- 1Division of Soil Science & Agricultural Chemistry, Indian Council of Agricultural Research (ICAR)-Indian Agricultural Research Institute, New Delhi, India
- 2Department of Soil Science and Agricultural Chemistry, Institute of Agricultural Science, Banaras Hindu University, Varanasi, India
Introduction: Soil quality deterioration with the introduction of modern agriculture is a major threat to agricultural sustainability and food security and the problem is more aggravated specially under rainfed agriculture. Asessment of soil quality is a tortuous task as it can not be measured directly. The objective of the present investigation was to evaluate the effect of long-term fertilization and manuring on soil quality and identify the most sensitive indicators of assessing soil quality under rainfed rice based system.
Methods: Soil samples were collected from selected six treatments viz. control, 100%NPK, 50%NPK, 50%FYM, 100%FYM and 50%NPK+FYM of 34 years old long-term fertilizer experiment with rainfed rice-lentil cropping system situated at BHU Varanasi, India.
Results and discussion: Result revealed that continuous organic manure application along with inorganic fertilizer increased soil organic carbon by 54.1% over control treatment.Principal component analysis (PCA) was done to screen out key indicators and mean weight diameter, available Fe, available N, potentially mineralizable N, available Zn, FDA hydrolase activity and Clay were selected as key indicators of soil quality. The highest soil quality index (SQI) of 0.95 was found in 50% NPK+FYM treatment. Regression analysis showed better agreement of equivalent rice yield and SQI (0.87). Therefore, the balanced fertilization with organic and inorganic fertilizers is important for sustainability of the rainfed rice-lentil cropping system and this practice may be recommended for rainfed rice based system of Indian Inceptisol.
1 Introduction
In the modern agricultural system, securing a sustainable source of income has arisen as the most crucial public concern. Richardson et al. (1) reported that there is need to guarantee food security without jeopardizing ecological stability and the environment has received considerable attention. The soil is a complex ecosystem that provides multiple agricultural and environmental services, as well as supplying food for most terrestrial life (2). Intensive land use practices have complex effects on the physical, chemical, and biological properties of the soil, which has resulted in a reduction in soil quality and sustainable agricultural yield (3, 4). Due to its interaction with soil physicochemical and biological features, soil quality degradation has a negative impact on the sustainability of yields (5). For agricultural production to be self sustaining, strategies that are efficient at sustaining soil quality are receiving more attention (6, 7).
Soil quality is defined as ‘the continued capacity of soil to function as a living system, within the ecosystem and land use boundaries, to sustain biological productivity, maintain the quality of air and water environment and promote plant, animal and human health’ (8). A soil quality indicator can be categorized as physical, chemical and biological attributes, and the interaction between these components makes up a complex functional state, making it necessary to define how the function of soil about each attribute (9). However, dynamic soil characteristics such as soil organic matter (SOM), aggregation, and the majority of microbiological traits are more sensitive to management techniques, disturbances, and/or changes in land use (10–13). Due to their high sensitivity, microbial and biochemical properties can serve as systematic potential indicators of soil quality for its assessment after ecological stress and perturbation (11, 14, 15). It is important to identify and determine soil quality indicators that are sensitive to changes and disturbances in the soil environment (14). Individual soil indicators cannot be used to determine soil quality because of their interdependence, which makes it difficult to assess soil conditions in a comprehensive manner (16, 17). Consequently, a combination of soil characteristics into a single overall index can make the evaluation more relevant and practical (18). Since the land capability classification system in 1961 (19), issued by United States Department of Agriculture, Soil Conservation Service, a numerous approaches have been developed to evaluate soil quality but the complexity and heterogeneity of soil systems represent a challenge to creating a universal model or method to determine soil quality (20). Among a variety of assessment methods, the approach of soil quality index has been most widely used, because of its quantitative flexibility and suitability for different types of soil (21, 22), and its integration of soil physical, chemical and biological properties. Soil quality indices have been used to assess the effect of agricultural practices (23) and crop production (24) on the soil as well as the impacts of soil management at a regional scale (25). Therefore, to achieve sustainable agricultural production, soil quality index (SQI) and soil productivity provides the key information (26). As soil parameters typically take a long time to manifest, long-term experiments are appropriate to investigate the relationship between soil characteristics and crop yield in order to develop management strategies for improved crop productivity and soil health (27–29).
Rice-based cropping systems are the backbone of India’s food security, covering a total area of 38 Mha (30). Throughout the Indo-Gangetic plains (IGP), rice-based cropping pattern is most prevalent among farmers due to region’s abundant water supply and fertile land but intensive cultivation, puddling induced soil structure deterioration, excessive use of agrochemicals and injudicious ground water depletion without any regard to environmental protection compromised crop yield throughout IGP region since post green revolution period at cost of soil health (31). Lentil (Lens esculenta) is also adapted to local harsh climate and low fertility soil conditions. Because of its adaptation to intercropping and relay cropping, lentil occupies a unique place in cropping systems in northern, eastern, and central India (32). However, limited information are available on long-term impact of chemical fertilization and integrated application of chemical and organic amendments on SQI in rice-lentil cropping systems in the Indo-Gangetic plains, particularly in the middle Indo-Gangetic plains of Varanasi, UP. The study hypothesized that the following soil parameters (physical, chemical and biological) under the influence of inorganic-organic fertilization combined with an organic amendment, such as farmyard manure (FYM), will be significant in enhancing productivity and soil quality in the study region. Thus, in the present study, we studied three aspects of soil quality: (i) To quantify the impact of long-term organic and inorganic amendments addition on soil quality and crop productivity, (ii) To screen physical, chemical and biological indicators of soil quality in a 34 years old long-term rice– lentil cropping system and (iii) to establish soil quality index based on these indicators. The novelty of the present investigation lies in the identification of the best management practices for maintaining soil quality and sustaining crop productivity under rainfed rice based system in Indo-Gangetic Plain.
2 Material and methods
2.1 Description of experimental site and treatment details
The long-term fertilizer experiment (LTFE) with a rice-lentil cropping system on an Inceptisol was initiated in the year of 1985 at Agriculture Research Farm of Banaras Hindu University, Varanasi (25°18′0′′ North latitude, 83°3′0′′ East longitude and at an altitude of 128.93 meter above the mean sea level). The soil of the study site belongs to the order of Inceptisol which is part of the middle Indo-Gangetic plains of India. The climate of the area is characterized by a dry tropical climate with strong variations in seasonal temperature and precipitation. The experimental site receives an average annual rainfall of approximately 1100 mm (80% of which is received by southwest monsoon between June to September), potential evapotranspiration of 1500 mm, and the mean annual minimum and maximum temperature of 8.9°C and 37.9°C, respectively. The mean relative humidity is about 68% which rises to 86% during the wet season and goes down to 33% during the dry season.
The initial (1985) physical and chemical properties of the surface soil (0-15cm) were: the soil pH of 6.7, total SOC content of 1.4 g kg-1 of soil, low available N (160 kg ha-1), medium available P (21.20kg ha-1) and available K (119 kg ha-1), respectively. The soil of the experimental site is sandy clay loam with a textural composition consisting of 582 g kg-1 of sand, 140 g kg-1 of silt and 278 g kg-1 of clay. The soil is classified as fine-silty, mixed, hyperthermic Udic Ustochrepts.
The rice (NDR- 97) and lentil (HUL-57) crop sequences were followed every year for 34 years (1985–2019). Rice was grown in the Kharif season (July 1st week every year) followed by lentil (HUL-57) in the rabi season (last week of October-November 1st week). The long-term field experiment was conducted using Randomized Complete Block Design (RCBD) with the following treatments: i) T1: control (no external fertilizer application), ii) T2: 50%NPK (50%RDF (@ 40-20-15 kg/ha N:P:K), iii) T3: 100%NPK (100%RDF (@ 80-40-30 kg/ha N:P:K), iv) T4: 50%NPK + FYM (@ 40-20-15 kg/ha N:P:K + 40kg/ha N through FYM), v) T5: 50%FYM (@40kg/ha N through FYM) and vi) T6: 100%FYM (@80 kg/ha N through FYM). The fertilizer NPK was applied in the form of urea, diammonium phosphate (DAP) and muriate of potash (MOP). The whole field was divided into three blocks each representing a replication (net plot size 10m x 9m).
2.2 Collection and analysis of soil samples
The representative field moist soil samples were collected from each of the plots in each replication at 0-15cm depth after harvesting of wet season rice in October 2019. Samples for biological assays were stored in the refrigerator at 40C and samples for chemical assays were dried at room temperature then ground and passed through a 2mm sieve and stored in a plastic container. The physical component of soil quality was assessed by determining soil bulk density (BD) by the core sampler method (33), mean weight diameter (MWD) (34) and soil texture was determined by the Bouyoucos hydrometer method (35). Chemical parameters of soil were assessed by determining soil pH (36), soil organic carbon (SOC) (37), total soil organic carbon (TOC) (38), alkaline potassium permanganate oxidizable soil nitrogen (available N) (39), Olsen phosphorus (available P) (40), NH4OAc extractable potassium (available K) (41), CaCl2 extractable sulfur (available S) (42), CaCl2 extractable boron (available B) (43) and diethylene triamine penta acetic acid (DTPA)-extractable micro-nutrients (available Zn, Cu, Fe and Mn) (44). The biological parameters of soil quality were determined by measuring microbial biomass C (MBC) (45), mineralizable C (Cmin) (46), mineralizable N (Nmin) (47) and enzyme activities viz., dehydrogenase (DHA) (48), acid (ACP) and alkaline phosphatase (ALKP) (49), arylsulphatase (ASP) (50), urease (URE), β-glucosidase (BGL) and fluorescein diacetate hydrolase (FDA) activity (48) of the soils.
2.3 Development of soil quality index
Soil quality index (SQI) was determined by using the steps as described by 17. Minimum data set (MDS) was prepared from the best representing variables, followed by scoring of selected indicators and finally summarizing the weighed and scored indicators into soil quality index. Most representing indicators were carefully chosen from principal component analysis (PCA) based on the eigenvalue >1 as described by (51). Highly weighed factor loadings in each PC for were retained for further analysis. Scoring of selected indicators was performed using linear function where, ‘more is better’, ‘less is better’ or ‘optimum is better’ approach was followed (12, 52, 53).
Weighing factors for each PC were obtained from PCA. Finally, the weighed variable scores were summarized to develop soil quality index (SQI) (53, 54).
where, Wi is the weighting factor and Si is the score of each selected indicator
2.4 Yield and equivalent rice yield
Average yield data of rice and lentil under different treatments was taken and equivalent rice yield (ERY) was calculated for each of the treatments for expressing the yield in a common unit following the formula given below
Results
3.1 Effect of long-term fertilization and manuring on soil physical and chemical properties
The result showed that BD ranged from 1.36 to 1.46 Mg m-3 in 0-15cm soil depth. In surface soil, the highest BD was observed in the T1 (1.46 Mg m-3) treatment and the lowest in the T6 (1.36 Mg m-3) treatment (Table 1). Continuous combined application of NPK+FYM significantly enhanced the MWD as compared with the control. In 0-15cm depth, MWD was varied between 0.61-0.89, where the highest value was found in the treatment T6, and the lowest value was found in the control (T1) treatment. The soil pH (Table 1) value ranged from 6.1 to 7.3 with the maximum value observed under T3 treatment. It was observed that SOC varied between 3.27-5.04 g kg-1 in the surface layer. Results showed that 54.1% higher SOC content was found in 50%NPK+FYM treatment than in control at 0-15 cm depth (Table 1). Total organic carbon (TOC) in different treatment was in the range of 4.10-6.82 g kg-1 in the surface layer.
3.2 Effect of long-term fertilization and manuring on soil macro-nutrients
The available N content under different treatments was in the range of 117 to 184 kg ha-1 (Table 2). The available N was significantly higher (184 kg ha-1) in T2 (100%NPK) treatment over control (117 kg ha-1). The available P content in the surface layer varied from 10.2 under control to 41.8 kg ha-1 in the T6 treatment. The available P varied significantly among all treatments (Table 2). The available K content under different treatments was in the range of 114-82 kg ha-1 (Table 2). Results showed that 39% higher available K content was found in 50%NPK+FYM treatment than in control at the surface layer. It was found that available sulphur content varied between 6.11-17.8 mg kg-1 (Table 2). Continuous combined application of organic and inorganic fertilizer significantly increases the available S content as compared to either inorganic fertilizer or control.
3.3 Effect of long-term fertilization and manuring on soil micro-nutrients
It was found that available Zn content was significantly higher in 50%NPK + FYM (1.36 ppm) and 100%FYM (0.95 ppm) treatments as compared to others in 0-15cm depth (Table 3). The extent of available Zn varied between 0.63 ppm under the treatment of 50% NPK (T3) to 1.36 ppm under the treatment of 50% NPK+FYM (T6). It was observed that available Cu ranged between 1.67 ppm under control (T1) to 2.39 ppm in T6. The sample showed significant variation in available Cu content as compared to the control. The available Fe content under different treatments was in the range of 58.5 ppm under the treatment control (T1) to 118 ppm under the treatment of 50% NPK+FYM (Table 3). There was no significant difference in available Fe content in the surface layer. Available Mn in the surface layer varied from 23.8 ppm under T4 to 34.0 ppm in T6. There was no significant treatment effect on available Mn content. The available B content significantly higher (1.66 mg kg-1) was in treatment T6 (50%NPK + FYM) over control (1.40 mg kg-1) at 0-15cm depth (Table 3).
3.4 Effect of long-term fertilization and manuring on biological properties of soil
Results showed that continuous cropping of rice-lentil with 50%NPK+FYM and 100% NPK input significantly increase the acid phosphatase activity over control in surface soil (Table 4). Acid phosphatase activity varied from being lowest (126 µg p-nitrophenol g-1 h-1) in the control treatment to highest (369 µg p-nitrophenol g-1 h-1) in 50%NPK+FYM treatment. Alkaline phosphatase activity was varied in the range of 72.9-108 µg p-nitrophenol g-1 h-1 at surface soil. Results showed that continuous application of organic and inorganic fertilizer for 34 years significantly improved 48.1%, 17.7%, 16.3%, 6.03% and 4.8% of alkaline phosphatase activity over control in 50%NPK+FYM, 50%FYM, 100%FYM, 100%NPK and 50%NPK treatment, respectively at surface layer (Table 4). Arylsulphatase activity under different treatments was in the range of 15.6-30.08 µg p-nitrophenol g-1 h-1. It was observed that 92.8% higher arylsuphatase activity was found in 50%NPK+FYM treatment than control (Table 4). β-glucosidase was varied in the range of 16.6-30.5 µg p-nitrophenol g-1 h-1 at surface soil (Table 4). Balanced fertilization with organic and inorganics contributed about 83.7% improvement in β-glucosidase over control. Fluorescein diacetate hydrolase activity varied from being lowest (7.66 µg fluorescein g-1 h-1) in 50%FYM treatment to highest (14.6 µg fluorescein g-1 h-1) in 100%FYM treatment (Table 4). Significantly higher dehydrogenase activity was found in sites supplemented with 50%NPK+FYM (54.89 µg TPF g-1 h-1) treatment over control (31.78 µg TPF g-1 h-1). The descending trend of DHA was as follows – 50%NPK+FYM > 100%FYM > 50%FYM > 100%NPK > 50%NPK > control (Table 4). Urease was varied in the range of 29.1-61.7 µg NH4-N g-1 2 h-1 at surface soil (Table 4). Plot with nutrient supplementation (50%NPK+FYM) exhibited the highest (61.7 µg NH4-N g-1 2 h-1) enzymatic activity compared to the control plot (29.1 µg NH4-N g-1 2 h-1). Results revealed that all treatments recorded significantly higher MBC than the control (Figure 1). The highest magnitude of increase was found in treatment 100%FYM (83.9%) followed by 50%NPK+FYM (82.4%), 50%FYM (62.3%), 100%NPK (44.7%) and 50%NPK (35.2%) over control. Mineralizable C (Cmin) content under different treatments varied from 223-339 µg g-1 64 d-1 and was in the following order: 50%NPK+FYM> 100%FYM> 100%NPK> 50%FYM > 50%NPK> control (Figure 2). Mineralizable N (Nmin) varied from being lowest (2.13µg NH4-N g-1 d-1) in control treatment to highest (2.85 µg NH4-N g-1 d-1) in 50% NPK+ FYM treatment (Figure 2).
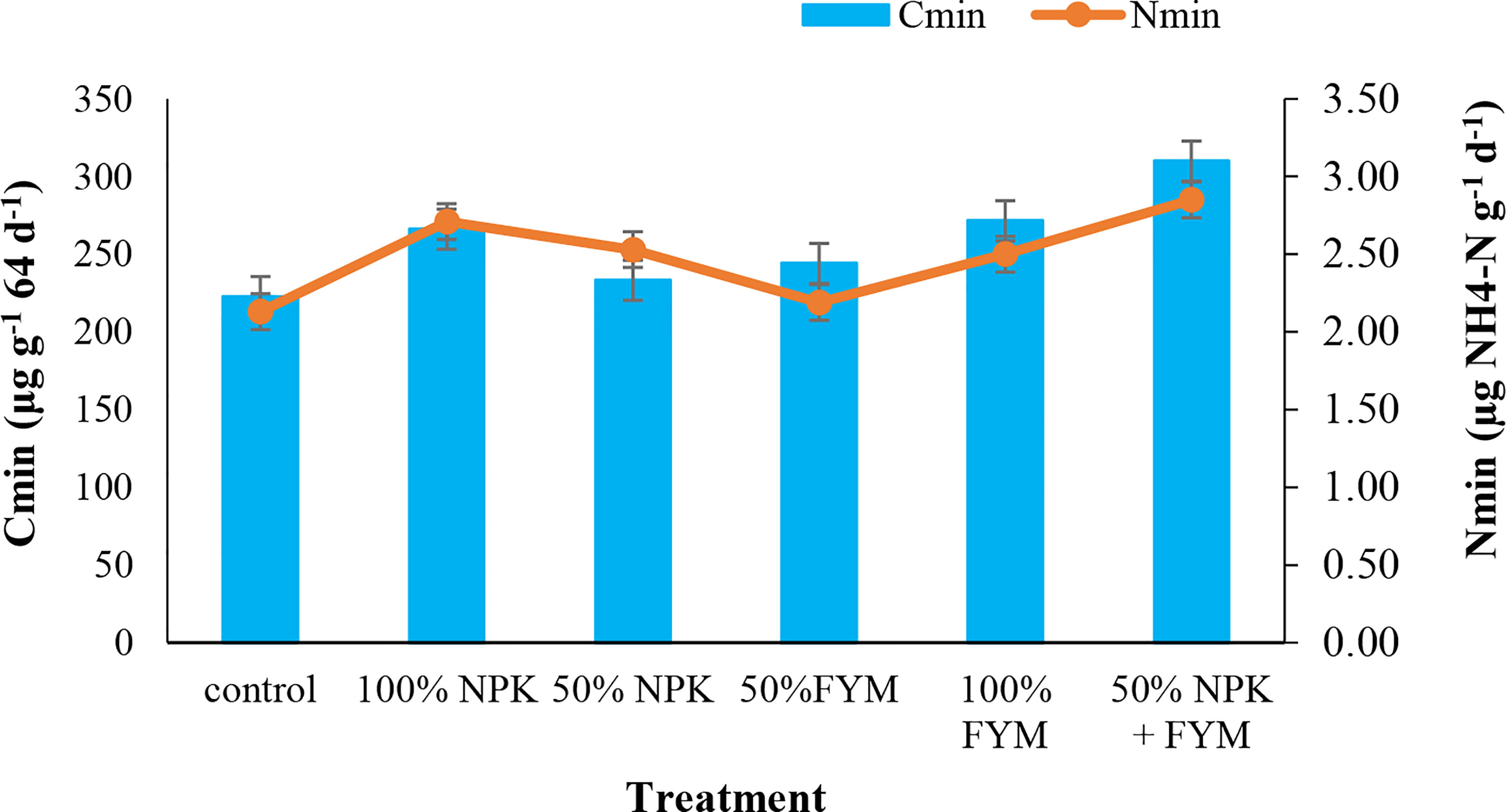
Figure 2 Effect of long-term fertilization and manuring on soil mineralizable carbon and mineralizable nitrogen.
3.5 Formulation of minimum dataset and development of soil quality index and validation
The analytical values of soil quality parameters were run through principal component analysis (PCA) to formulate minimum data set (MDS) (Table 5). Seven principle components (PC 1 to PC 7) were selected for further analysis. Highly weighed factor loading(s) from each PC were selected for formulation of MDS namely MWD, available Fe, available N, potentially mineralizable N, available Zn, FDA hydrolase activity and Clay. Although, PC1 and PC2 exhibited few absolute values greater than 10% of the highest factor loading, they were not considered for MDS as those parameters were correlated significantly (54).
Selected variables were scored using linear scoring function. Since, higher values of all the selected parameters are considered as good in relations to soil function they were grouped as ‘more is better’ function. The weighed scores were integrated into soil quality index (SQI). The calculated value of SQI was ranged from 0.68 to 0.95 among the treatments. The highest SQI was observed under 50% NPK+FYM treatment whereas; the lowest SQI was contributed by control (Figure 3). The developed SQI under different management practices was validated with equivalent rice yield (ERY) as productivity was management goal of the present study (Figure 4). To validate the SQI regression analysis was done, and it was found that there was good agreement between SQI and ERY (R2 = 0.87).
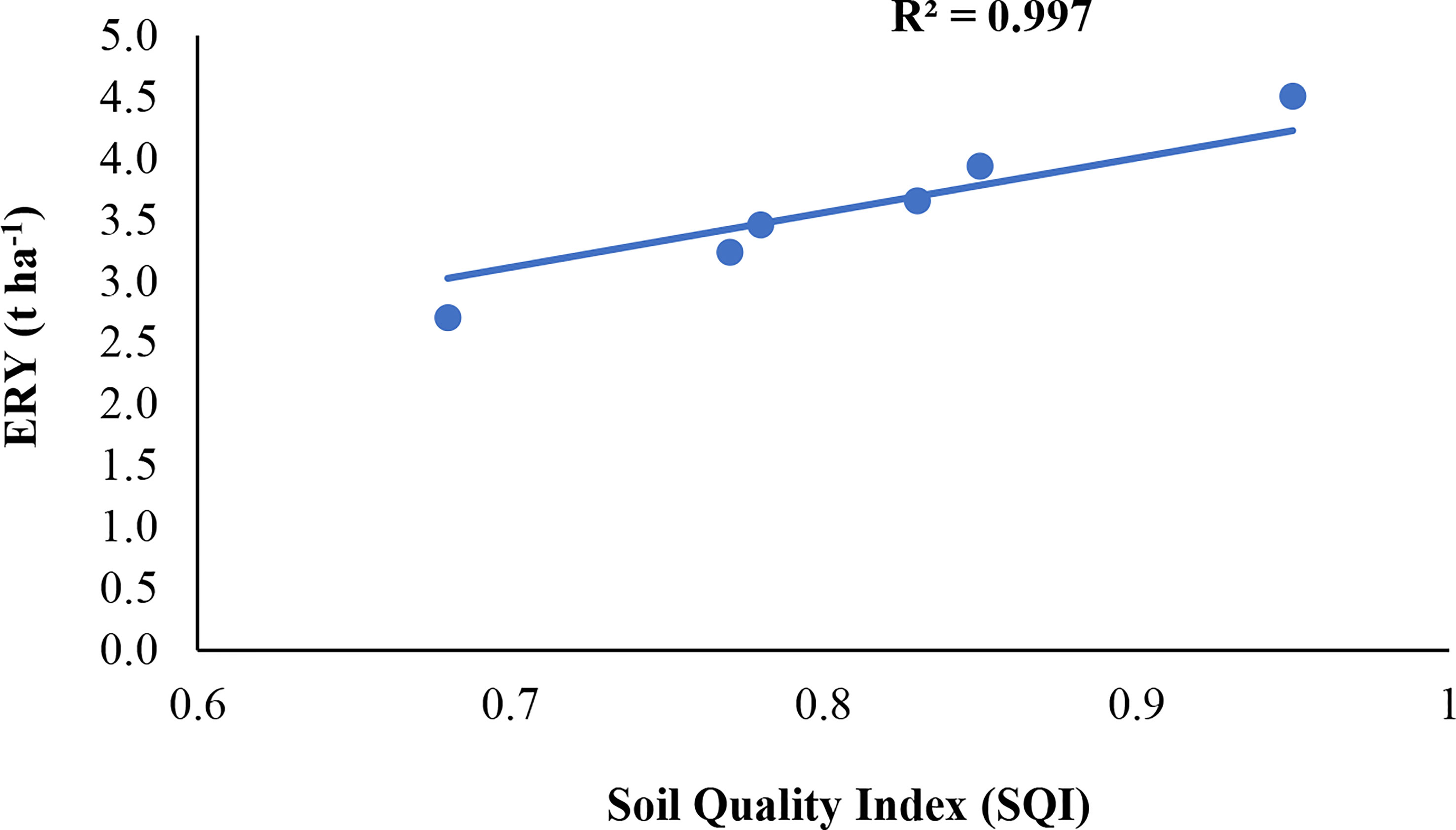
Figure 4 Relationship between soil quality index and equivalent rice yield as obtained through simple regression model.
4 Discussion
4.1 Effect of long-term fertilization and manuring on physical and chemical soil properties
Physical and chemical properties varied with different agronomic practices like tillage, irrigation, manuring and fertilization. In our study, it was found that the inclusion of FYM either alone or integrated use of with NPK lowers the bulk density of soil in comparison to control or NPK treatment. The significant decrease in the bulk density resulting from higher soil organic carbon content due to the addition of root and plant biomass, improve soil aggregation and increased root growth and bio pores in the NPK+FYM treated plot (55). The decomposition of organic residues in soil encourages microbial activity which produces organic acid and polysaccharides that act as a binding agent to change micropores into macropores, resulting in a lower BD of the soil (56). Aggregate stability of soil i.e., MWD in our study found to be higher under NPK+FYM treated plots which might be due to the higher organic carbon content of these soil. The incorporation of organic matter causes an increase in soil aggregates because organic substances that are added to the soil through FYM can bind the soil particles together (57). It was reported that the improvement in soil structure is likely to be associated with better root growth and microbial activity when organic matter is added to the soil (58). Combined application of organic and inorganic fertilizers results in slightly lower soil pH due to the presence of organic acids formed after decomposition (59). Hu et al. (60) reported that integrated inorganic and organic manure use increased the microbial activity that promotes the degradation of organic matter by producing organic acids which slightly lower the soil pH. The cation exchange capacity of these organic acids may increase with manure application and accelerate the pH decrease due to the high proton release (61). Long-term manuring and fertilization significantly increased the SOC concentration in surface soil. Soil organic C was higher in the plot receiving NPK + FYM fertilizer as compared to the plot receiving NPK fertilizer alone or unfertilized plot because this can be attributed to the direct addition of organic carbon through FYM and the addition of crop residues and root biomass (62–64). The continuous addition of root biomass, root exudates and plant residues, resulted in more organic residues in the soil, which after decomposition might have increased the concentration of SOC. Liu et al. (65) also found a similar effect.
4.2 Effect of long-term fertilization and manuring on soil macro-nutrients
Different fertilization treatments significantly influenced the availability of N, P, and K in soil. In this study, it was observed that long-term use of chemical fertilizer significantly increases the available N content of the soil in comparison to the control treatment. Qin et al. (66)reported that in sites receiving recommended doses of fertilizer, good growth in root and plant biomass could have a positive influence on the nitrogen content of the soil. In this study, it was observed that the application of either balanced chemical fertilizer alone or in combination with farmyard manure led to a significantly higher content of soil available P over the control treatment. It might be because, during decomposition, organic acids are released, which then contribute to releasing phosphorus from the native phosphorus pool in the soil (67). Long-term use of NPK either alone or in combination with FYM increases the available K content in the soil. The positive effect of organic manure on available K has been reported by many researchers (58, 68). Urkurkar et al. (69) reported that a significant increase in available K with the application of FYM is likely due to the reduction of K fixation and release of K due to the interaction of organic matter with clay, as well as the direct addition of K to the soil. Sulphur is an essential nutrient for plant growth, and it is considered a secondary nutrient because plants require them in smaller quantities than nitrogen, phosphorus and potassium. Sulphur can be found in soil in organic and inorganic forms. A variety of organic sources, such as farmyard manure, green manure and plant residues, can supply enough sulphur to plants (70). Sharma et al. (71) concluded that the application of inorganic and organic fertilizers together increases soil sulphur content as the decomposition and recycling of organic manures enhance sulphur content in the soil.
4.3 Effect of long-term fertilization and manuring on soil micro-nutrients
DTPA- extractable micronutrient concentration in soil may be affected by soil properties such as pH and their chemical nature (72, 73). Continuous cropping for 34 years increased DTPA extractable Zn, Cu, Fe, and Mn content of the soil in plots that received either FYM along with NPK or FYM alone over plots that received 50% NPK and control plots. The increase in soil micronutrient levels after FYM application can be attributed to several reasons, including mineralization of organically bound forms (74), dissolution of relatively unavailable forms (75), redox-potential reduction (76), and chelating action of organic compounds released during the decomposition of organic matter (77). Katyal & Sharma (78) reported that significant correlation between DTPA- extractable micronutrients and the organic matter content of the soil. There might be an explanation for the increase in DTPA extractable micronutrients in the soil based on the presence of protons/H+ in organic manure and decreasing soil pH (as well as Eh), thereby enhancing the dissolution and reduction of micronutrients, and therefore increasing their availability. These results are consistent with the findings of Keshavarzi et al. (79) and Zhang et al. (80). According to Setia and Sharma (81), chemical fertilizers decreased soil DTPA- extractable micronutrients. According to Singh et al. (82), the integration of inorganic and organic manure significantly promotes microbial decomposition of soil organic matter released complexing agents such as organic acid and humic acid, which allowed the movement of micronutrients from the less soluble forms to more plant-available forms. According to Das et al. (83), FYM contributed to a positive effect on soil B availability by mobilizing B from soil minerals and chelating it with breakdown products of soil organic matter. The authors reported that higher decomposition rates of organic matter increase the production of various organic acids including tartaric, citric, and humic acids, all of which can solubilize relatively unavailable B and make it available for plants (84).
4.4 Effect of long-term fertilization and manuring on biological properties of soil
The phosphatases are a group of enzymes that hydrolyze esters and anhydrides of phosphoric acid. Phosphomonoesterases also include acid and alkaline phosphatases, phosphoprotein phosphatases, phytases and nucleotidases. These are typically adaptive enzymes indicating their activity increases when plant available P content decreases. Orthophosphoric monoester phosphohydrolases (acid and alkaline phosphatases) particularly catalyze the hydrolysis of P-ester bonds binding P to C (C-O-P ester bonds) in organic matter (85). Phosphatase enzymes are important because they release PO4 from immobile organic P to improve soil phosphorus availability to plants (86). Based on our result of the present study, acid phosphatase activity in soil was higher than alkaline phosphatase activity. It is possibly due to the extensive root system of rice producing higher levels of acid phosphatase enzymes through exudation in rhizospheric soil as well as the long-term fertilization with phosphorus may have impeded the activity of alkaline phosphatase enzymes in soil (87). When farmyard manure was applied in combination with inorganic manure, significantly greater phosphatase activity has detected the reason for this may be that manure contains a higher level of microbial activity and greater diversity of phosphate-solubilizing bacteria (88).
The arylsulfatase enzyme in soil plays an essential role in releasing so that it can be used by plants. A balanced fertilization with and without FYM applications leads to significantly higher levels of arylsulfatase in soil than an imbalanced fertilizer application. According to our results, the highest ASP was found under NPK + FYM treatment, and the lowest was measured under control. It is mostly due to the high correlation between soil organic matter and arylsulphatase activity in FYM-treated plots (89). As discussed by Knauff et al. (90), arylsulfatase enzyme activity is greatly affected by soil organic C content and is further enhanced by the long-term addition of organic manure.
β-glucosidase is an important enzyme involved in the carbon cycle in the soil and it facilitates the breakdown of cellulose and the release of glucose utilized by microorganisms for growth (91, 92). According to our results, the highest β-glucosidase activity was found under the NPK+ FYM plot, and the lowest was observed under the control plot. There might be a reason for this because FYM supplies a large amount of easily decomposable carbon e.g., carbohydrates (93).
The fluorescein diacetate (FDA) method is widely accepted as a reliable and simple method for determining the abundance of active fungi and bacteria (94). The hydrolysis of fluorescein di acetate is mediated by several enzymes in soil, including protease, esterase, and lipase (95). The increased values of fluorescein diacetate activity were observed in the organically amended plot due to a strong correlation between FDA activity and soil organic C and nutrients (96). This corroborated with the finding of Nayak et al. (97), who reported that organic matter content has significant correlations with FDA under continuous rice-growing soil.
Continuous manuring and balanced fertilization for 34 years resulted in significantly higher activities of all major enzymes than the control. The study found that DHA was an effective indicator for maintaining soil microbial activity as well as maintaining soil health. DHA activity has been widely used to measure microbial activity in soils since it represents overall metabolic status. Bhattacharyya et al. (98), reported that increase in the DHA activity in soil due to the combined application of FYM with NPK. Hence, FYM treatment resulted in a higher level of biological activity and stabilization of extracellular enzymes via complexation with humic substances (99). An imbalanced and inadequate fertilizer application led to a significant decline in DHA activity.
In soil, the urease enzyme hydrolyzes urea into carbon dioxide and ammonia, which plays a crucial role in N cycling. In our study, the highest mean value for urease activity was found in NPK+FYM treatment, it might be the result of continuous farming under organic manure fertilization, which stimulates heterotrophic microbes, which decompose organic manure and provide carbon and energy to heterotrophic microbes (65, 100, 101). Similarly, Chhonkar and Tarafdar (102) reported that soil enzyme activities were significantly related to organic carbon and microbial populations in the soil. Dick et al. (103) and Bandick and Dick (104), have reported significant reductions in urease activity in the soil after the addition of inorganic nitrogen. This is mostly due to the build-up of , which suppresses urease activity.
Microbial biomass carbon is commonly used to evaluate the effect of different farming practices on soil fertility. It was found that the microbial biomass carbon was significantly higher in plots that received organic manure along with NPK fertilizer than in plots that received only inorganic fertilizer. This may be due to the catalytic effect of FYM which stimulates microbial growth, thus increasing microbial biomass C (89). Patil and Puranik (105) found similar positive effects of using organic manures combined with chemical fertilizers together. By applying FYM, nutrients and carbon were supplied in a balanced manner, which, in turn, supported the growth of microorganisms in the soil (106).
The results of a long-term experiment showed that amendments of organic manure with chemical fertilizer enhances microbial growth and activity and provides substrates for mineralization (107). The amount of Cmin vary from treatment to treatment, because of variations in soil labile organic carbon (108). A labile pool of soil organic carbon contributes significantly to nutrient cycling in soil by accelerating C mineralization (109).
The most commonly limited nutrient in agriculture is nitrogen. Plants take up nitrogen from a various mechanism, including fertilisation, biological N fixation, and mineralization of N from soil organic matter (110). In the present study we found significantly higher mineralizable N in NPK+FYM treated plot, which could be due to the addition of nitrogen through manuring and the increased microbial activity under abundant carbon resources (111).
4.5 Soil quality indicators, indices and validation
Highly weighed factor loading(s) from each PC were selected for formulation of MDS namely mean weight diameter (MWD), available Fe, available N, potentially mineralizable N, available Zn, FDA hydrolase activity and clay. Presence of MWD in MDS is justified because destruction of soil structure in puddled transplanted rice is of great concern for sustenance of soil productivity. Iron is vital for seed development, starch and chlorophyll synthesis and in the present study available Fe contributed to soil quality index. Masto et al. (112) proposed DTPA-Fe as component of minimum dataset under an assessment of soil health across different land uses including rice-wheat system. DTPA-Fe also emerged as sensitive indicators of rice productivity at Inceptisol of upper IGP (113). Permanganate oxidizable nitrogen was not found to be in sufficient amount in soil thus it can be a potential yield limiting factor. Available N was selected as key indicator of soil quality under rice based system in Indo-Gangetic Plain (114). Potentially mineralizable N is proposed as indicators of soil quality because it forecasts plant availability of nitrogen, thus must be included in soil health assessment framework (115). Since rice is one of the crops that are most susceptible to zinc deficiency, Zn is the micronutrient that has the most impact on the growth and output of rice (116). Av-Zn was chosen because it was crucial for preserving soil quality, especially in low-land rice-based agricultural systems (117). The availability of Zn is a sensitive indicator of soil quality in Inceptisols of semi-arid subtropics, and DTPA-Zn has also emerged as a sensitive predictor of rice production (21). Fluorescein diacetate has been used to determine abundance of active fungi and bacteria. Several enzymes in soil such as protease, esterase and lipase take part in hydrolysis of fluorescein di acetate (118). Enhanced activity of FDA under integrated treatment is attributed to greater microbial biomass carbon and higher carbon resources in rhizospheric soils (119). Higher values of fluorescein diacetate activity in organic amended plot due to strong correlation between FDA activity and bioavailability of soil organic C and nutrients (96). Nayak et al. (97) also showed that organic matter content has significant correlations with FDA under continuous rice growing soil. Clay was selected as key indicators of soil quality in semi arid Decan Plateau where both inherent and dynamic properties of soil were taken into account in developing soil quality index (120).
The highest SQI of 0.95 was found in 50% NPK+FYM treatment and the lowest value of 0.68 was obtained from control. The relative contribution of MWD was highest to the SQI in all the treatments, indicating MWD as the most influential among the variables. MWD was significantly correlated with SOC, similar finding was also observed by Rahaman et al. (121). Higher weightage of MWD attributed by the higher SOC content (54.1% higher than control) in 50% NPK+FYM treatment exhibited the highest SQI; in contrast, decline in the weighted value of key indicators contributed to poor SQI in the control. The lowest contributor to SQI was FDA, followed by clay content. The weighted additive SQI gives more pertinent and accurate information concerning the actual status of the soil by representing the proportional contributions of several variables to SQI (122). Application of organic matter via farmyard manure and crop wastes significantly affects the soil’s physical, chemical, and biological functional capability (123). As a result, farming systems, which include integrated treatment, are a fundamental strategy for enhancing soil quality. It also serves to keep soil quality at a desirable level (124). Therefore, balanced fertilization and manuring, which optimises the key indicators can be adopted to improve soil quality undere rainfed rice-lentil system of Indo-Gangetic Plain. Results of regression analysis confirmed better yield forecasting ability of PCA based SQI (R2 = 0.87) which might be due to inclusion of more responsive and meaningful dataset of the cropping system. Such type of information is very much crucial to assess performance of present system or scope of advancing alternate ones in a particular region. Performance of weighted additive index of soil quality in relation to crop yield was reported to be best in several scientific study worldwide (125). Vasu et al. (120) observed significantly higher correlation (0.34) o weighted SQI with crop yield at semi-arid Deccan Plateau of India.
5 Conclusion
The application of FYM along with NPK fertilizer for 34 years long in rainfed rice-lentil cropping system improved the soil quality and sustain the crop productivity. The recommended doses of fertilizers along with organic manure play a vital role in improving physical, chemical, and biological properties of the soil and thereby enhancing soil quality and ensuring sustainable yield for a long period of time. Mean weight diameter (MWD), available Fe, available N, potentially mineralizable N, available Zn, FDA hydrolase activity and clay were chosen as the key indicators of soil quality out of the 25 parameters examined. Despite the possibility that these indicators could serve as a management-induced early warning system for changes in soil quality, extensive validation is required before they can be successfully used for practical purposes. The application of integrated use of chemical fertilizer and organic sources stood out among the practices as the best management method for improving soil quality of the system. Thus, this practice may be recommended for assessing management-induced changes in soil quality under rainfed rice based system in subtropical areas of many south and south-east Asian countries.
Data availability statement
The original contributions presented in the study are included in the article/supplementary material. Further inquiries can be directed to the corresponding author.
Author contributions
SB: conceptualization, investigation, data analysis, supervision, project administration, funding, and manuscript writing and editing. PS: analysis of soil samples, data recording, and manuscript preparation, RR: investigation, data analysis, and manuscript preparation, KP: investigation, and manuscript preparation. ND: experimentation and manuscript editing. All authors contributed to the article and approved the submitted version.
Funding
Authors are grateful to the Science & Engineering Research Board (SERB)-Department of Science & Technology, Government of India for funding through the project entitled ‘Identification of key indicators and establishment of their critical limits in assessing soil health under different agro-ecological regions of India’ under Early Career Research Award Scheme (ECR/2017/002908) and ICAR-IARI, New Delhi for providing necessary facilities for the study.
Conflict of interest
The authors declare that the research was conducted in the absence of any commercial or financial relationships that could be construed as a potential conflict of interest.
Publisher’s note
All claims expressed in this article are solely those of the authors and do not necessarily represent those of their affiliated organizations, or those of the publisher, the editors and the reviewers. Any product that may be evaluated in this article, or claim that may be made by its manufacturer, is not guaranteed or endorsed by the publisher.
References
1. Richardson RB. Ecosystem services and food security: Economic perspectives on environmental sustainability. Sustainability (2010) 2(11):3520–48. doi: 10.3390/su21135201
2. Karlen DL, Mausbach MJ, Doran JW, Cline RG, Harris RF, Schuman GE. Soil quality: a concept, definition, and framework for evaluation (a guest editorial). Soil Sci Soc Am J (1997) 61:4–10. doi: 10.2136/sssaj1997.03615995006100010001x
3. Karchegani PM, Ayoubi S, Mosaddeghi MR, Honarjoo N. Soil organic carbon pools in particle-size fractions as affected by slope gradient and land use change in hilly regions, western Iran. J Mt Sci (2012) . 9:87–95. doi: 10.1007/s11629-012-2211-2
4. Havaee S, Ayoubi S, Mosaddeghi MR, Keller T. Impacts of land use on soil organic matter and degree of compactness in calcareous soils of central Iran. Soil Use Manag (2014) . 30(1):2–9. doi: 10.1111/sum.12092
5. Kumar N, Nath CP, Hazra KK, Das K, Venkatesh MS, Singh MK, et al. Impact of zero-till residue management and crop diversification with legumes on soil aggregation and carbon sequestration. Soil Till Res (2019) 189:158–67. doi: 10.1016/j.still.2019.02.001
6. Eivazi F, Bayan MR, Schmidt K. Select soil enzyme activities in the historic sanborn field as affected by long-term cropping systems. Commun Soil Sci Plant Anal (2003) 34:2259–75. doi: 10.1081/CSS-120024062
7. Nehrani SH, Askari MS, Saadat S, Delavar MA, Taheri M, Holden NM. Quantification of soil quality under semi-arid agriculture in the northwest of Iran. Ecol Indic (2020) 108:105770. doi: 10.1016/j.ecolind.2019.105770
8. Azizsoltani E, Honarjoo N, Ayoubi S. How soil pore distribution could help in soil quality studies as an appropriate indicator. Eurasian Soil Sci (2019) . 52:654–60. doi: 10.1134/S1064229319060036
9. Cavalcante DM, Silva APF, de Almeida BG, Freire FJ, dos Santos Silva TH, Cavalcante FMS. Physical soil quality indicators for environmental assessment and agricultural potential of oxisols under different land uses in the araripe plateau, Brazil. Soil Till Res (2021) . 209:104951. doi: 10.1016/j.still.2021.104951
10. Carter MR. Soil quality for sustainable land management: organic matter and aggregation interactions that maintain soil functions. Agron J (2002) 94:38–47. doi: 10.2134/agronj2002.3800
11. Bastida F, Moreno JL, Hernández T, García C. Microbiological degradation index of soils in a semiarid climate. Soil Biol Biochem (2006) 38:3463–73. doi: 10.1016/j.soilbio.2006.06.001
12. Masto RE, Chhonkar PK, Purakayastha TJ, Patra AK, Singh D. Soil quality indices for evaluation of long-term land use and soil management practices in semi-arid sub-tropical India. Land Degrad Dev (2008) 19:516–29. doi: 10.1002/ldr.857
13. Li Y, Dong S, Wen L, Wang X, Wu Y. Assessing the soil quality of alpine grasslands in the qinghai-Tibetan plateau using a modified soil quality index. Environ Monit Assess (2013) 185:8011–22. doi: 10.1007/s10661-013-3151-1
14. Gil-Sotres F, Trasar-Cepeda C, Leirós MC, Seoane S. Different approaches to evaluating soil quality using biochemical properties. Soil Biol Biochem (2005) 37:877–87. doi: 10.1016/j.soilbio.2004.10.003
15. Lopes AAC, Sousa DMG, Chaer GM, Junior FBR, Goedert WJ, Mendes IC. Interpretation of microbial soil indicators as a function of crop yield and organic carbon. Soil Sci Soc Am J (2013) 77:461–72. doi: 10.2136/sssaj2012.0191
16. Guo S, Han X, Li H, Wang T, Tong X, Ren G, et al. Evaluation of soil quality along two revegetation chronosequences on the loess hilly region of China. Sci Total Environ (2018) . 633:808–15. doi: 10.1016/j.scitotenv.2018.03.210
17. Raiesi F, Kabiri V. Identification of soil quality indicators for assessing the effect of different tillage practices through a soil quality index in a semi-arid environment. Ecol Indic (2016) 71:198–207. doi: 10.1016/j.ecolind.2016.06.061
18. Armenise E, Redmile-Gordon MA, Stellacci AM, Ciccarese A, Rubino P. Developing a soil quality index to compare soil fitness for agricultural use under different managements in the mediterranean environment. Soil Till Res (2013) . 130:91–8. doi: 10.1016/j.still.2013.02.013
19. Klingebiel AA, Montgomery PH. Land-capability classification (No. 210). Soil Conserv Service US Department Agric (1961).
20. de Paul Obade V, Lal R. Towards a standard technique for soil quality assessment. Geoderma (2016) 265:96–102. doi: 10.1016/j.geoderma.2015.11.023
21. Biswas S, Hazra G, Purakayastha T, Saha N, Mitran T, Roy SS, et al. Establishment of critical limits of indicators and indices of soil quality in rice-rice cropping systems under different soil orders. Geoderma (2017) 292:34–48. doi: 10.1016/j.geoderma.2017.01.003
22. Qi Y, Darilek JL, Huang B, Zhao Y, Sun W, Gu Z. Evaluating soil quality indices in an agricultural region of jiangsu province. China. Geoderma (2009) . 149:325–34. doi: 10.1016/j.geoderma.2008.12.015
23. Govaerts B, Sayre KD, Deckers J. A minimum data set for soil quality assessment of wheat and maize cropping in the highlands of Mexico. Soil Till Res (2006) 87:163–74. doi: 10.1016/j.still.2005.03.005
24. Juhos K, Szabó S, Ladányi M. Explore the influence of soil quality on crop yield using statistically-derived pedological indicators. Ecol Indic (2016) 63:366–73. doi: 10.1016/j.ecolind.2015.12.029
25. Gong L, Ran Q, He G, Tiyip T. A soil quality assessment under different land use types in keriya river basin, southern xinjiang, China. Soil Till Res (2015) . 146:223–9. doi: 10.1016/j.still.2014.11.001
26. Khaki BD, Honarjoo N, Davatgar N, Jalalian A, Golsefidi HT. Assessment of two soil fertility indexes to evaluate paddy fields for rice cultivation. Sustainability (2017) 9:1299. doi: 10.3390/su9081299
27. Nayak AK, Gangwar B, Shukla AK, Mazumdar PS, Kumar A, Raja R, et al. Long-term effect of different integrated nutrient management on soil organic carbon and its fractions and sustainability of rice– wheat system in indo gangetic plains of India. Field Crops Res (2012) 127:129–39. doi: 10.1016/j.fcr.2011.11.011
28. Shahid M, Shukla AK, Bhattacharyya P, Tripathi R, Mohanty S, Kumar A, et al. Micronutrients (Fe, Mn, zn and Cu) balance under long-term application of fertilizer and manure in a tropical rice– rice system. J Soils Sediments (2016) 16:737–47. doi: 10.1007/s11368-015-1272-6
29. Rakshit R, Das A, Padbhushan R, Sharma RP, Kumar S. Assessment of soil quality and identification of parameters influencing system yield under long-term fertilizer trial. J Indian Soc Soil Sci (2018) 66:166–71. doi: 10.5958/0974-0228.2018.00021.X
31. Das TK, Nath CP, Das S, Biswas S, Bhattacharyya R, Sudhishri S, et al. Conservation Agriculture in rice-mustard cropping system for five years: Impacts on crop productivity, profitability, water-use efficiency, and soil properties. Field Crops Res (2020) 250:107781. doi: 10.1016/j.fcr.2020.107781
32. Srinivasarao C, Venkateswarlu B, Lal R, Singh AK, Kundu S. Sustainable management of soils of dryland ecosystems of India for enhancing agronomic productivity and sequestering carbon. Adv Agron (2013) 121:253–329. doi: 10.1016/B978-0-12-407685-3.00005-0
33. Blake GR, Hartge KH. Particle density. methods of soil analysis: Part 1 physical and mineralogical methods. Am Soc Agron (1986) 5:377–82. doi: 10.2136/sssabookser5.1.2ed.c14
34. Yoder RE. A direct method of aggregate analysis of soils and a study of the physical nature of erosion losses. Am Soc Agron (1936) 28:337–51. doi: 10.2134/agronj1936.00021962002800050001x
35. Gee GW, Bauder JW. Particle-size Analysis.383-411 Methods of soil analysis. Physical and mineralogical methods. Agronomy Monograph 9 (2ed). Am soc agron Klute A (1986). doi: 10.2136/sssabookser5.1.2ed.c26
36. Page AL, Miller RH, Keeney DR. Methods of soil analysis, part 2: Chemical and microbiological properties, Wisconsin. Am Soc Agron Inc Soil Sci Soc Am Inc (1982), 1159.
37. Walkley A, Black IA. An examination of the degtjareff method for determining soil organic matter, and a proposed modification of the chromic acid titration method. Soil Sci (1934) 37:29–38. doi: 10.1097/00010694-193401000-00003
38. Snyder JD, Trofymow JA. A rapid accurate wet oxidation diffusion procedure for determining organic and inorganic carbon in plant and soil samples. Commun Soil Sci Plant Anal (1984) 15:587–97. doi: 10.1080/00103628409367499
39. Subbaiah BV. A rapid procedure for estimation of available nitrogen in soil. Curr Sci (1956) 25:259–60.
40. Olsen SR. Estimation of available phosphorus in soils by extraction with sodium bicarbonate (No. 939). US Department Agric (1954).
41. Hanway JJ, Heidel H. Soil analysis methods as used in Iowa state college soil testing laboratory. Iowa Agric (1952) 57:1–31.
42. Chesnin L, Yien CH. Turbidimetric determination of available sulfates. Soil Sci Soc Am J (1951) 15:149–51. doi: 10.2136/sssaj1951.036159950015000C0032x
43. Parker DR, Gardner EH. The determination of hot-water-soluble boron in some acid Oregon soils using a modified azomethine- h procedure. Commun Soil Sci Plant Anal (1981) 12:1311–22. doi: 10.1080/00103628109367237
44. Lindsay WL, Norvell W. Development of a DTPA soil test for zinc, iron, manganese, and copper. Soil Sci Soc Am J (1978) 42:421–8. doi: 10.2136/sssaj1978.03615995004200030009x
45. Vance ED, Brookes PC, Jenkinson DS. An extraction method for measuring soil microbial biomass c. Soil Biol Biochem (1987) . 19:703–7. doi: 10.1016/0038-0717(87)90052-6
46. Franzluebbers AJ, Arshad MA. Soil organic matter pools during early adoption of conservation tillage in northwestern Canada. Soil Sci Soc Am J (1996) 60:1422–7. doi: 10.2136/sssaj1996.03615995006000050019x
47. Keeney DR, Bremner JM. A chemical index of soil nitrogen availability. Nature (1966) . 211(5051):892–3. doi: 10.1038/211892a0
48. Dick RP, Breakwell DP, Turco RF. Soil enzyme activities and biodiversity measurements as integrative microbiological indicators. Methods assessing Soil Qual (1996) . 49:247–71. doi: 10.2136/sssaspecpub49.c15
49. Tabatabai MA, Bremner JM. Use of pnitrophenylphosphate for assay of soil phosphatase activity. Soil Biol Biochem (1969) 1:301–7. doi: 10.1016/0038-0717(69)90012-1
50. Tabatabai MA, Bremner JM. Arylsulfatase activity of soils. Soil Biol Biochem (1970) 34:225–9. doi: 10.2136/sssaj1970.03615995003400020016x
51. Brejda JJ, Moorman TB, Karlen DL, Dao TH. Identification of regional soil quality factors and indicators. i. central and southern high plains. Soil Sci Soc Am J (2000) 64:2115–24. doi: 10.2136/sssaj2000.6462115x
52. Liebig MA, Varvel G, Doran JW. A simple performance-based index forassessing multiple agroecosystem functions. Agron J (2001) 93:313–8. doi: 10.2134/agronj2001.932313x
53. Andrews SS, Karlen DL, Mitchell JP. A comparison of soil quality indexing methods for vegetable production systems in northern California. Agric Ecosyst Environ (2002) 90:25–45. doi: 10.1016/S0167-8809(01)00174-8
54. Andrews SS, Mitchell JP, Mancinelli R, Karlen DL, Hartz TK, Horwath WR, et al. On-farm assessment of soil quality in california’s central valley. Agron J (2002) 94:12–23. doi: 10.2134/agronj2002.1200
55. Dhaliwal SS, Naresh RK, Mandal A, Walia MK, Gupta RK, Singh R, et al. Effect of manures and fertilizers on soil physical properties, build-up of macro and micronutrients and uptake in soil under different cropping systems: a review. J Plant Nutr (2019) 42:2873–900. doi: 10.1080/01904167.2019.1659337
56. Agbede TM, Ojeniyi SO, Adeyemo AJ. Effect of poultry manure on soil physical and chemical properties, growth and grain yield of sorghum in southwest, Nigeria. Am Eurasian J Sustain Agric (2008) . 2:72–7.
57. Zeraatpisheh M, Ayoubi S, Mirbagheri Z, Mosaddeghi MR, Xu M. Spatial prediction of soil aggregate stability and soil organic carbon in aggregate fractions using machine learning algorithms and environmental variables. Geoderma Reg (2021) 27:e00440. doi: 10.1016/j.geodrs.2021.e00440
58. Das S, Bhattacharyya R, Das TK, Sharma AR, Dwivedi BS, Meena MC, et al. Soil quality indices in a conservation agriculture based rice-mustard cropping system in north-western indo-gangetic plains. Soil Till Res (2021) 208:104–24. doi: 10.1016/j.still.2020.104914
59. Bose P, Roy M, Patra PK. Evaluation of long term different nutrient management practices on crop productivity and soil quality in rice (Oryza sativa)-potato (Solanum tuberosum)-groundnut (Arachis hypogaea) cropping system in new alluvial soil zone of west bengal, India. Plant Arch (2021) 21:2383–92.
60. Hu Y, Zheng Q, Noll L, Zhang S, Wanek W. Direct measurement of the in situ decomposition of microbial-derived soil organic matter. Soil Biol Biochem (2020) 141:107660. doi: 10.1016/j.soilbio.2019.107660
61. Ross DS, Matschonat G, Skyllberg U. Cation exchange in forest soils: the need for a new perspective. Eur J Soil Sci (2008) . 59:1141–59. doi: 10.1111/j.1365-2389.2008.01069.x
62. Meena BP, Biswas AK, Singh M, Chaudhary RS, Singh AB, Das H, et al. Long-term sustaining crop productivity and soil health in maize–chickpea system through integrated nutrient management practices in vertisols of central India. Field Crops Res (2019) 232:62–76. doi: 10.1016/j.fcr.2018.12.012
63. Bhattacharyya R, Kundu S, Srivastva AK, Gupta HS, Prakash V, Bhatt JC. Long term fertilization effects on soil organic carbon pools in a sandy loam soil of the Indian sub-Himalayas. Plant Soil (2011) 341:109–24. doi: 10.1007/s11104-010-0627-4
64. Purakayastha TJ, Rudrappa L, Singh D, Swarup A, Bhadraray S. Long-term impact of fertilizers on soil organic carbon pools and sequestration rates in maize–wheat–cowpea cropping system. Geoderma (2008) 144:370–8. doi: 10.1016/j.geoderma.2007.12.006
65. Liu X, Yang T, Wang Q, Huang F, Li L. Dynamics of soil carbon and nitrogen stocks after afforestation in arid and semi-arid regions: a meta-analysis. Sci Total Environ (2018) 618:1658–64. doi: 10.1016/j.scitotenv.2017.10.009
66. Qin W, Wang D, Guo X, Yang T, Oenema O. Productivity and sustainability of rainfed wheat-soybean system in the north China plain: results from a long-term experiment and crop modelling. Sci Rep (2015) 5:17514. doi: 10.1038/srep17514
67. Thakur R, Sawarkar SD, Vaishya UK, Singh M. Impact of continuous use of inorganic fertilizers and organic manure on soil properties and productivity under soybean-wheat intensive cropping of a vertisol. J Indian Soc Soil Sci (2011) 59:74–81.
68. Patnaik PK. Soil enzyme activity and nutrient availability as influenced by long term manuring of a rice-rice cropping system in an acidic soil (Inceptisols) of bhubaneswar. [MSc Agric thesis] Orissa Univ Agric Technology Bhubaneswar odisha India. (2012).
69. Urkurkar JS, Alok T, Shrikant C, Bajpai RK. Influence of long-term use of inorganic and organic manures on soil fertility and sustainable productivity of rice (Oryza sativa) and wheat (Triticum aestivum) in inceptisols. Indian J Agric Sci (2010) 80:3 208–212.
70. Sarkar S, Singh SR. Integrated nutrient management in relation to soil fertility and yield sustainability under dryland farming. Indian J Agric Sci (1997) 67:431–3.
71. Sharma MP, Bali SV, Gupta DK. Soil fertility and productivity of rice (Oryza sativa) - wheat (Triticum aestivum) cropping system in an inceptisol as influenced by integrated management. Indian J Agric Sci (2001) 71:82–6.
72. Smith SR. A critical review of the bioavailability and impacts of heavy metals in municipal solid waste composts compared to sewage sludge. Environ Int (2009) 35:142–56. doi: 10.1016/j.envint.2008.06.009
73. Sidhu GS, Sharma BD. Diethylenetriaminepentaacetic acid–extractable micronutrients status in soil under a rice–wheat system and their relationship with soil properties in different agroclimatic zones of indo-gangetic plains of. India Commun Soil Sci Plant Anal (2010) 41:29–51. doi: 10.1080/00103620903360262
74. Hemalatha S, Radhika K, Maragatham S, Praveena KS. Influence of long term fertilization on soil fertility: A review. Res Rev (2013) 2:30–6.
75. Moharana PC, Sharma BM, Biswas DR. Changes in the soil properties and availability of micronutrients after six-year application of organic and chemical fertilizers using STCR-based targeted yield equations under pearl millet-wheat cropping system. J Plant Nutr (2017) 40:165–76. doi: 10.1080/01904167.2016.1201504
76. Walia MK, Walia SS, Dhaliwal SS. Long-term effect of integrated nutrient management of properties of typic ustochrept after 23 cycles of an irrigated rice (Oryza sativa l.), wheat (Triticum aestivum l.) system. J Sustain Agric (2010) 34:724–43. doi: 10.1080/10440046.2010.50751
77. Nikoli T, Matsi T. Influence of liquid cattle manure on micronutrients content and uptake by corn and their availability in a calcareous soil. Agron J (2011) 103:113–8. doi: 10.2134/agronj2010.0273
78. Katyal JC, Sharma BD. DTPA-extractable and total zn, Cu, Mn, and fe in Indian soils and their association with some soil properties. Geoderma (1991) 49:165–79. doi: 10.1016/0016-7061(91)90099-F
79. Keshavarzi B, Moore F, Ansari M, Rastegari MM, Kaabi H, Kermani M. Macronutrients and trace metals in soil and food crops of isfahan province, Iran. Environ Monit Assess (2015) 187:1–22. doi: 10.1007/s10661-014-4113-y
80. Zhang S, Li Z, Yang X. Effects of long-term inorganic and organic fertilization on soil micronutrient status. Commun Soil Sci Plant Anal (2015) 46:1778–90. doi: 10.1080/00103624.2015.1047843
81. Setia RK, Sharma KN. Effect of continuous cropping and long-term differential fertilization on profile stratification of DTPA-extractable micronutrients. J Food Agric Environ (2004).
82. Singh RJ, Ghosh BN, Sharma NK, Patra S, Dadhwal KS, Meena VS, et al. Effect of seven years of nutrient supplementation through organic and inorganic sources on productivity, soil and water conservation, and soil fertility changes of maize-wheat rotation in north-western Indian Himalayas. Agric Ecosyst Environ (2017) 249:177–86. doi: 10.1016/j.agee.2017.08.024
83. Das R, Mandal B, Sarkar D, Pradhan AK, Datta A, Padhan D, et al. Boron availability in soils and its nutrition of crops under long-term fertility experiments in India. Geoderma (2019) 351:116–29. doi: 10.1016/j.geoderma.2019.05.022
84. Niaz A, Ranjha AM, Rahmatullah AH, Waqas M. Boron status of soils as affected by different soil characteristics–pH, CaCO3, organic matter and clay contents. Pak J Agric Sci (2007) 44(3):428–35.
85. Nannipieri P, Giagnoni L, Landi L, Renella G. Role of phosphatase enzymes in soil. In: Bünemann EK, Oberson A, Frossard E, editors. Phosphorus in action. Berlin, Heidelberg: Springer (2011). p. 215–43.
86. Liu E, Yan C, Mei X, He W, Bing SH, Ding L, et al. Long-term effect of chemical fertilizer, straw, and manure on soil chemical and biological properties in northwest China. Geoderma (2010) 158(3-4):173–80. doi: 10.1016/j.geoderma.2010.04.029
87. Richardson AE, Simpson RJ. Soil microorganisms mediating phosphorus availability update on microbial phosphorus. Plant Physiol (2011) 156(3):989–96. doi: 10.1104/pp.111.175448
88. Mandal A, Patra AK, Singh D, Swarup A, Masto RE. Effect of long-term application of manure and fertilizer on biological and biochemical activities in soil during crop development stages. Bioresour Technol (2007) 98(18):3585–92. doi: 10.1016/j.biortech.2006.11.027
89. Bhatt B, Chandra R, Ram S, Pareek N. Long-term effects of fertilization and manuring on productivity and soil biological properties under rice (Oryza sativa)–wheat (Triticum aestivum) sequence in mollisols. Arch Agron Soil Sci (2016) 62(8):1109–22. doi: 10.1080/03650340.2015.1125471
90. Knauff U, Schulz M, Scherer HW. Arylsufatase activity in the rhizosphere and roots of different crop species. Eur J Agron (2003) 19(2):215–23. doi: 10.1016/S1161-0301(02)00035-7
91. Jain S, Mishra D, Khare P, Yadav V, Deshmukh Y, Meena A. Impact of biochar amendment on enzymatic resilience properties of mine spoils. Sci Total Environ (2016) 544:410–21. doi: 10.1016/j.scitotenv.2015.11.011
92. Zheng J, Chen J, Pan G, Liu X, Zhang X, Li L, et al. Biochar decreased microbial metabolic quotient and shifted community composition four years after a single incorporation in a slightly acid rice paddy from southwest China. Sci Total Environ (2016) 571:206–17. doi: 10.1016/j.scitotenv.2016.07.135
93. Purakayastha TJ, Das R, Kumari S, Shivay YS, Biswas S, Kumar D, et al. Impact of continuous organic manuring on mechanisms and processes of the stabilisation of soil organic c under rice–wheat cropping system. Soil Res (2019) 58(1):73–83. doi: 10.1071/SR19014
94. Adam G, Duncan H. Development of a sensitive and rapid method for the measurement of total microbial activity using fluorescein diacetate (FDA) in a range of soils. Soil Biol Biochem (2001) 33(7-8):943–51. doi: 10.1016/S0038-0717(00)00244-3
95. Dick RP, Breakwell DP, Turco RF. Soil enzyme activities and biodiversity measurements as integrative microbiological indicators. Methods assessing Soil Qual (1997). doi: 10.2136/sssaspecpub49.c15
96. Basak N, Datta A, Mitran T, Mandal B, Mani PK. Impact of organic and mineral inputs onto soil biological and metabolic activities under a long-term rice-wheat cropping system in sub-tropical Indian inceptisols. J Environ Biol (2016) . 37(1):83.
97. Nayak DR, Babu YJ, Adhya TK. Long-term application of compost influences microbial biomass and enzyme activities in a tropical aeric endoaquept planted to rice under flooded condition. Soil Biol Biochem (2007) 39(8):1897–906. doi: 10.1016/j.soilbio.2007.02.003
98. Bhattacharyya R, Kundu S, Prakash V, Gupta HS. Sustainability under combined application of mineral and organic fertilizers in a rainfed soybean–wheat system of the Indian Himalayas. Eur J Agron (2008) 28(1):33–46. doi: 10.1016/j.eja.2007.04.006
99. Burns RG. Enzyme activity in soil: location and a possible role in microbial ecology. Soil Biol Biochem (1982) 14(5):423–7. doi: 10.1016/0038-0717(82)90099-2
100. Zhang Q, Li Y, He Y, Liu H, Dumont MG, Brookes PC, et al. Nitrosospira cluster 3-like bacterial ammonia oxidizers and nitrospira-like nitrite oxidizers dominate nitrification activity in acidic terrace paddy soils. Soil Biol Biochem (2019) 131:229–37. doi: 10.1016/j.soilbio.2019.01.006
101. Chen QL, Ding J, Zhu D, Hu HW, Delgado-Baquerizo M, Ma YB, et al. Rare microbial taxa as the major drivers of ecosystem multifunctionality in long-term fertilized soils. Soil Biol Biochem (2020) 141:107686. doi: 10.1016/j.soilbio.2019.107686
102. Chhonkar PK, Tarafdar JC. Characteristics and location of phosphatases in soil-plant system. J Indian Soc Soil Sci (1981) 29(2):215–9.
103. Dick RP, Rasmussen PE, Kerle EA. Influence of long-term residue management on soil enzyme activities in relation to soil chemical properties of a wheat-fallow system. Biol Fertil Soils (1988) . 6:159–64. doi: 10.1007/BF00257667
104. Bandick AK, Dick RP. Field management effects on soil enzyme activities. Soil Biol Biochem (1999) 31(11):1471–9. doi: 10.1016/S0038-0717(99)00051-6
105. Patil RB, Puranik RB. Microbial biomass c and n as influenced by cropping systems and nutrient management. PKV Res (2001) 25(2):73.
106. Basak BB, Biswas DR, Rattan RK. Comparative effectiveness of value-added manures on crop productivity, soil mineral nitrogen and soil carbon pools under maize-wheat cropping system in an inceptisol. J Indian Soc Soil Sci (2012) . 60(4):288–98.
107. Zhang J, Qin J, Yao W, Bi L, Lai T, Yu X. Effect of long-term application of manure and mineral fertilizers on nitrogen mineralization and microbial biomass in paddy soil during rice growth stages. Plant Soil Environ (2009) 55(101):e109. doi: 10.17221/322-PSE
108. Majumder B, Mandal B, Bandyopadhyay PK, Chaudhury J. Soil organic carbon pools and productivity relationships for a 34 year old rice–wheat–jute agroecosystem under different fertilizer treatments. Plant Soil (2007) 297:53–67. doi: 10.1007/s11104-007-9319-0
109. Weil RR, Islam KR, Stine MA, Gruver JB, Samson-Liebig SE. Estimating active carbon for soil quality assessment: A simplified method for laboratory and field use. Am J Altern Agric (2003) 18(1):3–17. doi: 10.1079/AJAA200228
110. Keeney DR. Nitrogen–availability indices. methods of soil analysis: Part 2 chemical and microbiological properties. (1983) 9:711–33. doi: 10.2134/agronmonogr9.2.2ed.c35
111. Whalen JK, Chang C, Olson BM. Nitrogen and phosphorus mineralization potentials of soils receiving repeated annual cattle manure applications. Biol Fertil Soils (2001) 34:334–41. doi: 10.1007/s003740100416
112. Masto RE, Chhonkar PK, Singh D, Patra AK. Soil quality response to long-term nutrient and crop management on a semi-arid inceptisol. Agric Ecosyst Environ (2007) 118(1-4):130–42. doi: 10.1016/j.agee.2006.05.008
113. Bhaduri D, Purakayastha TJ. Long-term tillage, water and nutrient management in rice–wheat cropping system: Assessment and response of soil quality. Soil Till Res (2014) 144:83–95. doi: 10.1016/j.still.2014.07.007
114. Ghorai PS, Biswas S, Purakayastha TJ, Ahmed N, Das TK, Prasanna R, et al. Indicators of soil quality and crop productivity assessment at a long-term experiment site in the lower indo-gangetic plains. Soil Use Manag (2022) 27. doi: 10.1111/sum.12847
115. Gregorich EG, Carter MR, Angers DA, Monreal C, Ellert BH. Towards a minimum data set to assess soil organic matter quality in agricultural soils. Can J Soil Sci (1994) 74(4):367–85. doi: 10.4141/cjss94-051
116. Purakayastha TJ, Pathak H, Kumari S, Biswas S, Chakrabarty B, Padaria RN, et al. Soil health card development for efficient soil management in haryana, India. Soil Till Res J (2019) 1:191:294–305. doi: 10.1016/j.still.2018.12.024
117. Roy D, Datta A, Jat HS, Choudhary M, Sharma PC, Singh PK, et al. Impact of long term conservation agriculture on soil quality under cereal based systems of north West India. Geoderma (2022) 405:115391. doi: 10.1016/j.geoderma.2021.115391
118. Schnrer J, Rosswall T. Fluorescein diacetate hydrolysis as a measure of total microbial activity in soil and litter. Appl Environ Microbiol (1982) 43(6):1256–61. doi: 10.1128/aem.43.6.1256-1261.1982
119. Patra A, Sharma VK, Purakayastha TJ, Barman M, Kumar S, Chobhe KA, et al. Effect of long-term integrated nutrient management (INM) practices on soil nutrients availability and enzymatic activity under acidic inceptisol of north-Eastern region of India. Commun Soil Sci Plant Anal (2020) 51(9):1137–49. doi: 10.1080/00103624.2020.1751185
120. Vasu D, Singh SK, Ray SK, Duraisami VP, Tiwary P, Chandran P, et al. Soil quality index (SQI) as a tool to evaluate crop productivity in semi-arid deccan plateau, India. Geoderma (2016) 282:70–9. doi: 10.1016/j.geoderma.2016.07.010
121. Rahaman R, Bhindhu PS, Abraham M. Flood aftermath on soil quality in north central laterites of kerala. J Indian Soc (2022) 70(2):204–12. doi: 10.5958/0974-0228.2022.00020.2
122. Rinot O, Levy GJ, Steinberger Y, Svoray T, Eshel G. Soil health assessment: A critical review of current methodologies and a proposed new approach. Sci Total Environ (2019) 648:1484–91. doi: 10.1016/j.scitotenv.2018.08.259
123. Sharma B, Sarkar A, Singh P, Singh RP. Agricultural utilization of biosolids: A review on potential effects on soil and plant grown. J Waste Manag (2017) 64:117–32. doi: 10.1016/j.wasman.2017.03.002
124. Sharma K, Reddy KS, Chary GR, Singh VK, Lal M, Nath KG, et al. Effect of integrated nutrient management (INM) practices on soil quality indicators and indices under sorghum (Sorghum bicolor (L.) moench)+ pigeon pea (Cajanus cajan (L.) Millsp.)(4: 2) and soybean (Glycine max (L) merr)+ pigeon pea (Cajanus cajan (L.) millsp.) in rainfed vertisols of Western India. Ann Plant Soil Res (2021) 23:379–89. doi: 10.47815/apsr.2021.10088
Keywords: equivalent rice yield, PCA, rainfed, rice-lentil, soil quality
Citation: Biswas S, Singh P, Rahaman R, Patil KV and De N (2023) Soil quality and crop productivity under 34 years old long-term rainfed rice based cropping system in an Inceptisol of sub-tropical India. Front. Soil Sci. 3:1155712. doi: 10.3389/fsoil.2023.1155712
Received: 31 January 2023; Accepted: 30 March 2023;
Published: 24 April 2023.
Edited by:
Santosh Ranjan Mohanty, Indian Institute of Soil Science (ICAR), IndiaReviewed by:
Vishal Tripathi, Graphic Era University, IndiaShamsollah Ayoubi, Isfahan University of Technology, Iran
Copyright © 2023 Biswas, Singh, Rahaman, Patil and De. This is an open-access article distributed under the terms of the Creative Commons Attribution License (CC BY). The use, distribution or reproduction in other forums is permitted, provided the original author(s) and the copyright owner(s) are credited and that the original publication in this journal is cited, in accordance with accepted academic practice. No use, distribution or reproduction is permitted which does not comply with these terms.
*Correspondence: Sunanda Biswas, c3VuYW5kYWJpc3dhczEzQGdtYWlsLmNvbQ==