintenance to manage them encompass lumbering, marshland species restoration and managing fish farming. Aquaculture pond establishment is the primary source of mangrove destruction, resulting in significant CO2 emissions. This loss is referred to as the land use carbon footprint, and the soil ecology enormously impacts this loss. Mangrove land conversion rates are high, and the carbon footprint of shrimp farming is also significant. A more resource-efficient technique is required to develop fish farming, provide a constant source of seafood, and not inflict calamitous soil system impairment (99). As a result, there is a rising global awareness and interest in valuing existing resources, particularly the recycling of nutrients and byproducts, to sustain a circular economy. Mulazzani and Malorgio (100) state that the blue economy is an economic model for aquatic and marine industries. Aquaculture productivity may be significantly increased by recycling waste end-products and discharges into newly constructed systems used in aquaculture (101). The handling and disposal of fish waste have an essential effect on soil ecological systems and are becoming of widespread interest. Livestock nutrition, biofuels, dieting aids, natural colours, packaging of food, beauty products, enzymatic separation, soil chemical fertilizers, and food management of moisture are just a few of the uses. Aquaculture and fishing operations can cause severe damage to aquatic environments and habitats, affecting the services they provide. Implementing circular practices in aquaculture and fishery systems can help mitigate these negative impacts. Soil systems can act as a sink for waste products from the aquaculture and fish industry and require careful management to avoid soil system degradation.
2.1.3 Circular economy of chemical industry and soil ecosystem services
The sustainable economy strives to recognize the beneficial properties of each waste item. The circular economy helps develop strategies for utilizing and reusing garbage generated during the manufacturing phases of these essential elements, which is vital given that the chemical sector employs unprocessed resources like fossil fuels, oil, and water buried and confined in soils. According to the Millennium Ecosystem Assessment (13), the plastic sector relies on petroleum-based products to stay alive in the economy of circularity. The industry wants to take advantage of what the chemical sector is doing. It is crucial to understand techniques to produce essential components using additional components so companies may utilize and recycle them. Setting up the petrochemical sector in a circular system has several benefits, including handling waste and lowering emissions (102). Due to their significance, petroleum and natural gas might not constitute “true waste,” but in a circular economy, products are given a more realistic meaning (103). The chemical industry’s capacity to produce and renew energy to move humanity in the correct direction exemplifies its cyclical nature. In simple terms, it promotes society’s economic development. The diverse range of biomass assets offers tremendous opportunities for various goods to meet different societal needs, enabling biorefining to be an essential part of the emerging bioeconomy. All plant matter, including crops, algal cells, and waste, should be grown and used ethically (104). By 2050, humanity must reach zero net carbon emissions to stop the most severe effects of global warming. This is no easy task, but one obvious remedy could be found in industrial pollutants (105). Industrial pollution is responsible for 27 per cent of worldwide carbon dioxide released into the atmosphere, behind only the energy sector. The steel industry, cement-based material, chemical compounds, and aluminium account for as much as 60 percent of these emissions from industries, which amount to 7.1 Gt carbon dioxide annually (85). Because the need for such assets is expected to increase in future decades if current trends persist, effectively reducing the carbon emissions of these goods is critical to solving the climate crisis. The concept of the circular economy may be beneficial in this effort. Five business sectors: building/infrastructure, shipping, household goods and equipment, and packaging—need regenerative economic remedies for hard-to-abate commodities, including steel, aluminium, cement-based material, and solvents. Utilizing circular economy strategies will be made possible by four key enablers: elevated product utilization, goods replacement, decreased use of materials for each item, and reusing materials for new products. These options assist in concentrating and accelerating actions being made worldwide to lessen the consequences of the changing climate (85).
Circularity has gained popularity in recent years, but many ideas have yet to be adopted on a large scale. Implementing circular solutions requires multiple players along the value chain, and individual businesses face hurdles such as a lack of materials and capability. Soils are short- to long-term carbon storage media because they contain more significant carbon quantities than the atmosphere and all plant life on earth combined (106). Plant litter and additional detritus build up in soils as organic debris and become degraded by biological and chemical weathering. More durable carbon-based fibres are found in humus, including cellulosic materials, lignin, aliphatic materials, waxy substances, and organic compounds, including terpenes, diterpenes, and sesquiterpenes. However, because of excessive heat and leaching from rains, leaf debris and humus are quickly oxidized and inadequately cared for in subtropical and tropical climate conditions. Organic material typically builds up in waste and slightly cooler-weather soils. Shifting cultivation or slash-and-burn agriculture is often only productive for a while and eventually discontinued. Rainforests are efficient at storing and moving nutrients, which explains their lushness amid a nutrient desert. Human activities have caused a global spread of harmful substances, including obstinate biological contaminants, mercury and other metals, which have been applied to top soils and discharged into groundwater (107). These substances enter the water cycle after being temporarily stored in soil (108).
Soil ecosystems play a dual role as sources and sinks for these chemical substances. As elucidated by (108), the history of modern society’s economic development can be seen in the diffuse pollution of the subsurface environment, which continues today. Seepage waters from soils absorb pollutants, which are then transferred to groundwater and carried to rivers by groundwater movement. Many substances are demonstrated to persist over very long-time scales in the subterranean environment, and contaminated soils already or may in the future serve as a source of contamination for areas that are close by, like the atmosphere and groundwater (109). Present state and future prospects for groundwater ecosystems. Collaboration amongst industries will be critical in scaling circular solutions, and channels connecting these participants and offering direction could assist team heads in closing skill slits and developing novel procedures. Policymakers have tools to aid in implementing and scaling circular solutions, such as financial incentives, market regulation, and public procurement targets (110). Together with industrial collaborations, technological advances can evaluate change. Know-how can guarantee that ideas are executed on a large gauge without being hampered by prohibitive expenditure or a lack of cost competitiveness. Leaders will build new standards and practices to make circularity a reality through collaborations between innovators, industry leaders, and policymakers. The chemical industry relies on soil resources, such as fossil fuels and oil. Implementing circular practices in the chemical sector can help reduce waste and lower emissions. Soil ecosystems play a role in storing and cycling chemical substances, which can have implications for soil and groundwater contamination.
2.1.4 Circular construction industry and soil ecosystem services
An equitable shift to a sustainable economy is essential to achieve climate targets. Following that, efficient utilization of energy and sustainable power sources can tackle more than half of the emissions. The remaining 45% must be addressed by changing how we produce and consume. The circular economic system of creating, demanding, and developing products reduces wastage and renews the environment. To put it briefly, it guarantees that we may live within what we can afford and in balance with the environment (85). Given that GHG emissions from the development and building-related industries contribute 40% of all GHG emissions worldwide, this industry has enormous opportunities for radical transformation. To reach net zero by 2050, fifty percent of the metropolitan area will still be unbuilt. Recent technological advancements worldwide demonstrate what can be accomplished when business, authority, and civil society work together to transform linear development into circular construction. Recent technological advancements worldwide demonstrate what can be accomplished once corporations, authorities, and citizens combine to transform linear development into circular construction. Using natural ash as an economic asset is hampered by problems like substantial differences within particular portions and kinds, intense and prolonged reactions, possible toxicology, and an inconsistent, frequently absent, statutory and legal structure for managing the disposal of specific bio-wastes. With twenty per cent recyclable demolition and construction debris utilized in its worldwide line of green cement, Holcim Company ensures a twenty percent decrease in the consumption of natural resources and a 38 percent decrease in the environmental impact.
The World Economic Forum’s Centre for Nature and Climate promotes the shift to a circular economy through initiatives such as the Circular Vehicles programme and the Circular Transformation of Industries programme. The Global Plastic Action Partnership (GPAP) offers a forum for international learning and local action in nine countries (85). Buildings are essential in the theory of the circular economy, as they require numerous assets such as water, power, steel, and concrete. In certain metropolitan regions, the construction industry makes up more than a third of total energy use. As a structure’s useful life ends, only 40% of the construction waste gets recycled or reused. Most of these recycled materials are repurposed for secondary construction, such as roadways, rather than new buildings. They seek to reduce (or even eliminate) waste and pollution by increasing efficiency and reusing items and materials (85). Employing ecologically friendly building materials improves one’s well-being and can result in considerable social and economic benefits. Ten unique circular economy business models have been discovered that will drive the industry’s sustainable development and growth. These include improved software, environmentally focused engineering and consulting services, renewable and recyclable materials, and efficient new construction processes. Recycling critical resources at all stages can help reduce waste, and upcycling reuses materials towards the end of their lives. Due to the numerous ecosystem services soil provides, it is essential in creating circular structures and necessitates meticulous oversight. The concepts of the circular economy (CE) are well suited to primary earth resources since they are entirely reusable without any loss throughout the value chain. Nevertheless, the widespread growth of earth construction in certain regions might affect the availability of soil and contend with other applications for it (111). The construction industry has significant potential for circular transformation to reduce waste and promote sustainability. Soil ecosystem services are important in the construction industry, particularly in terms of soil conservation and the use of soil resources for construction materials.
2.1.5 Circular forestry, pulp and paper industry and soil ecosystem services
The carbon stored in forests and wood-based goods, and the replacement of timber for biofuels and other, more carbon-intensive materials, are all ways the forest-based bio-economy helps mitigate climate change. Nowadays, forests and products made from harvested wood absorb greenhouse gas emissions. However, many causes are causing trees’ ability to store carbon to diminish (112). Sustainable forest management techniques and reforestation are necessary to reverse this trend. The benefit of reducing climate change can be increased by a shift toward a more significant usage of wood goods with longer service lives and substitution advantages.
A “system-perspective” study aids in recognizing the compromises and benefits across various solutions and maximizes the forest-based bio economy’s contribution to achieving climate goals. By providing sustainable natural resources for building, decor, and biological energy, the woodland-based industry contributes significantly to additional value, economic development, and the creation of green employment in the circular bioeconomic setting (113). Forest-based businesses have the ability to develop unique, valuable, ecologically generated products such as polymers and plastics that are biodegradable, biochemical elements, lipids, and chemical-based solutions. Hardwood biomass has been identified as a viable raw material that can be improved via thermochemical or chemical processes. Woody biomass has several advantages, including ease of handling, a high energy content, and a relatively high fixed carbon content. It accounted for 9% of total energy use in 2019. The strategic importance of biological refineries in generating significant and low-value products from timber-based feedstock will prove key to achieving sustainable economic development and minimizing negative environmental consequences. Nevertheless, research has ignored the lignin component of wood-based biomass to concentrate on hemicellulose and cellulose conversion (114). Lignin is a renewable aromatic source that can be converted into various value-added chemicals in the biochemical, pharmaceutical and fabric industries. It can be isolated mechanically, chemically, physically, or enzymatically and classified as sulfur- or sulfur-free. Chemical agents used to separate lignin include Organosolv, Soda, Kraft, and lignosulfonate pulping (115). A “green biorefinery” is a facility that sustainably transforms green biomass into various energy and commercial products. Any naturally occurring wet biomass, such as crops and agricultural leftovers, can be called “green biomass”. These plants serve as an organic chemical factory and can be abundant in essential components, such as lipids, proteins, lignin, and carbohydrates. The potential range of products produced by green biorefineries is frequently referred to as a multiple-product system, with the composition of the feedstock determining the range of products (116). Single-product systems struggle to generate enough money to cover the costs of feedstock and processing, leading to a multiple-product strategy. The range of practical uses for the separated fractions will determine the sustainability of an industrial facility processing green biomass (117, 118). The forestry industry is essential for transitioning to a circular economy, as it has the potential to increase efficiency and replace non-renewable resources with wood. However, the increased use of biomass can displace other demands, such as those for biodiversity. Strong governance protects these services (119). Soil is crucial to forest and woodland ecosystems, providing trees with water, nutrition, and stability. Plants are vital in creating new topsoil when foliage and other greenery die and break down.
Forests and soils significantly affect one another and the larger environment, helping maintain agronomically favourable environmental conditions and contributing to a viable food system, enhanced rural livelihoods, and a healthy environment (120). Forests, forest soils, and their interconnections are essential for food security and environmental health. Carbon emissions significantly contribute to climate change; forests capture 650 billion tonnes of carbon annually. Forest soils store 45% of the carbon in global forest biomass, and 10% of carbon is contained in decaying wood and forest rubbish. Forests store the same amount of carbon as the atmosphere, and the world needs sustainably maintained forests to reduce erosion and conserve soil (120). Sustainable forest management reduces soil loss and the danger of landslides and snowstorms while substantially contributing to the structures that sustain the world’s safe water resources. Exemplary forest management procedures can help prevent or reduce soil loss and superficial avalanches by creating or maintaining dense forests on soils vulnerable to erosion and run-off. To control external landmass motions, tree roots anchor ridge, hill, and hillside slopes while offering the soil the structural support it needs.
Additionally, initiatives to restore and improve soil fertility, such as reforestation, offer several benefits and should be a part of any watershed management strategy. Forests and trees are the foundation of soil conservation in semi-arid and arid regions, safeguarding soil resources by minimizing soil erosion and preventing or lowering salinization. In arid-zone forests, forests can help lessen mountain soils’ sensitivity to deterioration (120). Mountain habitats are susceptible to erosion due to steep slopes and thin soil. Mountain soils frequently deteriorate and, as a result, do not provide adequate nutrients for plant growth. The FAO estimates that 45% of the world’s mountain land is unsuitable for agriculture. Mountain farmers encounter difficulties, including short vegetal stages, inclining terrain, thin soils, and landslides. They have created intricate and varied agricultural systems to thrive on agricultural land, grasslands, and forests. However, as forests are cut down, soil loss and land degradation undermine the agricultural resource base. We must protect our forests and trees to safeguard our soils, which are essential to food safety and ecological well-being (120). Sustainable forest management is essential for maintaining soil ecosystem services, as forests provide carbon storage, regulate water resources, and prevent soil erosion. The forest-based bioeconomy relies on soil ecosystems, and careful management is necessary to ensure the sustainable use of forest resources.
2.1.6 Circular renewable energy, bio-based raw material and soil ecosystem services
The energy sector’s evolution depends on the circular economy. The economy of circularity will prove crucial in three critical ways for the energy systems the world needs at pace and magnitude, as elucidated in World Economic Forum (106). A sustainable economy protects the environment, manages the planet’s assets responsibly, and eliminates wastage for energy efficiency in buildings, industrial processes and transport; a bio-based economy focuses on bio-based chemicals and biofuels (121). It is premised on some of the principles viz.
i. Recycling helps preserve essential resources, such as cobalt, lithium, and rare earth, for their production. Neodymium is an earth-based metal that is difficult to mine and is utilized in numerous electrically powered engines and machines. The economy of circularity may guarantee sustainable use of all these commodities while reducing reliance on exploitation and exploration. A mere one per cent of the almost sixty million tons of mobile phones, computer storage media, and other electronic gadgets are ever repurposed, even though reusing might retrieve metallic substances from these products. Poor recycling rates are faced by other metals used in technology that are essential to the shift.
ii. Utilizing circular, low-carbon materials.
The circular economy can be a source of low-carbon materials. Clean technology must be manufactured using components with no emissions and must not emit pollutants while utilized to achieve net zero. Examples include electric vehicles and energy transition machinery. This promises to be a challenging task. For instance, compared to virgin information, reused aluminium generates as much as 95% less carbon dioxide. Creating renewable energy architecture from recyclables will aid in the net zero transition.
iii. The creation of circular systems
The circular economy must be considered throughout the planning phase of any migration to renewable energy sources. This involves creating and running systems to deal with garbage and designing items for easier recycling and deconstruction. Because durable items may be put to different applications, extending their lifespan is particularly crucial.
Bio-based raw materials have the potential to replace products made from fossil fuels, but not enough are collected for one planet. Sources of energy that are renewable, like wind and sunlight, generate energy. Still, when making plastics, textiles, or other fibres, you need the biomass and its green carbon atoms when you need the actual carbon molecule in the material. The importance of residual streams cannot be overstated, as they can be used to generate paper pulp or biofuel. New goods or combinations made from bio-based residual streams are practically limitless in their potential, but there are obstacles to overcome along the road. There is interest from several businesses, including traditional bio-industries like pulp/paper and food and others like the chemical and refinery industries. Biorefinery is a long-term method of producing different bioenergy products from varied biomass feedstocks by incorporating applicable conversion technologies (104). With the increased focus on circular economy over the last half-decade, the biorefinery is a strategic mechanism for implementing a circular bioeconomy by holistically addressing the industrial sector’s economic, environmental, and social aspects. Biomass wastes have been crucial in installing biorefinery in the circular bioeconomy. Boundaries and openings of biorefineries towards sustainable biofuel production (122, 123). Thermochemical treatment of waste from biomass into macro-biopolymeric substances like biochar may be utilized as a soil supplement to improve soil quality and crop yield by lowering pH in the soil, boosting water-holding ability, changing the soil organisms, and conserving nutrients (124).
The literature has demonstrated the economic viability, and creating more affordable biochar may further strengthen it. Additionally, through fractionation/extraction, thermochemical or biological upgrading, and valuable organic molecules (such as succinic acid as a pesticide), acceptable compounds like nutrients (such as phosphorous) can be recovered from biomass wastes. Smith and Nayak (125) noted that energy production affects soil ecosystem services. Peat burning contributes only 0.02% of the world’s energy supply, but it emits 0.7-0.8% of the carbon dioxide that would otherwise come through forestry and changes in land use. Bioenergy crops account for 0.3-0.6% of harvested land and contribute about 0.3% of the energy supply. With protective regulations, incorporating waste products from power production might store some carbon. Wind farms, hydropower solar, and geothermal systems offer around 3.66%, but carbon losses may surpass savings in fossil fuel emissions if located on peatlands. Detailed regulations and administration standards are required to prevent peats, transform fixed land uses to fuel plants, and restore waste from converting energy technologies to the soil to ensure that clean energy is not detrimental. The transition to renewable energy sources and bio-based materials requires a circular approach. Soil ecosystems play a role in providing bio-based resources and can benefit from the development of renewable energy systems. However, the impact of energy production on soil ecosystem services, such as the use of peat in energy production, needs to be carefully managed.
2.1.7 Circular cultural services, recreation and soil ecosystem services
Along with supplying, regulating, and supporting services, cultural ecosystem services are crucial elements outlined in the Millennium Ecological System Assessment (126). The advantages humanity experiences through their interactions with various environmental settings, such as parks or wooded areas, and the activities they engage in, such as biking and strolling, are called cultural ecosystem services. These interactions result in various health advantages that can be measured in monetary, qualitative, and other forms. The intangible advantages that people get from ecosystems are known as cultural services. These include artistic inspiration, cultural identity, a sense of belonging, and spiritual experiences associated with nature. Generally, tourist and recreation prospects are also included within the group. Cultural services are highly interrelated and frequently linked to provisioning and regulating services. Small-scale fishing is about more than just food and cash; it is also about the fishermen’s way of life. Many kinds of ecosystem services are influenced by and are influenced by agriculture, forestry, and fisheries. Recreational activities grounded in nature are crucial for preserving physical and mental health, such as walking and playing sports in parks and other urban green spaces.
Grasslands are excellent outdoor recreation areas; aquatic systems serve vital leisure and health activities. Forest may be used for various sports, and nature tourism provides tourists with benefits and economic prospects. Nature is a recurring theme in most major faiths, and individual well-being is linked to the various community centre-level locations and cultural resources. The cocultural functions of soil include aesthetic qualities, social relations, and educational benefits (127). For responder groups with various cultural and social-demographic origins, it is essential to stress the natural character of cultural soil ecological services and distinctive trends in bundle interpretation (128). Cultural services reflect particular trends in magnitude, splendour, and variety rather than being scattered arbitrarily throughout a terrain.
Locations and cold spots for soil ecological supply of services correlate with topographic characteristics and land cover types. Cultural services mapping analyses should be essential for cultural landscape management and protection. Information on cultural ecosystem services that is spatially explicit (129). A solid foundation for creating sustainable land management plans can be found in spatiotemporally precise information on cultural services provided by an ecological community considering diverse opinions by the homegrown communities (130). They might reorient the conservation of biodiversity and the preservation of cultural assets, promoting multifunctionality. The soil ecosystem services greatly influence the promotion of cultural services. Significant cultural and recreational value can be found in enclosed farmland. According to Wang et al. (131) and Gasparatos et al. (132), the economy based on biological assets often comprises the land utilization intensity, which impacts specific regulating functions, like climate control, cultural assets, leisure and visual appeal. A better knowledge of the business and management of ecological services and how they are produced are necessary to deliver various ecosystem services effectively (133). It is widely understood that a thriving bioeconomy necessitates utilizing the potential of renewable biological resources. The questions are whether it can be accomplished without negatively impacting soil ecosystem services. How humanity may create future bio- and circular economies so that ecosystem services are not only protected but even enhanced and what the relationships between ecosystem services and a thriving and sustainable bioeconomy will be are all unanswered concerns. Soil ecosystems provide cultural services, such as recreational opportunities and aesthetic values. Understanding the spatial distribution of cultural services and their relationship to soil ecosystems can inform land management and conservation efforts.
2.1.8 Circular genetic, pharmaceutical, biochemical resources and soil ecosystem services
Many people in society support the biological economy as a sustainable path. Three bioeconomic concepts—biotechnology, agricultural ecology, and resources—are emerging within the research community (134). Implementing one or more of these visions into strategies entails altering how ecosystem services are delivered through changes in land use, with noticeable trade-offs. The basis of all terrestrial life is soil. They carry out crucial ecosystem tasks, offering people various ecosystem benefits. Nature’s contributions to people are defined as the power inherent in nature to govern or control specific creatures seen to be harmful; soil and soil biodiversity play crucial roles in regulating direct negative impacts on humans and on plants and animals that are vital to humans (31). Soil ecosystem services provided by intact, “healthy” soils are essential and heavily reliant on biotic activity. Human and soil health are related, yet our understanding of how soils operate remains limited. Soil, a genetic resource and a biodiversity hotspot, provides the foundation for delivering biochemical resources, therapeutic services, and goods. Unmatched biodiversity of species, particularly microbes, can be found in soils. Soils function as biological pesticides, aid in the breakdown of contaminants, and manufacture catalysts or compounds for commercial use thanks to helpful crop growth-promoting organisms (135). These are some of the capabilities of native microbes and the related enzymes that enhance plant development and other soil-based processes.
Human health is directly impacted by soils as well. The human microbiota is enriched by soil contact, which protects from allergies and fosters emotional well-being. Loams, clays, and other minerals, medically essential soil substrates, have healing properties and contain pharmaceutically active organic compounds, such as antibiotics, produced by microbes in the soil. On the other hand, irritating minerals, inhaling soil dust, and inappropriate soil ingestion might injure individuals (45). According to international agencies, increasing access to less expensive medications, including generic versions, will enhance drug use in the upcoming years (136). Medications for animals are thought to release far more active pharmaceutical ingredients into the environment than those for people (137). Unresolved environmental concerns include the presence of active medicinal ingredient residues in soil, water, and vegetation. This includes foodstuff grown in areas where sewage effluent and organic fertilizers nourish the land and where wastewater is used for irrigation with treated, partly treated, or untreated effluent (138). Some drugs might end up in the soil and move up the food chain from crops to people. Currently, no chemical regulations, including environmental risk assessment, apply to sewage used for irrigation. Drug-active substances may be eliminated by abiotic processes such as soil, natural processes in lakes and rivers, and biological wastewater treatment (139). These mechanisms lessen the possible side effects of drugs, although certain breakdown products are just as hazardous as their parent molecule. The strength of their sorption determines the distribution and functionality of pharmaceutical residues in soils on solid soil particles, including organic materials (140). Vegetation thriving in sandy soils will absorb and accumulate medications much more heavily than vegetation growing in soils with greater silty soils and organic matter percentages. The absorption capacity of pharmaceutically active components and their ability to persist in the soil environment is influenced by adsorption activity. This concept should be taken into account when irrigating farms with effluent from municipal treatment plants and fertilizing with either sewage sludge or natural fertilizers (manure, slurry) (137). The trophic chain and pharmacological metabolism in species need to be better understood. There needs to be more investigation done in the literature on how antibiotics contained in soil solutions affect higher species. Soils, hotspots for biodiversity and suppliers of genetic resources provide the foundation for delivering biochemical resources, therapeutic services, and products. Unmatched biodiversity of species, particularly microbes, can be found in soils. Soil ecosystems are biodiversity hotspots crucial in providing genetic resources and biochemical compounds. The disposal and presence of pharmaceutical residues in soils require careful consideration to avoid negative impacts on soil and ecosystem health.
2.2 Practical implications of circular bioeconomy
Circular Agriculture: The regenerative agricultural system utilized by the Maasai community in Kenya is an example of circular agriculture in Africa. This method combines livestock rotational grazing with rest periods for land regeneration. The Maasai community sustains soil fertility and crop yields over time by permitting land to regenerate. This circular agriculture system improves soil ecosystem services by enhancing water infiltration, reducing soil erosion, and increasing carbon sequestration (141, 142).
Food Industry: The circular bioeconomy is evident in Africa’s efforts to reduce food waste and increase the use of byproducts. In Nigeria, for instance, Innovations Farmers Association (IFA) has adopted the “zero-waste” strategy to combat food loss and waste. IFA transforms food refuse into products with added value, such as animal fodder, organic fertilizer, and bioenergy, in collaboration with producers. IFA’s circular approach reduces environmental contamination, conserves resources, and generates income for producers by closing the cycle and reusing agricultural refuse (79, 143).
Soil Ecosystem Services: In implementing agroforestry systems, the implications of the circular bioeconomy for soil ecosystem services can be observed. The World Agroforestry Centre has been assisting farmers in Malawi to implement agroforestry practices, such as incorporating trees into agricultural fields. This strategy not only diversifies the income streams of producers but also improves soil fertility, prevents soil erosion, and improves water regulation (144). Agroforestry systems in Africa contribute to circular agriculture by optimizing nutrient cycling, nurturing biodiversity, and promoting sustainable land management practices.
Data and Statistics: Research published in the Journal of Cleaner Production suggests that adopting circular agricultural methods in Africa may have various advantages. According to the study (145, 146), circular agriculture could increase annual agricultural production in seven African countries by 49 million tons, reduce greenhouse gas emissions by 121 million tons, and improve water availability by 7.8 billion cubic meters. These statistics demonstrate how circular agriculture in Africa has the potential to provide food security, lessen climate change, and guarantee resource sustainability.
Overall, these case studies, examples, and data highlight the practical applications of soil ecosystem services, food production, and circular agriculture in Africa’s circular bioeconomy. These techniques and methods provide possibilities for the region’s sustainable agricultural development, climatic adaptability, and economic expansion.
2.3 The potential challenges, limitations, or trade-offs associated with implementing circular bioeconomy strategies that rely on soil ecosystem services
While the circular bioeconomy strategies that rely on soil ecosystem services offer significant benefits, there are also potential challenges, limitations, and trade-offs that need to be considered. Some of these include:
Transition and Adoption Challenges: Land management methods, farmer behaviour, and legislative frameworks may need to be significantly altered to implement circular bioeconomy models that depend on soil ecosystem services. This change may be difficult, mainly when there is a lack of resources, technical expertise, and financial assistance. Social and cultural variables may also influence whether new techniques are adopted quickly or reluctantly.
Scale and Scope Limitations: To have a major effect, implementing circular bioeconomy initiatives often calls for a large-scale strategy. Scaling out these approaches across vast regions may be expensive and time-consuming. Maintaining uniformity and ensuring effective implementation across many landscapes with different soil types, climates, and socioeconomic circumstances may also be challenging.
Trade-offs between Production and Conservation Goals: Agricultural and conservation goals may need to be sacrificed in circular bioeconomy models that depend on soil ecosystem services. For instance, it would be necessary to take land away from conventional agriculture to expand agroforestry systems or restore degraded areas, which can result in a short-term decline in output levels. It may be challenging and needs careful planning to balance the requirement for increasing output with ecosystem protection and restoration.
Financial and Economic Considerations: It may be necessary to provide farmers and other stakeholders with financial resources and incentives to invest in circular bioeconomy methods that depend on soil ecosystem services. A lack of access to markets, credit, and money may constrain the adoption and expansion of these tactics. Additionally, it’s essential to ensure that farmers and other stakeholders get a fair share of the economic gains from circular bioeconomy initiatives, taking into account both immediate profitability and long-term sustainability.
Monitoring and Evaluation: Evaluating the efficacy and effects of circular bioeconomy initiatives on soil ecosystem services might be challenging. Long-term monitoring and assessment are often necessary to measure changes in soil fertility, biodiversity, water control, and carbon sequestration. Accurate data collection may be resource-intensive, and establishing uniform monitoring procedures may call for cooperation among many parties. Overall, while implementing circular bioeconomy strategies that rely on soil ecosystem services can bring multiple benefits, addressing these challenges, limitations, and trade-offs is essential to achieve their full potential. Integrated approaches involving multi-stakeholder collaborations, supportive policies, and capacity-building initiatives can help overcome these challenges and ensure the successful implementation of circular bioeconomy strategies in Africa and beyond.
2.4 The role of sustainable soil management practices and policies in promoting and preserving soil ecosystem services within the context of the circular bioeconomy
Within the framework of the circular bioeconomy, sustainable soil management techniques and policies are essential for increasing and maintaining soil ecosystem services. While guaranteeing sustainable resource use and limiting environmental degradation, these practices and regulations seek to preserve soil health, productivity, and ecosystem functioning. Here are several crucial elements:
Conservation and Restoration Practices: Sustainable soil management techniques strongly emphasise preserving and restoring the health and fertility of the soil. This encompasses cover crops, crop rotation, conservation tillage, and organic farming (147). These methods aid in reducing soil erosion, increasing water infiltration, enhancing nutrient cycling, and fostering advantageous soil organisms. These measures protect soil health by preserving soil ecosystem services and promoting circular bioeconomy techniques.
Integrated Nutrient Management: Proper nutrient management is essential for soil fertility to be maintained while reducing negative environmental effects. Sustainable soil management techniques encourage using organic fertilizers like compost and biochar to increase soil nutrient levels. Furthermore, using precision farming methods like targeted fertilizer application and soil testing may increase the effectiveness of nutrient utilization and decrease nitrogen losses to neighbouring ecosystems (148).
Agroecological Approaches: The maintenance and improvement of soil ecosystem services are given priority in agroecological techniques, which combine ecological principles with agricultural practices. These methods try to make agricultural systems more resilient, encourage biodiversity, and replicate natural ecosystems. Agroecology supports sustainable food production by preserving soil health and limiting synthetic inputs, promoting biological management of pests and diseases, and strengthening ecological interaction (149).
Soil Carbon Sequestration: In the circular bioeconomy, policies and practices that promote soil carbon storage are essential. By extracting carbon dioxide from the atmosphere and storing it in the soil, increasing carbon sequestration in soils reduces climate change. Agroforestry, cover crops, and decreased tillage are sustainable soil management techniques encourage organic matter accumulation (150). These techniques improve soil carbon sequestration and contribute to climate change mitigation and ecosystem services.
Knowledge Sharing and Capacity Building: Building farmers’ and other stakeholders’ capacity should be a primary goal of effective policies and initiatives. These programmes may include technical assistance and training to stimulate the adoption of circular bioeconomy techniques, increase understanding of the value of soil ecosystem services, and promote sustainable soil management practices (151). Adoption of sustainable soil management techniques may be aided further by policy frameworks that provide incentives, such as rewards or subsidies.
In summary, in the context of the circular bioeconomy, promoting and preserving soil ecosystem services depend on sustainable soil management practices and policies. These practices and policies improve food security, assist the transition to a more circular and resource-efficient bioeconomy, and contribute to the long-term sustainability of agricultural systems by prioritizing soil health, preserving soil fertility, and reducing environmental consequences.
3 Conclusions
The benefits of soil ecosystem service to people and the environment were examined, as well as their responsibilities in promoting the growth of sustainable bio- and circular economies. Many ecosystem services provided by soil are so essential to life that there is no limit to how valuable they are. Understanding the marginal costs associated with various human activities is critical to determining alternate management strategies for soil resources. Better characterization of the ecosystem services that the soil provides, particularly concerning the function of soil biodiversity, requires research. It’s also essential to understand how different services offered by soil and other systems interact with one another and how affecting one service might affect the performance of others.
Additionally, it would be worthwhile to invest in far better record-keeping and tracking of global changes in the state of the soil and the biological processes they control. Despite this unpredictability, it is evident that global soil waste is having a negative social and economic impact. Only through preserving and promoting the earth’s soil resources can the well-being of humans be sustained. Implementing a strategy to transform agricultural, pastoral, and forestry systems into sustainable businesses will safeguard the ecosystem services provided by the soil while significantly reducing pressure on many other priceless ecological services. A great way to reduce climate change is to move toward a circular economy based on biomaterials. Circular bioeconomy rules cannot be implemented without ecosystem services. According to the circular bioeconomy model, living feedstock is transformed into valuable products. Programmes for the bioeconomy are made more sustainable by linking them to the SDGs. To further promote soil’s role in providing ecosystem services and human welfare, soil scientists should collaborate with experts from other fields. Studies on soils could be used to establish local and national programmes and policies for managing and using natural resources. A holistic understanding of soil ecosystem services is crucial for successfully implementing circular practices across different bioeconomy sectors. Soil conservation, sustainable management, and the protection of soil resources are vital for maintaining the services that support a circular bioeconomy (152). The paper recommends further research on how soil ecosystem services contribute to the circular bioeconomy, including studying interactions between soil organisms, nutrient cycling, and bioeconomic processes. Policy interventions that promote sustainable soil management practices are needed, and practical recommendations for farmers include adopting soil conservation practices, using cover crops and crop rotations, and fostering composting. The findings highlight the critical role of soil ecosystem services in the circular bioeconomy and emphasize the need for research, policy, and practice to enhance soil health and achieve a sustainable bioeconomic system.
Author contributions
Conceptualization and execution was done by both authors. All authors contributed to the article and approved the submitted version.
Conflict of interest
The authors declare that the research was conducted in the absence of any commercial or financial relationships that could be construed as a potential conflict of interest.
The author(s) MANA declared that they were an editorial board member of Frontiers, at the time of submission. This had no impact on the peer review process and the final decision.
Publisher’s note
All claims expressed in this article are solely those of the authors and do not necessarily represent those of their affiliated organizations, or those of the publisher, the editors and the reviewers. Any product that may be evaluated in this article, or claim that may be made by its manufacturer, is not guaranteed or endorsed by the publisher.
References
1. Sokol NW, Slessarev E, Marschmann GL, Nicolas A, Blazewicz SJ, Brodie EL, et al. Life and death in the soil microbiome: How ecological processes influence biogeochemistry. Nat Rev Microbiol (2022) 20(7):415–30. doi: 10.1038/s41579-022-00695-z
2. Coleman DC, Wall DH. Soil fauna: Occurrence, biodiversity, and roles in ecosystem function. Soil microbiology Ecol Biochem (2015) 4:111–49. doi: 10.1016/B978-0-12-415955-6.00005-0
3. Canti MG. Earthworm activity and archaeological stratigraphy: a review of products and processes. J Archaeological Sci (2003) 30(2):135–48. doi: 10.1006/jasc.2001.0770
4. Jakhar SR, Singh J, Kumhar BL, Kumar V. Soil microbes: A key player on soil health and crop production under changing climate. Progressive Agric (2020) 20(1and2 and):129–40. doi: 10.5958/0976-4615.2020.00020.4
5. National Research Council. Climate stabilization targets: emissions, concentrations, and impacts over decades to millennia. Washington, DC USA: National Academies Press (2011).
6. Wagg C, Bender SF, Widmer F, Van Der Heijden MG. Soil biodiversity and soil community composition determine ecosystem multifunctionality. Proc Natl Acad Sci (2014) 111(14):5266–70. doi: 10.1073/pnas.1320054111
7. Santoyo G, Pacheco CH, Salmerón JH, León RH. The role of abiotic factors modulating the plant-microbe-soil interactions: toward sustainable agriculture. A review. Spanish J Agric Res (2017) 15(1):13. doi: 10.5424/sjar/2017151-9990
8. Rekwar RK, Patra A, Jatav HS, Singh SK, Mohapatra KK, Kundu A, et al. Ecological aspects of the soil-water-plant-atmosphere system. In: Plant perspectives to global climate changes. Amsterdam, The Netherlands: Academic Press (2022). p. 279–302.
9. Braat LC, De Groot R. The ecosystem services agenda: bridging the worlds of natural science and economics, conservation and development, and public and private policy. Ecosystem Serv (2012) 1(1):4–15. doi: 10.1016/j.ecoser.2012.07.011
10. Ehrlich PR, Ehrlich AH. Betrayal of science and reason: How anti-environmental rhetoric threatens our future. Washington, D.C USA: Island Press (1996).
11. Mooney HA, Ehrlich PR, Daily GE. Ecosystem services: a fragmentary history. Nature’s services: Societal Depend Natural Ecosyst (1997), 11–9. doi: 10.1073/pnas.1114773109
12. Corvalan C, Hales S, McMichael AJ, Butler C. Ecosystems and human well-being: health synthesis (Vol. 1). Geneva, Switzerland: World health organization (2005).
13. MEA. Ecosystems and human well-being: current state and trends. In: Millennium ecosystem assessment, global assessment reports (Geneva, Switzerland: WHO Press) (2005).
14. McMichael A, Hefny M, Foale S. Linking ecosystem services and human well-being. In: Ecosystems and human well-being (Geneva, Switzerland: WHO Press), vol. 43. (2005).
15. Bazzaz FA, Sombroek WG eds. Global climate change and agricultural production: direct and indirect effects of changing hydrological, pedological, and plant physiological processes. Rome Italy: Food & Agriculture Org (1996).
16. Balvanera P, Pfisterer AB, Buchmann N, He JS, Nakashizuka T, Raffaelli D, et al. Quantifying the evidence for biodiversity effects on ecosystem functioning and services. Ecol Lett (2006) 9(10):1146–56. doi: 10.1111/j.1461-0248.2006.00963.x
17. Sivakumar MVK. Interactions between climate and desertification. Agric For meteorology (2007) 142(2-4):143–55. doi: 10.1016/j.agrformet.2006.03.025
18. Enneth C. Impact of climate variability on selected kipsigis sacred plant species in Belgut division. Kericho county, Kenya: doctoral dissertation, Kenyatta University (2015).
19. Baker JL ed. Climate change, disaster risk, and the urban poor: cities building resilience for a changing world. Washington, DC USA: World Bank Publications (2012).
20. Kofinas GP, Chapin FS. Sustaining livelihoods and human well-being during social-ecological change. In: Principles of ecosystem stewardship. New York, NY: Springer (2009). p. 55–75.
21. Lal R. Soil health and carbon management. Food Energy Secur (2016) 5(4):212–22. doi: 10.1002/fes3.96
22. McMichael AJ, Woodruff RE, Hales S. Climate change and human health: present and future risks. Lancet (2006) 367(9513):859–69. doi: 10.1016/S0140-6736(06)68079-3
23. Adhikari K, Hartemink AE. Linking soils to ecosystem services—A global review. Geoderma (2016) 262:101–11. doi: 10.1016/j.geoderma.2015.08.009
24. Stromberger M, Comerford N, Lindbo D. Soil ecosystem services and natural capital. In: Soil ecosystems services (New Jersey, USA: Wiley Press) (2020). p. 1–12.
25. Glenk K, Hall C, Liebe U, Meyerhoff J. Preferences of Scotch malt whisky consumers for changes in pesticide use and origin of barley. Food Policy (2012) 37(6):719–31. doi: 10.1016/j.foodpol.2012.08.003
26. Pereira P, Bogunovic I, Muñoz-Rojas M, Brevik EC. Soil ecosystem services, sustainability, valuation and management. Curr Opin Environ Sci Health (2018) 5:7–13. doi: 10.1016/j.coesh.2017.12.003
27. FAO. Soil functions infographics. (2020). Available at: https://www.fao.org/resources/infographics/infographics-details/en/c/284478/.
28. Quijas S, Schmid B, Balvanera P. Plant diversity enhances provision of ecosystem services: a new synthesis. Basic Appl Ecol (2010) 11(7):582–93. doi: 10.1016/j.baae.2010.06.009
30. Pandey D. Agricultural sustainability and climate change nexus. In: Contemporary environmental issues and challenges in era of climate change. Singapore: Springer Nature (2020). doi: 10.1007/978-981-32-9595-7_4
31. De Groot RS, Wilson MA, Boumans RM. A typology for the classification, description and valuation of ecosystem functions, goods and services. Ecol economics (2002) 41(3):393–408. doi: 10.1016/S0921-8009(02)00089-7
32. Murphy SP, Allen LH. Nutritional importance of animal source foods. J Nutr (2003) 133(11):3932S–5S. doi: 10.1093/jn/133.11.3932S
33. Mottet A, Teillard F, Boettcher P, De’Besi G, Besbes B. Domestic herbivores and food security: current contribution, trends and challenges for a sustainable development. Animal (2018) 12(s2):s188–98. doi: 10.1017/S1751731118002215
34. Bradford GE. Contributions of animal agriculture to meeting global human food demand. Livestock production Sci (1999) 59(2-3):95–112. doi: 10.1016/S0301-6226(99)00019-6
35. Razafindratsima OH, Kamoto JF, Sills EO, Mutta DN, Song C, Kabwe G, et al. Reviewing the evidence on the roles of forests and tree-based systems in poverty dynamics. For Policy Economics (2021) 131:102576. doi: 10.1016/j.forpol.2021.102576
36. Mason CL, Lippke BR, Oneil EE. Baselines. Boundaries, and forest carbon conundrums. In: CORRIM final report. Washington, United States: CORRIM (Consortium for Research on Renewable Industrial Materials) (2019).
37. Tieminie RN, Loh CE, Tieguhong JC, Nghobuoche MF, Mandiefe PS, Tieguhong MR. Non-timber forest products and climate change adaptation among forest-dependent communities in Bamboko forest reserve, the southwest region of Cameroon. Environ Syst Res (2021) 10(1):1–13. doi: 10.1186/s40068-020-00215-z
38. FAO, ITPS. The Status of the World’s Soil Resources (SWSR)–main report. Rome: Food and Agriculture Organization of the United Nations and Intergovernmental Technical Panel on Soils (2015).
40. Lapointe NW, Cooke SJ, Imhof JG, Boisclair D, Casselman JM, Curry RA, et al. Principles for ensuring healthy and productive freshwater ecosystems that support sustainable fisheries. Environ Rev (2014) 22(2):110–34. doi: 10.1139/er-2013-0038
41. Schoenholtz SH, Van Miegroet H, Burger JA. A review of chemical and physical properties as indicators of forest soil quality: challenges and opportunities. For Ecol Manage (2000) 138(1-3):335–56. doi: 10.1016/S0378-1127(00)00423-0
42. Britannica T, Editors of Encyclopaedia. Fuller’s earth. Chicago, Illinois: Encyclopedia Britannica (2020). Available at: https://www.britannica.com/science/fullers-earth.
43. McAllister DE, Hamilton AL, Harvey B. Global freshwater biodiversity: striving for the integrity of freshwater ecosystems Vol. 11. Canada: Sea wind: bulletin of Ocean Voice International (1997).
44. Schleiss AJ, Franca MJ, Juez C, De Cesare G. Reservoir sedimentation. J Hydraulic Res (2016) 54(6):595–614. doi: 10.1080/00221686.2016.1225320
45. Thiele-Bruhn S. The role of soils in provision of genetic, medicinal and biochemical resources. Philos Trans R Soc B (2021) 376(1834):20200183. doi: 10.1098/rstb.2020.0183
46. Escobedo FJ. Understanding urban regulating ecosystem services in the Global South. New York, USA: Urban ecology in the Global South (2021) p. 227–44.
47. Comerford NB, Franzluebbers AJ, Stromberger ME, Morris L, Markewitz D, Moore R. Assessment and evaluation of soil ecosystem services. Soil Horizons (2013) 54(3):1–14. doi: 10.2136/sh12-10-0028
48. Olson KR, Al-Kaisi M, Lal R, Morton LW. Soil ecosystem services and intensified cropping systems. J Soil Water Conserv (2017) 72(3):64A–9A. doi: 10.2489/jswc.72.3.64A
49. Tahat M, Alananbeh KM, Othman YA, Leskovar DI. Soil health and sustainable agriculture. Sustainability (2020) 12(12):4859. doi: 10.3390/su12124859
50. Seiler KP, Gat JR. Groundwater recharge from run-off, infiltration and percolation (Vol. 55). New York, USA: Springer Science & Business Media (2007).
51. Lerner DN, Harris B. The relationship between land use and groundwater resources and quality. Land Use Policy (2009) 26:S265–73. doi: 10.1016/j.landusepol.2009.09.005
52. Scanlon BR, Reedy RC, Stonestrom DA, Prudic DE, Dennehy KF. Impact of land use and land cover change on groundwater recharge and quality in the southwestern US. Global Change Biol (2005) 11(10):1577–93. doi: 10.1111/j.1365-2486.2005.01026.x
53. Myers N. The world’s forests and their ecosystem services. New York, USA: Nature’s Services: societal dependence on natural ecosystems (1997) p. 215–35.
54. Lakshmi G, Okafor BN, Visconti D. Soil microarthropods and nutrient cycling. In: In Environment, climate, plant and vegetation growth. Cham: Springer (2020). p. 453–72.
55. Carter MR. Soil quality for sustainable land management: organic matter and aggregation interactions that maintain soil functions. Agron J (2002) 94(1):38–47. doi: 10.2134/agronj2002.3800
56. Gougoulias C, Clark JM, Shaw LJ. The role of soil microbes in the global carbon cycle: tracking the below-ground microbial processing of plant-derived carbon for manipulating carbon dynamics in agricultural systems. J Sci Food Agric (2014) 94(12):2362–71. doi: 10.1002/jsfa.6577
57. Afrin H. A review on different types of soil stabilization techniques. Int J Transportation Eng Technol (2017) 3(2):19–24. doi: 10.11648/j.ijtet.20170302.12
58. Filgueiras AV, Lavilla I, Bendicho C. Chemical sequential extraction for metal partitioning in environmental solid samples. J Environ Monit (2002) 4(6):823–57. doi: 10.1039/b207574c
59. Fischer D, Glaser B. Synergisms between compost and biochar for sustainable soil amelioration. Manage organic waste (2012) 1:167–98. doi: 10.5772/31200
60. Xia YQ, Shao MA. Soil water carrying capacity for vegetation: a hydrologic and biogeochemical process model solution. Ecol Model (2008) 214(2-4):112–24. doi: 10.1016/j.ecolmodel.2008.01.024
61. Motiejūnaitė J, Børja I, Ostonen I, Bakker MR, Bjarnadottir B, Brunner I, et al. Cultural ecosystem services provided by the biodiversity of forest soils: A European Review. Geoderma (2019) 343:19–30. doi: 10.1016/j.geoderma.2019.02.025
62. Fish R, Church A, Winter M. Conceptualising cultural ecosystem services: A novel framework for research and critical engagement. Ecosystem Serv (2016) 21:208–17. doi: 10.1016/j.ecoser.2016.09.002
63. Satterfield T, Gregory R, Klain S, Roberts M, Chan KM. Culture, intangibles and metrics in environmental management. J Environ Manage (2013) 117:103–14. doi: 10.1016/j.jenvman.2012.11.033
64. Wood M. Ecotourism: Principles, practices and policies for sustainability. Nairobi, Kenya: UNEp (2002).
65. Stålhammar S, Pedersen E. Recreational, cultural ecosystem services: How do people describe the value? Ecosystem Serv (2017) 26:1–9. doi: 10.1016/j.ecoser.2017.05.010
66. Bouma J. Using soil survey data for quantitative land evaluation. In: Advances in soil science (New York, USA: Springer-Verlag), vol. 9. (1989). p. 177–213.
67. Costantini EA. Possible policies and actions to protect the soil cultural and natural heritage of Europe. Geoderma Regional (2023) 32:e00599. doi: 10.1016/j.geodrs.2022.e00599
68. Costantini EA, L’Abate G. The soil cultural heritage of Italy: Geodatabase, maps, and pedodiversity evaluation. Quaternary Int (2009) 209(1-2):142–53. doi: 10.1016/j.quaint.2009.02.028
69. The Alpine Soil Partners. (2019). Available at: https://alpinesoils.eu/tuesdays-soil-ecosystem-service-cultural-and-natural-archives/#:~:text=Definition%3A%20The%20cultural%20and%20natural%20archives%20soil%20ecosystem,built%20structures%20%28foundations%20of%20houses%2C%20roads%2C%20workshops%2C%20etc.%29.
70. Lasaga AC, Ohmoto H. The oxygen geochemical cycle: dynamics and stability. Geochimica Cosmochimica Acta (2002) 66(3):361–81. doi: 10.1016/S0016-7037(01)00685-8
71. Guzman J, Golabi MH. Agroecosystem net primary productivity and carbon footprint. In: Soil health and intensification of agroecosystems. London, United Kingdom: Academic Press (2017). p. 215–29.
72. Smith J, Farmer J, Smith P, Nayak D. The role of soils in provision of energy. Philos Trans R Soc B (2021) 376(1834):20200180. doi: 10.1098/rstb.2020.0180
73. Giltrap D, Cavanagh J, Stevenson B, Ausseil AG. The role of soils in the regulation of air quality. Philos Trans R Soc B (2021) 376(1834):20200172. doi: 10.1098/rstb.2020.0172
74. Dominati E, Patterson M, Mackay A. A framework for classifying and quantifying the natural capital and ecosystem services of soils. Ecol economics (2010) 69(9):1858–68. doi: 10.1016/j.ecolecon.2010.05.002
75. Smith P, Cotrufo MF, Rumpel C, Paustian K, Kuikman PJ, Elliott JA, et al. Biogeochemical cycles and biodiversity as key drivers of ecosystem services provided by soils. Soil (2015) 1(2):665–85. doi: 10.5194/soil-1-665-2015
76. Marchese C. Biodiversity hotspots: A shortcut for a more complicated concept. Global Ecol Conserv (2015) 3:297–309. doi: 10.1016/j.gecco.2014.12.008
77. Smith P, House JI, Bustamante M, Sobocká J, Harper R, Pan G, et al. Global change pressures on soils from land use and management. Global Change Biol (2016) 22(3):1008–28. doi: 10.1111/gcb.13068
78. Misra AK. Climate change and challenges of water and food security. Int J Sustain Built Environ (2014) 3(1):153–65. doi: 10.1016/j.ijsbe.2014.04.006
79. Feleke S, Cole SM, Sekabira H, Djouaka R, Manyong V. Circular bioeconomy research for development in sub-Saharan Africa: innovations, gaps, and actions. Sustainability (2021) 13(4):1926. doi: 10.3390/su13041926
80. Province of Ontario. Strategy for a waste‐free ontario‐building the circular economy. (2017). Available at: https://files.ontario.ca/finalstrategywastefreeont_eng_aoda1_final-s.pdf.
81. International Advisory Council on Global Bioeconomy. Global bioeconomy policy report (IV). A decade of bioeconomy policy development around the world. In: Secretariat of the global bioeconomy summit 2020 (Brussels, Belgium: EU Commission) (2020). Available at: https://gbs2020.net/wp-content/uploads/2020/11/GBS-2020_Global-Bioeconomy-Policy-Report_IV_web.pdf.
82. Yessenbekova Z, Turezhanov S. Circular bioeconomics: conceptual aspects. Economic Annals-XXI (2021) 193(10):45–53. doi: 10.21003/ea.V193-05
83. Pyka A. Transformation of economic systems: The bio-economy case. In: Knowledge-driven developments in the bioeconomy. New York City, USA: Technological and Economic Perspectives, 3-16 (2017).
84. Koytsoumpa EI, Magiri–Skouloudi D, Karellas S, Kakaras E. Bioenergy with carbon capture and utilization: A review on the potential deployment towards a European circular bioeconomy. Renewable Sustain Energy Rev (2021) 152:111641. doi: 10.1016/j.rser.2021.111641
85. World Economic Forum. (2021). Available at: https://www.weforum.org/agenda/2021/04/4-ways-circular-economy-help-heavy-industry-reduce-emissions-wcef/.
86. Helming K, Diehl K, Geneletti D, Wiggering H. Mainstreaming ecosystem services in European policy impact assessment. Environ Impact Assess Rev (2013) 40:82–7. doi: 10.1016/j.eiar.2013.01.004
87. Helming K, Daedlow K, Paul C, Techen AK, Bartke S, Bartkowski B, et al. Managing soil functions for a sustainable bioeconomy—Assessment framework and state of the art. Land Degradation Dev (2018) 29(9):3112–26. doi: 10.1002/ldr.3066
88. Schulte VA, Hahn K, Dhanasingh A, Heffels KH, Groll J. Hydrogel–fibre composites with independent control over cell adhesion to gel and fibres as an integral approach towards a biomimetic artificial ECM. Biofabrication (2014) 6(2):024106. doi: 10.1088/1758-5082/6/2/024106
89. Munasinghe M. Millennium Consumption Goals (MCGs) for R io+ 20 and beyond: A practical step towards global sustainability. In: Natural resources forum (New Jersey, USA: Wiley Press), vol. 36. (2012). p. 202–12.
90. Ellen MacArthur Foundation. The circular economy in detail, in: Ellen macArthur foundation (2017). Available at: https://archive.ellenmacarthurfoundation.org/explore/the-circular-economy-in-detail (Accessed March. 1, 2023).
91. Roberts L, Brower A, Kerr G, Lambert S, McWilliam W, Moore K, et al. The nature of wellbeing: how nature’s ecosystem services contribute to the wellbeing of New Zealand and New Zealanders. Wellington, Australia: Department of Conservation (2015).
92. Dhyani S, Lahoti S, Khare S, Pujari P, Verma P. Ecosystem-based Disaster Risk Reduction approaches (EbDRR) as a prerequisite for inclusive urban transformation of Nagpur City, India. Int J disaster Risk reduction (2018) 32:95–105. doi: 10.1016/j.ijdrr.2018.01.018
93. Jurgilevich A, Birge T, Kentala-Lehtonen J, Korhonen-Kurki K, Pietikäinen J, Saikku L, et al. Transition towards circular economy in the food system. Sustainability (2016) 8(1):69. doi: 10.3390/su8010069
94. Daily GC. Nature’s services: societal dependence on natural ecosystems (1997). In: The future of nature. Connecticut, USA: Yale University Press (2013). p. 454–64.
95. Navare K, Muys B, Vrancken KC, Van Acker K. Circular economy monitoring–how to make it apt for biological cycles? Resources Conserv Recycling (2021) 170:105563. doi: 10.1016/j.resconrec.2021.105563
96. The Nature Conservancy (TNC). Regional conservation partnership program (RCPP). Washington DC, USA: Saginaw Bay Watershed Conservation Partnership (2015). Available at: http://www.conservationgateway.org/ConservationByGeography/NorthAmerica/UnitedStates/michigan/projects/Documents/Saginaw%20Bay%20RCPP%20facsheet.pdf.
97. Moberg F, Folke C. Ecological goods and services of coral reef ecosystems. Ecol economics (1999) 29(2):215–33. doi: 10.1016/S0921-8009(99)00009-9
98. Moberg F, Rönnbäck P. Ecosystem services of the tropical seascape: interactions, substitutions and restoration. Ocean Coast Manage (2003) 46(1-2):27–46. doi: 10.1016/S0964-5691(02)00119-9
99. Campanati C, Willer D, Schubert J, Aldridge DC. Sustainable intensification of aquaculture through nutrient recycling and circular economies: more fish, less waste, blue growth. Rev Fisheries Sci Aquaculture (2022) 30(2):143–69. doi: 10.1080/23308249.2021.1897520
100. Mulazzani L, Malorgio G. Blue growth and ecosystem services. Mar Policy (2017) 85:17–24. doi: 10.1016/j.marpol.2017.08.006
101. Aich N, Nama S, Biswal A, Paul T. A review on recirculating aquaculture systems: Challenges and opportunities for sustainable aquaculture. Innovative Farming (2020) 5(1):017–24.
103. Sherwood J. The significance of biomass in a circular economy. Bioresource Technol (2020) 300:122755. doi: 10.1016/j.biortech.2020.122755
104. Hingsamer M, Jungmeier G. Biorefineries. In: The role of bioenergy in the bioeconomy. Amsterdam, The Netherlands: Academic Press (2019). p. 179–222.
105. Sasse JP, Trutnevyte E. Regional impacts of electricity system transition in Central Europe until 2035. Nat Commun (2020) 11(1):4972. doi: 10.1038/s41467-020-18812-y
106. Swift RS. Sequestration of carbon by soil. Soil Sci (2001) 166(11):858–71. doi: 10.1097/00010694-200111000-00010
107. Omer AM. Energy, environment and sustainable development. Renewable Sustain Energy Rev (2008) 12(9):2265–300. doi: 10.1016/j.rser.2007.05.001
108. Grathwohl P. Geophysical research abstracts (Göttingen, Germany: Copernicus publications), Vol. 12. (2010), EGU2010-6616, 2010 EGU General Assembly 2010.
109. Danielopol DL, Griebler C, Gunatilaka A, Notenboom J. Present state and future prospects for groundwater ecosystems. Environ Conserv (2003) 30(2):104–30. doi: 10.1017/S0376892903000109
110. Mazzucato M. Governing missions in the european union. Brussels, Belgium: Independent Expert Report (2019).
111. Morel JC, Charef R, Hamard E, Fabbri A, Beckett C, Bui QB. Earth as construction material in the circular economy context: practitioner perspectives on barriers to overcome. Philos Trans R Soc B (2021) 376(1834):20200182. doi: 10.36333/fs07
112. Leskinen P, Cardellini G, González-García S, Hurmekoski E, Sathre R, Seppälä J, et al. Substitution effects of wood-based products in climate change mitigation. From Science to Policy 7 (European Forest Institute) (2018). doi: 10.1016/j.envdev.2015.03.006
113. Scarlat N, Dallemand JF, Monforti-Ferrario F, Nita V. The role of biomass and bioenergy in a future bioeconomy: Policies and facts. Environ Dev (2015) 15:3–34. doi: 10.1016/j.biortech.2021.125626
114. Solarte-Toro JC, Alzate CAC. Biorefineries as the base for accomplishing the sustainable development goals (SDGs) and the transition to bioeconomy: Technical aspects, challenges and perspectives. Bioresource Technol (2021) 340:125626. doi: 10.1016/j.biortech.2016.04.103
115. Carvajal JC, Gómez Á, Cardona CA. Comparison of lignin extraction processes: economic and environmental assessment. Bioresource Technol (2016) 214:468–76. doi: 10.1016/j.resconrec.2009.03.013
116. Cherubini F, Bird ND, Cowie A, Jungmeier G, Schlamadinger B, Woess-Gallasch S. Energy-and greenhouse gas-based LCA of biofuel and bioenergy systems: Key issues, ranges and recommendations. Resources Conserv recycling (2009) 53(8):434–47. doi: 10.1016/j.resconrec.2009.03.013
117. Mandle J. Persistent underdevelopment: Change and economic modernization in the West Indies. New York, USA: Routledge (2010).
118. Kromus S, Wachter B, Koschuh W, Mandl M, Krotscheck C, Narodoslawsky M. The green biorefinery Austria-development of an integrated system for green biomass utilization. Chem Biochem Eng Q (2004) 18(1):8–12. doi: 10.1108/JEIM-03-2020-0110
119. Beckmann A, Sivarajah U, Irani Z. Circular economy versus planetary limits: a Slovak forestry sector case study. J Enterprise Inf Manage (2021) 34(6):1673–98. doi: 10.1108/JEIM-03-2020-0110
120. FAO. (2015). Available at: https://www.fao.org/soils-2015/news/news-detail/en/c/285569/#:~:text=Soil%20is%20an%20important%20component%20of%20forest%20and,Soils%20provide%20trees%20with%20anchorage%2C%20water%20and%20nutrients.
121. Olabi AG. Circular economy and renewable energy. Energy (2019) 181:450–4. doi: 10.1016/j.energy.2019.05.196
122. Rajamehala M, Su R, Gopalakrishnan B, Pandian AMK, Singh MVP, Chozhavendhan S. Boundaries and openings of biorefineries towards sustainable biofuel production. In: Biofuels and bioenergy. Amsterdam, The Netherlands: Elsevier (2022). p. 3–22.
123. Ubando AT, Felix CB, Chen WH. Biorefineries in circular bioeconomy: A comprehensive review. Bioresource Technol (2020) 299:122585. doi: 10.1016/j.biortech.2019.122585
124. Yu IK, Tsang DC, Hou D. Biorefinery-assisted soil management for enhancing food security. J Soils Sediments (2020) 20:4007–10. doi: 10.1007/s11368-020-02723-y
125. Shaver GR, Billings WD, Chapin FS, Giblin AE, Nadelhoffer KJ, Oechel WC, et al. Global change and the carbon balance of arctic ecosystems. BioScience (1992) 42(6):433–41.
126. M.E.A. Ecosystems and Human Well-Being: a framework for assessment. Washington, Cavelo, London: UNEP (United nations Environmental Programme) (2022).
127. Bullock C, Joyce D, Collier M. An exploration of the relationships between cultural ecosystem services, socio-cultural values and well-being. Ecosystem Serv (2018) 31:142–52. doi: 10.1016/j.ecoser.2018.02.020
128. Martín-López B, Iniesta-Arandia I, García-Llorente M, Palomo I, Casado-Arzuaga I, Amo DGD, et al. Uncovering ecosystem service bundles through social preferences. PloS One (2012) 7(6):e38970. doi: 10.1016/j.landusepol.2012.12.013
129. Plieninger T, Dijks S, Oteros-Rozas E, Bieling C. Assessing, mapping, and quantifying cultural ecosystem services at community level. Land Use Policy (2013) 33:118–29. doi: 10.1016/j.landusepol.2012.12.013
130. Deng L, Zhu GY, Tang ZS, Shangguan ZP. Global patterns of the effects of land-use changes on soil carbon stocks. Global Ecol Conserv (2016) 5:127–38. doi: 10.1016/j.gecco.2015.12.004
131. Wang X, Dong X, Liu H, Wei H, Fan W, Lu N, et al. Linking land use change, ecosystem services and human well-being: A case study of the Manas River Basin of Xinjiang, China. Ecosystem Serv (2017) 27:113–23. doi: 10.1016/j.ecoser.2017.08.013
132. Gasparatos A, Romeu-Dalmau C, von Maltitz GP, Johnson FX, Shackleton C, Jarzebski MP, et al. Mechanisms and indicators for assessing the impact of biofuel feedstock production on ecosystem services. Biomass Bioenergy (2018) 114:157–73. doi: 10.1016/j.biombioe.2018.01.024
133. Firbank L, Bradbury RB, McCracken DI, Stoate C. Delivering multiple ecosystem services from Enclosed Farmland in the UK. Agriculture Ecosyst Environ (2013) 166:65–75. doi: 10.1016/j.agee.2011.11.014
134. D’Amato D, Bartkowski B, Droste N. Reviewing the interface of bioeconomy and ecosystem service research. Ambio (2020) 49:1878–96. doi: 10.1007/s13280-020-01374-0
135. Joutey NT, Bahafid W, Sayel H, El Ghachtouli N. (2013). Biodegradation: involved microorganisms and genetically engineered microorganisms. Biodegradation - Life of Science (2013) 1:289–320.
136. Ford N, Wilson D, Chaves GC, Lotrowska M, Kijtiwatchakul K. Sustaining access to antiretroviral therapy in the less-developed world: lessons from Brazil and Thailand. Aids (2007) 21:S21–9. doi: 10.1097/01.aids.0000279703.78685.a6
137. Gworek B, Kijeńska M, Wrzosek J, Graniewska M. Pharmaceuticals in the soil and plant environment: a review. Water Air Soil pollut (2021) 232:1–17. doi: 10.1039/C9EM00020H
138. Carter LJ, Chefetz B, Abdeen Z, Boxall AB. Emerging investigator series: towards a framework for establishing the impacts of pharmaceuticals in wastewater irrigation systems on agro-ecosystems and human health. Environ Science: Processes Impacts (2019) 21(4):605–22. doi: 10.1021/es040624v
139. Boxall AB, Sinclair CJ, Fenner K, Kolpin D, Maund SJ. Peer reviewed: when synthetic chemicals degrade in the environment. Environ Sci Technol (2004) 38(19):368A–75A. doi: 10.1021/es506034e
140. Shen X, Guo X, Zhang M, Tao S, Wang X. Sorption mechanisms of organic compounds by carbonaceous materials: site energy distribution consideration. Environ Sci Technol (2015) 49(8):4894–902. doi: 10.1021/es506034e
141. Kaumbutho P, Kienzle J. Conservation agriculture as practised in Kenya: two case studies. Nairobi, Kenya: African Conservation Tillage Network (ACT); Centre de Coopération Internationale de Recherche Agronomique pour le Développement (CIRAD); Food and Agriculture Organization of the United Nations (FAO (2007).
142. Tittonell P, El Mujtar V, Felix G, Kebede Y, Laborda L, Luján Soto R, et al. Regenerative agriculture—agroecology without politics? Front Sustain Food Syst (2022) 6:844261. doi: 10.3389/fsufs.2022.844261
143. Khan I, Chowdhury S, Techato K. Waste to energy in developing countries—a rapid review: opportunities, challenges, and policies in selected countries of sub-saharan Africa and south asia towards sustainability. Sustainability (2022) 14(7):3740. doi: 10.3390/su14073740
144. Paracchini ML, Justes E, Wezel A, Zingari PC, Kahane R, Madsen S, et al. Agroecological practices supporting food production and reducing food insecurity in developing countries. A study on scientific literature in 17 countries (Luxembourg: European Union). (2020).
145. Ikram M, Ferasso M, Sroufe R, Zhang Q. Assessing green technology indicators for cleaner production and sustainable investments in a developing country context. J Cleaner Production (2021) 322:129090. doi: 10.1016/j.jclepro.2021.129090
146. Ncube A, Sadondo P, Makhanda R, Mabika C, Beinisch N, Cocker J, et al. Circular bioeconomy potential and challenges within an African context: From theory to practice. J Cleaner Production (2022) 367:133068. doi: 10.1016/j.jclepro.2022.133068
147. Farmaha BS, Sekaran U, Franzluebbers AJ. Cover cropping and conservation tillage improve soil health in the southeastern United States. Agron J (2022) 114(1):296–316. doi: 10.1002/agj2.20865
148. Bongiovanni R, Lowenberg-DeBoer J. Precision agriculture and sustainability. Precis Agric (2004) 5:359–87. doi: 10.1023/B:PRAG.0000040806.39604.aa
149. Altieri MA, Nicholls CI, Montalba R. Technological approaches to sustainable agriculture at a crossroads: An agroecological perspective. Sustainability (2017) 9(3):349. doi: 10.3390/su9030349
150. Lal R, Kimble JM. Conservation tillage for carbon sequestration. Nutrient cycling agroecosystems (1997) 49:243–53. doi: 10.1023/A:1009794514742
151. Sharma R, Malaviya P. Ecosystem services and climate action from a circular bioeconomy perspective. Renewable Sustain Energy Rev (2023) 175:113–64. doi: 10.1016/j.rser.2023.113164
Keywords: circular bioeconomy, ecosystem services, human well-being, soils, Sustainable resource management
Citation: Anikwe MAN and Ife K (2023) The role of soil ecosystem services in the circular bioeconomy. Front. Soil Sci. 3:1209100. doi: 10.3389/fsoil.2023.1209100
Received: 14 May 2023; Accepted: 14 August 2023;
Published: 02 November 2023.
Edited by:
Gilles Joseph Lemaire, INRAE Nouvelle Aquitaine Poitiers, FranceCopyright © 2023 Anikwe and Ife. This is an open-access article distributed under the terms of the Creative Commons Attribution License (CC BY). The use, distribution or reproduction in other forums is permitted, provided the original author(s) and the copyright owner(s) are credited and that the original publication in this journal is cited, in accordance with accepted academic practice. No use, distribution or reproduction is permitted which does not comply with these terms.
*Correspondence: M. A. N. Anikwe, anikwema@yahoo.co.uk
†ORCID: M. A. N. Anikwe, orcid.org/0000-0002-9981-9248