- 1Institute of Geography, Johannes Gutenberg-University, Mainz, Germany
- 2Soil Science and Soil Protection, Martin Luther University Halle-Wittenberg, Halle (Saale), Germany
- 3Institute of Environmental Sciences and Geography, University of Potsdam, Potsdam, Germany
Cadaver-decomposition unleashes an ephemeral pulse of matter input that modifies microbial communities, as well as nutrient pools and fluxes. This leaves behind a measurable imprint on affected soils. However, the persistence of this imprint remains poorly understood. We define cadaver imprint persistence as the entire period between time of cadaver deposition and time when cadaver effects on microbial community structure and chemical indicators are no longer detectable. We present a brief overview of published results on the cadaver-induced changes in the bio-elements carbon, nitrogen and phosphorus, which regulate the structure and functions of the soil microbiome. Based on this, we identified conceptual and methodological gaps and biases and suggest potential research avenues to address them. This will help to better understand the relationships between cadaver-derived matter and microbial taxa and functions, as well as the role of cadaver-decomposition within and across ecosystems. The proposed future research on cadaver-derived imprint on soils has the potential to serve as a hub for connecting soil chemistry, microbial ecology, forensic sciences, and ecosystems science.
1 Introduction
After death, the remains of all organisms undergo decomposition. Decomposition of dead material is central to the functioning of all ecosystems and crucial to the redistribution of nutrients and energy (1). It unleashes an ephemeral pulse of matter input that modifies microbial communities as well as nutrient pools and fluxes, leaving behind a measurable imprint on the affected soil (2). The imprint is influenced by a complex set of factors, with interactions between soil microbes and the fluxes of matter and nutrients released by dead material decomposition playing a central, yet poorly understood role (3).
Research on decomposing dead material is predominately focused on plant litter. By contrast, the decay of cadavers has received far less attention. This might be mainly due to animal-derived biomass representing only 1% of the total organic material exposed to decomposition in terrestrial ecosystems (4). Research on cadaver decomposition mainly addresses two issues: (i) successional patterns of different cadaver-related organisms in various taxa, such as bacteria or protists, especially in order to determine the post-mortem interval (5, 6) and (ii) spatial patterns of energy and nutrient flow within and among ecosystems (7–9). Studies targeting spatial patterns have highlighted the key role of cadaver decomposition for shaping environmental heterogeneity (10), but provided no information about the mechanisms involved. Studies of temporal changes in soil chemistry (9, 11, 12) and/or microbial communities in response to cadaver decomposition revealed different decomposition stages (13–17), each characterized by specific microbial taxa (18, 19). Typically, these studies were restricted to determining the imprint of cadaver decomposition on soil chemical and microbial features but payed little attention to the functional linkages between microbes and matter and nutrient fluxes. As a consequence, little is known about the cycling (including translocation, transformation, and immobilisation) of matter that enters the soil during cadaver deposition. Similarly, the contribution of specific microbial taxa and functional groups to processes induced by the input of cadaver-derived matter (e.g., breaking down and transforming dead organic material) remains poorly understood (3, 20). In addition, different cadaver types and weights have been studied – ranging from mice (20) to elephants (15), which influences the extent and persistence of the cadaver imprint on soil and makes comparisons among studies difficult. Studies have also considered different time scales – from two weeks (21) to several years (22). Studies based on the same cadaver type and weight and lasting for several years are rare. The resulting knowledge gaps limit our capability of predicting the impact of cadaver decomposition on soil ecosystems, and thus, hamper the deduction of precise timescales of the individual decomposition stages (23–25) as well as of the extent and persistence of cadaver imprint on soil.
We aim at identifying the key issues impairing the study of cadaver imprint on soil ecosystems for better insight into the functional linkages between microbes and soil matter cycling. We address the potential persistence of changes in carbon (C), nitrogen (N), phosphorus (P), bio-elements that regulate structure and functioning of the soil microbiome (cell compounds, biomass production, activity, energy transfer; Figure 1). We define the persistence of cadaver imprint on soil as the entire period between time of cadaver deposition on soil and time when cadaver effects on microbial community structure and chemical indicators are no longer detectable. Cadaver imprint persistence of 3 (22) to 10 years (11) were already observed, which clearly exceeds the typical duration of most experiments (<1 (26) to 2 years (27)).
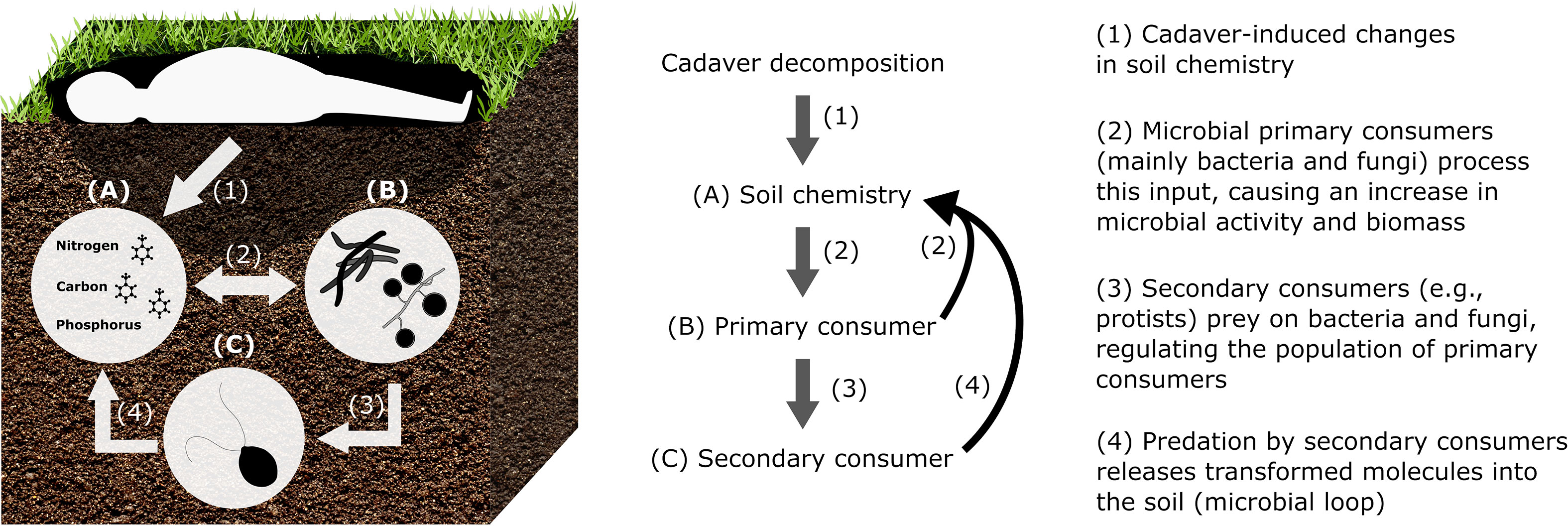
Figure 1 Simplified scheme of cadaver imprint on soil, including coupled chemical-microbial processes. (A) Cadaver-induced nutrient and matter changes soil chemistry. (B) Microbial primary consumers (mainly bacteria and fungi) process the cadaver-derived input and subsequent increase in microbial activity and biomass. (C) Secondary consumers (e.g., protists) prey on bacteria and fungi, and thus, regulate the population of primary consumers and the release of transformed molecules into the soil. Please note, temporal and spatial facets are not considered, since largely unknown. Furthermore, the rest of the food chain including plants, meso- and macro-fauna is not shown since we focus on soil chemistry and microbes.
We start with a brief summary of the current state of knowledge on temporal changes in soil induced by cadaver decomposition, followed by a list of identified major research issues and their possible relevance for the understanding cadaver-impacted soils. Finally, we propose potential research avenues to overcome these issues.
2 Imprint of cadaver-derived C, N, and P in soil
Cadavers are point sources of bio-elements, exposing the soil underneath to high inputs. These inputs can cause locally elevated concentrations of elements, but their persistence is poorly known and likely varies by element (28, 29). The amount of elemental release and input into soil is a function of cadaver weight (30) and decomposition stage (12). The persistence of elemental changes varies with soil properties affecting their transport and retention (31, 32).
The biochemical composition of cadavers resembles that of major degraders. The stoichiometries of major bio-elements is similar (33). This promotes rapid utilisation of cadavers for biomass growth of degraders, such as bacteria and other microbial taxa, with the extent being modified by environmental conditions controlling the balance of biomass built-up and mineralisation.
The potential imprint of cadaver-derived C depends on soil conditions supporting the long-term stabilisation of microbial metabolites and necromass (34). In contrast to plants, cadavers contain much of the C in compounds prone to simple enzymatic breakdown, such as lipids, sugar derivatives, and protein (13). Consequently, cadaver fluids are rapidly consumed by microorganisms upon entering the topsoil, with only small portions leached into deeper soil layers (35). Efficient C use by microorganisms results in production of metabolites and built up of microbial biomass (36). In soils containing reactive minerals (three-layer clay minerals, hydrous oxides of aluminum and iron), part of the microbial metabolites and necromass becomes mineral-bound, and thus, stabilized against degradation (37). This could increase C storage underneath cadavers (38), however, the period of time until it vanishes is unknown. In soils where the mineral phase is not supporting stabilisation of organic compounds or, as in many topsoils, is already saturated, microbial metabolites and necromass will cycle rapidly. Rapid metabolization of cadaver-derived C and subsequent release into the atmosphere as CO2 and/or CH4 explains the often insignificant increase in total C content in soil directly underneath decomposing cadavers (39, 40). High input of easily available C beneath cadavers can even cause decreases in soil C due to what is commonly referred to as priming (41, 42). Additionally, microbial oxidation of available C may result in O2 consumption underneath cadavers exceeding its replenishment via diffusion (43). The resulting anoxic conditions may drive the fate of cadaver-derived N (44) and support C losses via anaerobic respiration.
Cadavers, due to their narrow C/N ratios (<6) (45), represent an immense N concentration as compared to the underlying soil, which is often N limited (46). Most N in cadavers is within proteins but also amino sugars (13), which are all prone to simple enzymatic breakdown. Cadaver fluids delivers proteins into the soil; their subsequent decomposition cause increasing concentrations of free amino acids, peptides, and mineral N species (38). Solutions underneath cadavers feature often very narrow C/N ratios due to the production of amines (47). These might become leached from the soil or rapidly degraded, to unknown extents. Under toxic conditions, N mineralisation results in nitrate, which is mobile and easily leachable from soil in case production exceeds uptake by plants and microorganisms. In alkaline soils, ammonium released during decomposition may deprotonate and the resulting gaseous ammonia can be emitted into the atmosphere (48). Under anoxic conditions, which may establish under cadavers upon excessive O2 consumption (see above), nitrate is transformed into gaseous forms (N2, N2O) and released into the atmosphere. Anoxic conditions, however, terminate nitrification, and so N mineralisation is limited to the release of ammonium. Nevertheless, much of cadaver-derived N becomes incorporated into the microbial biomass and is linked to its stabilization and cycling, as described for C. Overall, it seems unlikely that cadaver-derived N accumulates more strongly in soils than cadaver-derived C. And as for C, it remains unresolved how long the imprint of the tremendous N input from cadavers into underlying soils will last.
Cadaver residues are also high in P, which – aside of bones – is mainly contained in phospholipids and nucleotides. These compounds are labile, and cleavage of phosphate ester bonds results in rapid release of ortho-phosphate (49). In contrast to C and N, P is rather immobile in most soils (50). Organic and especially mineral P species tend to sorb strongly to soil minerals, and therefore, are not prone to leaching as, e.g., nitrate. Also, P cycling in soils does not involve formation of gaseous species. However, P has been less frequently considered in studies on cadaver decomposition than C and N. Therefore, little is known about the potential intermediate storage of P in microorganisms (49, 51). On short-term, a strong increase in microbial P can be expected in the soil underneath cadavers. On long term, the overall low mobility of P is probably the decisive factor. Thus, of all bio-elements, P is likely to leave the strongest and longest lasting imprint on soils underneath cadavers (31).
3 Imprint of cadaver decomposition on soil microbial communities
The changes in soil C, N, and P following cadaver decomposition described above induce a strong response by soil microbial communities. The high input of bio-elements significantly changes their biomass, activity, diversity, and composition (18, 52, 53), with taxa-specific temporal trends varying with environmental conditions (18).
3.1 Microbial biomass and activity
Since free-living soil microorganisms are strongly C-limited (54), the C input derived from cadaver decomposition stimulates microbial activity and may promote biomass production. However, activity and biomass of some taxa, such as testate amoeba, are negatively impacted by the high input of bio-elements (22, 55). The effect of N and P on microbial biomass and activity is far less known. Soil microbial activity can start increasing within 24 hours (56–58) and peak within the first ten days after death (18, 59). Microbial biomass production peaks during the active decay phase and slows down thereafter (18). The exact timing and magnitude of the peaks depend on environmental conditions, such as temperature, with microbial biomass production being potentially more affected than activity (58). After their initial peaks, microbial biomass and activity can remain elevated for a long period of time. For instance, microbial biomass C remained elevated for 430 days after burial of pig cadavers (60) and bacteria colony forming units were found to be increased in soil underneath the carcasses for even 42 months after burial (61). Since burial can slow down decomposition (62, 63), the results of the latter two studies may not be representative for aboveground cadavers.
It is noteworthy that the different methods used to determine microbial biomass (e.g., substrate-induced respiration (60), lipid-P extraction (12), chloroform-fumigation extraction (64), colony-forming units (61), and microbial activity/carbon mineralisation (58)) all capture different fractions of the soil microbial biomass or activity, which may contribute to diverging conclusions by individual studies.
3.2 Diversity and community composition
Advances in high‐throughput multi-taxa identification using environmental DNA (65, 66) allowed for better characterization and understanding of changes in microbial community diversity and composition induced by cadaver decomposition.
The initial input pulse of nutrients constitutes a major disturbance of the soil microbial food web. This disturbance causes decreased microbial diversity by favouring few well-adapted taxa. In a deciduous forest, decomposing pig cadavers caused a drastic decrease in the abundance and richness of testate amoebae (22, 55). In turn, the relative abundance of Proteobacteria and Firmicutes increased while that of Acidobacteria decreased during the phase of active decomposition of human remains (18). Generally, studies point at a clear succession in microbial communities during cadaver decay, with the majority of microbial taxa occurring only during specific decomposition stages (53, 67). Nevertheless, the specific role of the different microbial taxa and functional groups in breaking down and transforming cadaver material remains poorly known.
Effects of cadaver impact on soil microbial community composition are long-lasting and vary as a function of the environmental settings (68). Differences in soil microbial community composition between cadaver-impacted and control plots were detectable for up to 1051 days (22). Interestingly, cadaver imprint on the microbial community composition appears to last longer than its imprint on functions. For instance, the study of Singh et al. (69) observed 732 days after the onset of cadaver decomposition only differences in soil bacterial community composition but not in soil functions. It has also been shown there is microbial fauna specifically associated with cadaver decomposition, including an important proportion of taxa not yet described (22). Only about 40% of these organisms are present in bulk soil while the remaining ones come from various sources, including blow flies and other scavengers, or derive from the cadaver itself (70).
4 Research gaps and perspectives
When compiling the literature, we identified gaps and biases in research on the fate of cadavers and related matter cycling in soil (Table 1). We grouped them according to three broad overarching issues, arranged hierarchically.
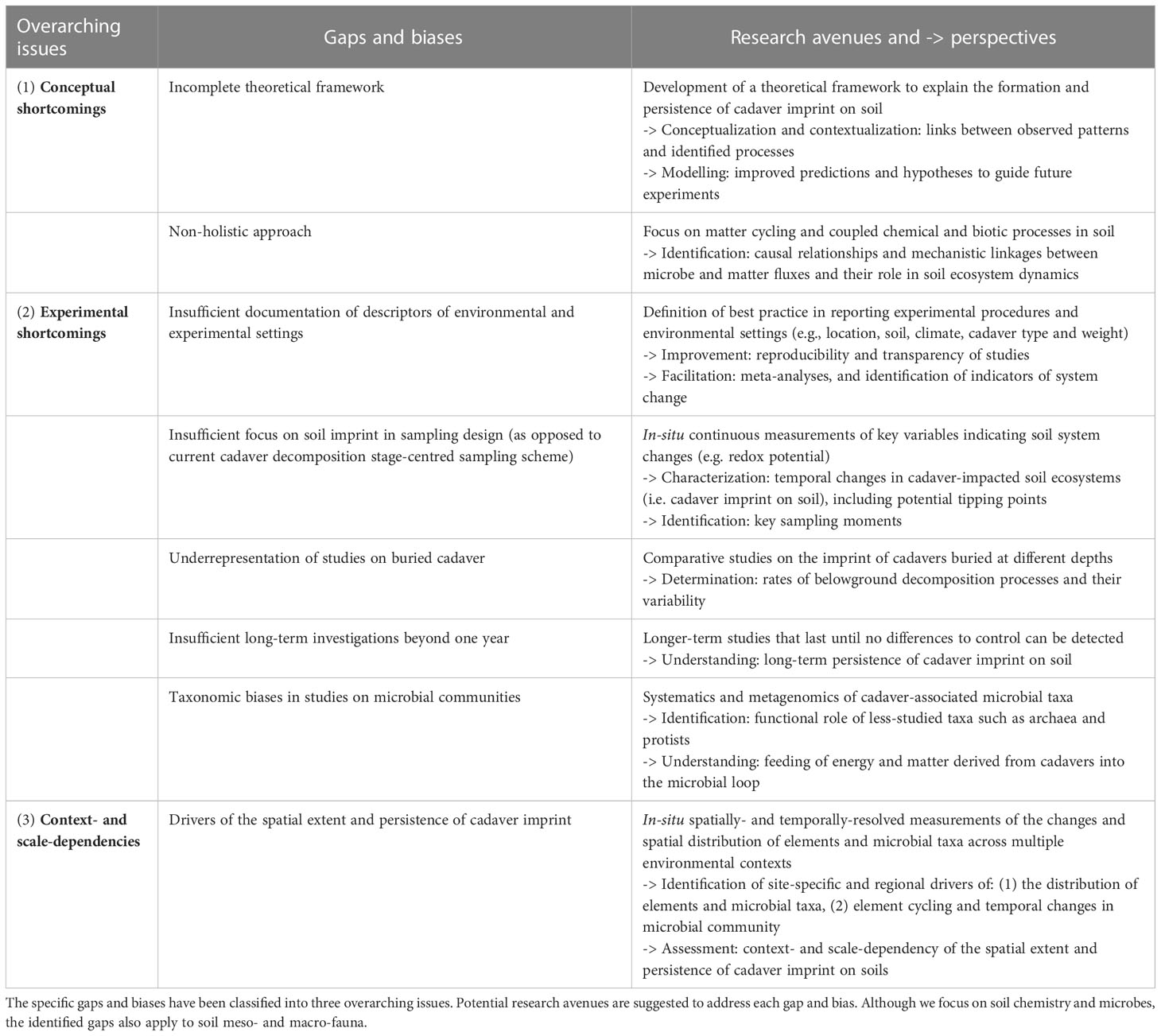
Table 1 Identified gaps and biases in studies on the imprint of cadaver-derived biomass on soil ecosystems.
We propose that the first priority should be to address conceptual shortcomings, since a strong conceptual framework directly contributes to tackling methodological issues and scale and context dependencies. For instance, holistic approaches based on profound theoretical frameworks, e.g., ecological stoichiometry theory (33), metacommunity theory (71), meta-ecosystem theory (72) can help identifying mechanistic relationships and causal linkages among microbial taxa and functional groups and matter fluxes, as well as their role for the rest of the food chain (plants and soil meso- and macrofauna). Improved knowledge of these linkages is key to develop innovative long-term experimental approaches that allow for continuously tracking the progressing changes in cadaver-impacted soils and to identify microbial taxa with key functional roles. Promising indicators of continuous changes in soil include gas fluxes and redox potential. Comparative studies on cadavers buried at different depths, although technically challenging, are needed to determine the rates of belowground decomposition processes and their variability. Belowground decomposition has a relatively marginal importance for ecosystem processes but is highly relevant for forensic applications. Improved documentation of descriptors of environmental and experimental settings are required to facilitate meta-analyses and comparisons among studies dealing with different environmental conditions and spatial scales. This would allow for generalizing the scale and context dependency of the temporal persistence and spatial extent of cadaver imprint in different soil ecosystems. This knowledge would, in turn, feed back to the validation and/or improvement of the theoretical framework.
In conclusion, solving the identified issues will support better understanding the linkages between cadaver-derived matter and microbial taxa and functions, as well as the ecological role of cadaver decomposition within and across ecosystems. In turn, an improved mechanistic understanding of the cadaver imprint on soil ecosystems would contribute to better transferability of models and consequently scaling the role of cadaver decomposition in global organic matter cycle. Practically, it would also pave way to new forensic applications, including improved detection of buried cadavers, identification of new bioindicators, and prediction and mapping of potential cadaver imprint persistence according to regions. Overall, the proposed future research on cadaver-derived imprint on soils has the potential to serve as a hub for connecting soil chemistry, microbial ecology, forensic sciences, and ecosystems science.
Author contributions
All authors listed have made a substantial, direct, and intellectual contribution to the work and approved it for publication.
Acknowledgments
The authors would like to thank and acknowledge Dr. C.V.W. Seppey and Dr. Ildikò Szelecz for comments on early version of the manuscript. This work was completed with financial support from the Germany’s joint federal and state program supporting early-career researchers (WISNA) to the last author. The publication of this article was funded by the Deutsche Forschungsgemeinschaft (DFG, German Research Foundation) – Project number 491466077.
Conflict of interest
The authors declare that the research was conducted in the absence of any commercial or financial relationships that could be construed as a potential conflict of interest.
Publisher’s note
All claims expressed in this article are solely those of the authors and do not necessarily represent those of their affiliated organizations, or those of the publisher, the editors and the reviewers. Any product that may be evaluated in this article, or claim that may be made by its manufacturer, is not guaranteed or endorsed by the publisher.
References
1. Benbow ME, Tomberlin JK, Tarone AM. Introduction to carrion ecology, evolution, and their applications. In: Benbow ME, Tomberlin JK, Tarone AM, editors. Carrion Ecology, Evolution, and their Applications. Boca Raton: CRC Press (2018). p. 3–11.
2. Carter DO, Tibbett M. Cadaver decomposition and soil: Processes. In: Tibbett M, Carter DO, editors. Soil analysis in forensic taphonomy. Boca Raton, FL: CRC Press (2008). p. 29–51.
3. Lauber CL, Metcalf JL, Keepers K, Ackermann G, Carter DO, Knight R. Vertebrate decomposition is accelerated by soil microbes. Appl Environ Microbiol (2014) 80:4920–9. doi: 10.1128/AEM.00957-14
4. Carter DO, Yellowlees D, Tibbett M. Cadaver decomposition in terrestrial ecosystems. Naturwissenschaften (2007) 94:12–24. doi: 10.1007/s00114-006-0159-1
5. Horenstein MB, Linhares AX, Ferradas BRde, Garcia D. Decomposition and dipteran succession in pig carrion in central Argentina: ecological aspects and their importance in forensic science. Med Vet Entomol (2010) 24:16–25. doi: 10.1111/j.1365-2915.2009.00854.x
6. Matuszewski S, Bajerlein D, Konwerski S, Szpila K. Insect succession and carrion decomposition in selected forests of central europe. part 3: Succession of carrion fauna. Forensic Sci Int (2011) 207:150–63. doi: 10.1016/j.forsciint.2010.09.022
7. Marcarelli AM, Baxter CV, Mineau MM, Hall RO. Quantity and quality: Unifying food web and ecosystem perspectives on the role of resource subsidies in freshwaters. Ecology (2011) 92:1215–25. doi: 10.1890/10-2240.1
8. Polis GA, Anderson WB, Holt RD. Toward an integration of landscape and food web ecology: The dynamics of spatially subsidized food webs. Annu Rev Ecol Syst (1997) 28:289–316. doi: 10.1146/annurev.ecolsys.28.1.289
9. Bump JK, Peterson RO, Vucetich JA. Wolves modulate soil nutrient heterogeneity and foliar nitrogen by configuring the distribution of ungulate carcasses. Ecology (2009) 90:3159–67. doi: 10.1890/09-0292.1
10. Barton PS, Cunningham SA, Lindenmayer DB, Manning AD. The role of carrion in maintaining biodiversity and ecological processes in terrestrial ecosystems. Oecologia (2013) 171:761–72. doi: 10.1007/s00442-012-2460-3
11. Pringle JK, Stimpson IG, Wisniewski KD, Heaton V, Davenward B, Mirosch N, et al. Geophysical monitoring of simulated homicide burials for forensic investigations. Sci Rep (2020) 10:7544. doi: 10.1038/s41598-020-64262-3
12. Benninger LA, Carter DO, Forbes SL. The biochemical alteration of soil beneath a decomposing carcass. Forensic Sci Int (2008) 180:70–5. doi: 10.1016/j.forsciint.2008.07.001
13. Stuart B. Decomposition chemistry: Overview, analysis, and interpretation. In: Siegel JA, Saukko PJ, editors. Encyclopaedia of forensic sciences. London, UK: Elsevier (2013). p. 11–5. doi: 10.1016/B978-0-12-382165-2.00120-3
15. Coe M. The decomposition of elephant carcases in the tsavo (East) national park, Kenya. J Arid Environ (1978) 1:71–86. doi: 10.1016/S0140-1963(18)31756-7
16. Megyesi MS, Nawrocki SP, Haskell NH. Using accumulated degree-days to estimate the postmortem interval from decomposed human remains. J Forensic Sci (2005) 50:618–26. doi: 10.1520/JFS2004017
17. Prahlow JA, Byard RW. Postmortem changes and time of death. In: Prahlow JA, Byard RW, editors. Atlas of forensic pathology. New York, Dordrecht, Heidelberg, London: Springer; Humana Press (2012). p. 145–98.
18. Cobaugh KL, Schaeffer SM, DeBruyn JM. Functional and structural succession of soil microbial communities below decomposing human cadavers. PloS One (2015) 10:e0130201. doi: 10.1371/journal.pone.0130201
19. Pechal JL, Crippen TL, Benbow ME, Tarone AM, Dowd S, Tomberlin JK. The potential use of bacterial community succession in forensics as described by high throughput metagenomic sequencing. Int J Legal Med (2014) 128:193–205. doi: 10.1007/s00414-013-0872-1
20. Metcalf JL, Wegener Parfrey L, Gonzalez A, Lauber CL, Knights D, Ackermann G, et al. A microbial clock provides an accurate estimate of the postmortem interval in a mouse model system. Elife (2013) 2:e01104. doi: 10.7554/eLife.01104
21. Fu X, Guo J, Finkelbergs D, He J, Zha L, Guo Y, et al. Fungal succession during mammalian cadaver decomposition and potential forensic implications. Sci Rep (2019) 9:12907. doi: 10.1038/s41598-019-49361-0
22. Seppey CVW, Fournier B, Szelecz I, Singer D, Mitchell EAD, Lara E. Response of forest soil euglyphid testate amoebae (Rhizaria: Cercozoa) to pig cadavers assessed by high-throughput sequencing. Int J Legal Med (2016) 130:551–62. doi: 10.1007/s00414-015-1149-7
23. Madea B, Kernbach-Wighton G. Early and late postmortem changes. In: Siegel JA, Saukko PJ, editors. Encyclopaedia of forensic sciences. London, UK: Elsevier (2013). p. 217–28. doi: 10.1016/B978-0-12-382165-2.00187-2
24. Graw M, Schmidt M, Schneckenberger K, Fiedler S. Degradation von leichen: Fäulnis, verwesung und artefaktbildung. Boden Wasser (2002) 54:16–9.
25. Nolan A-N, Mead RJ, Maker G, Bringans S, Chapman B, Speers SJ. Examination of the temporal variation of peptide content in decomposition fluid under controlled conditions using pigs as human substitutes. Forensic Sci Int (2019) 298:161–8. doi: 10.1016/j.forsciint.2019.02.048
26. Haslam TCF, Tibbett M. Soils of contrasting pH affect the decomposition of buried mammalian (Ovis aries) skeletal muscle tissue. J Forensic Sci (2009) 54:900–4. doi: 10.1111/j.1556-4029.2009.01070.x
27. Dick HC, Pringle JK. Inorganic elemental analysis of decomposition fluids of an in situ animal burial. Forensic Sci Int (2018) 289:130–9. doi: 10.1016/j.forsciint.2018.05.034
28. Szelecz I, Koenig I, Seppey CVW, Le Bayon R-C, Mitchell EAD. Soil chemistry changes beneath decomposing cadavers over a one-year period. Forensic Sci Int (2018) 286:155–65. doi: 10.1016/j.forsciint.2018.02.031
29. Yong SK, Jalaludin NH, Brau E, Shamsudin NN, Heo CC. Changes in soil nutrients (ammonia, phosphate and nitrate) associated with rat carcass decomposition under tropical climatic conditions. Soil Res (2019) 57:482. doi: 10.1071/SR18279
30. Heo CC, Tomberlin JK, Aitkenhead-Peterson JA. Soil chemistry dynamics of Sus scrofa carcasses with and without delayed diptera colonization. J Forensic Sci (2021) 66:947–59. doi: 10.1111/1556-4029.14645
31. Barton PS, Reboldi A, Dawson BM, Ueland M, Strong C, Wallman JF. Soil chemical markers distinguishing human and pig decomposition islands: A preliminary study. Forensic Sci Med Pathol (2020) 16:605–12. doi: 10.1007/s12024-020-00297-2
32. Perrault KA, Forbes SL. Elemental analysis of soil and vegetation surrounding decomposing human analogues. J Can Soc Forensic Sci (2016) 49:138–51. doi: 10.1080/00085030.2016.1184840
33. Sterner RW, Elser JJ. Ecological stoichiometry: The biology of elements from molecules to the biosphere. Princeton, NJ: Princeton University Press (2003).
34. Buckeridge KM, Creamer C, Whitaker J. Deconstructing the microbial necromass continuum to inform soil carbon sequestration. Funct Ecol (2022) 36:1396–410. doi: 10.1111/1365-2435.14014
35. Luong S, Forbes SL, Wallman JF, Roberts RG. Monitoring the extent of vertical and lateral movement of human decomposition products through sediment using cholesterol as a biomarker. Forensic Sci Int (2018) 285:93–104. doi: 10.1016/j.forsciint.2018.01.026
36. Geyer KM, Kyker-Snowman E, Grandy AS, Frey SD. Microbial carbon use efficiency: Accounting for population, community, and ecosystem-scale controls over the fate of metabolized organic matter. Biogeochemistry (2016) 127:173–88. doi: 10.1007/s10533-016-0191-y
37. Kästner M, Miltner A, Thiele-Bruhn S, Liang C. Microbial necromass in soils - linking microbes to soil processes and carbon turnover. Front Environ Sci (2021) 9:756378. doi: 10.3389/fenvs.2021.756378
38. Macdonald BC, Farrell M, Tuomi S, Barton PS, Cunningham SA, Manning AD. Carrion decomposition causes large and lasting effects on soil amino acid and peptide flux. Soil Biol Biochem (2014) 69:132–40. doi: 10.1016/j.soilbio.2013.10.042
39. Quaggiotto M-M, Evans MJ, Higgins A, Strong C, Barton PS. Dynamic soil nutrient and moisture changes under decomposing vertebrate carcasses. Biogeochemistry (2019) 146:71–82. doi: 10.1007/s10533-019-00611-3
40. Keenan SW, Emmons AL, Taylor LS, Phillips G, Mason AR, Mundorff AZ, et al. Spatial impacts of a multi-individual grave on microbial and microfaunal communities and soil biogeochemistry. PloS One (2018) 13:e0208845. doi: 10.1371/journal.pone.0208845
41. Wheeler TA, Kavanagh KL. Soil biogeochemical responses to the deposition of anadromous fish carcasses in inland riparian forests of the pacific Northwest, USA. Can J For Res (2017) 47:1506–16. doi: 10.1139/cjfr-2017-0194
42. Jilling A, Keiluweit M, Gutknecht JL, Grandy AS. Priming mechanisms providing plants and microbes access to mineral-associated organic matter. Soil Biol Biochem (2021) 158:108265. doi: 10.1016/j.soilbio.2021.108265
43. von der Lühe B, Fiedler S, Mayes RW, Dawson L. Temporal fatty acid profiles of human decomposition fluid in soil. Org Geochem (2017) 111:26–33. doi: 10.1016/j.orggeochem.2017.06.004
44. Keenan SW, Schaeffer SM, Jin VL, DeBruyn JM. Mortality hotspots: Nitrogen cycling in forest soils during vertebrate decomposition. Soil Biol Biochem (2018) 121:165–76. doi: 10.1016/j.soilbio.2018.03.005
45. Parmenter RR, MacMahon JA. Carrion decomposition and nutrient cycling in a semiarid shrub–steppe ecosystem. Ecol Monogr (2009) 79:637–61. doi: 10.1890/08-0972.1
46. Fanelli G, Lestini M, Sauli AS. Floristic gradients of herbaceous vegetation and P/N ratio in soil in a Mediterranean area. Plant Ecol (2007) 194:231–42. doi: 10.1007/s11258-007-9287-8
47. Ioan BG, Manea C, Hanganu B, Statescu L, Gheuca Solovastru L, Manoilescu I. The chemistry decomposition in human corpses. Rev Chim (2017) 68:1352–6. doi: 10.37358/RC.17.6.5672
48. Behera SN, Sharma M, Aneja VP, Balasubramanian R. Ammonia in the atmosphere: A review on emission sources, atmospheric chemistry and deposition on terrestrial bodies. Environ Sci pollut Res Int (2013) 20:8092–131. doi: 10.1007/s11356-013-2051-9
49. Richardson AE, Simpson RJ. Soil microorganisms mediating phosphorus availability update on microbial phosphorus. Plant Physiol (2011) 156:989–96. doi: 10.1104/pp.111.175448
50. Statheropoulos M, Agapiou A, Spiliopoulou C, Pallis GC, Sianos E. Environmental aspects of VOCs evolved in the early stages of human decomposition. Sci Total Environ (2007) 385:221–7. doi: 10.1016/j.scitotenv.2007.07.003
51. Mason-Jones K, Robinson SL, Veen GFC, Manzoni S, van der Putten WH. Microbial storage and its implications for soil ecology. ISME J (2022) 16:617–29. doi: 10.1038/s41396-021-01110-w
52. Moreno LI, Mills D, Fetscher J, John-Williams K, Meadows-Jantz L, McCord B. The application of amplicon length heterogeneity PCR (LH-PCR) for monitoring the dynamics of soil microbial communities associated with cadaver decomposition. J Microbiol Methods (2011) 84:388–93. doi: 10.1016/j.mimet.2010.11.023
53. Parkinson RA, Dias K-R, Horswell J, Greenwood P, Banning N, Tibbett M, et al. Microbial community analysis of human decomposition on soil. In: Ritz K, Dawson L, Miller D, editors. Criminal and environmental soil forensics. Dordrecht: Springer Netherlands (2009). p. 379–94. doi: 10.1007/978-1-4020-9204-6_24
54. Wardle D. A comparative assessment of factors which influence microbial biomass carbon and nitrogen levels in soil. Biol Rev Camb Philos Soc (2008) 67:321–58. doi: 10.1111/j.1469-185X.1992.tb00728.x
55. Szelecz I, Fournier B, Seppey C, Amendt J, Mitchell E. Can soil testate amoebae be used for estimating the time since death? a field experiment in a deciduous forest. Forensic Sci Int (2014) 236:90–8. doi: 10.1016/j.forsciint.2013.12.030
56. Putman RJ. Flow of energy and organic matter from a carcase during decomposition: Decomposition of small mammal carrion in temperate systems 2. Oikos (1978) 31:58. doi: 10.2307/3543384
57. Tibbett M, Carter DO, Haslam T, Major R, Haslam R. A laboratory incubation method for determining the rate of microbiological degradation of skeletal muscle tissue in soil. J Forensic Sci (2004) 49:560–5. doi: 10.1520/JFS2003247
58. Carter DO, Tibbett M. Microbial decomposition of skeletal muscle tissue (Ovis aries) in a sandy loam soil at different temperatures. Soil Biol Biochem (2006) 38:1139–45. doi: 10.1016/j.soilbio.2005.09.014
59. Stokes KL, Forbes SL, Benninger LA, Carter DO, Tibbett M. Decomposition studies using animal models in contrasting environments: Evidence from temporal changes in soil chemistry and microbial activity. In: Criminal and environmental soil forensics. Dordrecht: Springer (2009). p. 357–77. doi: 10.1007/978-1-4020-9204-6_23
60. Hopkins D, Wiltshire P, Turner B. Microbial characteristics of soils from graves: An investigation at the interface of soil microbiology and forensic science. Appl Soil Ecol (2000) 14:283–8. doi: 10.1016/S0929-1393(00)00063-9
61. Schotsmans EMJ, Fletcher JN, Denton J, Janaway RC, Wilson AS. Long-term effects of hydrated lime and quicklime on the decay of human remains using pig cadavers as human body analogues: Field experiments. Forensic Sci Int (2014) 238:141. doi: 10.1016/j.forsciint.2013.12.046
62. Corrĉa RC, Moura MO. Coleoptera associated with buried carrion: Potential forensic importance and seasonal composition. J Med Entomol (2014) 51:1057–66. doi: 10.1603/me13166
63. Marais-Werner A, Myburgh J, Becker PJ, Steyn M. A comparison between decomposition rates of buried and surface remains in a temperate region of south Africa. Int J Legal Med (2018) 132:301–9. doi: 10.1007/s00414-017-1618-2
64. Fiedler S, Schneckenberger K, Graw M. Characterization of soils containing adipocere. Arch Environ Contam Toxicol (2004) 47:561–8. doi: 10.1007/s00244-004-3237-4
65. Taberlet P, Coissac E, Pompanon F, Brochmann C, Willerslev E. Towards next-generation biodiversity assessment using DNA metabarcoding. Mol Ecol (2012) 21:2045–50. doi: 10.1111/j.1365-294X.2012.05470.x
66. Hilal MG, Yu Q, Zhou R, Wang Y, Feng T, Li X, et al. Exploring microbial communities, assessment methodologies and applications of animal’s carcass decomposition: A review. FEMS Microbiol Ecol (2021) 97(8):fiab098. doi: 10.1093/femsec/fiab098
67. Dangerfield CR, Frehner EH, Buechley ER, Şekercioğlu ÇH, Brazelton WJ. Succession of bacterial communities on carrion is independent of vertebrate scavengers. Peer J (2020) 8:e9307. doi: 10.7717/peerj.9307
68. Carter DO, Metcalf JL, Bibat A, Knight R. Seasonal variation of postmortem microbial communities. Forensic Sci Med Pathol (2015) 11:202–7. doi: 10.1007/s12024-015-9667-7
69. Singh B, Minick KJ, Strickland MS, Wickings KG, Crippen TL, Tarone AM, et al. Temporal and spatial impact of human cadaver decomposition on soil bacterial and arthropod community structure and function. Front Microbiol (2018) 8:2616. doi: 10.3389/fmicb.2017.02616
70. Metcalf JL, Xu ZZ, Weiss S, Lax S, van Treuren W, Hyde ER, et al. Microbial community assembly and metabolic function during mammalian corpse decomposition. Science (2016) 351:158–62. doi: 10.1126/science.aad2646
71. Leibold MA, Holyoak M, Mouquet N, Amarasekare P, Chase JM, Hoopes MF, et al. The metacommunity concept: A framework for multi-scale community ecology. Ecol Lett (2004) 7:601–13. doi: 10.1111/j.1461-0248.2004.00608.x
Keywords: cadaver decomposition, bio-elements, soil properties, C, N, P, microbial communities
Citation: Fiedler S, Kaiser K and Fournier B (2023) Cadaver imprint on soil chemistry and microbes - Knowns, unknowns, and perspectives. Front. Soil Sci. 3:1107432. doi: 10.3389/fsoil.2023.1107432
Received: 24 November 2022; Accepted: 02 February 2023;
Published: 15 February 2023.
Edited by:
Derrick Y.F. Lai, The Chinese University of Hong Kong, ChinaReviewed by:
Jens Amendt, Goethe University Frankfurt am Main, GermanyEdward Mitchell, Université de Neuchâtel, Switzerland
Copyright © 2023 Fiedler, Kaiser and Fournier. This is an open-access article distributed under the terms of the Creative Commons Attribution License (CC BY). The use, distribution or reproduction in other forums is permitted, provided the original author(s) and the copyright owner(s) are credited and that the original publication in this journal is cited, in accordance with accepted academic practice. No use, distribution or reproduction is permitted which does not comply with these terms.
*Correspondence: Sabine Fiedler, fiedlers@uni-mainz.de; Bertrand Fournier, bertrand.fournier@uni-potsdam.de