- 1Horticultural Sciences Department, Institute of Food and Agricultural Sciences, Indian River Research and Education Center, University of Florida, Fort Pierce, FL, United States
- 2Agricultural and Biological Engineering Department, Institute of Food and Agricultural Sciences, Indian River Research and Education Center, University of Florida, Fort Pierce, FL, United States
Introduction: The rhizosphere community composition has been shown to provide benefits in nutrient acquisition and plant health. Rhizosphere communities can be heavily influenced by cultural practices in citrus production systems, providing benefits in soil and root health, and plant physiology. Florida growers are implementing new and more sustainable soil management practices, such as using fabric mulch ground covers to improve weed and pest control, while retaining soil moisture. Little is known regarding the influence of these ground covers on the rhizosphere microbial communities of lemon trees, especially under endemic Huanglongbing pressure. Understanding how lemon roots and the rhizosphere microbiome are influenced by fabric mulch ground covers can potentially lead to improved management practices. The aim of this research was to evaluate the effects of fabric mulch ground covers on lemon tree rhizosphere health.
Methods: The experiment was conducted in a commercial citrus grove located in Fort Pierce, FL, US. Plant material consisted of four-year old lemon trees cv. ‘Meyer’ (Citrus limon) grafted on ‘sour orange’ (Citrus × aurantium) rootstocks. The experiment consisted of two treatments, which included trees grown with fabric mulch ground covers and trees grown without fabric mulch ground covers. Rhizosphere DNA was extracted, sequenced, and analyzed to assess differences in diversity and composition (alpha and beta diversity) of rhizosphere bacteria among treatments.
Results and Discussion: Obtained results showed that lemon trees treated with fabric mulch ground covers had significantly greater rhizosphere bacterial diversity when compared to the uncovered trees. The presence of fabric mulch ground covers resulted in significantly greater soil Zn, soil Mn, soil temperatures and pH, potentially contributing towards the significant more diverse rhizosphere bacterial commu nity composition compared than those grown without fabric mulch ground covers.
Introduction
Huanglongbing is a disease that impacts citrus on a global scale and has reduced citrus production in Florida by more than 67% over the course of 15 years. The bacterium Candidatus Liberibacter asiaticus (CLas) has been associated with HLB (1, 2). CLas is transmitted through the Asian citrus psyllid (ACP; Diaphorina citri; (3, 4) Currently, there is no known cure for HLB, and although some citrus germplasm can show a degree of tolerance, there are no HLB-resistant cultivars or rootstocks (5, 6).
Use of cultural practices in citrus production systems can provide benefits in soil health, plant physiology, distribution of roots, and plant health (7, 8). It has been shown that cultural practices could improve the functional and structural status of a root system (9). Soil conditions such as temperature, structure, and aeration increases root function (8). Cultural practices, such as fabric mulch ground covers, have been shown to reduce the pressure of pests and weeds on crops (8). By covering the soil surface, ground covers protect the topsoil by reducing mechanical damage and soil erosion that would be caused by rainfall (10). Furthermore, ground covers better assist in the maintenance of soil moisture for production systems, by reducing the rate of evaporation from the soil surface (11). However, much of the fabric mulch ground cover research has been applied to annual row crops, and recently grapefruit (Citrus × paradisi) (8).
Use of fabric mulch ground covers in Florida sandy soils can also benefit soil health by reducing rates of nutrient leaching, via decreased lateral movement of water. Reduced leaching rates allow for nutrients to persist within the root zone, increasing the availability of plant essential minerals that are critical towards plant health. Phosphorus (P), for instance, is an essential nutrient because it plays a key role in cell division and growth of meristematic tissues (12). Sufficient soil P is also crucial for root growth, notably for lateral and fibrous root development (12). Additionally, Potassium (K) is critical for physiological functions, some of which include the metabolism of sugar and starch, and cell division (13). In terms of citrus, K has vital interaction with the supply of carbon dioxide (CO2) by controlling the opening and closing of stomata (13). Furthermore, zinc (Zn) is integral to enzyme systems, as it facilitates metabolic processes in plants, which include RNA polymerases, superoxide dismutase, and protein synthesis (14). Zinc is also utilized by plants to assists in the production of auxins that promote growth, chlorophyll, and carbon metabolism (14).
Changes to the soil characteristics provided by ground covers may have subsequent effects on microbial communities residing within the bulk soil and rhizosphere. Many of these microorganisms are sensitive to changes in soil moisture and temperature, and pH, which are soil characteristics affected by ground cover use (11, 15). These live close to, on, and inside the root tissues, and include bacteria, archaea, and fungi. Collectively these microorganisms form communities associated with different rhizosphere zones, which include the rhizoplane, ecto-rhizosphere (zone of this study’s interest), and endo-rhizosphere (16). Microorganisms within the rhizosphere can affect plant health by providing a supply of essential nutrients (e.g., nitrogen fixation or phosphate solubilization), promoting plant growth via phytohormone production, and maintaining plant health through release of antibiotics that reduce growth and establishment of pathogenic organisms (17, 18).
During periods of stress (e.g., lack of water or nutrient availability), plants often respond by releasing root exudates that recruit beneficial microbes, also known as plant growth promoting rhizobacteria (PGPR), from the bulk soil to the rhizosphere. Plant growth promoting rhizobacteria (PGPR) are bacteria that reside within the rhizosphere and have the capability to promote plant development (19). Some beneficial functions provided by PGPR include the ability to break down immobile nutrients into soluble forms that can readily be up taken by roots of plants, including the ability to assist in plant host defenses through the production of antibiotics (e.g. lipopeptides, polyketides and antifungal metabolites) that suppress pathogens and natural outcompete pathogenic organisms that can negatively impact plant health (20, 21). Furthermore, some of these root-associated microbes are beneficial to citrus. For example, Pseudomonas, Agrobacterium, Bradyrhizobium, Rhizobium, and Burkholder have all been found to inhibit plant disease, specifically under Huanglongbing (HLB) (22–25). Further analysis of the effects shared between fabric mulch ground covers and citrus is needed to determine possible implications on citrus root and rhizosphere health, which is especially important under endemic HLB conditions (26, 27).
The objective of this study was to examine the effect of fabric mulch ground covers on citrus rhizosphere community composition, and subsequent effects on citrus root and soil health. It is predicted that the increased soil moisture, soil temperatures and pH from ground covers will result in a more diverse citrus rhizosphere composition. Additionally, since the fabric mulch ground covers are expected to retain more water and nutrients in the root zone, it is hypothesized that lemon trees treated with fabric mulch ground cover will result in a lower HLB bacterial titer count when compared to uncovered trees.
Materials and methods
Site description and experimental design
The experiment took place on an 81 Ha commercial citrus grove, located in Fort Pierce, FL (Latitude 27.462778; Longitude -80.540833) (Figure 1A). From the 81 Ha, 1.6 Ha were utilized for this study. Four-year old lemon trees cv. ‘Meyer’ (Citrus limon) grafted on ‘sour orange’ (Citrus × aurantium) rootstocks were planted on raised beds in 2019 and immediately covered with a fabric mulch system as a ground cover. The fabric mulch ground cover utilized in this study was manufactured by Lumite, Inc (http://www.lumiteinc.com/products/groundcover) and is comprised of a blend of woven fabrics and UV polypropylene that allows passage of water and nutrients at a rate of 720 L min/m2. Additionally, marker yarns were knitted with a 30 cm spacing. The soil that the trees were grown is classified by the United States Department of Agriculture (USDA) as an ‘Alfisol’ mainly comprised of ‘Riviera’ fine sand soils, characterized by both sandy and loamy sediments (USDA, Accessed on 9/2022). The grove was managed with an automated irrigation controller that uses soil moisture data for irrigation scheduling. Irrigation was provided through a micro-sprinkler irrigation system with one micro-sprinkler per tree providing 29.15 Lph at a pressure of 1.38 bar. Two Acclima Time Domain Transmissometer (TDT) SD-12 soil moisture sensors per treatment were installed vertically at 7.62 cm below ground level with a rod that measures the average moisture between 7-20 cm depth. The sensors collected soil moisture data every 30 minutes for the time of this study and a traceable thermometer (Fisher Scientific, Waltham, MA, USA) was used to retrieve soil temperature data. Rhizosphere sampling took place in June 2021. Additionally, root health was assessed using both root nutrients contents and root scans in June 2021.
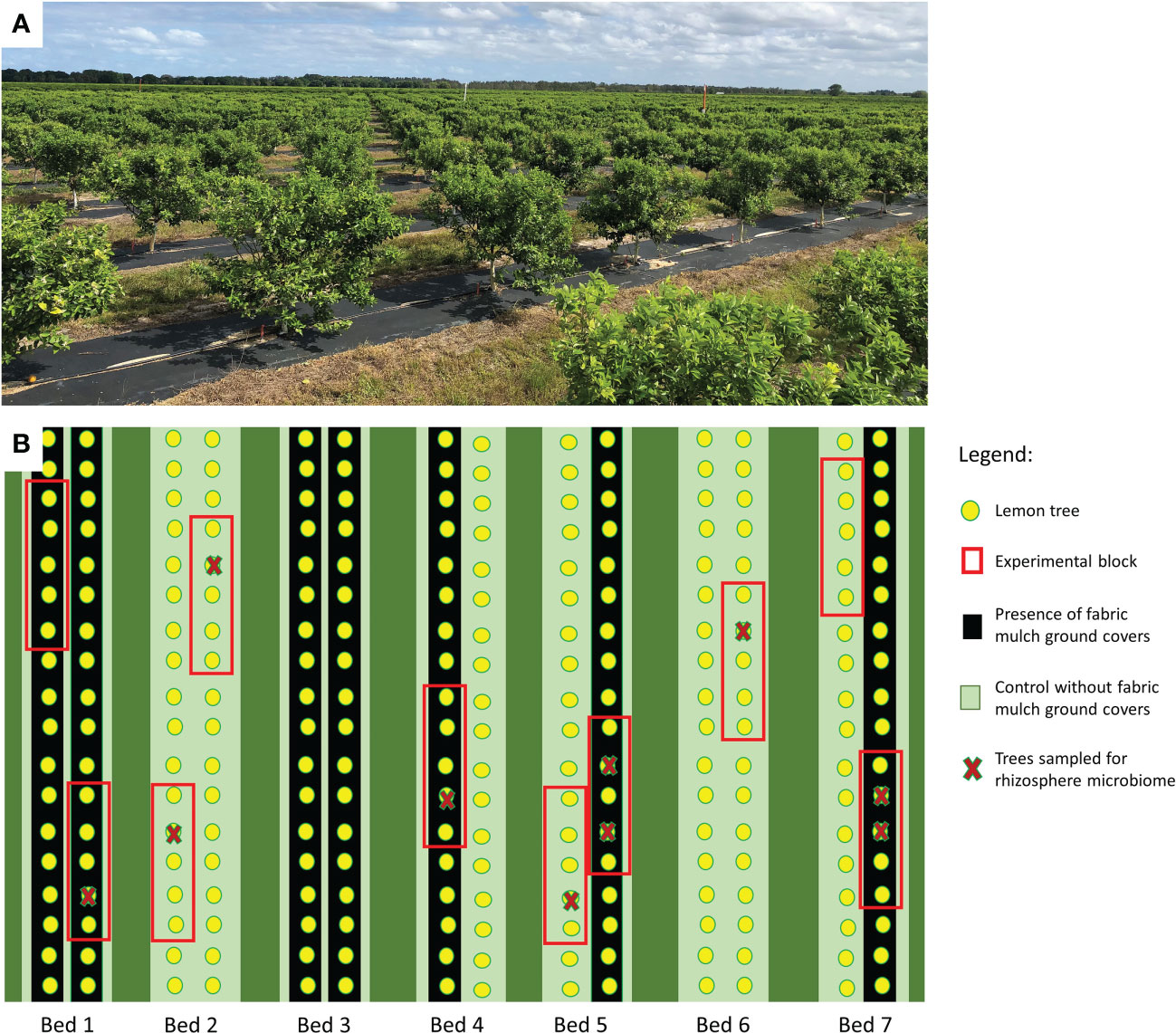
Figure 1 Lemon tree grove (A) and experimental design (B) of 4-year-old lemon trees planted in flatwood soils located in the Florida, USA and grown with or without fabric mulch ground covers.
The experiment consisted of a completely randomized block design with two treatments, which included trees grown with fabric mulch ground covers and trees grown without fabric mulch ground covers (Figure 1B). There was a total of 25 trees within each treatment, including five replication per treatment. A total of 5 trees from each treatment were randomly selected for rhizosphere sampling, thus providing 5 replicates per treatment.
Soil and leaf nutrient analysis
A soil auger (One-Piece Auger model #400.48, AMS, Inc., American Falls, ID, USA) 7 cm in diameter and 10 cm in depth was used to collect soil samples, which were later analyzed for nutrition. A single core was taken within the irrigated zone (80 cm from the stem of the tree) from 5 trees in the covered and 5 trees in the uncovered treatments across 10 different experimental blocks. Soil samples were analyzed for pH, cation exchange capacity (CEC) and nitrogen, phosphorous, potassium, magnesium, calcium, sulfur, boron, zinc, manganese, iron, and copper.
Soil samples were dried overnight and soil nutrients concentration was determined using Mehlich III extraction (28). Briefly, 2.5 g of soil were sieved through a 0.5 mm screen (10 mesh) into an extraction tube (125 mL). A 20 mL of Mehlich III extractant solution (0.2 M CH3COOH + 0.015 M NH4F + 0.013 M HNO3 + 0.001 M EDTA + 0.25 M H4NO3) was pipetted into the extraction tube containing the dry soil samples. The extraction tubes were placed on a mechanical shaker for 5 minutes. The soil suspension was filtered and placed into inductively coupled plasma (ICP) racks. Soil nutrients concentration was determined using ICP optical emission spectroscopy (ICP-OES, Spectro Ciros CCD, Fitzburg, MA, USA) (29). The ICP machine was calibrated following manufacturer instruction. The calibration was verified using verification standards and quality controls were ran every 40 samples. Concentrations values from ICP read directly in lbs/A and were later converted in kg/ha.
Leaf samples were rinsed with deionized water to remove excess nutrients from the sample surface. Samples were then dried at 80 °C overnight. After drying, samples were ground using a Thomas Wiley mill (Thomas Scientific, Swedesboro, NJ, USA) to pass through a 1.0 mm (20 mesh) screen. Ground samples were collected in a 20 mL vial and kept at room temperature. Five mL of concentrated HNO3 was added to each sample. Samples were than covered with a watch glass and then the tubes were placed on a digestor (DigiBlock 3000, SPC Science, USA) at 95 °C for 90 minutes. Tubes were placed out of the digestor and four mL of 30% H2O2 were added to each tube and the tubes were placed back to the digestor for 20 minutes. Tubes were thane cooled for 2 minutes and distilled water (DI) H2O was added to each tube to reach the 50 mL volume mark. Samples were then examined using an ICP-MS spectrophotometer (Spectro Ciros CCD, Fitzburg, MA, USA) (30). Calibrations were obtained using DI H2O as a blank couple with a set of standards. A quality check was run every 48 samples. The nutrient concentrations obtained from the ICP-MS were expressed by percentage of tissue dry mass.
Root parameter analysis
Root length was measured using the CID Bio-Science CI-602 Minirhizotron system (CID Bioscience, Inc. Camas, WA, USA). Each of the tubes consisted of 3 different scannable windows at different depths. All 3 windows in every tube were scanned during each sampling point. After the images were acquired, root length and density were calculated using RootSnap™ software (Software Version 1.3.2.25).
Huanglongbing (HLB) bacteria titer
Plant material utilized for CLas DNA concentration analysis consisted of HLB-symptomatic, mature, and fully expanded leaves. Among the trees that were selected for sampling, 4 leaves were sampled from the 4 cardinal sections. Leaf sampling took place in June 2021. Once collected, midribs were excised and chopped together (100 mg total). Samples were cooled with liquid nitrogen immediately prior to bead beating. The DNA was then extracted from freshly ground midrib using the DNeasy Plant Mini kit® (Qiagen, Hilden, Germany) following the kit protocol, except eluting with 2 rounds of 0.05 cm3 of extraction buffer instead of 2 rounds of 0.10 cm3. Quantification of CLas DNA was done with Applied Biosystems 7500 Fast Real-Time PCR System (Thermo Fisher Scientific, Waltham, MS, USA) using the CQULA primers/probe set (31) with a standard curve of 101 to 106 copies of pLBA2 plasmid (32). The PCR reaction was performed in a 25 µL reaction mixture containing 1 × PCR buffer (Bio-Rad Master Mix), 0.8 µm of each primer, 0.2 µm TaqMan probe and the appropriate amount of DNA template. The iCycler IQ Multiplex Real Time PCR DNA System (Bio-Rad) was used with the following program for DNA amplification: 95°C for 1 min, 45 cycles each of 95°C for 15 s, 59°C for 15 s and 72°C for 45 s. During the extension step (72°C for 45 s) of each cycle the fluorescent signal was generated from the excited reporter dye-labelling probe. Any samples that consisted of a cycle threshold (Ct) below 38 were deemed CLas-positive (33).
Rhizosphere DNA isolation
Rhizosphere samples were taken shortly after bulk soil sampling, from the same 5 trees in the covered and 5 trees in the uncovered treatments across the 10 different experimental blocks. The samples consisted of rhizosphere soil (located around the roots) being lightly shaken from the roots and placed in 50 ml sterile tubes. Approximately 50 g of rhizosphere soil was collected from each plant. Samples were stored at -20°C prior to DNA extraction. Approximately 15 mL of sterile Phosphate Buffered Saline (800 ml distilled water, 8 g NaCl, 0.2 g KCl, 1.44 g Na2PO4, 0.24 g KH2PO4) was added to the sample, and shaken by hand for 15 seconds. Roots were removed with forceps and discarded, the remaining soil was centrifuged at 3000 G for 15 minutes, and the supernatant was later discarded. Soil DNA was extracted from 0.25 g of the soil pellet using the DNeasy PowerSoil Kit (Qiagen Inc., Germantown, MD, USA) according to the manufacturer’s instructions.
DNA quantification
A Qubit fluorometer (Thermofisher Scientific, Waltham, MA, USA) was used to quantify extracted DNA and determine if DNA is concentrated enough for sequencing (> 1 ng μl-1). Rhizosphere DNA was amplified and sequenced at the Genomics and Microbiome Core Facility at Rush University (Chicago, IL, USA).
Rhizosphere DNA was PCR amplified with primers CS1_515F and CS2_926R targeting the V4-V5 variable regions of microbial small subunit ribosomal RNA genes. Amplicons were generated using a two-stage PCR amplification protocol as described previously. The primers contained 5’ common sequence tags (known as common sequence 1 and 2, CS1 and CS2). First stage PCR amplifications were performed in 10 µL reactions in 96-well plates, using repliQa HiFi ToughMix (Quantabio). PCR conditions were 98°C for 2 min, followed by 28 cycles of 98°C for 10 s, 50°C for 1 s and 68°C for 1 s.
Subsequently, a second PCR amplification was performed in 10 µL reactions in 96-well plates using repliQa HiFi ToughMix. Each well received a separate primer pair with a unique 10-base barcode, obtained from the Access Array Barcode Library for Illumina (Fluidigm, San Francisco, CA, USA). One microliter of PCR product from the first stage amplification was used as template for the 2nd stage, without cleanup. Cycling conditions were 98°C for 2 minutes, followed by 8 cycles of 98°C for 10 s, 60°C for 1 s and 68°C for 1 s. Libraries were then pooled and sequenced with a 15% phiX spike-in on an Illumina MiSeq sequencer employing V3 chemistry (2 × 300 base paired-end reads). Library preparation and sequencing were performed at the Genomics and Microbiome Core Facility (GMCF) at Rush University (Chicago, IL, USA).
Rhizosphere analysis
After sequencing, bioinformatic data was processed using DADA2 (34) within the Qiime 2 (35) package. Raw sequences were demultiplexed. DADA2 was used to filter chimeras, primers, and adapters, as well as assemble pair-ended sequences. Taxonomy was assigned to amplicon sequence variants (ASVs) with the reference datasets SILVA 128 database for 16S rRNA using naïve Bayes classifier in Qiime2 (36).
Plant and soil data statistical analysis
A Student’s t-test was performed with R software 3.6.0 (RStudio, Boston, MA, USA). Main effect means were separated using Tukey’s honestly significant difference post-hoc test. Differences were considered significant when p values were less than or equal to 0.05.
Rhizosphere composition statistical analysis
Alpha and beta diversity analyses of the bacterial community were performed on log-normalized data to avoid an increase in error rates due to rarefaction (37) with the R package “Phyloseq” v1.24.0 (38). Alpha diversity analyses included the number of observed ASVs (Shannon index), whereas beta diversity analyses included principal coordinate analysis (PCoA) on weighted UniFrac distances. A two-way analysis of similarities (ANOSIM) test was performed to determine significant differences in beta diversity between treatments. Canonical correspondence analysis (CCA) was performed to visualize the interactions between the rhizosphere bacterial communities among fabric mulch ground cover treatments, soil and root parameters, and soil nutrient concentrations. An analysis of similarity (ANOSIM) based on 999 permutations was then used to make multivariate comparisons of groups obtained with CCA to better determine the significance of the soil and root parameters and soil nutrient concentrations on rhizosphere bacterial community composition.
Results
Impact of fabric mulch ground covers on leaf mineral contents
Lemon trees planted without fabric mulch ground covers had a greater leaf macronutrient concentration of N, P, Mg, Ca, and S compared to trees treated with fabric mulch ground covers (Figure 2A). Conversely, lemon trees treated with fabric mulch ground covers had a greater leaf macronutrient concentration of K compared to those planted without fabric mulch ground covers (Figure 2A). Lemon treated with fabric mulch ground covers had a greater leaf micronutrient concentration of Zn, Mn, and Cu compared to trees planted without fabric mulch ground covers (Figure 2B). Alternatively, Lemon planted without fabric mulch ground covers had a greater leaf micronutrient concentration of B and Fe than those treated with fabric mulch ground covers (Figure 2B). However, no significant differences were detected in both leaf macro- and micronutrient contents.
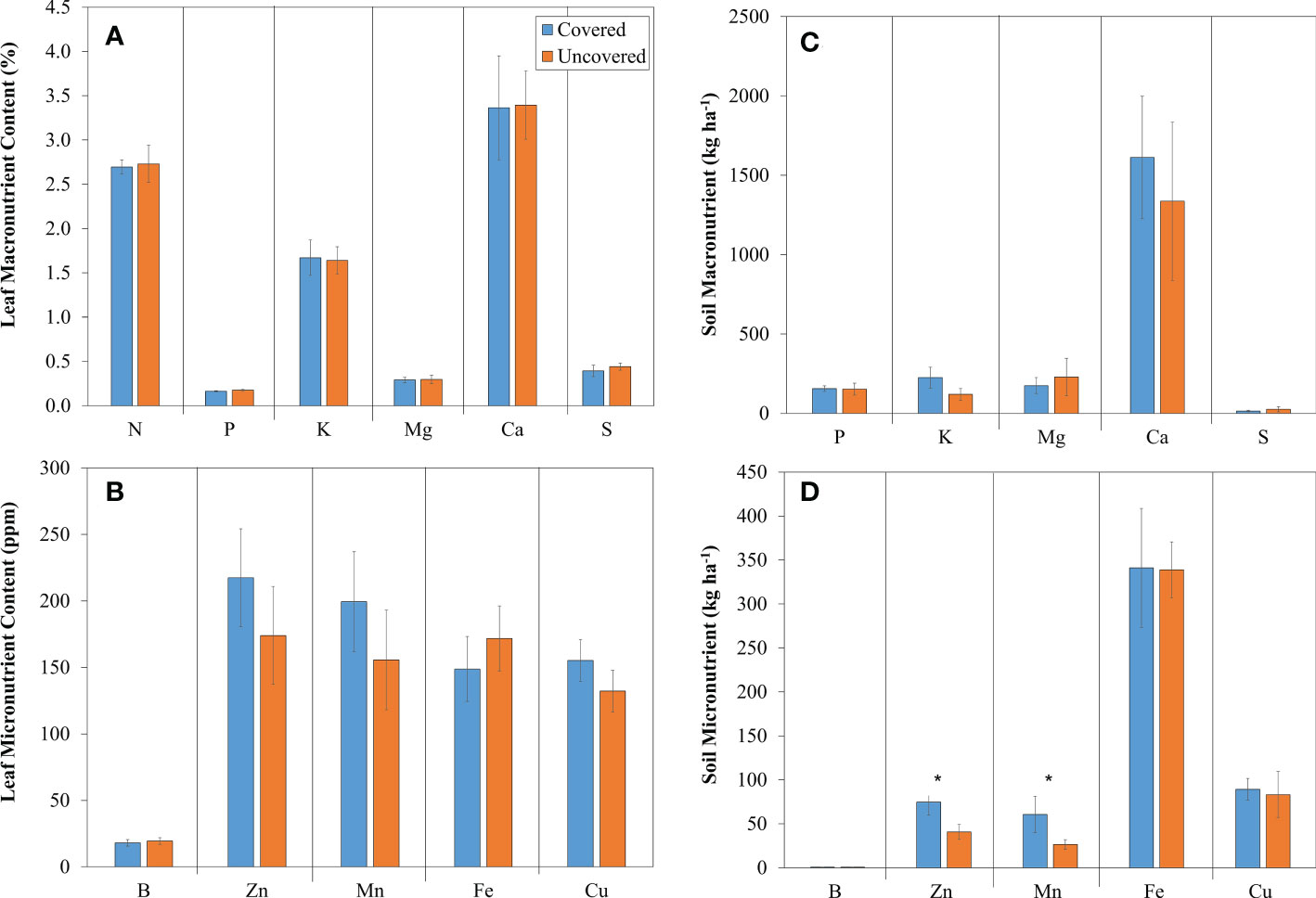
Figure 2 Macronutrients (A, C) and Micronutrients (B, D) in leaves and soil of 4-year-old lemon trees planted in flatwood soils located in the Florida, USA and grown with or without fabric mulch ground covers. Bars are ± standard deviation of the mean. Treatments with * were considered significantly different (p<0.05).
Impact of fabric mulch ground covers on soil mineral concentrations
Lemon trees treated with fabric mulch ground covers had greater soil macronutrient concentrations of K, P and Ca compared to those planted without fabric mulch ground covers (Figure 2C). Lemon trees planted without ground covers had greater soil macronutrient concentrations of Mg, and S than those treated with fabric mulch ground covers (Figure 2C). Lemon treated with fabric mulch ground covers had a statistically significantly greater soil micronutrient concentration of Mn (180% higher) and Zn (100% higher) than those planted without fabric mulch ground covers (Figure 2D). Greater soil micronutrient concentrations of Fe and Cu were also found in lemon trees treated with fabric mulch ground covers when compared to those planted without fabric mulch ground covers, but these differences were not significant (Figure 2D). Lemon planted without fabric mulch ground covers had a greater soil micronutrient concentration of B compared to those treated with fabric mulch ground covers (Figure 2D).
Impact of fabric mulch ground covers on root growth
Total root length and average root diameter did not vary across treatments. Lemon trees planted without fabric mulch ground covers did not have a greater total root length or average root diameter when compared to trees treated with fabric mulch ground covers (Figures 3A, B).
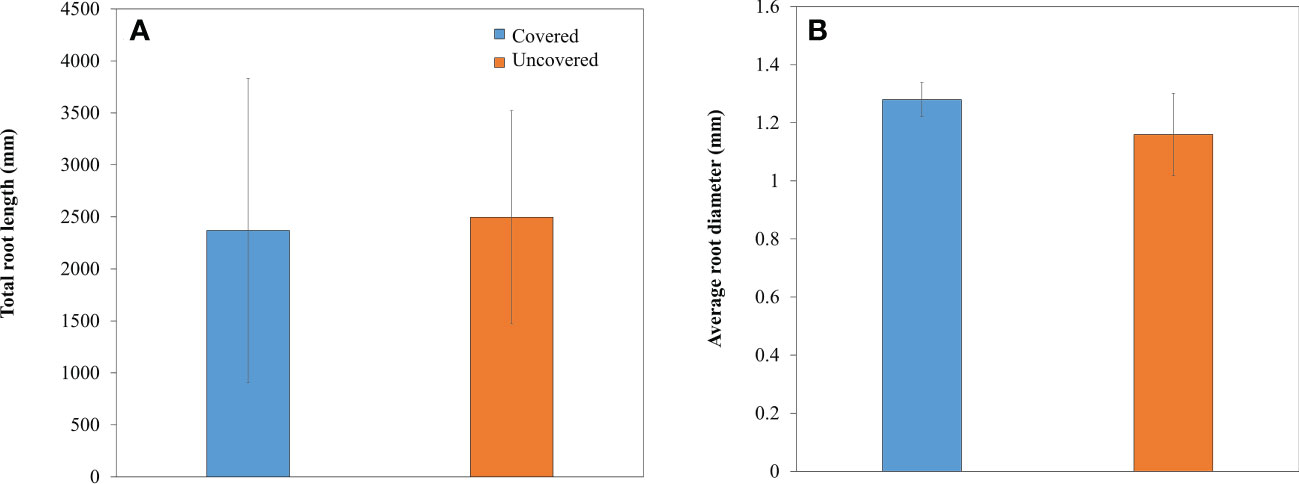
Figure 3 Total root length (A) and average root diameter (B) of 4-year-old lemon trees planted in flatwood soils located in the Florida, USA and grown with or without fabric mulch ground covers. Bars are ± standard deviation of the mean.
Impact of fabric mulch ground covers on HLB bacteria titer
Lemon trees treated with fabric mulch ground covers had a greater titer count (31.78) than those planted without ground covers (27.96). However, the difference was not statistically significant. The Ct values of trees from both treatments indicate that the trees were HLB positive (Figure 4).
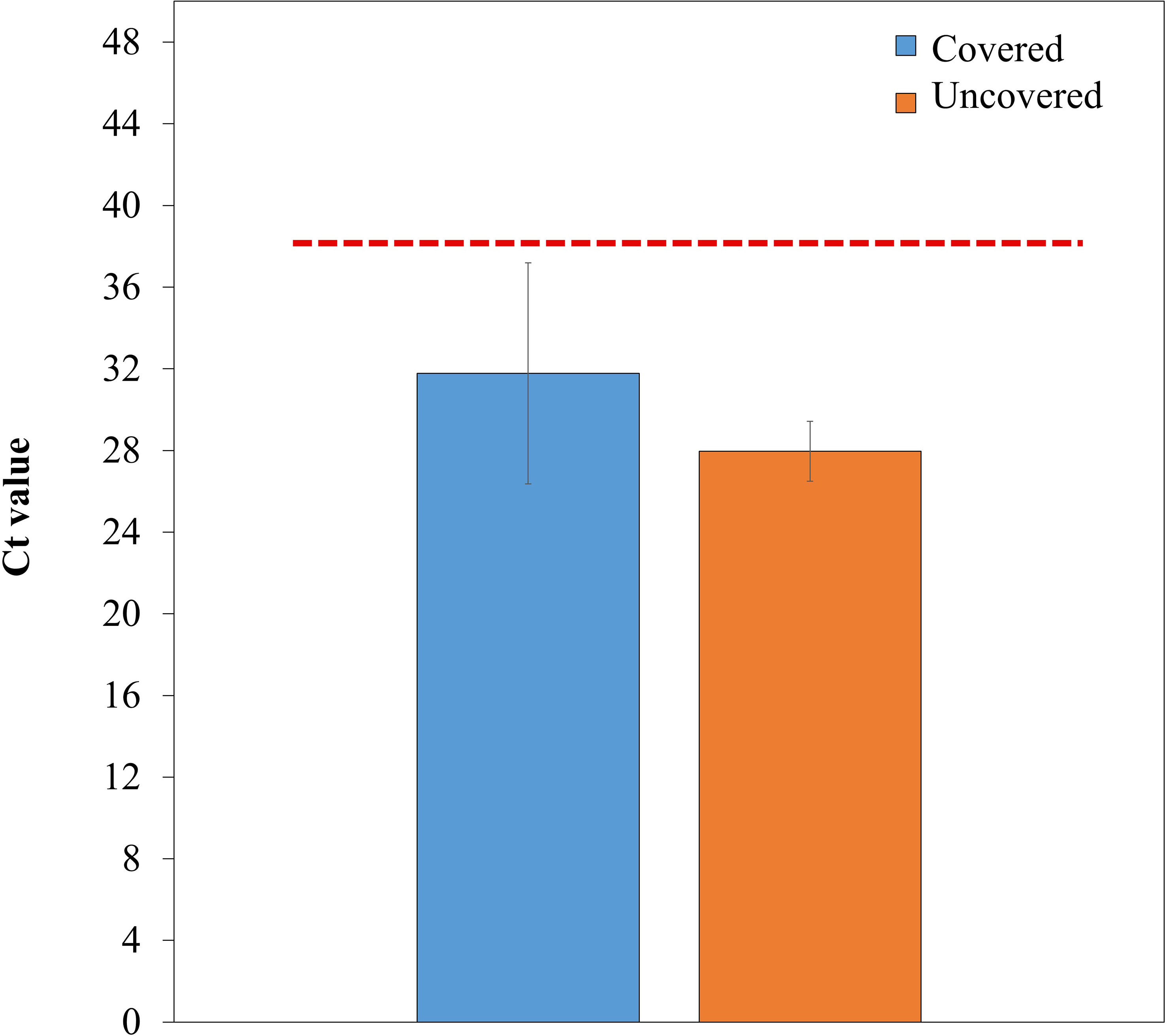
Figure 4 Ct value of Candidatus Liberibacter asiaticus (CLas) DNA of 4-year-old lemon trees planted in flatwood soils located in the Florida, USA and grown with or without fabric mulch ground covers. Values below the red-dotted line (38.0) are considered infected by CLas (33). Bars are ± standard deviation of the mean.
Impact of fabric mulch ground covers on soil parameters
Differences in parameters, such as pH, CEC, moisture, and temperature, were found in soils of lemon trees across treatments. The pH of soils treated with fabric mulch ground covers was significantly greater (23% higher) compared to those without fabric mulch ground covers (Figure 5A). The CEC of soils without fabric mulch ground covers (7.2 meq/100 g) was not significantly different from the one with ground covers (Figure 5B). Soils of lemon trees treated with fabric mulch ground covers had greater moisture at 12:00 PM and 5:00 PM compared to those grown without fabric mulch ground covers (Figure 6A). Soils of lemon trees grown with fabric mulch ground covers exhibited greater temperatures at all 3 measured timepoints of the day (8:00 AM, 12:00 PM, and 5:00 PM) compared to soils in uncovered trees. However, these differences were only significant between treatments at 5:00 PM (Figure 6B).
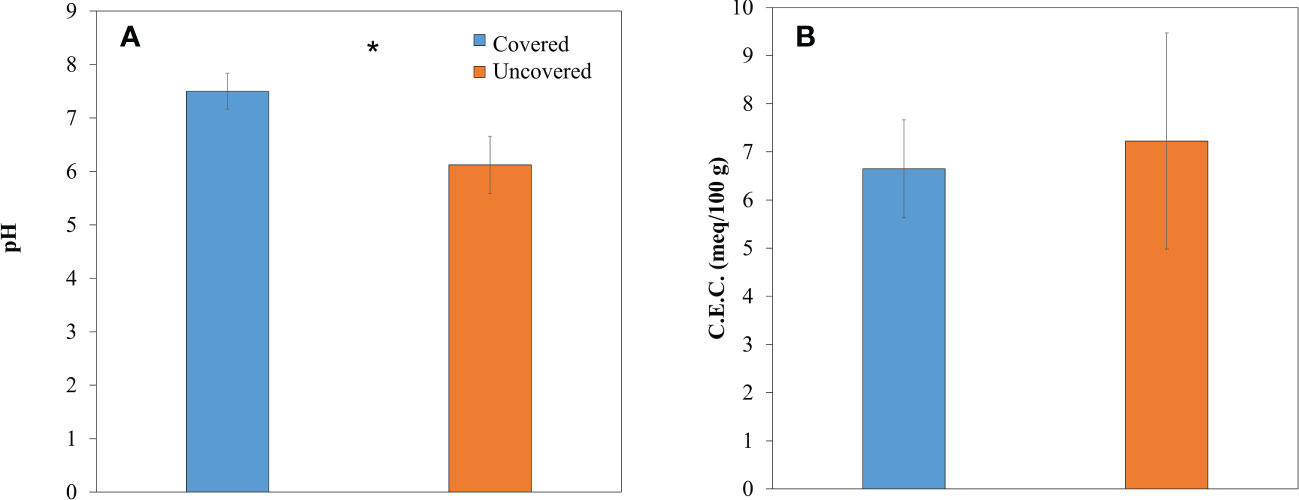
Figure 5 pH (A) and cation exchange capacity (B) in soils of 4-year-old lemon trees planted in flatwood soils located in the Florida, USA and grown with or without fabric mulch ground covers. Data were collected in June 2021. Bars are ± standard deviation of the mean. Treatments with * were considered significantly different (p<0.05).
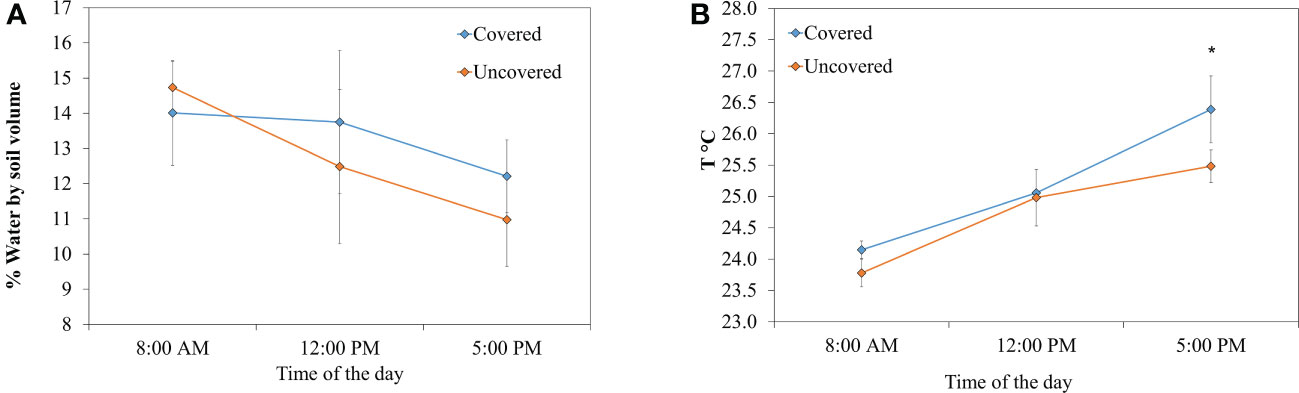
Figure 6 Moisture (A) and temperature (B) in soils of 4-year-old lemon trees planted in flatwood soils located in the Florida, USA and grown with or without fabric mulch ground covers. Measurements were taken three times (8:00 AM, 12:00 PM and 5:00 PM). No significant differences were reported for soil moisture. Significant differences were found in soil temperatures between covered and uncovered treatments at 5:00 PM (p=0.049; F = 6.939). Bars are ± standard deviation of the mean. Treatments with * were considered significantly different (p<0.05).
Impact of fabric mulch ground covers on rhizosphere bacterial communities
Data was log-transformed to reduce error rates caused by rarefaction, and later utilized for alpha and beta diversity analyses of the rhizosphere bacterial community through use of the R package “Phyloseq” v1.24.0 (38). Rhizosphere bacterial alpha diversity did vary according to treatments according to the Shannon index (Figure 7A). Lemon trees treated with fabric mulch ground covers had a lower bacterial alpha diversity compared to trees grown without fabric mulch ground covers (Figure 7A). However, there were no statistically significant results.
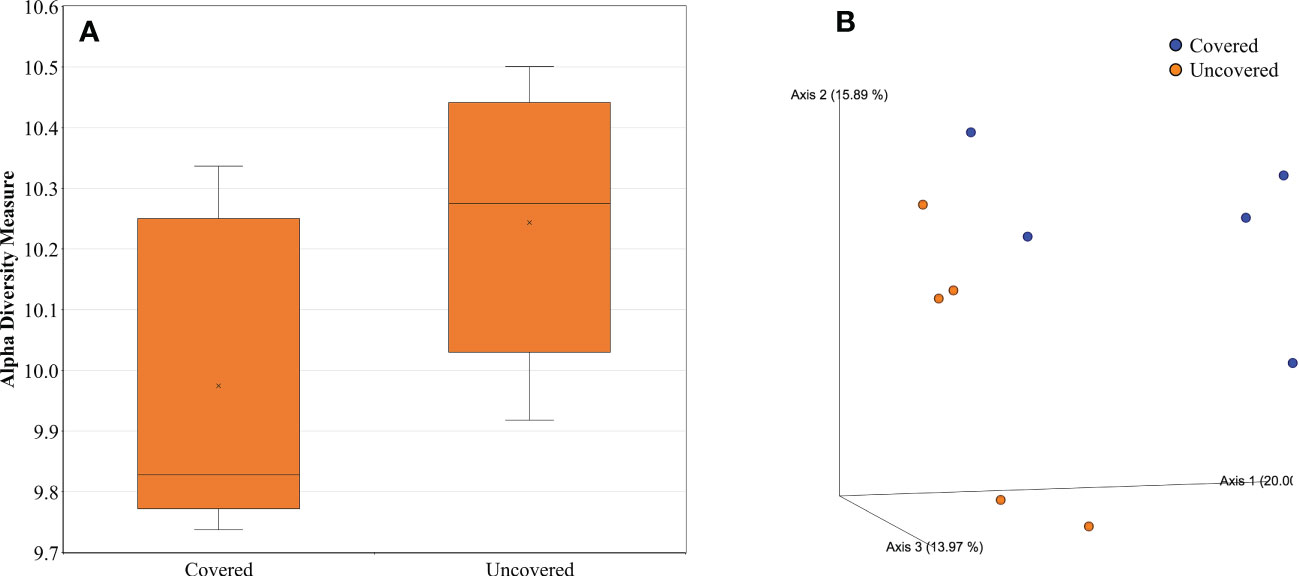
Figure 7 Alpha (A) and Beta Diversity (B) of rhizosphere bacteria of 4-year-old lemon trees planted in flatwood soils located in the Florida, USA and grown with or without fabric mulch ground covers. Alpha-diversity was measured by Shannon index of rhizosphere bacteria among treatments. Plotted in figure A are boxes, (interquartile), median (line within each box), and whiskers (lowest and greatest values). A principal coordinates analysis (PCOA) based on Bray-Curtis dissimilarity matrix of rhizosphere bacterial samples can be found in figure B, where colors indicate treatment and include covered (blue) and uncovered (orange). Symbol “x” represents the mean of the values.
Beta diversity analyses included principal coordinate analysis (PCoA) on Bray–Curtis distances (Figure 7B). A PERMANOVA test was performed to determine significant differences in beta diversity between treatments (39). Rhizosphere bacterial beta diversity was significantly impacted by treatment (Figure 7B). Rhizosphere bacterial beta diversity of lemon trees treated with fabric mulch ground covers was significantly greater (p value < 0.05) compared to those grown without fabric mulch ground covers (Figure 7B). Furthermore, Bray-Curtis distances had a total explained variance of 49.86% when estimating beta diversity between treatments (Figure 7B).
Among the orders of bacteria in the lemon rhizosphere, those treated with fabric mulch ground covers consisted of a greater relative frequency of Burkolderiales, Solirubrobacterales, Gemmatimonadales, Chitinophalages, and Bacillales compared to those treated without fabric mulch ground covers. Conversely, lemon trees treated without fabric mulch ground covers had a greater relative frequency of Rhizobiales, Vicinamibacterales, Gaiellales, Gemmatales, and Pirelluales. Burkolderiales was the most frequent order of bacteria found in the rhizosphere of lemon trees treated with fabric mulch ground covers, whereas Rhizobiales was found to be most frequent in the lemon trees grown without fabric mulch ground covers (Table 1). There were no significant differences in the relative frequency of bacterial orders in response to fabric mulch ground cover use.
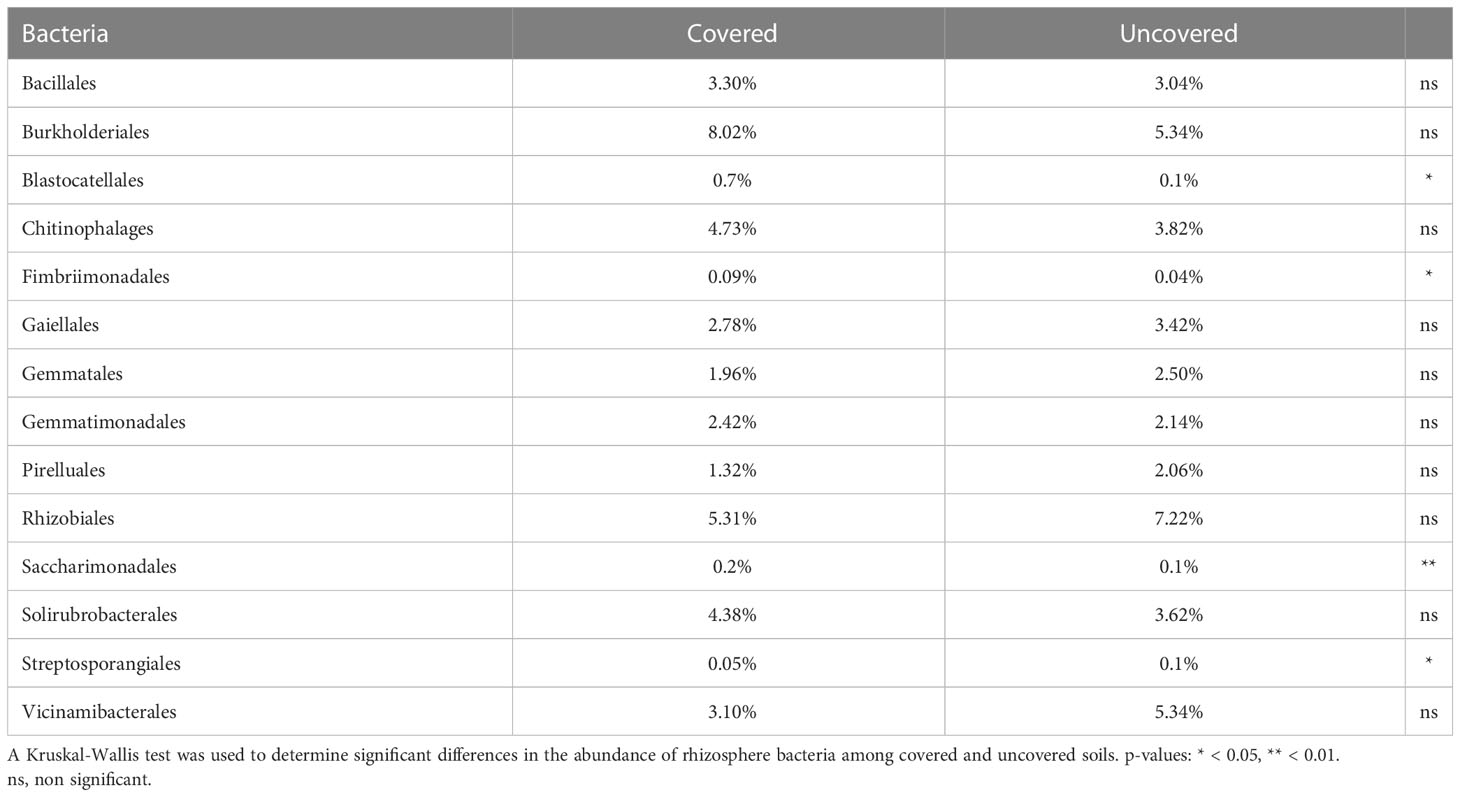
Table 1 Relative frequency of bacterial orders in the rhizosphere of 4-year-old lemon trees grown in Florida, USA and treated with fabric mulch ground covers and without fabric mulch ground covers.
There was variation in the relative abundance of bacterial taxonomic orders among treatments. Lemon trees from the fabric mulch ground cover treatment had a rhizosphere bacterial community with a significantly greater abundance of Saccharimonadales (p < 0.01), Fimbriimonadales (p < 0.05), and Blastocatellales (p < 0.05). Conversely, a significantly greater relative abundance of Streptosporangiales was found in the rhizosphere of lemon grown without fabric mulch ground covers (p < 0.05) (Table 1).
The CCA was utilized to examine the impact of soil and root parameters and soil nutrient concentrations on rhizosphere bacterial communities (Figure 8). The length of each arrow corresponds to the relative importance of each environmental parameter in explaining the variation in rhizosphere bacterial profiles. An analysis of similarity (ANOSIM) determined that Ct value, and soil pH and phosphorus were significantly correlated (p < 0.05) with the lemon rhizosphere bacterial communities between treatments (Figure 8).
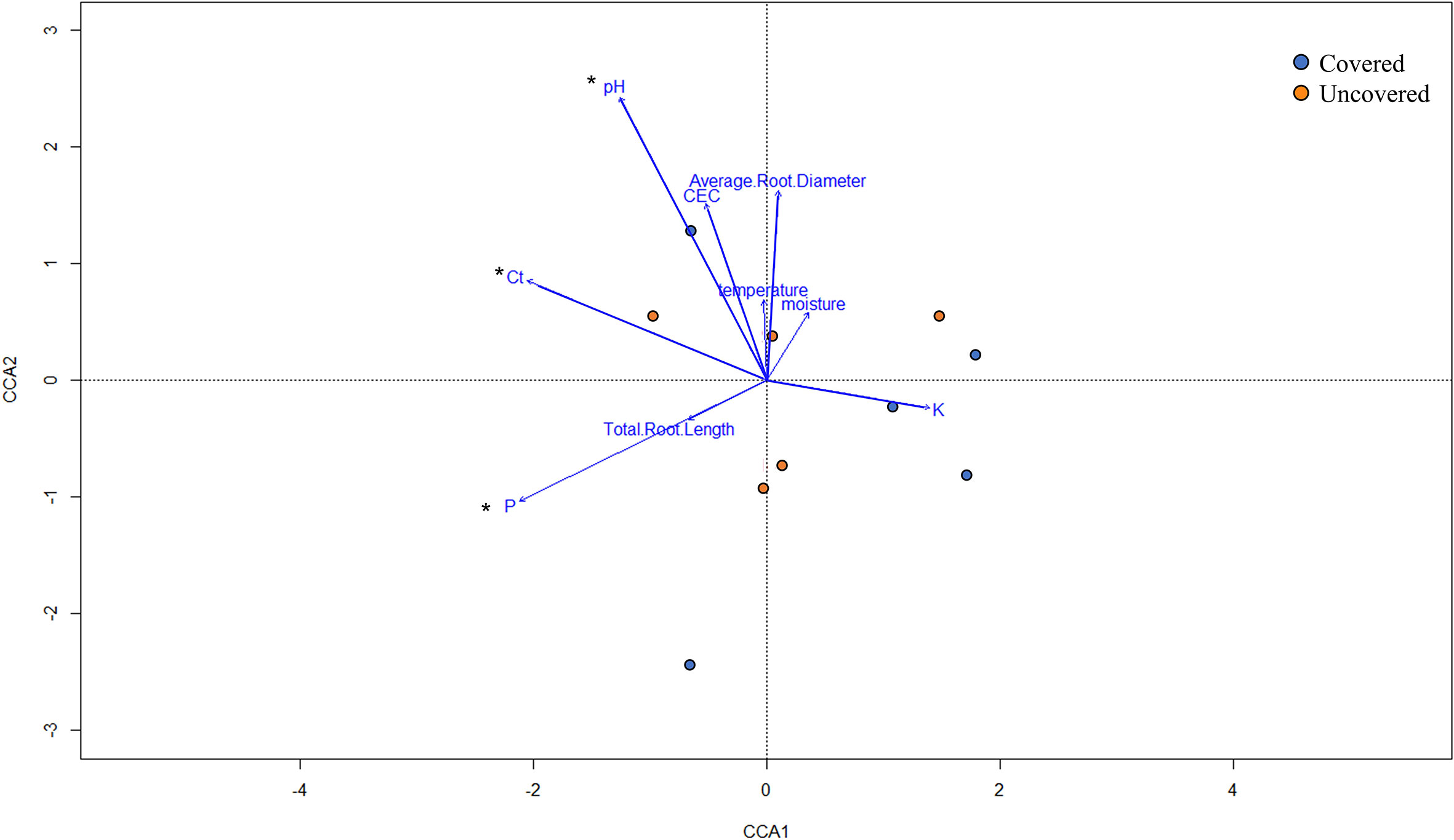
Figure 8 Canonical correspondence analysis (CCA) of rhizosphere bacterial communities of 4-year-old lemon trees planted in flatwood soils located in the Florida, USA and grown with or without fabric mulch ground covers. Each dot represents the rhizosphere bacterial community within a sample, while the colors indicate treatment (trees grown with fabric mulch ground covers in blue and trees grown without fabric mulch ground covers in orange). Measured parameters include phosphorus (P), potassium (K), cation exchange capacity (CEC), HLB bacterial titer (Ct), soil temperature, soil moisture, soil pH, total root length, and average root diameter. The * symbol indicates parameters considered significantly different (p<0.05).
Discussion
Reduced lateral movement of water through the soil profile during periods of rainfall and irrigation from fabric mulch ground cover use may have contributed towards significantly greater Mn and Zn concentrations in soils of lemon trees (Figure 2D). Similar to the results of this study, even if conducted on a different soil type, Simpson et al. (8) found that soils treated with fabric mulch ground covers had reduced rates of leaching and increased water availability, facilitating the availability of nutrients in the root zone. The greater Zn concentrations found in soils with fabric mulch ground cover use may have also contributed to the significantly greater lemon rhizosphere bacterial beta diversity of trees (Figure 7B). For instance, Zn has been shown to exhibit host–pathogen interactions, as increased concentrations have been shown to suppress the growth of potential phytopathogens, thus promoting soil and plant health (i.e., more diverse rhizosphere community composition) (40).
When examining the microorganisms that reside in the rhizosphere, both composition and diversity function as indicators of plant health, including nutrient acquisition and cycling (41). Additionally, rhizosphere microbial communities are sensitive to changes in the plant host and soil environment (42). In the case of this study, variability in the soil parameters, such as pH (Figure 5A), moisture (Figure 6A), and temperature (Figure 6B) across treatments may have influenced lemon tree rhizosphere community composition (Figure 7B). Increased water availability provided by fabric mulch ground covers can be linked with the significantly greater rhizosphere bacterial beta diversity in lemon trees. Previous research applied towards the impacts of water availability on soil microorganisms have brought further emphasis on the sensitivity of these communities. One study conducted by Xu et al. (43) found that lack of soil moisture during periods of drought stress had a negative correlation with the diversity of sorghum rhizosphere bacterial communities. Furthermore, both Naylor et al. (44) and Santos-Medellín et al. (45) found that lack of soil moisture altered the rhizosphere bacterial community composition in both other crop species. Additionally, soil moisture has also been shown to have subsequent effects on carbon input and rates of decomposition related to organic matter, thus potentially affecting plant growth (46).
Increases in nightly soil temperatures (5:00 PM) from fabric mulch ground cover use may have contributed towards significantly greater rhizosphere bacterial beta diversity found in lemon trees (Figure 6B). Similar to our results, Lin et al. (47) found that bamboo rhizosphere communities that were subject to greater temperatures resulted in significantly more diverse bacterial communities. Temperature is commonly a limiting factor associated with soil microorganism processes, such as respiration and enzyme activity, affecting overall community composition (48). Additionally, variation in temperatures can have implications on soil carbon and nitrogen cycles, potentially affecting overall crop health and yield (49).
The significant more diverse rhizosphere bacterial community composition found in lemon trees treated with fabric mulch ground covers can also be attributed to significant differences in pH found among treatments (Figure 5A). Similar to the results of our study, Wan et al. (50) found that citrus rhizosphere community composition was predominately influenced by shifts in soil pH. Soil pH serves as a notable indicator of rhizosphere characteristics, such as abundance and diversity (51) and explains more than 69% of the variation associated with species diversity (52).
The CCA suggests that a strong relationship was shared among several environmental parameters and rhizosphere bacterial communities among treatments (Figure 8). Notably, soil pH significantly correlated with lemon rhizosphere bacterial communities, which further emphasizes the impact soil pH may have had on community structure and composition (Figure 8). Lopes et al. (15) reported on the effects of soil pH on rhizosphere and root endosphere microbiomes of plants growing in a Sandhills ecosystem. In addition, a study conducted by Mandakovic et al. (53) found that soil bacterial diversity was correlated to pH, plateauing at near-neutral conditions, and declining as pH shifted towards more acidic and alkaline conditions. Similarly, in our study, the rhizosphere bacterial communities residing in soils with a near-neutral pH (7.5) in the fabric mulch ground cover treatment resulted in a significantly more diverse community composition compared to those in slightly acidic soils (pH 6.1) of uncovered lemon trees (Figure 5A). The differences in soil pH between the two treatments can be attributed to the ground water supply that was used for irrigation. The water was tested and found to have an alkaline pH (8.25). The alkaline irrigation water, in addition to the reduced vertical flow of water due to the fabric mulch ground cover, and the increased retention of nutrients, lead to an increase of the soil pH, which translated in an increased diversity of the microbial communities. Additionally, the CCA infers that rhizosphere bacterial communities were also significantly correlated with Ct value (Figure 8). An earlier study conducted by Padhi et al. (54) utilized a correlation analysis and revealed several significant correlations between HLB bacterial titers (Ct value) in leaf tissue and lemon rhizosphere microbial communities, specifically with bacteria on different taxonomic levels, which included Betaproteobacteria and Solibacteres, Methylocystaceae, and Burkholderia. Interestingly, although there were no significant differences in soil P concentrations between treatments, there was a significant correlation present between the lemon rhizosphere bacterial communities across treatments and soil phosphorus (Figure 8). Other studies have shown that P availability does exhibit a strong relationship with rhizosphere community composition (55). For instance, increases in soil P resulted in greater fibrous root biomass, subsequently improving C availability to soil microbes, soil microbial biomass, and rhizosphere community composition (56). Further analysis into the interaction shared between the soil conditions of fabric mulch ground covers, nutrient availability, and rhizosphere community composition may have proven useful in optimizing management strategies for lemon production.
Conclusion
In this study we examined the impact of fabric mulch ground covers on both soil and plant parameters, including rhizosphere community composition. Presence of fabric mulch ground covers resulted in significantly greater soil Zn, soil Mn, soil temperatures (specifically evening temperatures) and pH, potentially contributing towards the significant more diverse rhizosphere bacterial community composition compared than those grown without fabric mulch ground covers. Correlations between nutrient concentrations, soil parameters (temperature and pH), bacterial community composition, and plant growth parameters suggest the possibility for future opportunities regarding the manipulation of bacteria within rhizosphere to assist plant-microbe nutritional status and interactions. Future experimentation and samplings are required to provide further insight on the interactions shared among the lemon tree rhizosphere bacterial community composition, seasonality, environmental parameters, and fabric mulch ground cover use.
Data availability statement
The datasets presented in this study can be found in online repositories. The names of the repository/repositories and accession number(s) can be found below: BioProject, PRJNA913113.
Author contributions
JS contributed to the conceptualization, methodology, data analysis, and writing the original draft. J-PF contributed to the sample collection, methodology, and writing, review and editing. SG contributed to the conceptualization and writing, review and editing. LR contributed to conceptualization, supervision, methodology, resources, writing-review and editing, and overall project administration. All authors contributed to manuscript revision, read, and approved the submitted version.
Funding
This work was supported by the U.S. Department of Agriculture National Institute of Food and Agriculture, Hatch project #FLA-IRC-005743.
Acknowledgments
The authors are grateful to Mr. Daniel Scott for providing use of his commercial citrus grove for the execution of the study. The authors would also like to thank Rush University for their services in processing and analyzing the microbiome samples.
Conflict of interest
The authors declare that the research was conducted in the absence of any commercial or financial relationships that could be construed as a potential conflict of interest.
Publisher’s note
All claims expressed in this article are solely those of the authors and do not necessarily represent those of their affiliated organizations, or those of the publisher, the editors and the reviewers. Any product that may be evaluated in this article, or claim that may be made by its manufacturer, is not guaranteed or endorsed by the publisher.
References
1. Bové JM. Huanglongbing: a destructive, newly-emerging, century-old disease of citrus. J Plant Pathol (2006) 88:7–37. https://www.jstor.org/stable/41998278
2. Bové JM. Huanglongbing or yellow shoot, a disease of gondwanan origin: Will it destroy citrus worldwide? Phytoparasitica (2014) 42:579–83. doi: 10.1007/s12600-014-0415-4
3. Halbert SE, Manjunath K, Ramadugu C, Lee RF. Incidence of huanglongbing-associated ‘Candidatus liberibacter asiaticus’ in diaphorina citri (Hemiptera: Psyllidae) collected from plants for sale in Florida. Florida Entomologist (2012) 95:617–24. doi: 10.1653/024.095.0312
4. Rugno GR, Cuervo JGB, Garcia AG, Qureshi J, Yamamoto PT. Abundance and diversity of lacewings in grower operated organic and conventional pest management programs for diaphorina citri (Hemiptera: Liviidae). Crop Prot (2021) 146:105682. doi: 10.1016/j.cropro.2021.105682
5. Stover E, Hall DG, Shatters RG, Moore GA. Influence of citrus source and test genotypes on inoculations with candidatus liberibacter asiaticus. HortScience (2016) 51:805. doi: 10.21273/HORTSCI.51.7.805
6. Stover E, Inch S, Richardson ML, Hall DG. Conventional citrus of some scion/rootstock combinations show field tolerance under high huanglongbing disease pressure. HortScience (2016) 51:127–32. doi: 10.21273/HORTSCI.51.2.127
7. Simpson CR, Melgar JC, Nelson SD, Sétamou M. Growth and yield responses under different grove floor management strategies for water conservation in young grapefruit trees. Scientia Hortic (2019) 256:108567. doi: 10.1016/j.scienta.2019.108567
8. Simpson CR, Gonzales J, Enciso J, Nelson SD, Sétamou M. Root distribution and seasonal fluctuations under different grove floor management systems in citrus. Scientia Hortic (2020) 272:109364. doi: 10.1016/j.scienta.2020.109364
9. Wang L, Li X. Steering soil microbiome to enhance soil system resilience. Crit Rev Microbiol (2019) 45:743–53. doi: 10.1080/1040841X.2019.1700906
10. Kasirajan S, Ngouajio M. Polyethylene and biodegradable mulches for agricultural applications: a review. Agron Sustain Dev (2012) 32:501–29. doi: 10.1007/s13593-011-0068-3
11. Schlautman B, Bartel C, Diaz-Garcia L, Fei S, Flynn S, Haramoto E, et al. Perennial groundcovers: an emerging technology for soil conservation and the sustainable intensification of agriculture. Emerg Top Life Sci (2021) 5:337–47. doi: 10.1042/ETLS20200318
12. Kadyampakeni DM, Chinyukwi T. Are macronutrients and micronutrients therapeutic for restoring performance of trees affected by citrus greening? a discussion of current practices and future research opportunit ies. J Plant Nutr (2022) 44:2949–69. doi: 10.1080/01904167.2021.1927079
13. Hasanuzzaman M, Bhuyan M, Nahar K, Hossain M, Mahmud J, Hossen M, et al. Potassium: A vital regulator of plant responses and tolerance to abiotic stresses. Agronomy (2018) 8:31. doi: 10.3390/agronomy8030031
14. Palmer CM, Guerinot ML. Facing the challenges of Cu, fe and zn homeostasis in plants. Nat Chem Biol (2009) 5:333–40. doi: 10.1038/nchembio.166
15. Lopes LD, Hao J, Schachtman DP. Alkaline soil pH affects bulk soil, rhizosphere and root endosphere microbiomes of plants growing in a sandhills ecosystem. FEMS Microbiol Ecol (2021) 97:1–14. doi: 10.1093/femsec/fiab028
16. Schlaeppi K, Bulgarelli D. The plant microbiome at work. Mol Plant-Microbe Interactions® (2015) 28:212–7. doi: 10.1094/MPMI-10-14-0334-FI
17. Vandenkoornhuyse P, Quaiser A, Duhamel M, Le Van A, Dufresne A. The importance of the microbiome of the plant holobiont. New Phytol (2015) 206:1196–206. doi: 10.1111/nph.13312
18. Blaustein RA, Lorca GL, Meyer JL, Gonzalez CF, Teplitski M. Defining the core citrus leaf- and root-associated microbiota: Factors associated with community structure and implications for managing huanglongbing (Citrus greening) disease. Appl Environ Microbiol (2017) 83:e00210–17. doi: 10.1128/AEM.00210-17
19. Bhattacharyya PN, Jha DK. Plant growth-promoting rhizobacteria (PGPR): emergence in agriculture. World J Microbiol Biotechnol (2012) 28:1327–50. doi: 10.1007/s11274-011-0979-9
20. Backer R, Rokem JS, Ilangumaran G, Lamont J, Praslickova D, Ricci E, et al. Plant growth-promoting rhizobacteria: Context, mechanisms of action, and roadmap to commercialization of biostimulants for sustainable agriculture. Front Plant Sci (2018) 9. doi: 10.3389/fpls.2018.01473
21. Roberts EL. Chapter 1 - plant growth promotion by rhizosphere dwelling microbes. In: Dubey RC, Kumar P, editors. Rhizosphere engineering. Cambridge, Massachusetts, USA: Academic Press (2022).
22. Trivedi P, He Z, Van Nostrand JD, Albrigo G, Zhou J, Wang N. Huanglongbing alters the structure and functional diversity of microbial communities associated with citrus rhizosphere. ISME J (2012) 6:363–83. doi: 10.1038/ismej.2011.100
23. Riera N, Handique U, Zhang Y, Dewdney MM, Wang N. Characterization of antimicrobial-producing beneficial bacteria isolated from huanglongbing escape citrus trees. Front Microbiol (2017) 8. doi: 10.3389/fmicb.2017.02415
24. Zhang Y, Xu J, Riera N, Jin T, Li J, Wang N. Huanglongbing impairs the rhizosphere-to-rhizoplane enrichment process of the citrus root-associated microbiome. Microbiome (2017) 5:97. doi: 10.1186/s40168-017-0304-4
25. Zhang Y, Trivedi P, Xu J, Roper MC, Wang N. The citrus microbiome: From structure and function to microbiome engineering and beyond. Phytobiomes J (2021) 5:249–62. doi: 10.1094/PBIOMES-11-20-0084-RVW
26. Trivedi P, Spann T, Wang N. Isolation and characterization of beneficial bacteria associated with citrus roots in Florida. Microbial. Ecol (2011) 62:324–36. doi: 10.1007/s00248-011-9822-y
27. Munir S, He P, Wu Y, He P, Khan S, Huang M, et al. Huanglongbing control: Perhaps the end of the beginning. Microbial. Ecol (2018) 76:192–204. doi: 10.1007/s00248-017-1123-7
28. Bhatta A, Prasad R, Chakraborty D, Shaw JN, Lamba J, Brantley E, et al. Mehlich 3 as a generic soil test extractant for environmental phosphorus risk assessment across Alabama soil regions. Agrosystems Geosci. Environ (2021) 4:e20187. doi: 10.1002/agg2.20187
29. Kadyampakeni DM, Morgan KT, Schumann AW, Nkedi-Kizza P, Mahmoud K. Ammonium and nitrate distribution in soil using drip and microsprinkler irrigation for citrus production. Soil Sci Soc America J (2014) 78:645–54. doi: 10.2136/sssaj2013.07.0319
30. Isaac RA, Johnson WC. Elemental analysis of plant tissue by plasma emission spectroscopy: Collaborative study. J Assoc Off Analytical Chem (2020) 68:499–505. doi: 10.1093/jaoac/68.3.499
31. Wang Z, Yin Y, Hu H, Yuan Q, Peng G, Xia Y. Development and application of molecular-based diagnosis for ‘Candidatus liberibacter asiaticus’, the causal pathogen of citrus huanglongbing. Plant Pathol (2006) 55:630–8. doi: 10.1111/j.1365-3059.2006.01438.x
32. Trivedi P, Sagaram US, Kim J-S, Brlansky RH, Rogers ME, Stelinski LL, et al. Quantification of viable candidatus liberibacter asiaticus in hosts using quantitative PCR with the aid of ethidium monoazide (EMA). Eur J Plant Pathol (2009) 124:553–63. doi: 10.1007/s10658-009-9439-x
33. McCollum G, Hilf M, Irey M. Relationship between ct values, HLB symptoms and CLas titer. J Cit Pathol (2014) 1:97. doi: 10.5070/C411024727
34. Callahan BJ, Mcmurdie PJ, Rosen MJ, Han AW, Johnson AJA, Holmes SP. DADA2: High-resolution sample inference from illumina amplicon data. Nat Methods (2016) 13:581–3. doi: 10.1038/nmeth.3869
35. Caporaso JG, Kuczynski J, Stombaugh J, Bittinger K, Bushman FD, Costello EK, et al. QIIME allows analysis of high-throughput community sequencing data. Nat Methods (2010) 7:335–6. doi: 10.1038/nmeth.f.303
36. Thompson LR, Sanders JG, Mcdonald D, Amir A, Ladau J, Locey KJ, et al. A communal catalogue reveals earth’s multiscale microbial diversity. Nature (2017) 551:457–63. doi: 10.1038/nature24621
37. McMurdie PJ, Holmes S. Waste not, want not: Why rarefying microbiome data is inadmissible. PloS Comput Biol (2014) 10:e1003531. doi: 10.1371/journal.pcbi.1003531
38. McMurdie PJ, Holmes S. Phyloseq: An r package for reproducible interactive analysis and graphics of microbiome census data. PloS One (2013) 8:e61217. doi: 10.1371/journal.pone.0061217
39. Tang Z-Z, Chen G, Alekseyenko AV. PERMANOVA-s: association test for microbial community composition that accommodates confounders and multiple distances. Bioinformatics (2016) 32:2618–25. doi: 10.1093/bioinformatics/btw311
40. Wu D, Ma Y, Yang T, Gao G, Wang D, Guo X, et al. Phosphorus and zinc are strongly associated with belowground fungal communities in wheat field under long-term fertilization. Microbiol Spectr (2022) 10:e00110–22. doi: 10.1128/spectrum.00110-22
41. Ling N, Wang T, Kuzyakov Y. Rhizosphere bacteriome structure and functions. Nat Commun (2022) 13:836. doi: 10.1038/s41467-022-28448-9
42. Pathan SI, Ceccherini MT, Sunseri F, Lupini A. Rhizosphere as hotspot for plant-Soil-Microbe interaction. In: Datta R, Meena RS, Pathan SI, Ceccherini MT, editors. Carbon and nitrogen cycling in soil. Singapore: Springer Singapore (2020).
43. Xu J, Zhang Y, Zhang P, Trivedi P, Riera N, Wang Y, et al. The structure and function of the global citrus rhizosphere microbiome. Nat Commun (2018) 9:4894. doi: 10.1038/s41467-018-07343-2
44. Naylor D, Degraaf S, Purdom E, Coleman-Derr D. Drought and host selection influence bacterial community dynamics in the grass root microbiome. ISME J (2017) 11:2691–704. doi: 10.1038/ismej.2017.118
45. Santos-Medellín C, Edwards J, Liechty Z, Nguyen B, Sundaresan V. Drought stress results in a compartment-specific restructuring of the rice root-associated microbiomes. mBio (2017) 8:e00764–17. doi: 10.1128/mBio.00764-17
46. Olatunji OA, Luo H, Pan K, Tariq A, Sun X, Chen W, et al. Influence of phosphorus application and water deficit on the soil microbiota of N2-fixing and non-n-fixing tree. Ecosphere (2018) 9:e02276. doi: 10.1002/ecs2.2276
47. Lin YT, Jia Z, Wang D, Chiu CY. Effects of temperature on the composition and diversity of bacterial communities in bamboo soils at different elevations. Biogeosciences (2017) 14:4879–89. doi: 10.5194/bg-14-4879-2017
48. Liu Y, He N, Wen X, Xu L, Sun X, Yu G, et al. The optimum temperature of soil microbial respiration: Patterns and controls. Soil Biol Biochem (2018) 121:35–42. doi: 10.1016/j.soilbio.2018.02.019
49. Li Z, Tian D, Wang B, Wang J, Wang S, Chen HYH, et al. Microbes drive global soil nitrogen mineralization and availability. Global Change Biol (2019) 25:1078–88. doi: 10.1111/gcb.14557
50. Wan Y, Li W, Wang J, Shi X. Bacterial diversity and community in response to long-term nitrogen fertilization gradient in citrus orchard soils. Diversity (2021) 13:282. doi: 10.3390/d13070282
51. Zeng Q, An S, Liu Y, Wang H, Wang Y. Biogeography and the driving factors affecting forest soil bacteria in an arid area. Sci Total Environ (2019) 680:124–31. doi: 10.1016/j.scitotenv.2019.04.184
52. Fierer N, Jackson RB. The diversity and biogeography of soil bacterial communities. Proc Natl Acad Sci U.S.A. (2006) 103:626–31. doi: 10.1073/pnas.0507535103
53. Mandakovic D, Maldonado J, Pulgar R, Cabrera P, Gaete A, Urtuvia V, et al. Microbiome analysis and bacterial isolation from lejía lake soil in atacama desert. Extremophiles (2018) 22:665–73. doi: 10.1007/s00792-018-1027-6
54. Padhi EMT, Maharaj N, Lin S-Y, Mishchuk DO, Chin E, Godfrey K, et al. Metabolome and microbiome signatures in the roots of citrus affected by huanglongbing. Phytopathology® (2019) 109:2022–32. doi: 10.1094/PHYTO-03-19-0103-R
55. Li J, Li Z, Wang F, Zou B, Chen Y, Zhao J, et al. Effects of nitrogen and phosphorus addition on soil microbial community in a secondary tropical forest of China. Biol Fertility Soils (2015) 51:207–15. doi: 10.1007/s00374-014-0964-1
Keywords: Citrus limon, microbiome, soil health, groundcovers, flatwoods
Citation: Santiago JM, Fox J-P, Guzmán SM and Rossi L (2023) Effect of fabric mulch ground covers on lemon trees rhizosphere microbiome in Florida flatwood soils. Front. Soil Sci. 3:1110370. doi: 10.3389/fsoil.2023.1110370
Received: 28 November 2022; Accepted: 08 February 2023;
Published: 22 February 2023.
Edited by:
Agnieszka Maria Sekara, University of Agriculture in Krakow, PolandReviewed by:
Sara Ondoño, Spanish National Research Council (CSIC), SpainMarketa Sagova-Mareckova, Crop Research Institute (CRI), Czechia
Copyright © 2023 Santiago, Fox, Guzmán and Rossi. This is an open-access article distributed under the terms of the Creative Commons Attribution License (CC BY). The use, distribution or reproduction in other forums is permitted, provided the original author(s) and the copyright owner(s) are credited and that the original publication in this journal is cited, in accordance with accepted academic practice. No use, distribution or reproduction is permitted which does not comply with these terms.
*Correspondence: Lorenzo Rossi, l.rossi@ufl.edu