- 1Department of Science, Ministry of Education and Vocational Training, Zanzibar, Tanzania
- 2Department of Soil and Geological Sciences, Sokoine University of Agriculture, Morogoro-Tanzania, Tanzania
Rice (Oryza sativa L.) is the second cereal food crop grown in Tanzania after maize (Zea mays L.) and covers approximately 18% of the agricultural land. Soil degradation due to intensive cultivation along with low organic matter input and nutrient imbalance has led to a decline in rice crop yields. This study was conducted to characterize, classify, and assess the fertility status of soils in two rice irrigation schemes of Morogoro region in Tanzania. The data obtained through this study will contribute significantly to land use planning and will facilitate the transfer of agro-technology and other development of the regions with similar ecological conditions. The studied pedons were named MKU-P1 and MKD-P1 for Mkula and Mkindo irrigation schemes, respectively. A total of seven composite soil samples (0–20 cm) were collected for soil fertility assessments. Landform, soil morphological features, parent material, natural vegetation, drainage, erosion, and laboratory data were used to classify the soils in their respective order as per the United States Department of Agriculture (USDA) Soil Taxonomy and the World Reference Base (WRB) soil classification systems. Results showed that the pedons were sandy clay loam in the topsoil and sandy clay to clay in the subsoil; soil reaction ranged from medium acid (pH 5.7) to strongly alkaline (pH 8.6). The topsoil and subsoil nutrients of the studied pedons including available K+, total N, soil organic matter, and organic carbon are low. Based on the USDA Soil Taxonomy, MKU-P1 is classified as Inceptisols cumulic humaquepts and MKD-P1 as Vertisols Fluvaquentic endoaquerts corresponding to Subaquatic fluvisols (loamic, oxyaquic) and Irragric vertisols (gleyic) in the WRB, respectively. The pedons were ranked as suitable for rice production. However, the chemical fertility of the soil is ranked as low fertile associated with deficient in total N; available P, K+, and Ca2+ with excessive iron and manganese; and likely to pose toxicity to crops. The application of organic and mineral amendments in recommended rates and timing for N and P is therefore essential to increase the nutrient content of these soils and minimize losses. Salinity in the subsurface pedon MKD-P1 needs to be taken into future consideration.
1 Introduction
Soil classification and soil fertility assessments are fundamental disciplines and the basic features of modern agricultural technology (1, 2), and their vital purpose is to identify and quantify the soils and their ability to supply nutrients for plant growth and production (3). In comparison, land suitability assessment may be defined as an estimate of the land or soil to fit for specific crop production (4). It involves a wide range of criteria such as climatic factors, soil characteristics, and landforms to identify land use options and most suitable management solution for crop production (5, 6) and provides information related to the major factors of shortage in the production of a particular crop (7, 8). In quantitative approaches, several simulation modeling systems are used to evaluate land suitability and quantify the potential uses of land (9). FAO guidelines on land evaluation systems (10) and physical land assessment methods (11) are widely used for land suitability assessment and are adopted for the present study.
According to FAO (12), rice consumption in Africa is projected to reach 34.9 million tons by 2025. However, if the production trends continue to remain constant, the African countries will meet only two-thirds of the demand and more than 12 million tons will need to be imported annually corresponding to more than 5 billion USD (13). In Tanzania, productivity is estimated at 0.5–2.0 t ha-1 in the uplands rice production systems and at 4.5–6.0 t ha-1 in the lowland irrigated rice production system, which is far below the potential of 5 t ha-1 and 10–11 t ha-1 under proper resource endowment (14).
Previous studies carried out in some irrigated schemes of Tanzania showed that soil fertility degradation is one of the major threats facing rice production systems (15, 16), which is associated with the depletion of important nutrients such as nitrogen (N), phosphorus (P), potassium (K), sulfur (S), and zinc (Zn) (17, 18). The soils are also very low in organic carbon (OC), which is attributed to insufficient use of manure (19), topsoil erosion and leaching (20), and the deterioration of other physical soil properties (21). Therefore, the efficient management of soil is essential for ensuring proper rice production in the country (22).
Understanding soil characteristics and managing soil fertility are important to the sustainable use of soils (23), and they provide information on the soil type, fertility, and productivity (24–26), which are vital for livelihoods while maintaining food security and minimizing the risk of malnutrition (6, 19). Yet, in Tanzania, such information is still limited to specific zones of interest (27), and available information remains rather scanty relative to the large size of the country (28), particularly in lowland rice production systems (29). This makes farmers use inappropriate soil and water management options (1) and overdependence on agrochemicals as a key nutrient management option resulting in severe soil degradation and environmental pollution (30). Therefore, it is important to undertake various studies on soils to determine the appropriate management option for improved crop production (31). It is for this reason that the present study was conducted to characterize and classify the soils based on the USDA Soil Taxonomy and Tier-2 of the World Reference Base (WRB) for Soil Resources and assess the suitability and fertility status of the study areas for improved rice production.
2 Materials and methods
2.1 Description of the study areas
The study was carried out at Mkula and Mkindo irrigated schemes located in Kilombero and Mvomero districts, which lie between latitudes 8°14’28.93” S and 6°14’8.221” S and longitudes 36°20’5.71” E and 38°41’37.4928” E, respectively. The physiography and climate of the representative study sites are summarized in Table 1. Morogoro Region has a tropical savanna climate with a bimodal rainfall distribution pattern, having a dry spell separating the short rains between October and December and long rains between March and May (28). The mean annual rainfall and temperature in the Kilombero valley range from 1,200 to 1,400 mm and between 22°C and 23°C, respectively (32), and the mean annual rainfall and temperature in Mvomero range between 716.5 and 1,503.5 mm and 24°C–34°C, respectively (14). Both sites are characterized by a sandy clay loam topsoil, and clay contents increase toward the subsoil. Both sites are located within the agroecological zone E10 in the “Eastern Plateaux and Mountain Blocks.” The areas are mainly characterized by lowland irrigated rice production having two crop production patterns per year. The first pattern starts as long rains between February and June, and the second pattern is short rains between August and December. The sites receive sufficient water drained from the forest reservoir on the eastern side of Udzungwa Mountain for Mkula and the Wami basin for Mkindo. Figure 1 shows the location of the studied areas and representative sites of trials depicted on the generalized soil map of the Morogoro region of Tanzania and Figure 2 show the studied profile (MKU-P1 and MKD-P1).
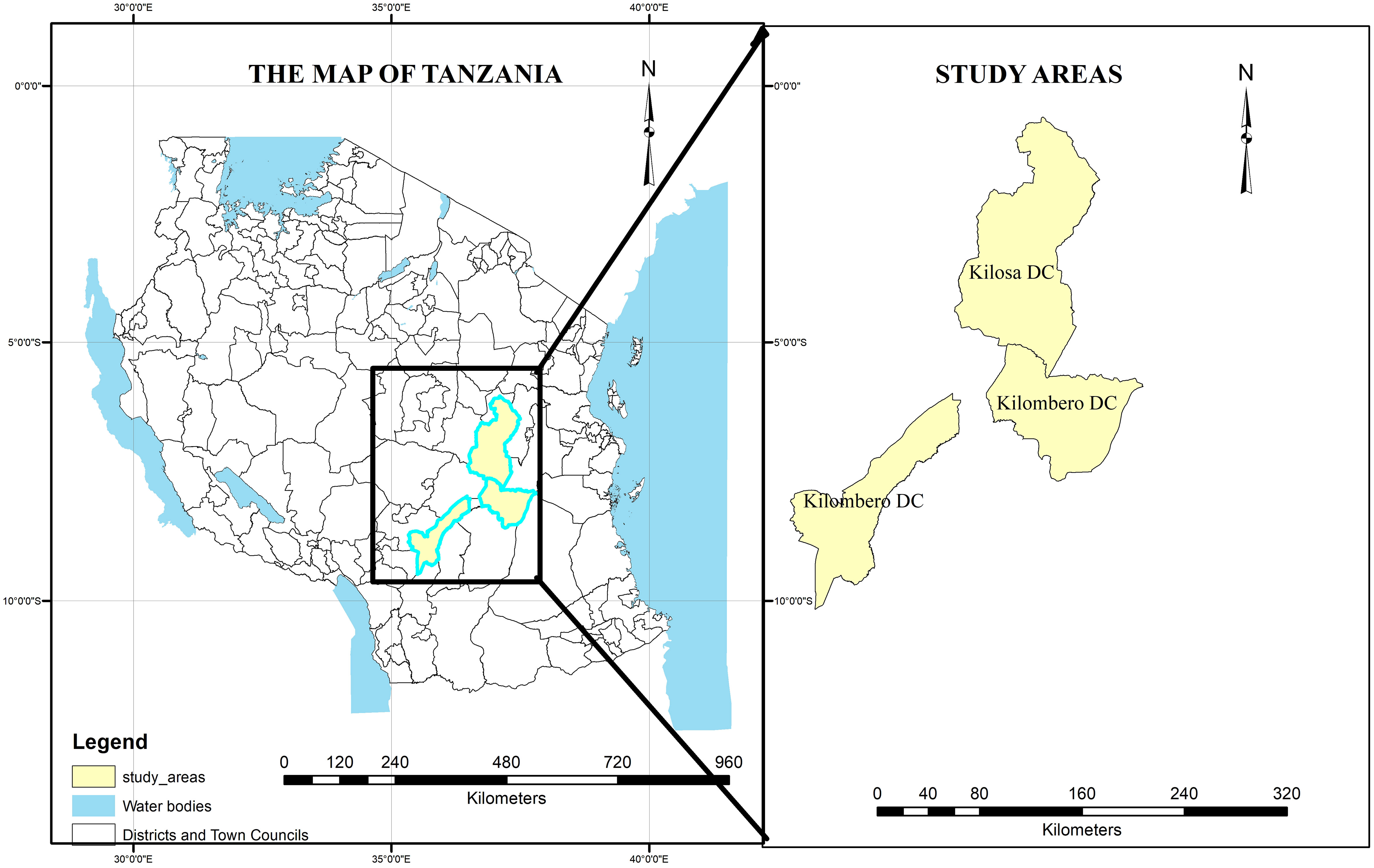
Figure 1 Location of the studied soil profile depicted on the generalized soil map of Mvomero and Kilombero districts—Tanzania (Source: Tanzania Government Printer).
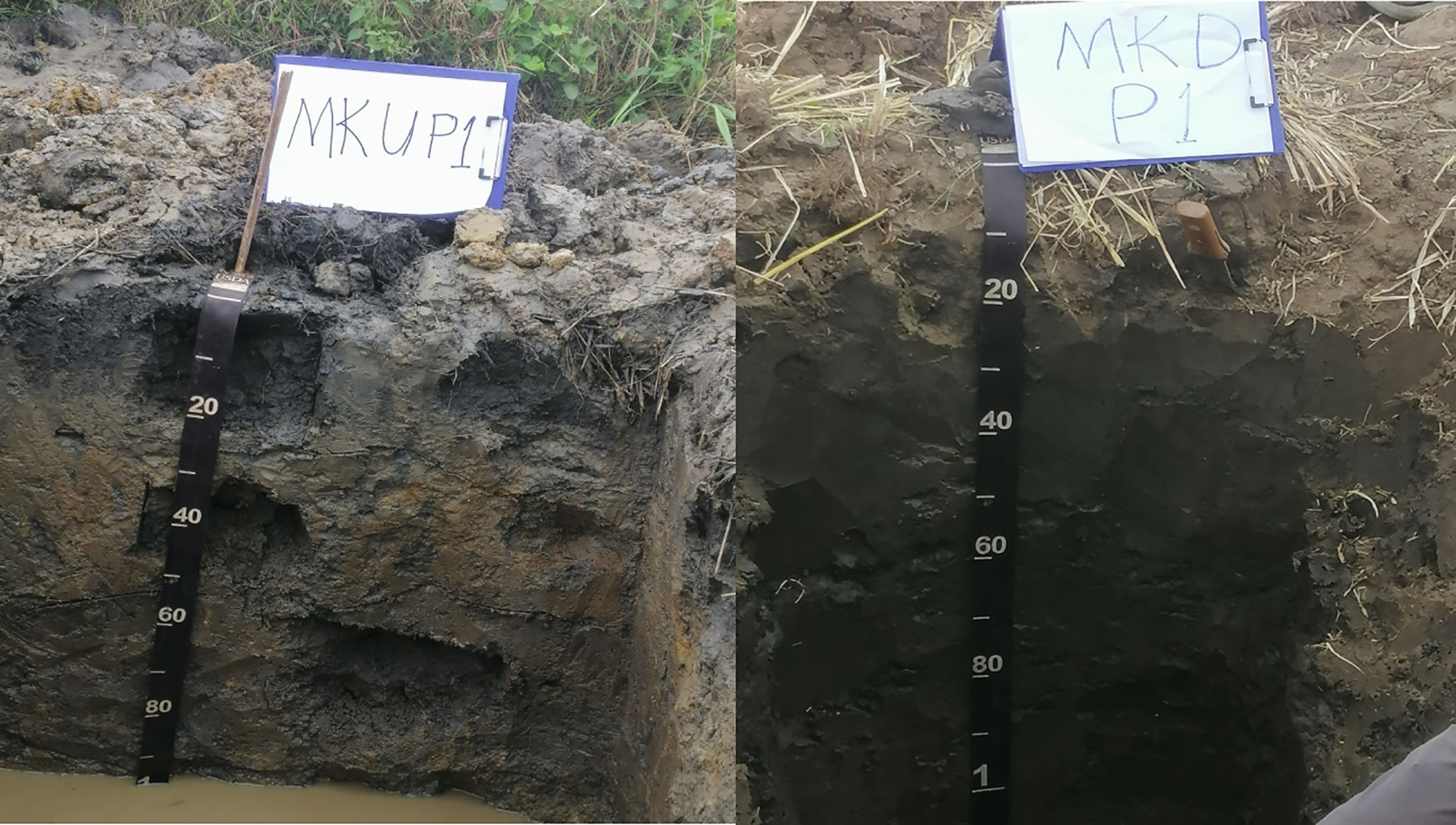
Figure 2 Soil profiles excavated at Mkula (MKU-P1) and Mkindo (MKD-P1) villages in Kilombero and Mvomero districts—Tanzania.
2.2 Fieldwork and laboratory methods
A reconnaissance field survey using transect walks and an auger observation was done in both sites from July to August 2022 (Figure 3). Data on landform, soil morphological features (color, texture, consistency, structure, porosity, and depth), parent material, natural vegetation, drainage, slope gradient, elevation, erosion, and land use were recorded and filled in forms designed by the National Soil Service, Tanzania, adopted from the FAO Guidelines for Soil Description (33). The representative study sites were georeferenced by international coordinates using the Global Positioning System (GPS) (model GARMIN etrex 20), and, in each site, one representative soil profile pit was excavated to describe the characteristics of the soil. Soil color was determined using Munsell color charts (34). Disturbed soil samples were taken from designated pedogenic horizons, whereas undisturbed core soil samples were collected from three sections of the profile (0–5, 45–50, and 95–100 cm) for laboratory analysis. Using a soil auger, seven composite soil (0–20 cm depth) samples were collected from four sampling spots. The four subsamples collected from the sampling spots were mixed and quartered to obtain representative composite samples of 1 kg for laboratory analysis. In the Mkula irrigation scheme, the land was divided into three parts: the upper slope (MKU-U), middle slope (MKU-M), and lower slope (MKU-L), while at the Mkindo irrigation scheme, the samples were taken at the upper slope (MKD-U), middle slope (MKD-M), lower slope (MKD-L), and at the valley (MKD-V).
Soil samples were collected and transported to the Sokoine University of Agriculture in Tanzania at Soil Laboratory for the determination of physical and chemical properties following standard procedures. Particle size analysis was done by the hydrometer method after dispersion with 5% sodium hexametaphosphate (35), Soil bulk density and moisture retention characteristics were done by drying undisturbed core soil samples at 105°C for 24 h (36). Soil pH and electrical conductivity were determined potentiometrically in soil-to-water suspension (1:2.5) and 1 N KCL (37). Soil OC was determined by the Walkley and Black wet oxidation method (38, 39). Extractable P was determined by a spectrophotometer (40). Total nitrogen was analyzed by the micro-Kjeldahl distillation method (37). Cation exchange capacity (CEC) was determined by the neutral ammonium acetate saturation method (NH4-Ac, pH 7.0) followed by Kjeldahl distillation (41). Exchangeable bases (K+, Mg2+, Ca2+, and Na+) were determined by the 1N NH4-Ac (pH 7.0) method. Mg and Ca were read by the UV-Vis spectrophotometer and K and Na by flame (42). Extractable micronutrients (Fe, Cu, Zn, and Mn) were extracted by diethylenetriamine pentaacetic acid (DTPA) and determined using atomic absorption spectroscopy (AAS) (43).
2.3 Soil classification and land suitability assessments
The first step in delineating land suitability for crop production was to identify the relevant climate, soil, and landscape of the areas (4, 5). In this study, field and laboratory data were used to classify and assess the suitability of the soils for rice production. The soils in each pedon were classified into their appropriate order, suborder, great group, and subgroup following the guideline of the USDA Soil Taxonomy (44) and tier-2 of the FAO World Reference Base for Soil Resources (45). The suitability assessments of the studied pedons were done by using simple limitation methods (46). The land diagnostic property was selected, and the corresponding levels of generalization were established related to the suitability classes through gradation matrices (47). Each pedon was assigned to a suitability class by matching the ecological and soil nutrient requirements for rice production (Table 2) (46). The group of land qualities considered for evaluation includes climate (c), topography (t), drainage characteristics (w), soil physical characteristics (s), and soil chemical fertility (f). The land suitability was ranked as S11 (96–100)—no limitation; S12 (86–95)—slight limitation; S2 (61–85)—moderate limitation; S3 (41–60)—severe limitation; N1 (20–40)—Very severe limitation that can be corrected; N2 (0–19)—very severe limitation that cannot be corrected. The overall soil suitability for rice production was assessed through the maximum limitation method where suitability is taken from the most limiting factor of soil characteristics (4, 47). The requirements of each kind of land use are obtained from Osinuga et al. (46) and Sys et al. (11). Table 3 show factor rating and rating values of soils.
2.4 Soil fertility assessments
Soil fertility was assessed using the soil fertility index (SFI) model (48–50). This procedure uses a numerical scale ranging between the highest value of 100 and the lowest value of 0 assigned to a particular characteristic of soil. If a characteristic was quite good for the intended crop, the highest rate of 100 was assigned to it, and, when it met some limitations, a lesser rate was assigned. The rating values were classified as per Saglam and Dengiz (49) (Table 4).
2.5 Correlation analysis
The relationship between the soil parameters was evaluated through correlation analyses using SPSS software.
3 Results and discussion
3.1 Soil morphological characteristics
Field survey involves soil observations (auguring and profiles) including topography; slope; and the nature of the parent materials, soil horizon depths, color, texture, vegetations, mottles, and soil biological activities. The key morphological properties of the profiles are shown in Table 1. The studied pedons show slight variation in drainage patterns, but the MKU-P1 pedon is poorly drained and very shallow (20 cm), while MKD-P1 is well drained and very deep (>160 cm). The soils are of friable moist consistency and are slightly hard to very hard when dry. Soil horizons were quite distinct in both pedons ranging from smooth clear, smooth diffused to gradual wavy with smooth horizon topography without any evidence of the rock outcrop and surface crusting throughout the profiles. However, few fine cracks were observed in the topsoil (1–10 cm) of the pedon MKD-P1. The topsoil color of the pedon MKU-P1 varies from gray (2.5Y5/1) when moist to dark gray (7.5YR4/1) when dried, and the subsoil color varies from light yellowish brown (2.5Y6/4) when moist to yellowish brown (10YR5/6) when dried with a predominance of a few fine to medium-yellowish mottles indicating the presence of reduced iron-oxide minerals probably due to the elluviation–illuviation process as a result of waterlogged conditions (51, 52). In MKD-P1, the topsoil color varies from dark brown (7.5YR3/2) when moist to very dark grayish brown (10YR3/2) when dried, and the subsoil varies from dark gray (5YR4/1) when moist to very dark-grayish brown (10YR3/2) when dried with very few faint to brownish-gray fine mottle characteristics of the vertisol soils that dry very rapidly when water saturation is minimized in the areas. Several researchers also observed the same trends of a distinct pattern of mottling associated with alternating conditions of the reduction and oxidation of sesquioxides of Al and Fe caused by seasonal waterlogging conditions (18, 23, 53). However, the observed waterlogging condition and soil structure of the pedons are favorable for the growth of the rice crops (46). Plant roots and a few earthworms were found in the studied pedons ranging from numerous fine roots and few earthworms in the topsoil and few fine roots in the subsoil.
3.2 Soil physical and chemical characteristics
Soil texture is an important characteristic that influences agricultural production, affecting crop selection, crop growth, soil moisture availability, erodibility, root penetration, and the movement of nutrients and water (54). Generally, the topsoil of both studied pedons had sandy clay loams and the sand contents decrease down the profiles, which may be due to the leaching of the finer particles as a result of an elluviation–illuviation process or washing out of the surface soil through erosion. The silt contents of the studied pedons did not show any regularity with depth and are low in all profiles compared to sand and clay. The pedon MKU-P1 has higher sand contents compared to MKD-P1. This result is consistent with observations by Kalala (18) that pedons were characterized by higher sand contents and an increase of clay with depth in the Kilombero valley. However, the subsoil of the pedon MKD-P1 has higher clay contents compared to MKU-P1. The silt–clay ratio is an indication of the susceptibility of the soils to detachment and transport that also depends on other properties like bulk density, soil organic matter, and climate (54). The silt–clay ratio of both studied pedons is higher (0.4) in top soils, which falls within the threshold level of 0.4 implying moderate resistance to erosion (54). The subsoil levels in all studied pedons fall below the threshold level of 0.4 (Table 5) indicating more susceptibility to weathering compared to the topsoil, probably due to the illuviation of clay contents that increases with depth (1, 23).
Soil bulk density describes the quality of the soils, and its information is crucial in the determination of soil compaction and root penetration (55). According to Landon (54), surface soils with bulk densities ranging from 1.1 to 1.4 Mg m-3 indicate that the soils are less compact and suitable for agricultural production. The pedon MKU-P1 has a lower bulk density value in the topsoil (0.8 Mg m-3) and varies from 1.36 to 1.39 Mg m-3 in the subsoil. Also, the pedon MKD-P1 has lower bulk in the topsoil (1.14 Mg m-3) and varies from 1.35 to 1.60 Mg m-3 in the subsoil. In both pedons, bulk density increases with depth (Table 6) and the values of the topsoil fall below the critical level of sandy loam for root restriction (1.8 Mg m-3) indicating that the soils are favorable for rice growth (56). Similar trends of increasing bulk density with depth were observed by Lufega and Msanya (57) working on the soil units of Morogoro District and Msanya et al. (3) working on the soil of Dodoma City, Tanzania. The lower bulk density in the topsoil might be due to the tillage and decomposed organic materials in the areas. The topsoil total porosity of the study areas ranged from 5.95% to 68.24%. According to Landon (54), the topsoil with a total porosity of more than 40% is favorable for root penetration. In both pedons, total porosity decreases with depth, which is inversely correlated with bulk density. This indicates less compaction in the surface soils compared to the subsoil, which may be attributed to higher organic matter contents and tillage. According to Lipiec and Hatano (58), soil porosity and pore size distributions are important determinants of water, nutrients, and gaseous exchanges within and throughout the root zone.
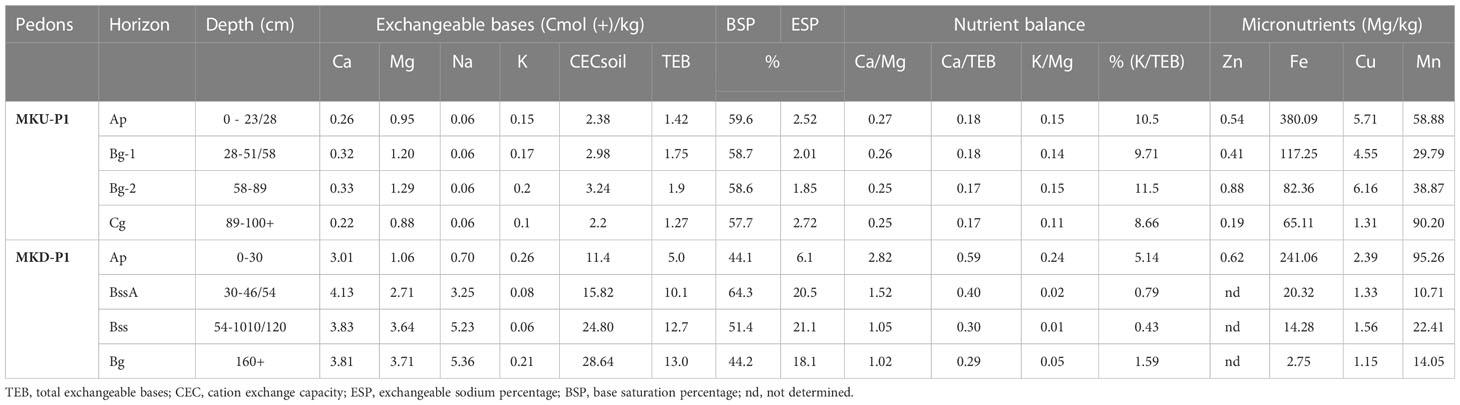
Table 6 Some exchangeable bases, micronutrients, and other related properties and the nutrient balance of the studied pedons.
Soil reaction (pH) is a very important chemical characteristic that may be used as a guide in assessing the suitability of soils for various stages of crop production (56). The pH of the study areas ranged from moderately acidic (5.75) to moderately alkaline (8.69). The MKU-P1 pedon has moderate acidity (5.7) in the topsoil, which increases the profile to slightly acidic (6.1–6.2). On the other hand, the topsoil of MKD-P1 has slight acidity (6.0), which also increases on moving the subsoil to moderately alkaline (8.1–8.6). The low pH (acidic) in the topsoil might be due to the leaching of some basic cations toward the subsoil leaving H+ in the topsoil or the nature of the parent materials and the contribution of Fe3+ oxide reduction to H+ consumption, which is far greater than the reduction of Mn4+- Mn3+ oxides and SO42- during the submerging (59). Guo et al. (60) reported that soil pH in paddy soil undergoes periodic changes during submergence, which is mainly attributed to the soil redox reactions. In each pedon, the value of pH(H2O) is higher than that of pH(KCl) (Table 5), an indication of a net negative charge in the soils (3).
Electrical conductivity is a measure of water-soluble salts in the soil suspension such as Na+, Ca2+, and Mg2+, which may be chlorides, sulfates, or carbonates; it is an indication of soil salinity (54). The values of EC in the topsoil of the study areas are very low and ranged between 0.1 and 0.07 dS/m. The topsoil values of exchangeable sodium percentage ranged between 2.5% and 6.1%, which falls below the range of sodic soils (less than 15%) (54). In MKU-P1, all values are below 15% indicating that the salinity effects of the area are negligible. However, in MKD-P1, the value increases toward the subsoil and ranges from 20.5% to 21.1%, which is sodic (56). According to Wakeel (61), soils with ESP ≥ 13 at the exchange sites but with low concentrations of total soluble salts (EC < 4 dS m–1) are described as sodic soils, and those with highly soluble salt concentrations of EC ≥ 4 dS m–1 and ESP ≥ 13 are termed saline-sodic soils. Hence the subsoil of the pedon MKD-P1 is sodic. However, since the topsoil is not sodic, the soil is suitable for rice production. Conversely, care must be taken to reduce the amount of sodium in the subsoil that in the future might rise and dominate the topsoil or form a hard layer and cause poor infiltrations and drainage in the subsoil (27).
Soil OC is one part of the larger global carbon cycle that involves carbon cycling in the soils and atmosphere. It affects the physical, chemical, and biological properties of the soil such as water infiltration, water-holding capacity, nutrient availability, soil structure, bulk density, and the activity of soil microorganisms (62). Generally, soil OC in the study areas ranged from very low (0.53%) to high (2.60%) (54). The low to high OC could be due to the limited use of organic manure and the burning of rice straws, which is a vital organic source of nutrients in the rice cropping systems. The studied pedon MKU-P1 has higher (2.6%) topsoil OC compared to MKD-P1, which decreases to very low (0.53%) in the subsoil. In contrast, the pedon MKD-P1 was moderate (1.73%) in OC in the topsoil and decreases to low (0.91%) in the subsoil. It was observed that OC decreased the profile possibly due to the influence of fresh organic matter such as dead roots on the topsoil. However, the trend of decrease is not regular in MKDP-1 resulting in higher OC in the Bg horizon (1.3%) than that of the BssA horizon (1.2%). This may be due to the clay texture of the lower subsoil compared to the sandy clay texture, indicating that clay plays an important role in OC stabilization, particularly in the subsoil (63).
The total nitrogen of the studied pedons ranged from medium (0.31%) to very low (0.06%) (54, 56). The pedon MKUP-1 is higher in the total nitrogen of the topsoil compared to MKDP-1, which varies from medium (0.31%) to very low (0.06%) whereas that of MKD-P1 varies from low (0.15%) to very low (0.05%). The low observed nitrogen levels may be attributed to erosion, leaching, and continued nutrient mining by plants. According to Fageria et al. (53), in an anaerobic soil environment, the major part of N is lost through leaching and denitrification. However, the N loss can be supplemented by the N fertilizer and or manure application. The C:N ratio is a measure of the relative nitrogen contents of organic materials, and it is a good indicator of the quality of organic materials that are very useful tools in the prediction of organic matter mineralization (26, 64). The topsoil C:N ratio of the study areas ranged between 8.6 and 11.7, which are below and close to the guideline value of 10:1 indicating that soils have good-quality organic matter (54).
The available P of the study areas ranged from very low (0.95 mg P/kg) to low 7.8 mg P/kg and falls within S3 and N1 classes indicating that soils have limited soil P that needs to be corrected for effective rice production (46). In MKU-P1, the P content of the topsoil is low (7.8 mg P/kg) and varies to very low (1.4–0.9 mg P kg-1) in the subsoil. The pedon MKD-P1 has very low P throughout the profile (Table 7). According to Landon (54), P-values ranging from 5 to 7 mg P kg-1 are problematic for rice production. The low available P in the soils of the study areas may be due to low-phosphorus parent materials or P fixation and the formation of insoluble compounds of Ca2+ as a result of alkaline soils in the case of the MKD-P1 subsoil or Al and Fe compounds in acid condition for MKU-P1 and the topsoil of MKD-P1(65, 66). Uwitonze (24) and Haryuni et al. (67) reported that P availability to plants is strongly influenced by the pH of the soils and maximized when the soil pH is between 5.5 and 7.5.
The CEC is a measure of the ability of the soil to hold positively charged ions and is an important property in overall assessments of soil fertility (56). According to a rating by Landon (54) the CEC in the topsoil of the study areas ranged from very low (2.38 cmol(+) kg-1) to low (11.4 cmol(+) kg-1) (Table 8), an indication of poor fertility and being unsuitable for irrigated agriculture. The values of CEC in MKU-P1 are very low throughout the profile, while that of MKD-P1 varies from low in the topsoil (11.4 cmol(+) kg-1) through a medium (15.82 cmol(+) kg-1) to high 28.64 cmol(+) kg-1 in the subsoil. Generally, the CEC increases with depth in pedon MKD-P1, whereas in MKU-P1, the increase is less pronounced; this may be due to higher clay contents in the subsoil of MKD-P1 and higher pH values (68). The low CEC values, in MKU-P1, may be due to the leaching of some basic cations. According to McLean (69), leaching leads to higher H+ in the soils; the H+ replaces the basic cations from the exchange complex of the soils and built-up exchangeable acidity that begins to attack the mineral crystal releasing Al3+. As some of the H+ degenerate into the minerals increasing the pH, as a result, hydroxyl-Al ions are formed, which are chelated by organic matter or polymerized on the cation exchange sites of the soil minerals. Additionally, Landon (54) reported that CEC is lowest at the soil pH of 3.5–4.0 and increases as the pH is increased by liming; the positive charges retain anions (negatively charged ions) such as chloride (Cl-) and sulfate (SO42-).
The exchangeable cations of the studied pedons ranged from very low (0.22 cmol (+) kg-1) to medium (4.13 cmol(+) kg-1) (Table 8). The pedon MKU-P1 is low in Ca2+ throughout the profile compared to MKD-P1 (56). Exchangeable Mg2+ in both pedons is high ranging from 0.88 to 3.64 cmol(+) kg-1 (54). The soil K+ reserve and its availability to plants are vital in determining the K-supplying capacity of soils (70). The concentration of K+ in both topsoils and subsoils was low and ranged from 0.06 to 0.26 cmol(+) kg-1 in the two pedons (54). Wakeel (61) reported that K+ concentration in soils depends on clay minerals and other exchangeable cations, especially Ca2+, Mg2+, and Na+. High concentrations of these cations in irrigated soils lead to K+ desorption, leaching, and even loss from the soil profile. Low K+ in soils might be due to low K+ inherent parent materials and/or excessive use of N and P fertilizers with insufficient K+ application.
The base saturation percentage (BSP) is defined as the sum of basic cations (Ca2+, Mg2+, K+, and Na+) over the total cation exchange capacity (71). It is an important chemical property in soil classification and soil fertility assessments that influences soil structure stability, nutrient availability, soil pH and soil response to fertilizers, and other soil amendments (25, 72). The values of the BSP in the topsoil of the study areas are moderate and ranged between 44.1% and 59.6% (54). According to Hazelton and Murphy (56), the soils with base saturation below 30% indicated strongly leached soils and the ones with BSP above 70% indicated very weakly leached soils. The individual pedon has the following BSP: The pedon MKU-P1 has a medium BSP throughout the profile that varies from 59.6% in the topsoil and decreases the profile to 57.7% (Table 6). Munsell Color (Firm), (2019) (34) reported that soils with low base saturation levels of <60% may result in very acidic soils and potentially toxic cations such as Al and Mn. The BSP in the topsoil of the pedon MKD-P1 is moderate (44.1%) but varies from high (64.3%) to moderate (44.2%) in the subsoils. The moderate BSP in both pedons might be due to poor cultivation practices, poor soil and water conservation, and an inadequate supply of fertilizer. The higher BSP in the subsoil of the pedon MKD-P1 might be due to the higher level of sodium concentration since, according to Landon (54), the base saturation percentage does not distinguish between different bases and imbalance may cause nutrition problems. However, based on descriptions picked from Osinuga et al. (46), all values of the BSP in the studied pedons are classified as suitable for rice production.
In assessing the soil requirement for plant nutrients, the relationship that exists between certain ions (Ca2+, Mg2+, and K+) and their balanced ratio must be determined to ensure proper plant growth (18). The exchangeable cations in the study areas have different trends of bases. In MKU-P1, the basic cations follow this trend of Mg > Ca > K > Na, while the trend in MKD-P1 is K+ > Ca2+ > Mg2+ > Na+ (Table 8). The result of the pedon MKU-P1 is consistent with Kalala (18), working on the soils of the Kilombero valley, who also observed higher Mg2+ in the soils. In both pedons, the Ca/TEB ratio is below 0.5 throughout the profiles indicating that Ca2+ has no effects on the uptake of other cations such as K+ and Mg2+ (31). The Ca/Mg ratio in the studied pedons ranges from 0.25 to 2.82, and all are within favorable levels (1.2–5.2) for crop production (1, 23). The Mg2+/K+ ratios in pedons MKU-P1 and MKD-P1 vary from 0.11 to 0.15 and 2.1 to 5.09, respectively. Based on the rating by Landon (54), all Mg2+/K+ ratios of the pedon MKU-P1 are within the favorable level for rice production. However, the topsoil of the pedon MKD-P1 and the last subsoil is above the critical level (1–4), implying potential nutrient imbalance and toxicity, hence causing Mg2+ deficiency due to a higher K level. According to Landon (54), the minimum level of the K/TEB ratio is 2% to avoid K+ deficiency and above 25%, which is rare to occur; the soils will have similar effects to high Na. The K/TEB ratio of the studied pedons ranges from favorable (9.71%) to unfavorable (55.4%). All values of K/TEB in MKU-P1 fall within the favorable levels, and those of MKD-P1 fall within the unfavorable levels (Table 10). This finding indicated that the levels of K in the pedon MKD-P1 have nutrient imbalance and the K+ level may induce the deficiency of other cations such as Ca2+ and Mg2+.
The value of Cu of the studied pedons ranged from 1.15 to 5.7 mg kg-1 which is under the sufficient level of 0.2 mg kg-1 for optimum plant growth (54, 73). The topsoil Zn levels ranged from 0.5 to 0.6 mg kg-1. Based on categorization by Landon (54), both Zn levels fall within the sufficient range of 0.5 mg kg-1 for rice growth. However, the studied pedon MKD-P1 has a low level of Cu throughout the subsoil that was below the detection limit. All values of Fe (2.75–380.09 mg kg-1) and Mn (10.71–95.26 mg kg-1) were within the sufficient range for plant growth (23, 54).
3.3 Soil classification
Based on field and laboratory data, the pedons were classified according to the family level of the USDA Soil Taxonomy and Tier-2 of the WRB (44, 45). The diagnostic horizons, diagnostic properties, and other diagnostic materials are shown in Table 7. The soil of the pedons of MKU-P1 is classified as Inceptisols per the USDA and Fluvisols in FAO-WRB soil classifications with Mollic epipedon having redoximorphic features and glayic properties as a result of frequent irrigation and flooding. The soil of the pedon MKD-P1 is classified as Vertisols in both USDA and FAO-WRB soil classifications having vertic horizons with shrink–swell cracks in the upper horizon and very hard consistency. The details of classifications are shown in Table 8.
3.4 Soil-site suitability for rice production
The suitability of land for rice crop production was assessed based on climatic and physiochemical properties that represent the fertility states of the soil (Table 9). The slopes and climatic conditions of the study areas are rated as highly suitable for rice production (S11) in terms of rainfall, and temperature while drainage is rated S3 for MKU-P1 and N for MKD-P1. The textural classes of the topsoil are sandy clay loam and sandy clay, or clay in the subsurface horizons is rated as S11 in both pedons. The base saturation is rated as S11 for the pedon MKD-P1 and S2 for MKU-P1. The pedon MKU-P1 is low in CEC (cmol(+) kg-1) and low exchangeable K+ that is rated within N1 and S3, while the pedon MKD-P1 is rated within S12 for both CEC (cmol(+) kg-1) and exchangeable K+. The land included in these classes of CEC has certain fertility limitations that reduce crop performances unless appropriate nutrient management is taken into consideration (47). Using the FAO land suitability evaluation based on climate and topography the areas were potentially suitable for rice production (11, 46) with soil fertility limitation factors such as total N and available P, K+, and Ca2+ that might be due to poor agricultural practices associated with low organic matter application, nutrient imbalance, inappropriate water management, and increased carbon release (6, 74). These results were consistent with other studies carried out in some rice irrigation schemes in Tanzania (17, 75, 76). The areas can be ameliorated through the management strategies of applying inorganic and organic fertilizers with good agricultural practices (77–79).
3.5 Soil fertility status of the study areas
The textural classes of the studied pedons ranged from sandy clay for MKU-S1 and MKU-S3, sandy loam for MKU-S2 and MKD-S1, and sandy clay loam for MKD-S2, MKD-S3, and MKD-S4. Based on rice requirements, all textural classes are favorable for rice production (46). The pH of the surface soils of the pedon MKU-P1 is very strongly acidic and varies from pH 4.62–4.83 while the pH of MKD-P1 ranged from very strongly acidic (4.78) to neutral (6.9). Results indicated that 100% of the surface soils in pedon MKU-P1 and 25% for MKD-P1 were low in pH falling within the N class indicating low fertility. The low pH might be due to the leaching of basic cations, resulting in a high concentration of hydrogen ions, manganese, and aluminum on soil colloids (80). Soils with low pH (<5.5) are potentially great for Mn, Al, and Fe toxicity and the deficiency of some essential nutrients, hence poor soil fertility (53). The OC of the surface soils ranged from moderate (1.28%) in the MKD-S2 pedon to high (2.17%) in the MKD-S4 pedon (57). In both pedons, OC increases down the slope, and this may be associated with the erosion of organic materials from the upper to lower slope. The low OC in the studied areas might have resulted from poor management techniques of organic matter by poor farming practices (81). Kalala (18) observed similar trends of low OC in the Kilombero valley and related this with increasing soil CEC and activity of soil microbes. Integrating organic materials can contribute to replenish fertility levels, thereby enhancing mineralization and the release of essential plant nutrients (48–50). Merumba et al. (23) reported that the critical level of N that would support the growth of the majority of crops in Tanzanian soils is 2.0 g kg-1. The low nitrogen in the studied pedons could be associated with low N fertilizer input, erosion, and volatilization due to the anaerobic environments of the soils (82, 83). The phosphorus of the surface soils varies from very low (0.68 mg kg-1) in MKU-S3 to low (8.8 mg kg-1) in MKD-S4. Based on P requirements, 66.6% and 50% of the soils were classified as poor in fertility (N), and 33.3%–50% were classified as marginal in fertility (S3) in MKU-P1 and MKD-P1 pedons, respectively. The low P content in the studied soils might be due to the low inherent P of the parent rocks and P fixations with Al and Fe as a result of the low pH of the soils (84). According to Landon (54), the low P level in soils can be corrected by the application of both inorganic and organic fertilizers. However, organic matter improves soil pH and enhances P availability (23). Based on cation exchange capacity, 100% of the surface soils in both pedons have Mg2+ concentration below the required level for fertile soils that fall within the N class indicating poor fertility (48–50). The concentrations of Ca2+ in all soils of the pedons MKU-P1 and only 25% of the MKD-P1 soils ranked within the N class, indicating poor fertility. The remaining 75% of the soils in MKD-P1 are within the S3 class, suggesting a marginal fertile status. In both pedons, the exchangeable sodium percentage of the surface soils is less than 13, indicating that the soils are not sodic (61).
Extractable Zn contents in the soils of the pedon MKU-P1 varied from 0.38 mg kg-1 in MKU-S3 to 1.06 mg kg-1 in MKU-S2, while that of MKD-P1 soil varies from 0.12 mg kg-1 in MKD-S3 to 2.37 mg kg-1 in MKD-S4 (Table 15). The critical level for Zn deficiency in the soil is 0.4–0.6 mg kg-1, and values higher than 10–20 mg kg-1 are regarded as excess (85). The results show that 66.6% and 50% of soils are ranked as S1, while 33.3% and 50% are ranked as N in MKU-P1 and MKD-P1 pedons, respectively, indicating adequate and deficient Zn levels. The surface soils in both sites are high in extractable Fe (>4.5 mg kg-1), falling within the N classes (80). The high extractable Fe may be due to the nature of the parent materials, which are siliceous and ferruginous with low Mg and K contents (23). According to Hazelton and Murphy (56), Fe concentration (>400 mg kg-1) is associated with toxicity and its management is vital to minimize nutrient loss. Management techniques include the use of organic matter, inorganic fertilizer, and liming of soils with CaCO3 and or MgCO3 or CaMg(CO3) to increase pH (23). The extractable Cu of the surface soils ranges from 4.28 to 6.92 mg kg-1 and 0.97 to 2.81 mg kg-1 for MKU-P1 and MKD-P1, respectively. The concentration of Cu is 0.2 mg kg-1, which is above the required amount for plant growth (54). Extractable Mn ranges from 11.56 to 73.29 mg kg-1; according to the rating by Saglam and Dengiz (49), with exception of MKD-S3, all other soils are sufficient in Mn (15–50 mg kg-1), falling within S1 class of fertile soils. These results are in agreement with Bissah et al. (86); Dai and Dong (87), and Kouadio et al. (71) who demonstrated a rapid decline in soil chemical fertility following intensive cultivation with inappropriate use of organic matter and suggested that for effective soil fertility improvement plans, the sole application of a mineral fertilizer is unlikely to succeed.
4 Conclusions and recommendations
The objective of this study was to classify and investigate the suitability and fertility of two lowland rice irrigation schemes in Morogoro region, Tanzania. The results of the study revealed that both studied pedons were ranked as suitable for rice production. However, some chemical fertility such as total N, available P, OC, and exchangeable bases were in a state of degradation and less favorable for rice production. The areas will benefit if proper soil fertility management practices will be employed with the following recommendations:
i. The application of the nitrogen fertilizer such as urea in 100 kg N ha-1 in split application to avoid severe nitrogen loss.
ii. The application of the P fertilizer such as diammonium phosphate, and triple super phosphate at a rate of 30 kg P ha-1 at a time of rice transplanting.
iii. Avoid burning straws and incorporate them into the soils together with the application of organic manure to improve soil OC, which will aid in improving CEC and soil health.
iv. iv. Improving drainage systems in the areas enhances the removal of irrigated water and minimizes the salinity stress that may develop from the subsoil.
v. Crop rotation with legumes improves soil fertility by nitrogen fixation and minimizes rice diseases.
Data availability statement
The original contributions presented in the study are included in the article/supplementary material. Further inquiries can be directed to the corresponding author.
Author contributions
This work was carried out in collaboration among all authors. All authors contributed to the article and approved the submitted version.
Conflict of interest
The authors declare that the research was conducted in the absence of any commercial or financial relationships that could be construed as a potential conflict of interest.
Publisher’s note
All claims expressed in this article are solely those of the authors and do not necessarily represent those of their affiliated organizations, or those of the publisher, the editors and the reviewers. Any product that may be evaluated in this article, or claim that may be made by its manufacturer, is not guaranteed or endorsed by the publisher.
References
1. Karuma A, Gachene C, Msanya B, Mtakwa P, Amuri N, Gicheru P. Soil morphology, physico - chemical properties and classification of typical soils of mwala district, Kenya. Int J Plant Soil Sci (2015) 4(2):156–70. doi: 10.9734/ijpss/2015/13467
2. Massawe IH, Msanya BM, Rwehumbiza FB. Pedological characterization and fertility evaluation of paddy soils of mvumi village, kilosa district, Tanzania. Int J Curr Res Biosci Plant Biol (2017) 4(4):49–60. doi: 10.20546/ijcrbp.2017.404.009
3. Msanya BM, Mwasyika TA, Amuri N, Semu E, Mhoro L. Pedological characterization of typical soils of Dodoma capital city district, Tanzania: soil morphology, physico-chemical properties, classification and soil fertility trends. Ann Advanced Agric Sci (2018) 2(4):59–71. doi: 10.22606/as.2018.24002
4. Bock M, Gasser P, Pettapiece WW, Brierley AJ, Bootsma A, Schut P, et al. The land suitability rating system is a spatial planning tool to assess crop suitability in Canada. Front Environ Sci (2018) 6:1–16. doi: 10.3389/fenvs.2018.00077
5. Belal AB, Sciences S, Sciences S, Abu-hashim M. Land evaluation based on GIS-spatial multi-criteria evaluation (SMCE) for agricultural development in dry wadi, Eastern Desert. Int. J Sci (2015) 3:100–16. doi: 10.3923/ijss.2015.100.116
6. El Baroudy AA, Ali AM, Mohamed ES, Moghanm FS, Shokr MS, Savin I, et al. Modeling land suitability for rice crop using remote sensing and soil quality Indicators : the case study of the Nile delta. (2020). doi: 10.3390/su12229653
7. Mueller L, Schindler U, Mirschel W, Graham Shepherd T, Ball BC, Helming K, et al. Assessing the productivity function of soils. a review. Agron Sustain Dev (2010) 30(3):601–14. doi: 10.1051/agro/2009057
8. Prudat B, Bloemertz L, Kuhn NJ. Local soil quality assessment of north-central Namibia: integrating farmers’ and technical knowledge. Soil (2018) 4(1):47–62. doi: 10.5194/soil-4-47-2018
9. El Baroudy AA. Catena mapping and evaluating land suitability using a GIS-based model. Catena (2016) 140:96–104. doi: 10.1016/j.catena.2015.12.010
10. FAO. The FAO guidekines for land evaluation. In: Land use, land cover and soil sciences, II (2007). p. 1–9. Available at: https://www.eolss.net/Sample-Chapters/C12/E1-05-02-03.pdf.
11. Sys C, Van Ranst E, Debaveye J. Land evaluation, part I: principles in land evaluation and crop production calculations. In: Agricultural publications-N0 7, General Administration for Development Cooperation Brussels. Belgium: ISRIC LIBRARY (1991). p. 280.
13. Kaloi FK, Isaboke HN, Onyari CN, Njeru LK. Comparing productivity of rice under system of rice intensification and conventional flooding : a switching regression approach. African J Agric Res (2020) 16(10):1355–63. doi: 10.5897/AJAR2020.14921
14. Mboyerwa PA, Kibret K, Mtakwa P, Aschalew A. Rice yield and nitrogen use efficiency with system of rice intensification and conventional management practices in mkindo irrigation scheme, Tanzania. Front Sustain Food Syst (2022) 6:802267. doi: 10.3389/fsufs.2022.802267
15. Van Nguyen N, Ferrero A. Meeting the challenges of global rice production. Paddy Water Environ (2006) 4:1–9. doi: 10.1007/s10333-005-0031-5
16. Senthilkumar K, Sillo FS, Rodenburg J, Dimkpa C, Saito K, Dieng I, et al. Rice yield and economic response to micronutrient application in Tanzania. Field Crops Res (2021) 270:108201. doi: 10.1016/j.fcr.2021.108201
17. Hussein B, Massawe J, Slater B, Dick W, Lindsey L. Digital soil mapping and GIS-based land evaluation for rice suitability in kilombero. (2017). Available: https://www.proquest.com/openview/666013e1fdc137d99bda5ca960df793e/1?pq-origsite=gscholar&cbl=18750.
18. Kalala AM. Pedological characterization of some typical alluvial soils of kilombero district, Tanzania. Am J Agric Forestry (2017) 5(1):1. doi: 10.11648/j.ajaf.20170501.11
19. Amuri NA, Mhoro L, Mwasyika T, Semu E. Potential of soil fertility management to improve essential mineral nutrient concentrations in vegetables in Dodoma and kilombero, Tanzania. J Agric Chem Environ (2017) 06(02):105–32. doi: 10.4236/jacen.2017.62007
20. Msofe NK, Sheng L, Li1 Z, Lyimo J, & Lingyan W. Influence of agricultural land use change on the selected physico-chemical soil properties in kilombero valley floodplain, southeastern Tanzania. Int J Environ Sci Natural Resour (2019) 21(5):556074. doi: 10.19080/ijesnr.2019.21.556074
21. Alavaisha E, Manzoni S, Lindborg R. Different agricultural practices affect soil carbon, nitrogen and phosphorous in kilombero -Tanzania. J Environ Manage (2019) 234(December 2018):159–66. doi: 10.1016/j.jenvman.2018.12.039
22. Senthilkumar K, Tesha BJ, Mghase J, Rodenburg J. Increasing paddy yields and improving farm management: results from participatory experiments with good agricultural practices (GAP) in Tanzania. Paddy Water Environ (2018) 16(4):749–66. doi: 10.1007/s10333-018-0666-7
23. Merumba MS, Michael Msanya B, Semu E, Mashambo Semoka J. Pedological characterization and suitability assessment for cassava production in bukoba, missenyi and biharamulo districts, Tanzania. Am J Agric Forestry (2020) 8(4):144. doi: 10.11648/j.ajaf.20200804.18
24. Uwitonze P. Pedological characterization of soils developed from volcanic parent materials of northern province of Rwanda. Agricult Forestry Fisheries (2016) 5(6):225. doi: 10.11648/j.aff.20160506.13
25. Rawal A, Chakraborty S, Li B, Lewis K, Godoy M, Paulette L, et al. Determination of base saturation percentage in agricultural soils via portable X-ray fluorescence spectrometer. Geoderma (2019) 338:375–82. doi: 10.1016/j.geoderma.2018.12.032
26. Mohamed SH, Michael Msanya B, Juma Tindwa H, Semu E. Pedological characterization and classification of selected soils of morogoro and mbeya regions of Tanzania. Int J Natural Resource Ecol Manage (2021) 6(2):79. doi: 10.11648/j.ijnrem.20210602.17
27. Mbaga HR, Mrema JP, Balthazar, Msanya M. Response of rice to nitrogen and phosphorus applied on typical soils of dakawa irrigation scheme, morogoro, Tanzania. Imperial J Interdiscip Res (IJIR (2017) 3:378–84.
28. Msanya BM, Kaaya AK, Araki S, Otsuka H, Nyadzi GI. Pedological characteristics, general fertility and classification of some benchmark soils of morogoro district, Tanzania. Afr J Sci Technol (AJST) Sci Eng Ser (2003) 4(2):101–12.
29. Makoi JH, Mmbaga H. Soil fertility characterization in mvumi and mbogo - komtonga irrigation schemes in kilosa and mvomero districts, morogoro region, Tanzania. Int J Environment Agric Biotechnol (2018) 3(3):1088–99. doi: 10.22161/ijeab/3.3.49
30. Stewart ZP, Pierzynski GM, Middendorf BJ, Vara Prasad PV. Approaches to improve soil fertility in sub-Saharan Africa. J Exp Bot (2020) 71(2):632–41. doi: 10.1093/jxb/erz446
31. Kebeney S, Msanya B, Ng’etich W, Semoka J, Serrem C. Pedological characterization of some typical soils of busia county, Western Kenya: soil morphology, physico-chemical properties, classification and fertility trends. Int J Plant Soil Sci (2015) 4(1):29–44. doi: 10.9734/ijpss/2015/11880
32. Alaivasha E, Tumbo M, Senyangwa J, Mourice S. Influence of water management farming practices on soil organic carbon and Nutrients : a case study of rice farming. Agronomy (2022). doi: 10.3390/agronomy12051148
33. Daliya P, Ljungqvist O, Brindle ME, Lobo DN. Guidelines for guidelines. Guidelines for Guidelines. Enhanced Recovery After Surgery, 4th edition (2020). doi: 10.1007/978-3-030-33443-7_3
34. Munsell Color (Firm). Munsell soil color book: with genuine Munsell color chips (2009 year rev. 2019 production). Munsell Color (2019).
35. Beretta AN, Silbermann AV, Paladino L, Torres D, Bassahun D, Musselli R, et al. Análisis de textura del suelo con hidrómetro: modificaciones al método de bouyoucus. Ciencia e Investigacion Agraria (2014) 41(2):263–71. doi: 10.4067/S0718-16202014000200013
36. Rochette P, Bertrand N. Soil-surface gas emissions. In: Carter Taylor M. R., Francis group LLC Soil sampling and methods of analysis: second edition. Canada (2007). doi: 10.1201/9781420005271.ch65
37. Okalebo JR, Gathua KW, Paul LW. Laboratory methods of soil and plant analysis: a working manual the second edition. SACRED Africa, Kenya Any (2002), 1–131. [Online]. Available: https://books.google.co.tz/books/about/Laboratory_Methods_of_Soil_and_Plant_Ana.html?id=BRWKAAAACAAJ&redir_esc=y.
38. Walkley A, Black IA. An examination of the degtjareff method for determining soil organic matter, and a proposed modification of the chromic acid titration method. Soil Sci (1934) 37(1):29–38). doi: 10.1097/00010694-193401000-00003
39. Nelson D, Sommers L. Chemical methods soil science society of America book series. Bigham JM, editor. Madison WI, USA: Soil Science Society of America (1996) p. 961–1010. Available at: https://www.waterboards.ca.gov/waterrights/water_issues/programs/bay_delta/california_waterfix/exhibits/docs/Islands/II_41.pdf. Methods of Soil Analysis. Part 3. Chemical Methods, SSSA.
40. Bray RH, Kurtz LT. Determination of total, organic and available forms of phosphorus in soils. Soil Sci (2009) 59:39–45. doi: 10.1097/00010694-194501000-00006
41. Mattigod SV, Zachara JM. METHODS OF SOIL ANALYSIS PART 3 chemical methods soil science society of America book series. In J.B. D., Cheng S. B. H. H., Westerman R. L. Methods of Soil Analysis Madison WI, USA (1996), 53711.
42. Edmeades DC, Clinton E. A simple rapid method for the measurement of exchangeable cations and effective cation exchange capacity. Commun Soil Sci Plant Anal (1981) 12(7):683–95. doi: 10.1080/00103628109367184
43. Lindsay WL, Cox FR. 7. micronutrient soil testing for the tropics. Fertilizer Res (1985) 7(1–3):169–200. doi: 10.1007/BF01049000
44. Soil Survey Staff. Soil survey manual agriculture. handbook no. 18. United States Department of Agriculture, Washington D.C.: USDA, Natural Resources Conservation Servicen (2017) 18:483. Available at: https://www.nrcs.usda.gov/wps/portal/nrcs/detail/soils/ref/?cid=nrcs142p2_054262.
45. IUSS Working Group WRB. World reference base for soil resources, update 2015: international soil classification sysytem for naming soils and creating lengends for soil maps. world soil report no. 106. Rome Italy: Food and Agricultural Organization (2014) 4:203. doi: 10.1038/nnano.2009.216
46. Osinuga OA, Aiboni VU, Oyegoke CO, Management L, Production, C. CLASSIFICATION AND SUITABILITY EVALUATION OF SOILS ALONG a classification and suitability evaluation of soils for rice production in southwest, Nigeria. J Trop Agric Food, Environ Ext (2020) 19(4):43–50. doi: 10.4314/as.v19i4.8
47. Saleh AM, Belal AB, Mohamed ES. Land resources assessment of El-galaba basin, south Egypt for the potentiality of agriculture expansion using remote sensing and GIS techniques. Egyptian J Remote Sens Space Sci (2015) 18(1):S19–30. doi: 10.1016/j.ejrs.2015.06.006
48. Fresco LO, Huizing H, van Keulen H, Luning H, Schipper R. Land evaluation and farming systems analysis for land use planning 3rd ed. FAO Working Document, FAO, Rome, Rome, Italy (1994) 13(4):622–37.
49. Saglam M, Dengiz O. Distribution and evaluation of soil fertility based on geostatistical approach in bafra deltaic plain. Türkiye Tarımsal Araştırmalar Dergisi (2014) 1(2):186. doi: 10.19159/tutad.27089
50. Khaki BD, Honarjoo N, Davatgar N, Jalalian A, Golsefidi HT. Assessment of two soil fertility indexes to evaluate paddy fields for rice cultivation. Sustainability (Switzerland) (2017) 9(8):1299. doi: 10.3390/su9081299
51. Singer A. Soil horizon designations in the wrb soil classification system. In Chesworth W. ed Encyclopedia of Soil Science. Encyclopedia of Earth Sciences Series Springer, Dordrecht (2008), 668–70. doi: 10.1007/978-1-4020-3995-9_541
52. Callesen I., Brændholt A., Schrumpf M., Vesterdal L., Magnussen A., Vorenhout M., et al. A high-resolution digital elevation model in combination with water table depth and continuous soil redox potential measurements explain soil respiration and soil carbon stocks at the ICOS site sorø. Front For Glob Chang (2021) 3:1–16. doi: 10.3389/ffgc.2020.563355
53. Fageria NK, Carvalho GD, Santos AB, Ferreira EPB, Knupp AM. Chemistry of lowland rice soils and nutrient availability. Commun Soil Sci Plant Anal (2011) 42(16):1913–33. doi: 10.1080/00103624.2011.591467
54. Landon JR. Booker tropical soil manual: a handbook for soil survey and agricultural land evaluation in the tropics and subtropics. (New York and London: Routledge Taylor & Francis Group) (1991).
55. Chaudhari PR, Ahire DV, Ahire VD, Chkravarty M, Maity S. Soil bulk density as related to soil texture, organic matter content and available total nutrients of coimbatore soil. Int J Sci Res Publications (2013) 3(1):2250–3153.
56. Hazelton P, Murphy B. Interpreting soil test results. Interpreting Soil Test Results (2019) 169. doi: 10.1071/9781486303977
57. Lufega S, Msanya B. Pedological characterization and soil classification of selected soil units of morogoro district, Tanzania. Int J Plant Soil Sci (2017) 16(1):1–12. doi: 10.9734/ijpss/2017/32681
58. Lipiec J, Hatano R. Quantification of compaction effects on soil physical properties and crop growth. Geoderma (2003) 116(1–2):107–36. doi: 10.1016/S0016-7061(03)00097-1
59. Lu H, Li K, Nkoh JN, He X, Xu R, Qian W, et al. Effects of pH variations caused by redox reactions and pH buffering capacity on Cd(II) speciation in paddy soils during submerging/draining alternation. Ecotoxicol Environ Saf (2022) 234(February):113409. doi: 10.1016/j.ecoenv.2022.113409
60. Guo T, Zhang Q, Ai C, Liang G, He P, Zhou W. Nitrogen enrichment regulates straw decomposition and its associated microbial community in a double-rice cropping system. Sci Rep (2018) 8(1). doi: 10.1038/s41598-018-20293-5
61. Wakeel A. Potassium-sodium interactions in soil and plant under saline-sodic conditions. J Plant Nutr Soil Sci (2013) 176(3):344–54. doi: 10.1002/jpln.201200417
62. Casci T. Evolution: arabidopsis’ hidden potential. Nat Rev Genet (2008) 9(4):248. doi: 10.1038/nrg2350
63. Niang A. Organic matter stocks under different types of land use in the peanut basin of the nioro area, Senegal. Senegal: Wageningen University and Research Center (2004).
64. Bird JA, Horwath WR, Eagle AJ, Van Kessel C. Immobilization of fertilizer nitrogen in rice: effects of straw management practices. Soil Sci Soc Am (2001) 65:1143–52. doi: 10.2136/sssaj2001.6541143x
65. Nagumo T, Tajima S, Chikushi S, Yamashita A. Phosphorus balance and soil phosphorus status in paddy rice fields with various fertilizer practices. Plant Production Sci (2013) 16(1):69–76. doi: 10.1626/pps.16.69
66. Jiang B, Shen J, Sun M, Hu Y, Jiang W, Wang J, et al. Soil phosphorus availability and rice phosphorus uptake in paddy fields under various agronomic practices. Pedosphere (2021) 31(1):103–15. doi: 10.1016/S1002-0160(20)60053-4
67. Haryuni, Kamalasari A, Widijanto H, Supriyadi. Soil fertility index on various rice field management systems in central Java, Indonesia. Am J Agric Biol Sci (2020) 15(1):75–82. doi: 10.3844/ajabssp.2020.75.82
68. Donn MJ, Menzies NW, Rasiah V. Chemical characterisation of deep profile ferrosols under sugarcane in wet tropical northern Queensland. Aust J Soil Res (2004) 42(1):69–77. doi: 10.1071/SR03052
69. McLean EO. Principles underlying the practice of determining lime requirements of acid soils by use of buffer methods. Commun Soil Sci Plant Anal (1978) 9(8):699–715. doi: 10.1080/00103627809366845
70. Li T, Wang H, Zhou Z, Chen X, Zhou J. A new grading system for plant-available potassium using exhaustive cropping techniques combined with chemical analyses of soils. Sci Rep (2016) 6(October):1–9. doi: 10.1038/srep37327
71. Koné I, Kouadio K-KH, Kouadio EN, Agyare WA, Owusu-Prempeh N, Amponsah W, et al. Assessment of soil fertility status in cotton-based cropping systems in Cote d ‘ Ivoire. Front Soil Sci (2022) 2:959325. doi: 10.3389/fsoil.2022.959325
72. Arshad MA, Martin S. Identifying critical limits for soil quality indicators in agro-ecosystems. Agricult Ecosyst Environ (2002) 88(2):153–60. doi: 10.1016/S0167-8809(01)00252-3
73. Mbaga HR, Msanya BM, Mrema JP. Pedological characterization of typical soil of dakawa irrigation scheme, mvomero district, morogoro region, Tanzania. Int J Curr Res Biosci Plant Biol (2017) 4(6):77–86. doi: 10.20546/ijcrbp.2017.406.009
74. Abate K, Mohammed M, Kibret K. Soil fertility assessment and mapping of spatial variability at amareganda-abajarso Sub-watershed, north-Eastern Ethiopia. East Afr J Sci (2016) 10(1):1–14.
75. Mwamba Kalala A. Pedological characterization of some typical alluvial soils of kilombero district, Tanzania. Am J Agric Forestry (2017) 5(1):1. doi: 10.11648/j.ajaf.20170501.11
76. Mfaume DP, Msanya BM, Msaky JJ. Pedological characterization and fertility assessment of mbimba substation soils under coffee production in mbozi district, Tanzania. Int J Adv Sci Res Eng (2019) 05(10):221–36. doi: 10.31695/ijasre.2019.33540
77. Balasubramanian V, Sie M, Hijmans RJ, Otsuka K. Increasing rice production in Sub-Saharan Africa: challenges and opportunities. In: Advances in agronomy. India: Elsevier Masson SAS, Velandipalayam Post, Coimbatore (2007) 94:641025. doi: 10.1016/S0065-2113(06)94002-4
78. Hikmatullah, Al-Jabri M. Soil properties of the alluvial plain and its potential use for agriculture in donggala region. Soil Properties Alluvial Plain Donggala (2007), 1973. [Online] Available: https://media.neliti.com/media/publications/64187-EN-soil-properties-of-the-alluvial-plain-an.pdf.
79. Nishigaki T, Sugihara S, Kobayashi K, Hashimoto Y, Kilasara M, Tanaka H, et al. Fractionation of phosphorus in soils with different geological and soil physicochemical properties in southern Tanzania. Soil Sci Plant Nutr (2018) 64(3):291–9. doi: 10.1080/00380768.2018.1436406
80. Biosci IJ, Stanley Kiriba D, Mtei K, Wichern F, Alois Ndakidemi P. Soil fertility status of smallholder farms in northern highlands. Tanzania Int J Biosci | IJB |. (2021) 18(3):104–19. doi: 10.12692/ijb/18.3.104-119
81. Hague SA, Chowdhury L. Effects of rice straw and sulphur on the growth and yield of rice. J Bangladesh Agril. Univ (2004) 2(1):15–8.
82. Damodar Reddy D, Sharma KL. Effect of amending urea fertilizer with chemical additives on ammonia volatilization loss and nitrogen-use efficiency. Biol Fertility Soils (2000) 32(1):24–7. doi: 10.1007/s003740000208
83. Jones CB, Brad DE, Engel R, Horneck D, Olson-Rutz K, Brown BD, et al. Nitrogen fertilizer volatilization. Montana State Univ Extension (2013) EBO208, 1–6.
84. Yin Y, Liang CH. Transformation of phosphorus fractions in paddy soil amended with pig manure. J Soil Sci Plant Nutr (2013) 13(4):809–18. doi: 10.4067/S0718-95162013005000064
85. Ndakidem PA, Semoka JM. Soil fertility survey in Western usambara northern tanzania*’ mountains. Pedosphere (2006) 16(2):237–44. doi: 10.1016/S1002-0160(06)60049-0
86. Bissah MN, Kotey DA, Tongoona P, Egbadzor KF, Gracen V, Danquah EY. Factors influencing rice production in the south-eastern belt of Ghana. Heliyon (2022) 8(12):e12404. doi: 10.1016/j.heliyon.2022.e12404
Keywords: soil fertility, soil classification, paddy soils, pedons, soil health
Citation: Marzouk SH, Tindwa HJ, Massawe BHJ, Amuri NA and Semoka JM (2023) Pedological characterization and soil fertility assessment of the selected rice irrigation schemes, Tanzania. Front. Soil Sci. 3:1171849. doi: 10.3389/fsoil.2023.1171849
Received: 22 February 2023; Accepted: 11 April 2023;
Published: 24 May 2023.
Edited by:
Ahmed Abd El Fattah El Baroudy, Tanta University, EgyptReviewed by:
Mohamed Shokr, Tanta University, EgyptElsayed Said Mohamed, National Authority for Remote Sensing and Space Sciences, Egypt
Copyright © 2023 Marzouk, Tindwa, Massawe, Amuri and Semoka. This is an open-access article distributed under the terms of the Creative Commons Attribution License (CC BY). The use, distribution or reproduction in other forums is permitted, provided the original author(s) and the copyright owner(s) are credited and that the original publication in this journal is cited, in accordance with accepted academic practice. No use, distribution or reproduction is permitted which does not comply with these terms.
*Correspondence: Said H. Marzouk, binmarzouk@gmail.com