- 1Agronomy Department, INTA Balcarce Research Station, Instituto Nacional de Tecnología Agropecuaria (INTA), Balcarce, Buenos Aires, Argentina
- 2Instituto De Investigación Recursos Biológicos, Instituto Nacional de Tecnología Agropecuaria (INTA), Hurlingham, Buenos Aires, Argentina
- 3Department of Plant & Environmental Sciences, New Mexico State University, Las Cruces, NM, United States
- 4Instituto Nacional de Investigación Agropecuaria (INIA), Sistema Vegetal Intensivo. Estación Experimental Wilson Ferreira Aldunate, INIA Las Brujas, Canelones, Uruguay
- 5Departamento de Geoquímica, Universidade Federal Fluminense (UFF), Niterói, Rio de Janeiro, Brazil
- 6Instituto De Investigación Suelos, Instituto Nacional de Tecnología Agropecuaria (INTA), Hurlingham, Buenos Aires, Argentina
- 7Núcs Temáticos Agr Fam, Embrapa Clima temperado, Empresa Brasileira de Pesquisa Agropecuaria (EMBRAPA), Pelotas, RS, Brazil
- 8Facultad de Ciencia y Tecnología, Universidad Autónoma de Entre Ríos, Entre Ríos, Argentina
This work aims to contextualize and analyze the potential contribution of pecan to SDG2 under the dual perspective of carbon storage and human nutrition. Particularly, the study focuses on the pecan agroecosystems in the Americas, representing the most important pecan-producing countries (the United States, Mexico, Brazil, Argentina, Uruguay, and Peru). We observed that pecan is a reliable sink for storing atmospheric C and also for quality nuts with high nutritional density. The Americas, hold a population of ca. 23 M pecan trees, with the younger tree populations and the highest C-storing potential in South America. This pecan tree population has removed 51.3 Mt CO2eq immobilizing the OC in their aboveground biomass, but if the C sequestration for the whole system is considered, the value reaches nearly 80 Mt CO2eq. From a nutritional perspective, there are different dietary needs to cover according to the country, although the common analysis output is a low proportion of nuts in the diet, which is expected to improve, given the efforts of each country to promote domestic consumption. All the mentioned countries in this study have a low pecan consumption going from 8 to 293 g per capita yr-1, which in the light of the Global Burden of Disease represents 0.08 to 3.2% of the recommended yearly dietary basis for nuts overall. The inclusion of pecan nuts in the daily diet is of utmost importance to offset the food nutrient dilution carbohydrates-based, linked to the excess of atmospheric CO2. Also, pecan orchards function as a platform to integrate sustainable systems. The global benefit of having pecan and alley crops has been proved in regions other than the Americas with interesting economic outputs leading to energizing the life of rural communities. Pecan orchards and pecan agroforestry may lead to sustainable agri-food systems, with global gains in SOC and nutritional richness and diversity. Therefore, more in-depth studies are needed not only to fully understand the functioning of the systems at a productive level but also to design and plan sustainable landscapes in rural land.
1 Introduction
Current ways of field engineering and management of agri-food systems (AFSs) are increasingly undermining environmental sustainability and human health through two main negative impacts: (a) the soil organic carbon (SOC) depletion–greenhouse gas (GHG) emission process (1) and (b) the poor-quality diet–based global nourishment. Many initiatives with worldwide—as well as regional—reach are addressing these impacts through the point of view of, for example, practices leading to soil carbon (C) enrichment; such is the case for the “Four per Thousand” initiative (2, 3). Unlike this soil and agricultural standalone perspective, the framework of the UN Sustainable Development Goals (SDGs) approaches the problem from a more holistic, integrated point of view, aiming for AFSs with less GHG emissions and a global population with a more balanced, healthy diet (4). Among the SDGs, SDG 2 (“Zero Hunger”) is the one straightforwardly linking sustainable agriculture to nutrition, recognizing the strong bond between environmental sustainability and human health (4, 5). This bond can be visualized in targets 2.2 and 2.4, where SDG 2 suggests to “…end all forms of malnutrition…” and “…ensure sustainable food production systems and implement resilient agricultural practices that increase productivity and production, that help maintain ecosystems, that strengthen capacity for adaptation to climate change, extreme weather, drought, flooding, and other disasters, and that progressively improve land and soil quality….” To reach those targets by 2030, the contributions of AFSs yielding edible products are essential.
The focus on edible crops as contributors to SDG 2 is of growing importance, even more so knowing that a continuous decline in the nutrient density of produce for the USA and UK has been revealed since ca. 1940, with iron (Fe), zinc (Zn), phosphorus (P), and sulfur (S) concentrations being significantly diluted in edible fruits (6). The latter can be considered a representative trend since the author (6) analyzed and compared the data of several studies, nutrient concentration–related for edible fruits, concluding that the change for more yielding varieties explained this dilution. Nonetheless, to attribute nutrient dilution in edibles only to genetic improvement means to overlook other problems. On one hand, continuous agriculture has been inducing not only SOC stock depletion through C mineralization and respiration but also soil nutrient depletion, mainly in those areas where SOC stock in the top 100 cm layer concentrates only 50–100 t C ha-1 and agricultural systems have not adopted neither soil conservation practices (1, 7) nor soil nutrient replacement through soil fertilization. On the other hand, the atmospheric CO2 increase may have also contributed to this process (8): in a recent study, Chen et al. (9) revealed that atmospheric CO2 increase induced a mean annual gain in global primary productivity of 44 kg C ha-1 year-1 since the 2000s, contributing to the dilution of nutrients contained in food. Furthermore, Myers et al. (10) reported that, under an environment concentrating 550 ppm CO2, a 5%–10% decrease in mineral content is produced for cereal grains and legumes (the vitamin B group, iron, zinc, and sulfur are affected). Therefore, this is why the dynamics of carbon storage (either in soil or in plant biomass) and human nutrition are so intrinsically linked, making the systems with dual contributions (carbon sequestration in soil and plant biomass and the provision of high nutrient density—food) a key toward the achievement of SDG 2 by 2030.
Few food systems have this inherent duality of increasing carbon storage in the environment through C sequestration and providing nutrient-dense food, being the dried fruit-based systems important contributors to this matter (11). Pecan (Carya illinoinensis [Wangenh.| K. Koch) production is among these systems and has this duality enhanced because it not only produces a high-quality nut but also sequesters C in its biomass (mainly in the trunk) and the soil, given its strong association with ectomycorrhizal fungi (12). Many research efforts currently concentrate on pecan, and this growing interest is reflected in the publishing trend of the last decades, where studies about pecan have skyrocketed. For example, the ScienceDirect page shows that the annual publishing of pecan-related articles went from 83 to 423 during the period 2000–2021, with 58% of the articles produced between 2013 and 2021. Additionally, the browser Scopus showed a similar trend but refined the search (Figure 1A). Considering the same period, articles were labeled as belonging to “Agricultural and Biological Sciences,” “Environmental Science,” “Medicine and Dentistry,” or “Immunology and Microbiology” (Figure 1B). Additionally, if performed in Google Scholar, the search with the keyword “pecan” casts the raw number of 9,230 articles only for the period 2020–2021, without excluding languages other than English. Those results are no minor data since pecan is ranked sixth among the main nuts globally produced (3% of the global nut production), with an annual increase of production of 4.74 t (13). Furthermore, the global area planted with pecan represents 4% (ca. 410,000 ha) of the total area planted with nuts (14). Despite the mentioned importance of this edible crop, no assessment up to date has analyzed pecan contributions to SDG 2, targeting its capacity to build climate resilience and improve the human diet.
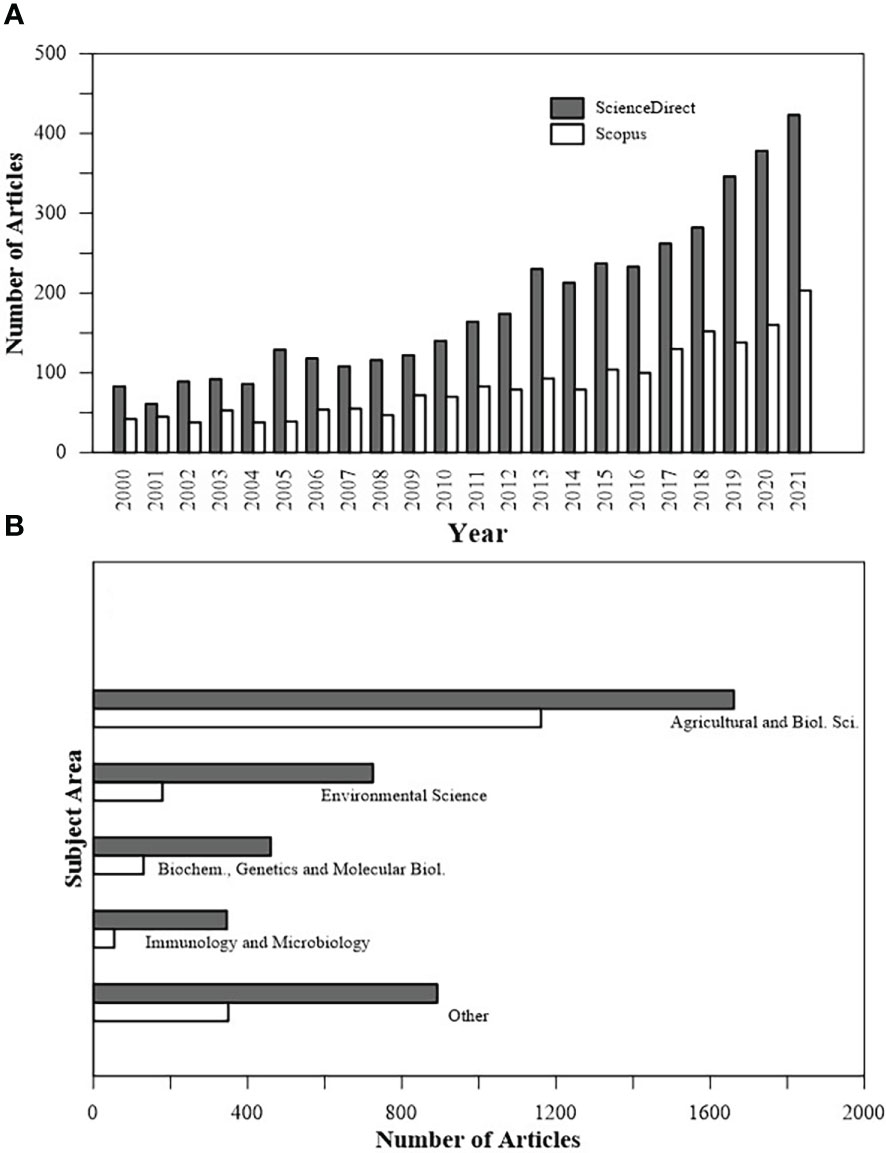
Figure 1 Published articles related to pecan during the period 2000–2021. (A) According to year and scientific browser. (B) According to subject area and scientific browser.
The conceptual framework for our analysis is shown in Figure 2, where C enters the pecan agroecosystem through C fixation; is sequestered in tree biomass; and eventually moves through several compartments such as leaves, husks, and pruning residues until is harvested along with other nutrients. We also considered that C sequestered in leaves, pruning residues, and other debris effectively contributes to build the SOC pool since ectomycorrhizal fungi win the competition for water against decomposer fungi, and the latter cannot rapidly decompose carbonated chains (15).
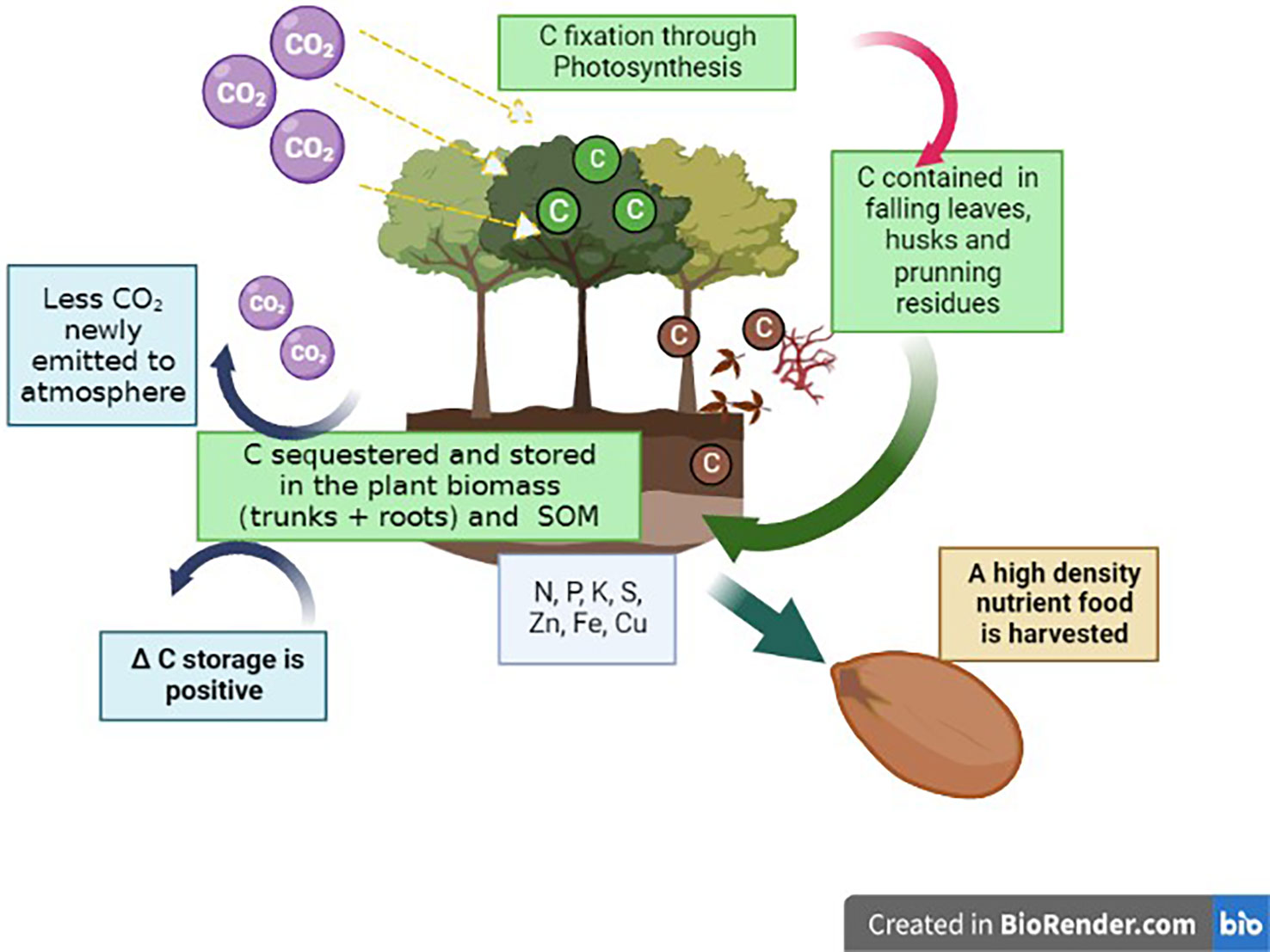
Figure 2 Carbon recycling through the pecan agroecosystem and its two main results: positive C balance (Δ C storage) and high-density nutrient food. Diagram created in Biorender.com (2022).
Few studies have analyzed what happens with C under a pecan orchard (16–18), although from a single point of view. An interesting contribution is that of Lee and Jose (17), which evaluated how soil respiration and C stock responded to pecan orchard aging, with the older pecan orchard having not only greater CO2 emissions but also greater SOM, microbial biomass, and fine root biomass than the younger orchard. Hence, the integration of these three approaches, C fixation (16), C balance (18), and soil C respiration and microbial biomass (17), gives an idea of the building of not only climatic resilience with pecan but also a proper “muscle” plant architecture to extract nutrients and turn them into a nutrient-dense edible product. Thus, a standalone, partial perspective could not wholly address any contribution to SDG 2 by pecan.
1.1 Methodology for literature screening
To properly conduct a review of this kind, we refined our search in ScienceDirect, Scopus, Google Scholar, and other additional scientific browsers in order to build a sufficiently strong literature database of long-term and short-term field research, meta-analyses published in peer-reviewed journals by introducing the terms “pecan,” “nuts,” “noz pecá,” “nogales,” “nuez pecanera,” “orchard,” “carbon dioxide,” “carbon sequestration,” and “nutritional quality.” We also related the aspects of this search with regional information such as maps and statistics.
1.2 Objectives
Unlike previous research efforts focused on analyzing standalone components of the pecan production system, this review adopts a novel perspective to contextualize and analyze the potential contribution of pecan to SDG 2 under the dual perspective of carbon storage and human nutrition. Particularly, the study focuses on the pecan agroecosystems in the Americas, considering the most important pecan-producing countries (the USA, Mexico, Brazil, Argentina, Uruguay, and Peru). Therefore, the American pecans are here understood as an AFS that may potentially provide several opportunities to meet SDG 2’s 2.2 and 2.4 targets. Our review is particularly relevant as the value of pecans is often measured only based on their economic and social benefits, while their function and current and potential role in climate change mitigation has been systematically overlooked.
The rest of this paper is structured as follows. Section 2 summarizes the literature on the problem of soil carbon depletion in AFSs and the role of pecan-based agroforestry as an SOC improver. Section 3 demonstrates the global need for a balanced diet and the nutritional value of pecan. Section 4 mainly exposes relevant statistics about pecan production and planted area in the Americas, with estimates about carbon stock. Section 5 puts forward the relevance of pecan orchards as a platform to integrate sustainable AFSs. Section 6 analyzes pecan orchards as a sink for carbon sequestration in aboveground and belowground biomass. Finally, Section 7 bears conclusions and recommendations.
2 The challenge of soil organic carbon depletion in agricultural food systems and the role of pecan-based agroforestry as a soil organic carbon improver
In the previous paragraphs, we introduced some of the SOC depletion problems from the point of view of the AFSs overall, highlighting some advantages of the pecan AFS to store C. To fully grasp the contribution of pecan to carbonize the AFS through C storage and sequestration, we will focus on two C loss paths: soil GHG emissions and soil erosion. We will also remark on the role of pecan orchards and pecan-based agroforestry.
2.1 Soil organic carbon loss and soil greenhouse gas emissions vs. carbon sequestration
The Agriculture, Forestry, and Other Land Use activities contributed to 13% of CO2, 44% of CH4, and 81% of N2O emissions from human activities during 2007–2016 (19); however, depending on the soil management and land use, agricultural activities can be considered as a source or sink of GHGs, changing the carbon and nitrogen dynamics (19, 20) and contributing negatively or positively to the global C budget. For example, Sanderman et al. (21) estimated that, at the global level, soils accumulated a C loss of 116 Pg at 2 m depth. This loss is often associated to regions with soil degradation caused by crop and pasture mismanagement, leading to high soil respiration rates and SOC exhaustion, suggesting that these regions should be targeted in efforts to restore SOC (21). The global estimates of total degraded areas range from 1 to 6 billion ha, which represents 40% of the world’s soil (22, 23). Planting pecan orchards can contribute to mitigate SOC loss in such areas by acting over soil C respiration (15, 17) and through C sequestration (16).
Carbon sequestration (as in soil as well as in trees biomass) can significantly contribute to the stabilization of global warming levels, helping to keep the annual global temperature increases below 2°C or even 1.5°C in relation to pre-industrial levels, a target stipulated in the Paris Agreement (21st Session of the Conference of the Parties or COP-21) (23, 24). In such a sense, it has been estimated that an annual 0.4% increase in the SOC stock of the 0–40 cm layer of the soil can offset anthropogenic emissions and stabilize climate change, in addition to ensuring food security (3). Nonetheless, the latter estimate overlooked the contribution of C sequestered by tree trunks.
Likewise, although some conservationist agricultural practices (e.g., no till) to protect the soil and increase SOC are essential for maintaining the sustainability of agricultural systems and the environment (25–27), they demand more inputs every year. Therefore, the adoption of agricultural systems based purely on low-carbon agriculture technologies is a condition sine qua non to guarantee a positive C budget. Agroforestry plays a significant role to guarantee that result, with South America having a high potential to offset global emissions, estimated approximately 8.24 Pg C for the period 2016–2050 (28). As mentioned earlier, and given its suitability for agroforestry, pecans perfectly fit to meet this challenge. Thus, the assessment of the environmental impacts caused by agricultural systems has increasing focus, as well as the development of technologies and agricultural management practices to reduce and offset these impacts (29, 30). Agricultural management practices can significantly change the dynamics of GHG emission; thus, it is essential to study and monitor GHG fluxes in different climate and soil conditions (31–33).
Agroforestry systems seek sustainable agricultural production by associating the different agricultural, livestock, and forestry production systems, which can be done in intercropping, in succession, or in rotation, mutually benefiting the components (34, 35). The adoption of less aggressive management practices, such as the agroforestry system replacing the conventional system of cultivation, contributes to the mitigation of CO2 and stores greater amounts of C in the soil (36–38). That system increases the plant residues in the soil surface and the soil organic matter content, reducing soil erosion and improving their resilience and their capacity to store carbon (34, 39). For example, after 4 years of conversion of degraded pasture to an agrosilvopastoral system in Brazil, there was an increase in soil organic carbon stocks from 52.6 to 66.5 Mg ha-1, indicating that it is a sustainable alternative and option for the recovery of degraded soils (37). In addition to the recovery of degraded areas, the agrosilvopastoral system preserves forest areas due to the land spare effect and brings economic and social impacts, promoting positive effects throughout society (40, 41). Similarly, a nut tree–based agroforestry can lead to counterbalance SOC loss and soil GHG emissions through C sequestration (11).
2.2 Soil organic carbon lost by soil erosion as mitigated by cover crops in pecan-based agroforestry
Soil C erosion is driven by a weakened soil structure as result of poor agricultural practices applied in susceptible soils, which impacts long-term soil sustainability (21, 27). This loss of C also reflects the exhaustion of other nutrients as it has been demonstrated in the Argentina`s case (42). Alike the soil GHG emissions problem, several farming practices have been identified to minimize or restore SOC loss by reducing soil erosion and improving soil quality (43). One of the most widespread practices is the use of cover crops, which have been demonstrated to enhance SOC sequestration when applied to pecan-based agroforestry systems (44).
Overall, soil C sequestration depends upon the weather (especially temperature and rainfall) and soil characteristics (45) that affect the activity of the soil microorganisms involved in degrading organic residues (46) and the amount and quality of the C input (47). For that, indicators such as the precipitation/temperature ratio are essential for understanding cover crop residue decomposition in different environments (48). In addition, soil texture is another key factor that may modify soil C fixation (49) by regulating soil aeration and, consequently, SOM decomposition and mineralization (27). In fine-textured soils, the smaller average pore size and the presence of organic–mineral complexes reduce SOM exposure to microbes for mineralization compared with the large pore sizes typical of coarse-textured soils (50). Thus, cover crop residues decompose differently according to the location and the system they are included in. For example, the inclusion of cover crops in cropping systems showed average SOC sequestration rates of 0.45 t C ha-1 yr-1 in the Pampa Region (51–53), fertilizer use (urea, 46% N) showed an increment of approximately 0.18 t C ha yr-1 (54, 55), and the inclusion of cycles with perennial pastures in crop rotations showed average SOC sequestration rates of 0.76 t C ha-1yr-1 (56, 57). Then, with proper management, a cover crop growing upon a pecan-based agroforestry system may boost soil C sequestration, adding up to soil biomass produced by the association between pecan and ectomycorrhizal fungus (15).
It worth mentioning that current area meant for agroforestry could sequester up to 2.2 Pg C above- and belowground in the next 50 years, but estimations on the global land area occupied by agroforestry systems are particularly uncertain. Global areas under tree intercropping, multistrata systems, protective systems, silvopasture, and tree woodlots are estimated at 700, 100, 300, 450, and 50 M ha, respectively. The SOC storage in agroforestry systems is also uncertain and may amount to up to 300 Mg C ha−1 to 1 m depth (58). In China, for example, a meta-analysis provided evidence that existing agroforestry systems, particularly shelterbelts and agrosilvicultural systems, increase SOC stocks due to not only SOC sequestration but also the effect of protection against wind and water C dispersal. Moreover, Mayer et al. (59) observed that the use of agroforestry systems increases SOC compared to cropping systems in a temperate climate. In either case, pecan-based agroforestry integrated in the green belts of the cities may bring substantial benefits by increasing and conserving SOC stocks.
2.3 Pecan orchards, pecan-based agroforestry, and their role as a soil organic carbon improver
Under the context described above, pecan orchards and pecan-based agroforestry have interesting implications on soil health and particularly on SOC. In such a sense, pecan trees growing in their native region (the USA and Mexico) will successfully prevail in well-drained, OM-rich soils, which, in addition to being subjected to C enrichment via the shedding of pecan leaves, shucks, sticks, bark, and other debris, self-generate high-quality conditions (60). In a well-detailed study performed during 2020 and 2021, Slade and Wells (61) found that the values of active carbon, aggregate stability, organic matter, total N, and Solvita CO2-burst (a test for measuring soil microbiological activity) for different aged pecan orchards amply overpassed those of row crops, with the most aged orchards having better soil quality. In another study, Dold et al. (44) marked the relevance of the contribution of the leafy material to carbon sequestration and N supply to soil in a 17-year-old pecan-based agroforestry system, estimating a mean contribution of 17 and 0.68 kg N tree-1 for falling pecan leaves during 2016. Finally, Idowu et al. (62) proved that the addition of pecan husks to arid soils boosted P, K, and wet aggregate stability for sandy clay and sandy loam textured soils. Therefore, this positive feedback between what is produced aboveground and the soil ensures soil with high standards of quality, offering a unique AFS to cope with soil C emissions and soil erosion. Moreover, soil quality is intrinsically associated with food quality and with the agricultural practices leading to maintain such quality. In that sense, after evaluating the effects of conventional and organic fertilization on pecan, Noperi-Mosqueda et al. (63) concluded that the nutritional properties of the pecan nut were improved under a balanced combination of both fertilization practices, with phenolic compounds N, P, and K being in the highest values. In addition, falling pecan husks have been proven to absorb heavy metals like chromium (64); thus, food produced under this type of agroecosystem can be considered environmentally safer than other AFSs.
In future studies, effort should be put on understanding the response of the biophysics (soil temperature, soil moisture, etc.) to management in the pecan orchard when integrated to agroforestry, in order to develop robust models to predict C cycling in these systems.
3 The global need for a balanced diet and the nutritional value of pecan
Food production is scarce to cover the needs of the world’s population, but there are also serious drawbacks in its distribution and unacceptable loss and waste that call for a profound and detailed reconsideration of the actual food system (4). While a big part of the world’s population is suffering from undernourishment, the percentage of people affected by overweight, obesity, and other non-transmissible diseases that are directly conditioned by the diet keeps growing. Efforts done by doctors, researchers, health organizations, and others have made it possible to accumulate much knowledge about the needs for a healthy life. Numerous balanced diets and food intake recommendations are spread around the world. Nevertheless, average consumption varies vastly from country to country, from region to region, being in many places far from the suggested healthier values. It is painful and embarrassing for humanity that 1,562 persons are facing starvation and death daily because of not accessing the food they need (65).
Average dry fruit consumption in the world appears to be among the lowest values compared with those of other food groups (4). The importance of consuming dry fruits and nuts, including pecans, has been determined in many studies, and there are different efforts to promote their consumption considering the benefits they generate (66). The nutritional composition of pecan nuts (Table 1) varies from cultivar to cultivar (67–73), and, most likely, the growing site conditions influence this composition. In average, 100 g of pecan nuts contain 69.3 g of oil, and significant concentrations of micronutrients (K, P, and Mg) and antioxidants (phenols, tannins, and tocopherols) (Table 1). In any case, the inputs accounted for in our diets from pecan consumption will be highly beneficial.
Antioxidant potential due to the high content of phenols has been determined and puts pecan among the highest when compared to the rest of the food sources (74, 75). The nutraceutical compounds present and the fatty acid profile of pecan oil (76) make it possible to classify it as one of the healthier oils together with olive oil.
Studies carried out by numerous researchers prove the beneficial effect of consuming pecan due to increased blood circulation and the reduction of cardiovascular diseases (77, 78), reduced risks of type 2 diabetes (79), a positive influence on the development of the immune response, and the alleviation of inflammatory processes (74).
A recent review by Naghshi et al. (80) looked at the association between nut consumption and the risk of having cancer and its mortality. The meta-analysis carried out over 52 published papers led them to conclude that a 5 g day-1 increase in total nut intake can be associated to 3% and 25% lower risks of overall and colon cancers occurrence respectively, values that increased with higher nut intakes. Moreover, regarding cancer mortality, the authors found a 4% lower risk of cancer mortality with the 5 g day-1 total nut intake.
The consumption promotion of nuts has also developed the interest to determine if there is a health issue to be considered in regard to the negative effects that could be caused by too high an intake. As an example, some studies have considered the potential of reaching harmful levels of heavy metals (81), but no special consideration arises under normal production and consumption conditions.
Although there is enough information to support all the measures toward the increase of pecan production and consumption, more studies and analyses linking nutritional and environmental aspects are needed for pecan.
4 Pecan-planted area and production in the Americas
Although the pecan-planted area is concentrated around its origin region of origin (the USA and Mexico altogether contribute 76.2% of the planted area worldwide), the species is making progress in other regions of the world as seen in Table 2. These statistics about the planted area show not only pecan’s distribution worldwide but also its capability to adapt to a diversity of soils and environments, which is especially notorious in the American continent, the focus of this analysis. The following two sections characterize pecan production in North and South America.
4.1 Pecans in North America: Contribution of pecan to carbon stocks and daily diet
4.1.1 Pecans in the USA
Pecan nut production in the USA is concentrated in the states of Georgia (55,870 ha), Texas (44,534 ha), Oklahoma (38,057 ha), New Mexico (18,623 ha), and Arizona (8,907 ha), with 40.3, 16.3, 5.1, 35.8, and 18.6 kt of nut harvested during 2021, respectively (82). The historical particularities of pecan distribution in the USA were well noted by Hall (83) who tracked the location of native and improved varieties of pecan (Figure 2). According to the latter assessment and the Global Soil Organic Carbon Map (84), pecans located in the two most planted states have a significant role in keeping carbon accounts beneficial for the environment since the soils beneath them are not well provided of C (Figure 2), if compared to other regions. For example, in Georgia, pecans are planted in a zone where SOC is 10–20 t ha-1 in the top 30 cm soil layer (Figure 3A), while, in Texas, they are planted at soils with 20–60 t C ha-1 in the same soil layer (Figure 3B); however, as the orchards age, their benefits grow more tangible, with the fine root mass supplying more C to the soil as noted by Lee and Jose (17). Additionally, the importance of roots as providers of C-derived energy for shoot growth must also be remarked since their role is crucial to designing best management practices to mitigate the effects of alternate bearing on nut yield (85). Associated to this alternate bearing is the husk production pulse, which may influence C return to the soil every year since husks are rich in carbohydrates and fiber (64).
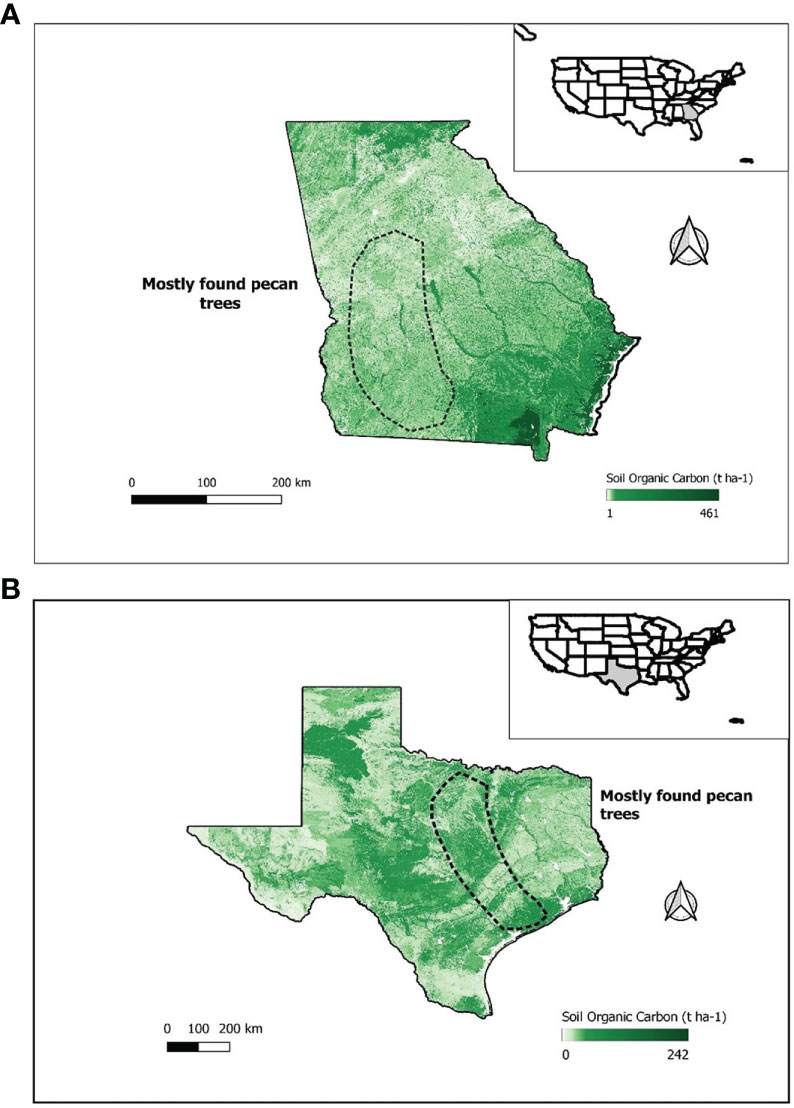
Figure 3 Soil organic carbon (SOC) and pecan’s most frequently planted area according to Hall (83) for (A) Georgia and (B) Texas states. Source: own elaboration in QGis v. 3.26.2 (2022) based on the Global Soil Carbon Map (84). The map in the upper right indicates the location of the states in the USA.
The previously mentioned cycling is weak in newly planted orchards but grows stronger as the orchard ages and eventually interrupted when the orchard is put off production. Consequently, abandoned and newly planted orchards are relevant to build a C stock inventory since most of sequestered C is in the plant biomass. According to Wells (86), between 2010 and 2014, 391,448 pecan trees were planted in Georgia, which, to date, means a pool of 8- to 12-year-old trees storing 5.6–23.1 kt C aboveground if we consider an annual trunk diameter growth of 12 mm and an exponential relationship between trunk diameter and aboveground biomass (87, 88). A gross 18% of that aboveground C (16) is associated with nut production (including nutshells) and necessary to densify the essential nutrients into the nut kernel. Those depositions may also offset methane and nitrous oxide emissions that occur after irrigation (89).
From a nutritional perspective, the entering of new orchards into production satisfies an increasing demand and interest of consumers for pecans. On a kernel basis, the USA produces nearly 60 kt of nuts every year, out of which 41% are for export and the rest covers an annual consumption of 293 g per capita (13) if the total population is considered. This per capita annual consumption is closer to that suggested by Food and Agriculture Organization of the United Nations (FAO) (90) for nuts overall (2.13 kg per capita) than the current global consumption of pecan (34 g per capita) (13). However, under the parameters of the last Global Burden of Disease, a healthy diet must include 25 g of either nut in the per capita dietary daily intake (9.12 kg on an annual basis), which would reduce global CO2 emissions between 3.8% and 23.1% compared to 2010, contributing significantly toward achieving SDG 2 (4).
4.1.2 Pecans in Mexico
In Mexico, pecan production is centralized in the north of the country, being the states of Chihuauhua (89,188 ha), Coahuila (20,880 ha), Sonora (19,536 ha), Durango (6,252 ha), and Nuevo León (4,173 ha) with remarkable nut production (77.8, 18.8, 18.4, 9, and 4.3 kt of harvested nut, respectively) (Herrera-Aguirre, pers. comm.). The importance of the pecan orchards as a carbon sink is even more relevant provided that the top 30 cm soil layer has SOC ranging from 1 to 105 t C ha-1 across the pecan-producing states (Figure 4). According to Lopez and Esteva (91), Chihuahua and Durango have their pecan orchards mostly planted in the east, precisely where soils are less C provided (4–30 t C ha -1). Unlike the former states, Coahuila and Sonora have pecan production less concentrated and pecan orchards can be located at any point in the state (91). Knowing the distribution of the orchards allows for contextualizing the production for future trends. Considering the projection of climate change for Mexico, accounting with a plastic, adaptable woody species such as pecan is important provided that variables such as frost-free days and the number of hot nights are expected to increase within the next years, particularly in Chihuahua and Coahuila (92).
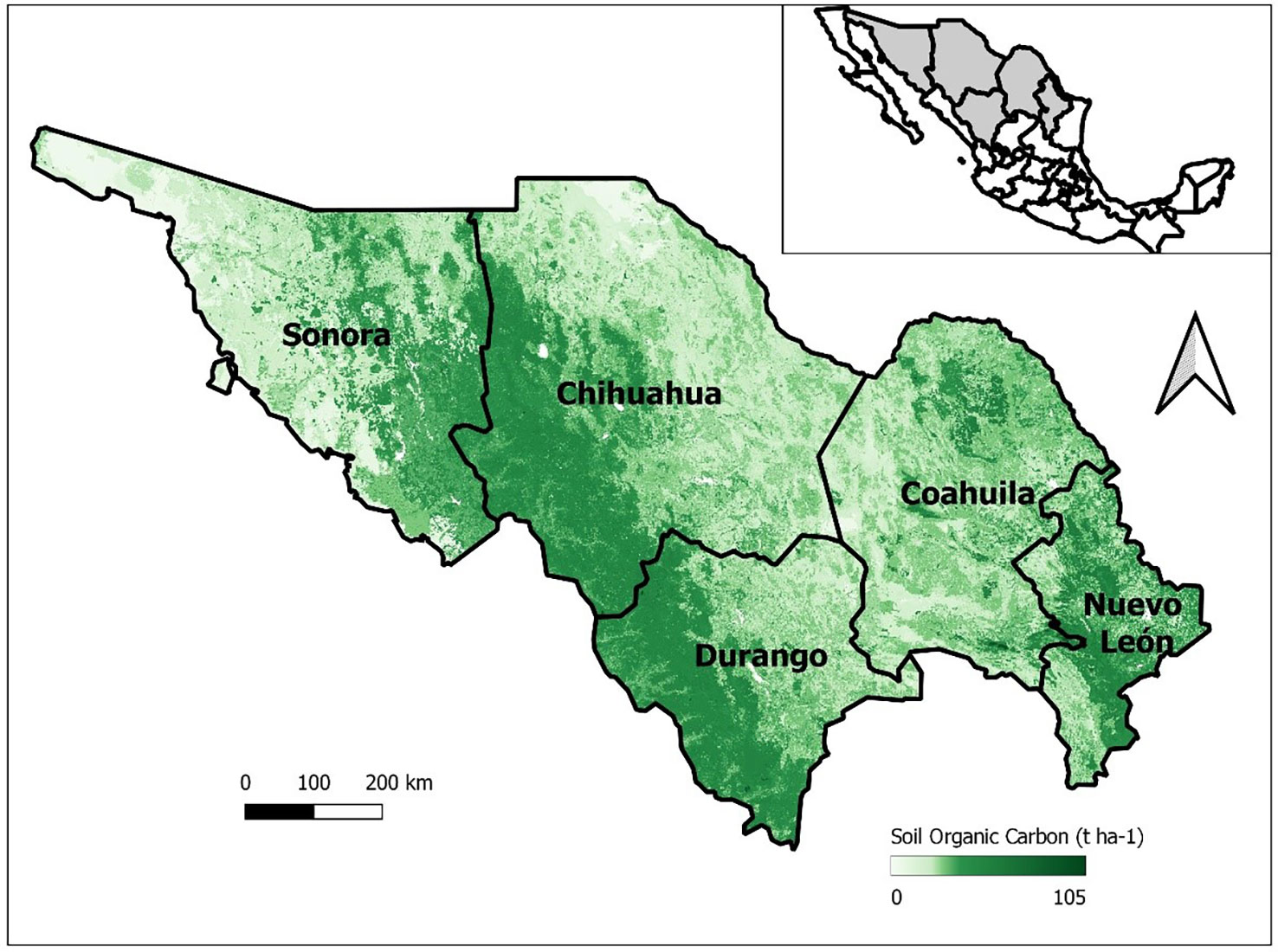
Figure 4 SOC for the Mexican states of Chihuahua, Coahuila, Durango, Nuevo León, and Sonora. Source: own elaboration in QGis v. 3.26.2 (2022) based on the Global Soil Carbon Map (84). The map in the upper right indicates the location of the states in Mexico.
At the same time, the building back of SOM via the deposition of leaves, husks, and pruning residues is rather important under a scenario of low SOC and higher temperatures every year. In such a sense, from the perspective of C storage, there are many things to take into account in the Mexican context. Between 2005 and 2015, 46,938 ha were planted, resulting in plants aged from 7 to 17 years up to date (93). If we consider by a rather simple approach a homogeneous annual C sequestration rate of 9.11 t C ha-1 for mature orchards (18), an estimated pool of 204 kt C would be sequestered by 50% of the planted area during 2022 only, considering the current age strata of trees (93). Annually, this represents 68% of the C circulating the agroecosystem, out of which 29.5% remains in the trunk and branches and 41.3% returns to the soil via leaves and husks, with 13.4% stored as starch in the roots and 15.6% being harvested as nuts (16, 94).
Unlike the USA, Mexico exports a considerable amount of its domestic pecan production (65%), having an annual consumption per capita that is lower than that of the USA (16 g) and approximately half of the global mean (34 g) (13). From a nutritional point of view focused on SDG 2.2, the stimulation of domestic consumption of pecan represents an opportunity in this country since it may add diversity and balance to a daily intake mostly dominated by cereals and tubers (43.8% of the daily intake of energy) (95).
4.2 Pecans in South America: National trends, contribution to C stocks, and opportunities to match dietary needs
Unlike North America, South American countries have their pecan orchards mostly in the initial stage of implantation and production; thus, most of their trees have not yet attained the potential nut yield. Although most actions promoting the increase of planting area and nut production are being carried out in Brazil and Argentina, pecan is growing in importance in other South American countries like Uruguay and Peru.
4.2.1 Pecans in Brazil
In Brazil, the area planted with pecan is ca. 12,000 ha, with an estimated annual increase of 600 ha (Martins, pers. comm.), with most of the orchards being at a juvenile stage. The sector involves more than 1,850 growers distributed mainly in the states of Rio Grande do Sul (70%), Santa Catarina (22%), and Parana (8%) (96) (Figure 5). Brazilian pecan farmers have adopted both technology and new management techniques in order to cope with alternate bearing. The evolution of such improvements allowed the cycle 2020/21 to be the year with the highest pecan production in Brazil’s history (5,500 t) since the average annual production is ca. 4,000 t (Martins, pres. comm.). Additionally, a recent edaphoclimatic survey performed by EMBRAPA detected 2.11 M ha in Parana, 3.65 M ha in Santa Catarina, and 11.34 M ha in Rio Grande do Sul suitable for pecan production. Thus, the pecan production is expected to grow even more during the coming years. From the point of view of management, the farmers adopt conventional rather than organic management, with the average farm occupying between 2 and 15 ha, and a basically familiar type of production (97).
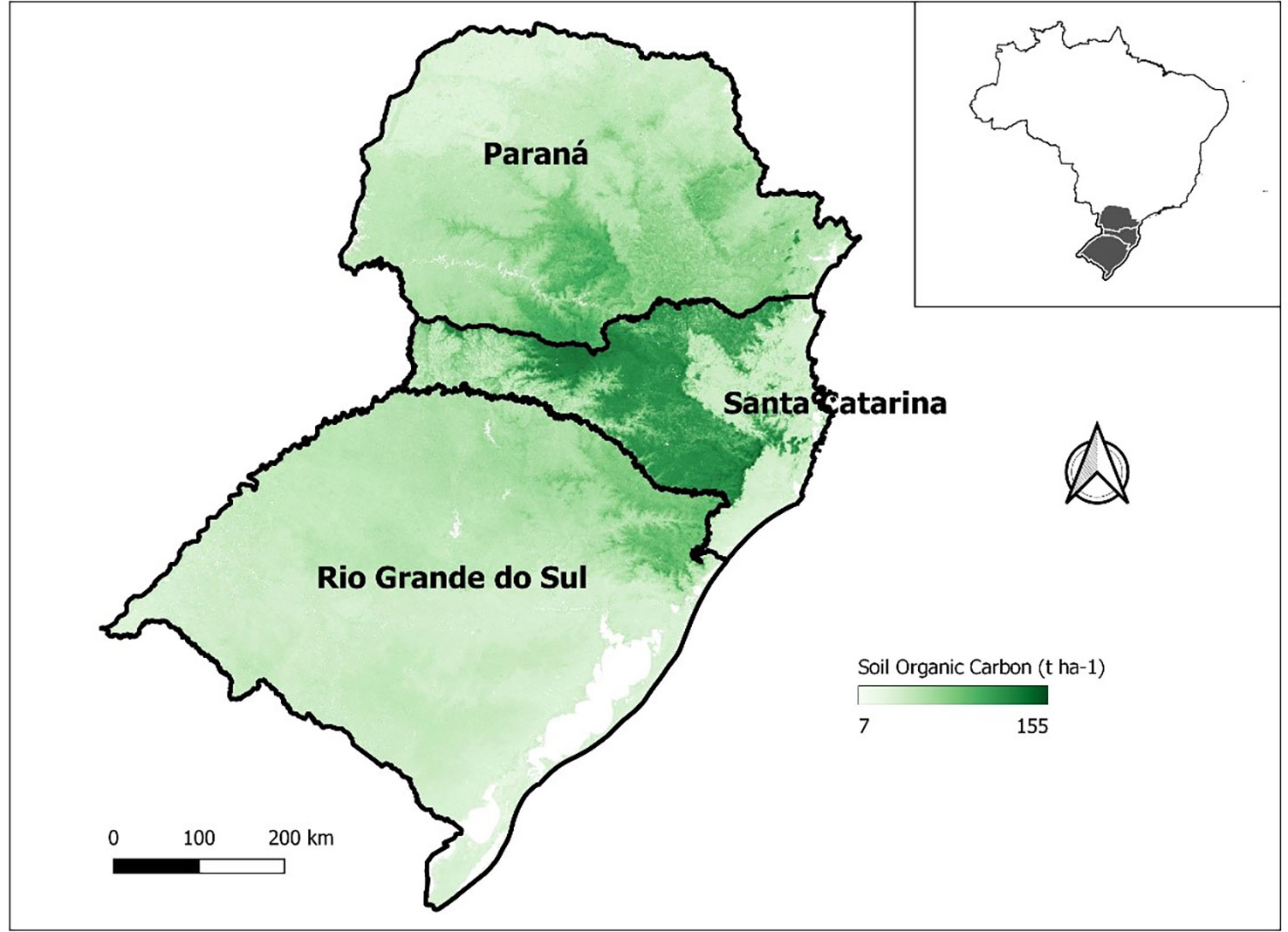
Figure 5 SOC for the Brazilian states of Rio Grande do Sul, Santa Catarina, and Paraná. Source: own elaboration in QGis v. 3.26.2 (2022) based on the Global Soil Carbon Map (84). The map in the upper right indicates the location of the states in Brazil.
The state of Rio Grande do Sul has its orchards located at the center-west (97), where soils have an average of 40 t C ha-1 (Figure 5), resembling Texas’ soil conditions. Although orchards are mostly cultivated standalone, they complement crops such as tobacco (Nicotiana tabacum), rice (Oryza sativa), beans (Phaseolus vulgaris), soybeans (Glycine max), and cassava (Manihot esculenta) and are eventually integrated into either beef or dairy cattle production, resulting in silvopastoral systems (97). On one hand, given the agricultural landscape is dominated by the former-mentioned highly extracting and GHG-emitting crops, the pecan orchards constitute C sinks to offset these externalities. On the other hand, from the nutritional perspective, the domestic consumption of pecan nuts is also low (8 g per capita year-1) when the total population is considered, but it 10-folds that amount if domestic consumption is weighed by the estimated percentage of usual nuts consumers (13). Given its nutritional benefits, the increase in pecan consumption may help to attain SDG 2.2 and 2.4 in Brazil since 28.9% of the population is subjected to the prevalence of moderate to severe food insecurity and 22.1% of the population older than 18 suffer from being overweight with the risk of cardiovascular disease (95).
4.2.2 Pecans in Argentina
In Argentina, the pecan orchards are mostly concentrated in the provinces of Entre Ríos and Buenos Aires (64% of the national area), with nearly 8,000 ha planted to date (98). Within these provinces, the regions more climatically suitable to plant pecan are the Delta of the Paraná River, the Northeast of Buenos Aires, and the whole Entre Ríos province, although there is a small region in the Southeast of Buenos Aires where pecan may thrive (99) (Figure 6). These three regions have different ranges in SOC; while the Delta Region exhibits 85–142 t C ha-1, Entre Ríos, Northeast of Buenos Aires, and Southeast of Buenos Aires have their orchards located at soils where SOC ranges 48–82, 41–89, and 82–106 t C ha-1, respectively (Figure 6). Unlike Brazil, most orchards in Argentina are located in areas relatively well provided with SOC. Thus, in this case, pecan orchards may play a significant role in counterbalancing the CO2 released by extensive agriculture.
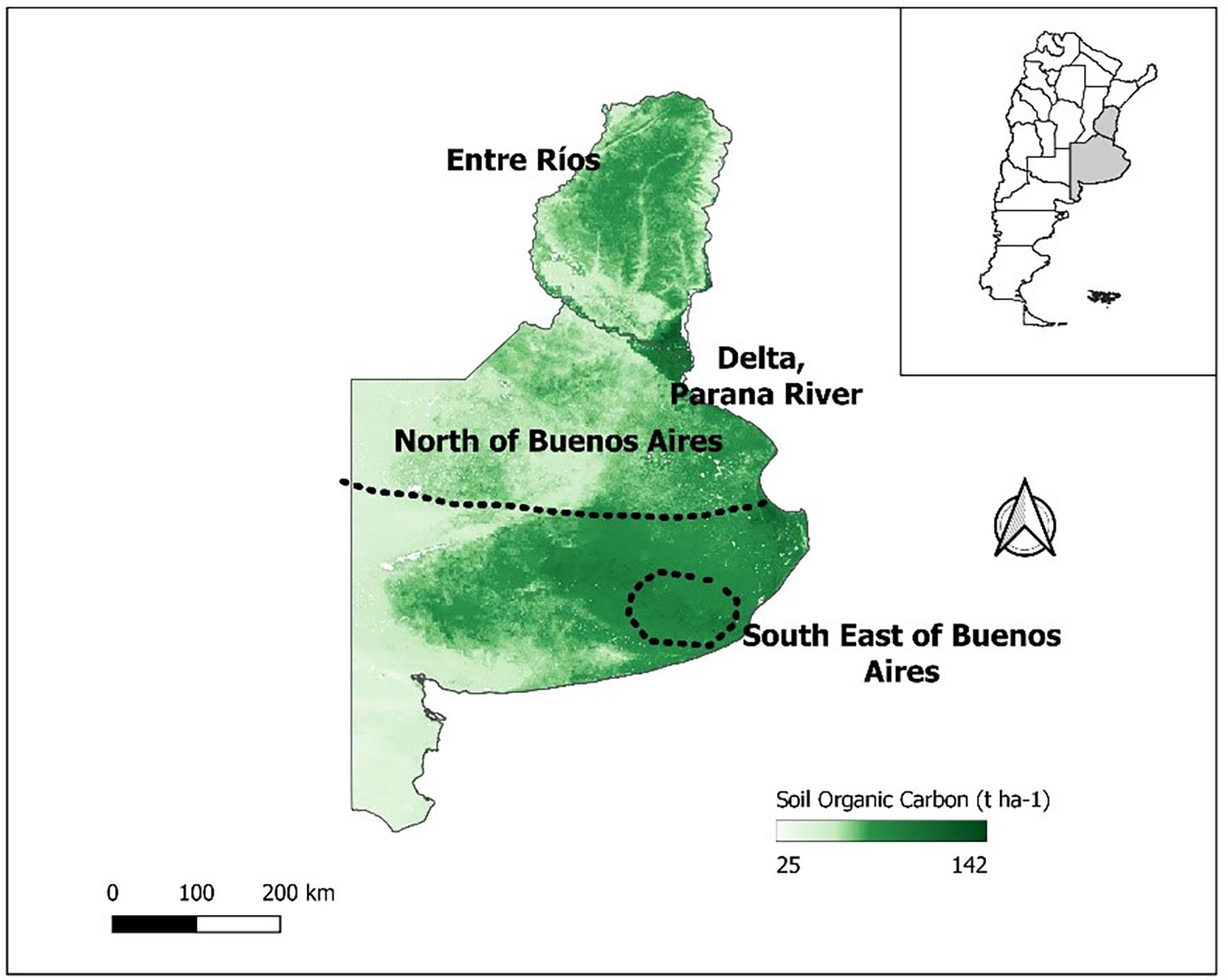
Figure 6 SOC for the provinces of Entre Ríos and Buenos Aires. Source: own elaboration in QGis v. 3.26.2 (2022) based on the Global Soil Carbon Map (84). Dotted lines indicate the most suitable area to plant pecan (99). The map in the upper right indicates the location of the provinces within Argentina.
The Argentinian pecan sector consists of 300–400 producers with most of them owning 15–50 ha, where pecan production complements their main agricultural business; however, there are a few specialized pecan companies that own up to 1,000 ha (100). The domestic market is still small, with a national per capita consumption estimated at 10 g yr-1. Unlike the USA and Mexico, pecan nut consumption in Argentina is low compared to the global mean (34 g yr-1); however, this year, Argentina launched a domestic and international pecan marketing campaign. Thus, these domestic consumption data are expected to change (101). In Argentina, the inclusion of nuts as pecan in the daily diet would help to diminish the prevalence of obesity in the 28.3% of the population older than 18, decreasing at the same time the risk of cardiovascular diseases (95).
4.2.3 Pecans in Uruguay and Peru
In Uruguay and Peru, pecan orchards are also in expansion. The surface occupied by pecan orchards in Uruguay is approximately 1,000 ha (Zoppolo, pers. comm.), with mostly 5 ha surface orchards distributed around the whole country. Planting is performed under conditions quite similar to those of the province of Entre Ríos in Argentina, with SOC ranging from 4 to 141 t ha-1 (Figure 7A). Furthermore, similarly to the previously mentioned countries of South America, most orchards planted in Uruguay can be considered still young, with the most emphasis on planting from 2009 onward, although the first plantations started around the ‘60s (102). Regardless of the latter consideration, the contribution of pecan to store C in Uruguay seems promising, even in a small area, to counterbalance methane emissions produced by beef cattle fed on rangelands. In a theoretical approach, if we consider a range of tree ages between 4 and 13 years (plantings between 2009 and 2018), with an annual surface increase of 75 ha and a starting point of 149 ha of mature trees (103), contribution to C storage during 2022 attains 3,675 t C ha-1, what equals to have permanently removed 13,475 t CO2 eq from the atmosphere during 2022. Regarding the nutritional perspective, the inclusion of pecan in the daily diet can help to correct diet imbalances observed in this country. For example, in 2016, 20.8% of Uruguayan women aged between 15 and 49 were found to suffer from anemia (95), an iron deficiency in the blood. Pecans are not only rich in iron but also in other micronutrients (Table 1); thus, the increase in pecan production for domestic consumption would help to improve such indicators leading to SDG 2.2.
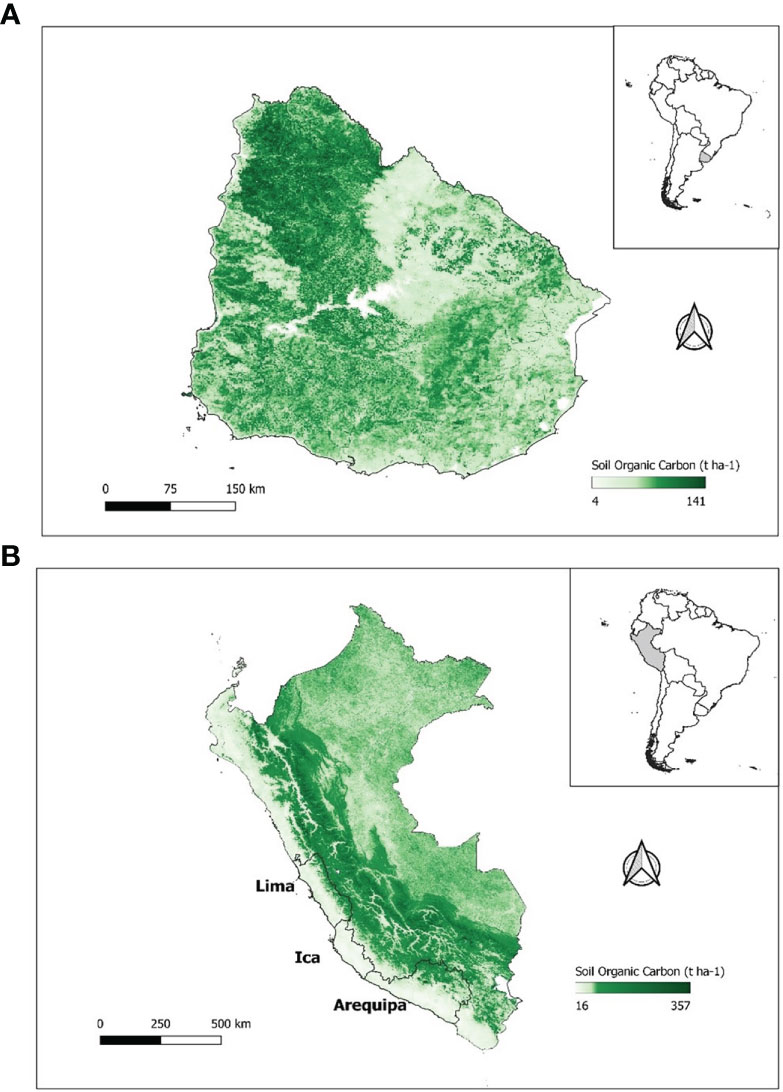
Figure 7 SOC in (A) Uruguay and (B) Peru. Source: own elaboration in QGis v. 3.26.2 (2022) based on the Global Soil Carbon Map (84).
In Peru, most of the pecan orchards are located in three departments: Ica (1,820 ha), Lima (400 ha), and Arequipa (130 ha) (Figure 7B), with ‘Mahan’ being the main cultivar planted (García, pers. comm.). The soil beneath these orchards has a shorter range than in the previously mentioned South American countries, with the department of Ica having the lowest SOC (18–50 t C ha-1). Under that context, pecan orchards represent a unique opportunity to build SOC and resilience. Despite this, the information about pecan in Peru has not yet been published in scientific papers; rather, it results from empirical data and consultant agronomists’ experience.
4.3 Contribution of pecan to carbon storage in the Americas
As repeatedly mentioned earlier, pecan trees represent a singular sink for atmospheric C; however, the literature seems to have overlooked the global dimension of this ecosystemic service for this edible fruit tree. Given this knowledge gap and through a rather simplistic method, we made the first approach by gathering the data of planted area during the period 2012–2022 for all the Americas’ countries only excluding Peru due to the scarcity of temporal data. The planted area was multiplied by an estimated mean tree density of 70 trees ha-1, and then, the mature pecan tree population by country was weighed by a biomass of 1.2 t tree-1 year-1 and a gain of 0.2 t year-1 tree-1 for mature trees (104). Immature trees were considered to have 50% of mature tree biomass and 50% of the yearly gain in biomass. Carbon stored in biomass was considered to be half the biomass, and CO2 equivalents removed from the atmosphere were calculated by multiplying C stored in biomass by 44 and dividing it by 12.
The results shown in Table 3 reflect a statistical indicator that has been neglected in the bibliography so far: the existence of ca. 23 million pecan trees in the Americas (excluding Peru), storing 14 Mt C in their biomass or, what is the same, removing 51.3 Mt CO2eq from the atmosphere. However, this is only part of the story since, if we consider a mean annual C sequestration rate of 9.11 t C ha-1 yr-1 (18) for mature trees only, including the vegetation surrounding pecan, and integrate the planted area from 2012 to 2022, the cumulative stored C in the system (pecan biomass + soil) during that period results in 21.92 Mt C, having removed 80.36 Mt CO2eq, with mature orchards occupying 72.3%–77.3% of the planted area in the Americas for the considered period. This denotes not only the environmental potential of pecan orchards as C sinks alone but also a hypothetical increase in C sequestration when integrated into other activities, for example, silvopastoral systems or alley crops. Although we found some studies on pecan pioneering with calculations of this sort (16, 94), future studies should provide friendly tools for pecan farmers to be able to calculate environmental gains in their orchards.
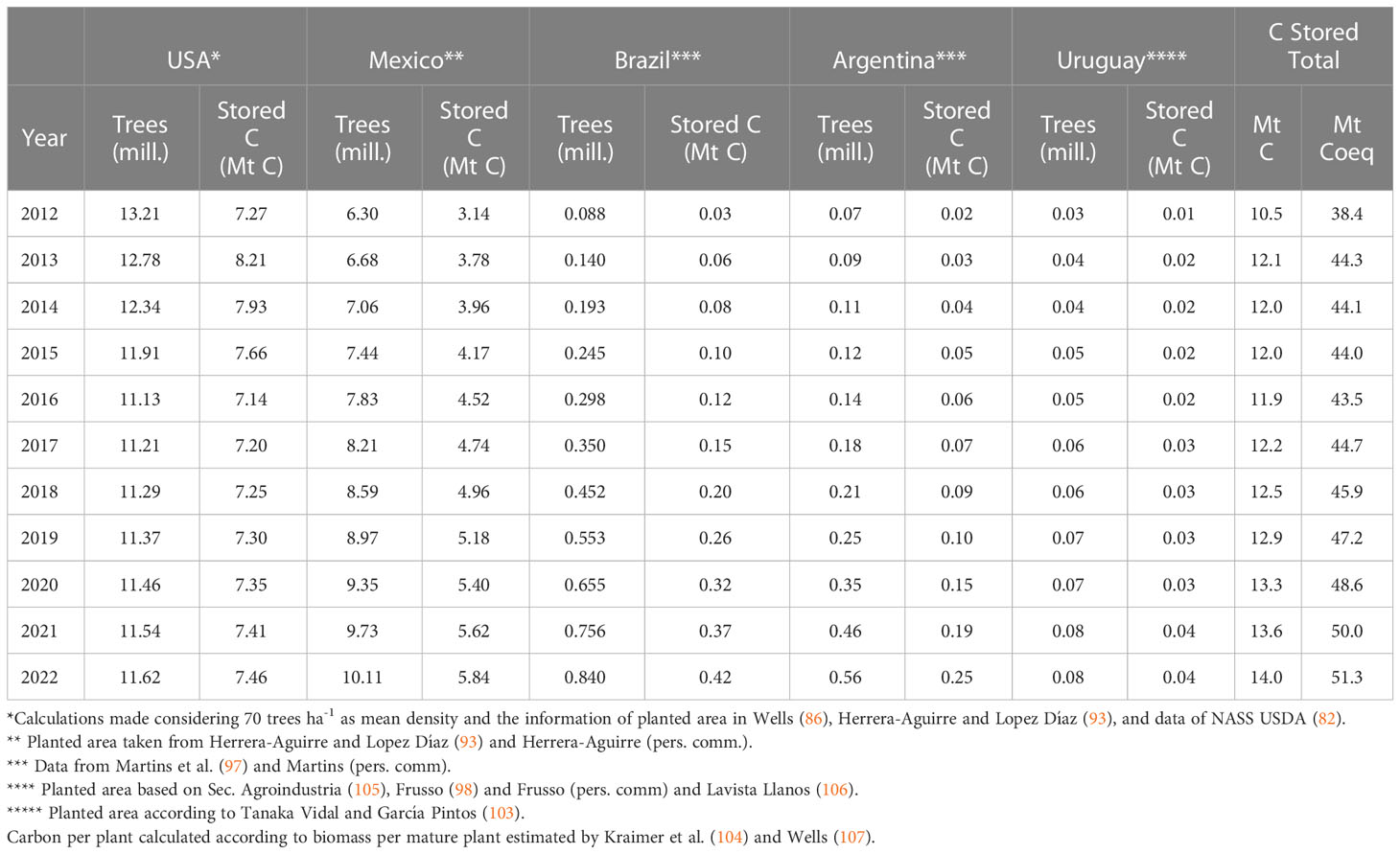
Table 3 Tree population and C stored in pecan trees’ biomass during the period 2012-2022 for USA, Mexico, Brazil Argentina and Uruguay.
5 Pecan as a platform to integrated sustainable systems
Agroforestry has been defined as a combination of woody plants with either crop (silvoarable systems) or cattle (silvopastoral systems), aiming to capture the economic and ecological benefits of such interaction (108). In such a sense, pecan orchards fit perfectly with that definition since they may be integrated not only to winter crops such as wheat and barley due to its deciduous nature but also to beef or dairy cattle given the possibility of seeding high-quality pastures between plant rows. Therefore, pecan orchards can provide a platform to be combined with either agri-food system, leading to integrated sustainable systems (ISS). When the query “agroforestry” is introduced in the Scopus science browser for the period 2011–2020, 5,965 articles were found, with half of the articles written in nine countries: USA, Brazil, India, Germany, Indonesia, China, France, the United Kingdom, and Spain (109). If the search is refined to tree nuts, the total of studies produced during that period is 27, out of which 4 were related to pecans.
In such a sense, there are recent standalone studies that evaluated the economic and environmental dimensions of pecan as integrated into other systems. On one hand, Moore et al. (110) determined the economic value of sustaining a silvopastoral system compound by native pecan and stocker cattle grazing for 90 days and found that mutually beneficial. On the other hand, other studies demonstrated that integrating cover crops into pecans enhanced the prevalence of mycorrhizal fungi, with ectomycorrhizal fungi located in the tree rows and arbuscular mycorrhizal fungi located in the alleys, both boosting SOC (111). The novel study supported the evidence found by Kremmer and Kussman (112) on the effects of cover crops on soil quality under a pecan orchard. Additionally, Cabrera-Rodriguez et al. (113) found that, under an organically managed pecan orchard, the capture of recalcitrant carbon was supported by a greater diversity of bacterial communities, while, in a conventionally managed orchard, SOC mineralization and enzymatic activity to metabolize labile C were stronger. In terms of circularity, Noperi-Mosequeda et al. (63) found that combined mineral and organic fertilizers support the production and preserve nut quality ranges, increasing the phenolic compounds and antioxidant capacity.
Regarding alley cropping in pecan orchards, there are studies evaluating competition for water (114) and light (115) between cotton (Gossypium hirsutum) and 50-year-old pecans in Florida with cotton alley-cropped at 23,600 seeds ha-1 within a distance of 18.3 m of spacing between tree rows. The former studies concluded that this alley crop is a valid alternative to producing lint and nuts for the southeastern USA. Additionally, Yadav et al. (116) evaluated a densely planted pecan orchard (277 pl ha-1) in the Himalayas, alley-cropped with lentil (Lens esculentum), finger millet (Eluesine coracana), wheat (Triticum aestivum), and soybean (G. max) for 7 years, concluding that although the sole cropping situation provides better yields, there are environmental gains in diversifying through alley cropping (more C) and, above all, increased returns when the global yield (grains + nuts) is considered.
As a final remark, the pecan orchard as a platform for ISS can provide many services other than economic such as nutritional diversity, carbon sequestration, and comfort for animals when integrated into animal production systems. Furthermore, this integration may help to reduce crops and cattle C footprint since, as shown in Poore and Nemececk (117), the C footprint for nuts is very small [1.61 kg CO2 eq kg nuts-1 for pecans, 118)], while beef meat C footprint rounds 60 kg CO2 eq kg meat.
6 Pecan orchards as sink for carbon sequestration in aboveground and belowground biomass
6.1 Carbon sequestration in above ground biomass
The global apprehension is that carbon management should be in forests to mitigate the increased concentration of greenhouse gases in the atmosphere. However, the worldwide forest cover is diminishing fast in view of great biotic stress, industrialization, urbanization, land use changes for developmental activities, and the conversion of forests to agricultural land. The importance of terrestrial vegetation as significant sinks of atmospheric carbon dioxide and its other derivatives is highlighted under the Kyoto Protocol (119).
Pecan trees have recently drawn attention as climate-smart production systems for temperate regions as they can provide high net carbon (C) gains per area and generally occupy a relatively small fraction of the agricultural landscape (44). Pecan trees form an important component in the different production forest systems, which provide extensive ecosystem services. In this context, biomass and carbon inventory in these production systems are essential (119).
Pecans are grown in many climates under different environmental conditions, and this diversity can generate variations in the carbon sequestration in aboveground biomass between regions. In the wetter areas of the USA, pecans can grow much larger and at rates different than in the arid Southwest, simply as a result of soil moisture. Usually, the age structure of the pecan population in humid regions is older than in the West. Although this may not be good for the constant production of pecan, the longer a tree lives, the more carbon is stored in aboveground biomass (107).
Globally, the estimated range between 40 and 150 t C ha–1 is the average quantity of carbon stored in the aboveground components of agroforestry systems (19). One of the most important benefits of pecan trees is their high photosynthetic rate due to their long phenology, which increases its potential annual atmospheric CO2 capture (18). The pecan can store significant amounts of carbon in aboveground biomass for a long time. Based on the calculations of Kraimer et al. (104), a mature pecan (approximately 15 years old) has a biomass of 1.2 t per tree and accumulates approximately 0.2 t of biomass per year, depending on climatic and edaphic conditions and nutritional and cultural management (107).
Carrying out studies related to carbon sequestration in aerial biomass in pecan forests, associated with different production systems in India, the tree density of an 11-year-old C. illinoinensis orchard was 238 trees ha-1, and the total tree basal area was 6.11 m2 ha–1. The pecan orchard had a mean diameter at chest height (dbh) of 18.04 cm and a wood volume of 0.266 m3. The maximum values of biomass (56.5 t ha–1) and carbon (25.3 t ha–1) were determined (119). With annual herbaceous productions cultivated between pecan lines, these mixed productions raise the possibility of maximizing global production per surface unit, evaluating positive interactions between pecan and herbaceous crops, and controlling weed development.
Tests carried out with organic amendments recorded a significant increase in both height and diameter (Figure 8).
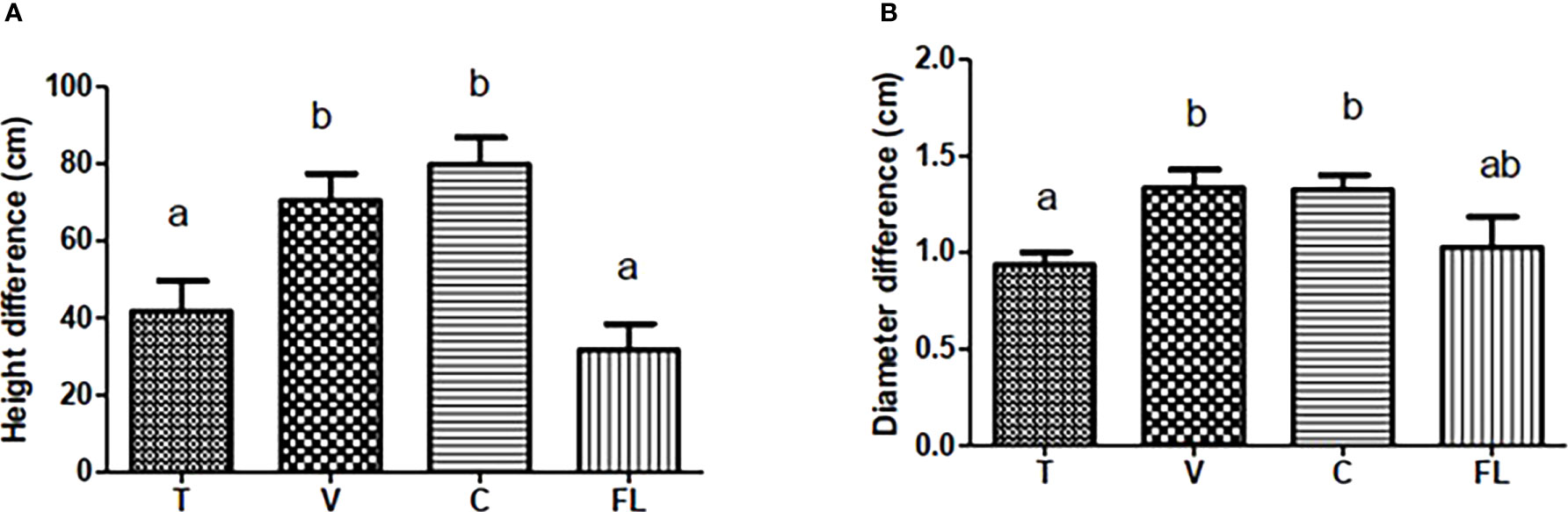
Figure 8 Mean values of control treatment (T), vermicompost (V), compost (C), and liquid fertilizer (FL). Significant differences are shown in (A, B). References: (A) differences in plant height and (B) differences in plant diameter between two consecutive years (adapted from (120)).
Timber production in pecan silvopastures is often neglected despite pecan’s wood use for cabinetmaking, paneling, furniture, and flooring (121). Most pecan timber comes from tree thinning aimed at reducing overcrowding to sustain nut production. A fundamental step towards the improvement in the management of the timber resource in pecan silvopastures is the determination of standing stocks and annual increments, thus enabling the derivation of potential extraction rates (122).
In summary, pecan orchards represent an important reservoir of C in the aerial biomass, representing a stable compartment of the CO2 fixed from the atmosphere, given the longevity of the tree and its potential timber use. In addition to the commercialization of nuts, the implementation of walnut forests is considered of great environmental and commercial value.
6.2 Belowground carbon sequestration and soil quality improvement
Pecan trees require approximately 12–15 years of growth after establishment to deliver economic yield with nuts; thus, the use of an agroforestry system where the alley between tree rows can be used with perennial crops can be used to sustain livelihood security and maintain soil cover and quality (112). In addition to the diversification of products that the agroforestry system with pecan delivers (food, fodder, fruit, pruned material such as firewood, nuts, etc.), it provides carbon sequestration, capturing CO2 from the atmosphere and storing it in the soil as organic matter–stabilized forms, while increasing productivity (116).
Agroforestry system with pecan has the potential to increase soil fertility and microbial biomass carbon due to the constant input of the deciduous leaves and root turnover to the soil in a long term (47 years). The presence of older trees also leads to a shading effect, with a reduction in soil temperature and an increase in soil moisture, fine root biomass, and soil carbon content when compared to young trees (17). Another agroforestry system with pecan in the Ozark Highlands region of northwest Arkansas, United States, that received poultry litter as organic fertilizer for 17 years increased superficial (0–15 cm) nutrient concentrations and reduced bulk density when compared to inorganic fertilization (123). That management practice was reflected in higher soil organic matter concentrations and soil carbon stock, which was also influenced by the differential decomposition of pecan leaf litter (123). In that same agroforestry system study, pecan soil that received poultry litter application got higher soil quality index (SQI) than agroforestry with red oak (124). The SQI index used soil organic carbon, pH, P, K, and bulk density as part of the methodology Soil Management Assessment Framework (SMAF), which is a quantitative soil quality evaluation tool that is used to check soil responses to management systems (125).
The pecan orchards with organic management have increased concentrations of nitrogen, microbial biomass carbon, and soil organic carbon when compared to conventional practices (113). Furthermore, the soil organic matter in the organic pecan management is more stabilized than more recalcitrant forms, reducing the CO2 released to the atmosphere, which promotes more efficiency in carbon sequestration when compared to conventional management (113). In an agroforestry system with 19-year-old pecan trees (C. illinoinensis) and bluegrass (Poa trivialis), the total soil organic carbon and total nitrogen were higher in the tree rows than in the middle of the alley, but it found no difference in the particulate organic matter and microbial biomass carbon between those sites (126). Litter quality also influences soil carbon dynamic, as found in a 21-year-old pecan and bluegrass (P. trivialis) intercrop study in which the bluegrass litter mineralized significantly higher amounts of C and decomposed 2.3 times faster than pecan litter, which has higher C:N and lignin compounds (127).
Additionally, aged, mature pecan orchards present better soil aggregate stability, mostly because of the higher levels of soil organic matter and microbial activity, which result in better soil quality when compared to row crop fields (61). Pecan roots are capable of increasing nutrient cycling and cation exchange capacity (CEC) as they can remove nitrogen from the deeper soil profiles and bring it to the upper layers and can also reduce nitrate leaching below the root zone (61). The use of a perennial cover cropping with Kura clover (a nitrogen-fixing legume) between pecan tree rows in an agroforestry system promoted a restoration of soil physical properties by rhizodeposition, which encouraged soil-stable aggregation, reducing erodibility and enhancing soil organic matter and providing economic benefits for pecan while achieving maturation (112). Deficiency in mineral nitrogen in pecans may lead to nutrient imbalance and toxicity; thus, that nutrient is very important for the growth and development of that species, which is more inclined to use –N than -N, with a ratio of 75:25 considered the most favorable for pecan seedlings (128).
As observed in this part of the review, there are some studies that assessed soil and nitrogen soil content, but few studies evaluate soil carbon stock in pecan orchards and their potential to increase it when compared to a monoculture or native vegetation. That marks a research gap in the study of the environmental aspect of pecan for climate resilience production.
7 Conclusions
Throughout this analysis, it was repeatedly observed that pecan is a reliable sink for storing atmospheric C and producing quality food with high nutritional density. The Americas has a diversity of soils where pecan orchards are planted, with SOC ranging from less favorable (Perú and México) to relatively high (Brazil, Uruguay, and Argentina). Despite these ecological differences, a population of ca. 23 M trees prevails, with the younger tree populations and the highest C-storing potential in South America. This pecan tree population has removed 51.3 Mt CO2eq, immobilizing the OC in their aboveground biomass, but, if the C sequestration for the whole system is considered, the value reaches nearly 80 Mt CO2eq in three-quarters of the planted area. Therefore, the potential of pecan orchards to store C is a research topic with an enormous prospect and is useful for designing national plans for GHG mitigation measurements.
From a nutritional perspective, there are different dietary needs to cover according to the country, although the common analysis is a low proportion of nuts in the diet, which is expected to improve, given each country’s efforts to promote domestic consumption. All the countries mentioned in this study have low pecan consumption going from 8 to 293 g per capita yr-1, which, in light of the Global Burden of Disease (4), represents 0.08%–3.2% of the recommended yearly dietary basis for nuts overall. Increasing the inclusion of pecans in the diet is the most important method to cope with the food nutrient dilution produced by the effect of atmospheric CO2 fertilization.
The third remark goes about pecan orchards’ function as a platform to integrate sustainable systems. Even though the studies found are still few, the global benefit of having pecan and alley crops has been proven in regions other than the Americas with interesting economic outputs, leading to energizing the life of rural communities. In America, we found several studies about the integration between pecan orchards and cotton, although all of them performed at the level of competition by resources (water and light) between species. We also found one study about the profitability of combining pecan and stocker cattle.
Finally, pecan orchards and pecan agroforestry may lead to sustainable agri-food systems, with global gains in SOC and nutritional richness and diversity. Therefore, more in-depth studies related to C cycling in the orchard, links between the nutritional composition of pecan nuts, and tools to calculate C budget are needed to not only fully understand the functioning of the systems at the productive level but also allow stakeholders to design and plan sustainable pecan orchards in rural areas.
Author contributions
GC took the lead in writing and shaping the manuscript. EF revised critically the manuscript and provided the contact of the other authors. EH-A revised critically the manuscript and significantly contributed to Section 4. RZ revised critically the manuscript and significantly contributed to Section 3. FL significantly contributed to Sections 2.1 and 6.2. MB significantly contributed to Section 2.2. CMa significantly contributed to Section 4.2. CMe significantly contributed to Section 6.1. All authors contributed to the article and approved the submitted version.
Acknowledgments
The authors wish to thank Catherine Clark for her valuable suggestions and English proofreading.
Conflict of interest
CMa was employed by the company Embrapa. The remaining authors declare that the research was conducted in the absence of any commercial or financial relationships that could be construed as a potential conflict of interest.
Publisher’s note
All claims expressed in this article are solely those of the authors and do not necessarily represent those of their affiliated organizations, or those of the publisher, the editors and the reviewers. Any product that may be evaluated in this article, or claim that may be made by its manufacturer, is not guaranteed or endorsed by the publisher.
References
1. Bhattacharyya SS, Leite FFGD, Adeyemi MA, Sarker AJ, Cambareri GS, Faverin C, et al. A paradigm shift to CO2 sequestration to manage global warming–with the emphasis on developing countries. Sci Total Environ (2021) 790:148169. doi: 10.1016/j.scitotenv.2021.148169
2. Wiesmeier M, Mayer S, Burmeister J, Hübner R, Kögel-Knabner I. Feasibility of the 4 per 1000 initiative in Bavaria: A reality check of agricultural soil management and carbon sequestration scenarios. Geoderma (2020) 369:114333. doi: 10.1016/j.geoderma.2020.114333
3. Soussana JF, Lutfalla S, Ehrhardt F, Rosenstock T, Lamanna C, Havlík P, et al. Matching policy and science: Rationale for the ‘4 per 1000-soils for food security and climate’initiative. Soil Tillage Res (2019) 188:3–15. doi: 10.1016/j.still.2017.12.002
4. Willet W, Rockström J, Loken B, Springmann M, Lang T, Vermeulen S, et al. Food in the anthropocene: the EAT–lancet commission on healthy diets from sustainable food systems. EAT Lancet (2019) 393(10170):447–92. doi: 10.1016/S0140-6736(18)31788-4
5. Grosso G, Mateo A, Rangelov N, Buzeti T, Birt C. Nutrition in the context of the sustainable development goals. Eur J Public Health (2020) 30(Supplement_1):i19–23. doi: 10.1093/eurpub/ckaa034
6. Davis DR. Declining fruit and vegetable nutrient composition: What is the evidence? HortScience (2009) 44(1):15–9. doi: 10.21273/HORTSCI.44.1.15
7. Köchy M, Hiederer R, Freibauer A. Global distribution of soil organic carbon–part 1: Masses and frequency distributions of SOC stocks for the tropics, permafrost regions, wetlands, and the world. Soil (2015) 1(1):351–65. doi: 10.5194/soil-1-351-2015
8. Fan M-S, Zhao FJ, Fairweather-Tait SJ, Poulton PR, Dunham SJ, McGrath SP. Evidence of decreasing mineral density in wheat grain over the last 160 years. J Trace Elem Med Bio (2008) 22:315–24. doi: 10.1016/j.jtemb.2008.07.002
9. Chen C, Riley WJ, Prentice IC, Keenan TF. CO2 fertilization of terrestrial photosynthesis inferred from site to global scales. Proc Natl Acad Sci (2022) 119(10):e2115627119. doi: 10.1073/pnas.2115627119
10. Myers SS, Smith MR, Guth S, Golden CD, Vaitla B, Mueller ND, et al. Climate change and global food systems: Potential impacts on food security and undernutrition. Annu Rev Public Health (2017) 38:259–77. doi: 10.1146/annurev-publhealth-031816-044356
11. Wolz KJ, Lovell ST, Branham BE, Eddy WC, Keeley K, Revord RS, et al. Frontiers in alley cropping: Transformative solutions for temperate agriculture. Global Change Biol (2018) 24(3):883–94. doi: 10.1111/gcb.13986
12. Bonito G, Brenneman T, Vilgalys R. Ectomycorrhizal fungal diversity in orchards of cultivated pecan (Carya illinoinensis; juglandaceae). Mycorrhiza (2011) 21:601–12. doi: 10.1007/s00572-011-0368-0
13. International Nut and dried fruit Council (INC). INC nuts & dried fruits statistical yearbook 2021/22 (2022). Available at: https://www.nutfruit.org/industry/technical-resources.
14. Food and Agriculture Organization (FAO) of the United Nations. FAOSTAT statistical database (2022). Available at: https://www.fao.org/faostat/en/#data/QCL.
15. Wallander H, Ekblad A, Bergh J. Growth and carbon sequestration by ectomycorrhizal fungi in intensively fertilized Norway spruce forests. For Ecol Manage (2011) 262(6):999–1007. doi: 10.1016/j.foreco.2011.05.035
16. Mexal JG, Herrera EA, Sammis TW, Zachritz WH. Noncommensurable values of the pecan industry. In: Guide h-654 Cooperative Extension Service College of Agriculture and Home Economics, New Mexico State University, vol. 4. (2003).
17. Lee KH, Jose S. Soil respiration and microbial biomass in a pecan–cotton alley cropping system in southern USA. Agroforestry Syst (2003) 58(1):45–54. doi: 10.1023/A:1025404019211
18. Rodríguez JC, Farias SO, Rodríguez Casas J. Potencial de secuestro de carbono y uso de agua en nogal pecanero (Carya illinoinensis) en la Costa de Hermosillo, México. Rev Nuez sureña (2021) 8–17.
19. IPCC. Guidelines for national greenhouse gas inventories (2006). Available at: http://www.ipcc-nggip.iges.or.jp/public/2006gl/index.html (Accessed 28 August 2022).
20. Friedlingstein P, O'sullivan M, Jones MW, Andrew RM, Hauck J, Olsen A, et al. Global carbon budget 2020. Earth Syst Sci Data (2020) 12(4):3269–340. doi: 10.5194/essd-12-3269-2020
21. Sanderman J, Hengl T, Fiske GJ. Soil carbon debt of 12,000 yea-rs of human land use. Proc Natl Acad Sci (2017) 114(36):9575–80. doi: 10.1073/pnas.1706103114
22. Gibbs HK, Salmon JM. Mapping the world's degraded lands. Appl Geogr (2015) 57:12–21. doi: 10.1016/j.apgeog.2014.11.024
23. Rumpel C, Amiraslani F, Koutika LS, Smith P, Whitehead D, Wollenberg E. Put more carbon in soils to meet Paris climate pledges. Nature (2018) 564:32–4. doi: 10.1038/d41586-018-07587-4
24. Rogelj J, Den Elzen M, Höhne N, Fransen T, Fekete H, Winkler H, et al. Paris Agreement climate proposals need a boost to keep warming well below 2 c. Nature (2016) 534(7609):631–9. doi: 10.1038/nature18307
25. Chenu C, Angers DA, Barré P, Derrien D, Arrouays D, Balesdent J. Increasing organic stocks in agricultural soils: Knowledge gaps and potential innovations. Soil Tillage Res (2019) 188:41–52. doi: 10.1016/j.still.2018.04.011
26. Lal R. Eco-intensification through soil carbon sequestration: Harnessing ecosystem services and advancing sustainable development goals. J Soil Water Conserv (2019) 74(3):55A–61A. doi: 10.2489/jswc.74.3.55A
27. Lal R. Soil carbon sequestration and aggregation by cover cropping. J Soil Water Conserv (2015) 70(6):329–39. doi: 10.2489/jswc.70.6.329
28. de Moraes Sá JC, Lal R, Cerri CC, Lorenz K, Hungria M, de Faccio Carvalho PC. Low-carbon agriculture in south America to mitigate global climate change and advance food security. Environ Int (2017) 98:102–12. doi: 10.1016/j.envint.2016.10.020
29. Cusack DF, Kazanski CE, Hedgpeth A, Chow K, Cordeiro AL, Karpman J, et al. Reducing climate impacts of beef production: A synthesis of life cycle assessments across management systems and global regions. Global Change Biol (2021) 27(9):1721–36. doi: 10.1111/gcb.15509
30. Vibart R, de Klein C, Jonker A, van der Weerden T, Bannink A, Bayat AR, et al. Challenges and opportunities to capture dietary effects in on-farm greenhouse gas emissions models of ruminant systems. Sci Total Environ (2021) 769:144989. doi: 10.1016/j.scitotenv.2021.144989
31. Renan Besen M, Henrique Ribeiro R, Rigo Monteiro ANT, Seiki Iwasaki G, Piva JT. Práticas conservacionistas do solo e emissão de gases do efeito estufa no brasil. Scientia Agropecuaria (2018) 9(3):429–39. doi: 10.17268/sci.agropecu.2018.03.15
32. Cerri CEP, Cerri CC, Maia SMF, Cherubin MR, Feigl BJ, Lal R. Reducing Amazon deforestation through agricultural intensification in the cerrado for advancing food security and mitigating climate change. Sustainability (2018) 10(4):989. doi: 10.3390/su10040989
33. Piva JT, Sartor LR, Sandini IE, Moraes AD, Dieckow J, Bayer C, et al. Emissions of nitrous oxide and methane in a subtropical ferralsol subjected to nitrogen fertilization and sheep grazing in integrated crop-livestock system. Rev Bras Ciec do Solo (2019) 43:1–13. doi: 10.1590/18069657rbcs20180140
34. de Moraes A, de Faccio Carvalho PC, Anghinoni I, Lustosa SBC, de Andrade SEVG, Kunrath TR. Integrated crop–livestock systems in the Brazilian subtropics. Eur J Agron (2014) 57:4–9. doi: 10.1016/j.eja.2013.10.004
35. Nair MR, Sejian V, Silpa MV, Fonsêca VFC, de Melo Costa CC, Devaraj C, et al. Goat as the ideal climate-resilient animal model in tropical environment: revisiting advantages over other livestock species. Int J Biometeorol (2021) 65(12):2229–40. doi: 10.1007/s00484-021-02179-w
36. da Conceição HR, Reis CS, de Araujo QR. Agrobiodiversity and food safety in the agroforestry gardens at PA Mariana, camamu, bahia. Agrotrópica (2017) 29(3):227–34. doi: 10.21757/0103-3816.2017v29n3p227-234
37. Coser TR, de Figueiredo CC, Jovanovic B, Moreira TN, Leite GG, Cabral Filho SLS, et al. Short-term buildup of carbon from a low-productivity pastureland to an agrisilviculture system in the Brazilian savannah. Agric Syst (2018) 166:184–. doi: 10.1016/j.agsy.2018.01.030
38. Sacramento J. A. A. S. D., Araújo ACDM, Escobar MEO, Xavier FADS, Cavalcante ACR, Oliveira TSD. Soil carbon and nitrogen stocks in traditional agricultural and agroforestry systems in the semiarid region of Brazil. Rev Bras Ciec do Solo (2013) 37:784–95. doi: 10.1590/S0100-06832013000300025
39. Soares MB, Freddi ODS, Matos EDS, Tavanti RF, Wruck FJ, de Lima JP, et al. Integrated production systems: An alternative to soil chemical quality restoration in the cerrado-Amazon ecotone. Catena (2020) 185:104279. doi: 10.1016/j.catena.2019.104279
40. Costa MP, Schoeneboom JC, Oliveira SA, Vinas RS, de Medeiros GA. A socio-eco-efficiency analysis of integrated and non-integrated crop-livestock-forestry systems in the Brazilian cerrado based on LCA. J Cleaner Prod (2018) 171:1460–71. doi: 10.1016/j.jclepro.2017.10.063
41. Perfecto I, Vandermeer J. The agroecological matrix as alternative to the land-sparing/agriculture intensification model. Proc Natl Acad Sci (2010) 107(13):5786–91. doi: 10.1073/pnas.0905455107
42. Lavado RS, Taboada MA. The argentinean pampas: A key region with a negative nutrient balance and soil degradation needs better nutrient management and conservation programs to sustain its future viability as a world agroresource. J Soil Water Conserv (2009) 64(5):150A–3A. doi: 10.2489/jswc.64.5.150A
43. Poffenbarger HJ, Olk DC, Cambardella C, Kersey J, Liebman M, Mallarino A, et al. Whole-profile soil organic matter content, composition, and stability under cropping systems that differ in belowground inputs. Agricult Ecosyst Environ (2020) 291:106810. doi: 10.1016/j.agee.2019.106810
44. Dold C, Andrew L, Thomas . AJ, Ashworth . D, Philipp . DK, Brauer T, et al. Carbon sequestration and nitrogen uptake in a temperate silvopasture system. Nutr Cycl Agroecosyst (2019) 114:85–98. doi: 10.1007/s10705-019-09987-y
45. Gabarrón-Galeote MA, Martínez-Murillo JF, Quesada MA, Ruiz-Sinoga JD. Seasonal changes in the soil hydrological and erosive response depending on aspect, vegetation type and soil water repellency in different Mediterranean microenvironments. Solid Earth (2013) 4(2):497–509. doi: 10.5194/se-4-497-2013
46. Bastida F, Zsolnay A, Hernández T, García C. Past, present and future of soil quality indices: A biological perspective. Geoderma (2008) 147(3-4):159–71. doi: 10.1016/j.geoderma.2008.08.007
47. Beltrán M, Galantini JA, Salvagiotti F, Tognetti P, Bacigaluppo S, Sainz Rozas HR, et al. Do soil carbon sequestration and soil fertility increase by including a gramineous cover crop in continuous soybean? Soil Sci Soc America J (2021) 85(5):1380–94. doi: 10.1002/saj2.20257
48. Alvarez R, Lavado RS. Climate, organic matter and clay content relationships in the pampa and chaco soils, Argentina. Geoderma (1998) 83(1-2):127–41. doi: 10.1016/S0016-7061(97)00141-9
49. Galantini JA, Senesi N, Brunetti G, Rosell R. Influence of texture on organic matter distribution and quality and nitrogen and sulphur status in semiarid pampean grassland soils of Argentina. Geoderma (2004) 123(1-2):143–52. doi: 10.1016/j.geoderma.2004.02.008
50. Matus FJ, Maire G CR. Interaction between soil organic matter and texture and the mineralization rates of carbon and nitrogen. Agricult Técnica (2000) 60(2):112–26.
51. Alvarez R, Steinbach HS, De Paepe JL. Cover crop effects on soils and subsequent crops in the pampas: A meta-analysis. Soil Tillage Res (2017) 170:53–65. doi: 10.1016/j.still.2017.03.005
52. Beltrán MJ, Sainz-Rozas H, Galantini JA, Romaniuk RI, Barbieri P. Cover crops in the southeastern region of Buenos Aires, Argentina: effects on organic matter physical fractions and nutrient availability. Environ Earth Sci (2018) 77(12):1–11. doi: 10.1007/s12665-018-7606-0
53. Romaniuk R, Navarro R, Beltrán M, Eiza MCM, Mousegne F. Short-term effect of the inclusion of vicia and wheat as cover crops on the availability of macro and micronutrients, and c, n and p on the different organic matter fractions. RIA Rev Investigaciones Agropecuarias (2018) 44(2):48–60.
54. Duval ME, Martinez JM, Galantini JA. Assessing soil quality indices based on soil organic carbon fractions in different long-term wheat systems under semiarid conditions. Soil Use Manage (2020) 36(1):71–82. doi: 10.1111/sum.12532
55. Restovich SB, Andriulo AE, Armas-Herrera CM, Beribe MJ, Portela SI. Combining cover crops and low nitrogen fertilization improves soil supporting functions. Plant Soil (2019) 442(1):401–17. doi: 10.1007/s11104-019-04205-8
56. Costantini EA, Branquinho C, Nunes A, Schwilch G, Stavi I, Valdecantos A, et al. Soil indicators to assess the effectiveness of restoration strategies in dryland ecosystems. Solid Earth (2016) 7(2):397–414. doi: 10.5194/se-7-397-2016
57. Gil JDB, Garrett R, Berger T. Determinants of crop-livestock integration in Brazil: Evidence from the household and regional levels. Land Use Policy (2016) 59:557–68. doi: 10.1016/j.landusepol.2016.09.022
58. Lorenz K, Lal R. Soil organic carbon sequestration in agroforestry systems. A review Agron Sustain Dev (2014) 34(2):443–54. doi: 10.1007/s13593-014-0212-y
59. Mayer S, Wiesmeier M, Sakamoto E, Hübner R, Cardinael R, Kühnel A, et al. Soil organic carbon sequestration in temperate agroforestry systems–a meta-analysis. Agricult Ecosyst Environ (2022) 323:107689. doi: 10.1016/j.agee.2021.107689
61. Slade H, Wells L. Soil quality enhancement with orchard age in pecan orchards of the southeastern US coastal plain. HortScience (2022) 57(9):1099–105. doi: 10.21273/HORTSCI16685-22
62. Idowu OJ, Sanogo S, Brewer CE. Short-term impacts of pecan waste by-products on soil quality in texturally different arid soils. Commun Soil Sci Plant Anal (2017) 48(15):1781–91. doi: 10.1080/00103624.2017.1395448
63. Noperi-Mosqueda LC, Soto-Parra JM, Sánchez E, Piña-Ramírez FJ, Pérez-Leal R, Flores-Córdova MA, et al. Impact of organic and mineral fertilization in pecan nut on production, quality and antioxidant capacity. Agric Sci (2019) 10(2):227–40. doi: 10.4236/as.2019.102019
64. Corral-Escárcega MC, Ruiz-Gutiérrez MG, Quintero-Ramos A, Meléndez-Pizarro CO, Lardizabal-Gutiérrez D, Campos-Venegas K. Use of biomass-derived from pecan nut husks (Carya illinoinensis) for chromium removal from aqueous solutions. column modeling and adsorption kinetics studies. Rev Mexicana Ingeniería Química (2017) 16(3):939–53.
65. UN-OCHA, United Nations Office for the Coordination of Humanitarian Affairs. Global report on food crises. joint analysis for better decisions (2022). Available at: https://reliefweb.int/report/world/global-report-food-crises-2022 (Accessed 9/6/2022).
66. Braesco V, Ros E, Govindji A, Bianchi C, Becqueriaux L, Quick B. A slight adjustment of the nutri-score nutrient profiling system could help to better reflect the European DietaryGuidelines regarding nuts. Nutrients (2022) 14(13):2668. doi: 10.3390/nu14132668
67. Ferrari V, Gil G, Heinzen H, Zoppolo R, Ibáñez F. Influence of cultivar on nutritional composition and nutraceutical potential of pecan growing in Uruguay. Front Nutr (2022) 9:868054. doi: 10.3389/fnut.2022.868054
68. Curiel-Maciel NF, Arreola-Ávila JG, Esparza-Rivera JR, Luna-Zapién EA, Minjares-Fuentes JR, Sierra-Campos E, et al. Nutritional quality, fatty acids content and antioxidant capacity of pecan nut fruits from criolla and improved walnut varieties. Notulae Botanicae Horti Agrobotanici Cluj-Napoca (2021) 49(2):1–9. doi: 10.15835/nbha49212021
69. Ozdemir M, Agsaran B, Gubbuk H, Kafkas E. Comparison of some pomological characteristics, fat and fatty acid composition of some pecan (Carya illinoensis) cultivars. Acta Hortic (2021) 1318:209–14. doi: 10.17660/ActaHortic.2021.1318.30
70. Binici SA, Muradoglu F, Ak BE, Akkus G. Determination of pomological and biochemical features of some pecan nut cultivars which are grown in the southeastern Anatolia region. Acta Hortic (2020) 1299:295–300. doi: 10.17660/ActaHortic.2020.1299.44
71. Bouali I, Tsafouros A, Ntanos E, Albouchi A, Boukhchina S, Roussos PA. Inter-cultivar and temporal variation of phenolic compounds, antioxidant activity and carbohydrate composition ofpecan (Carya illlinoinensis) kernels grown in Tunisia. Horticult Environ Biotechnol (2020) 61(1):183–96. doi: 10.1007/s13580-019-00188-8
72. Poletto T, Poletto I, Moraes Silva LM, Brião Muniz MF, Silveira Reiniger LR, Richards N, et al. Morphological, chemical and genetic analysis of southern Brazilian pecan (Carya illinoinensis) accessions. Scientia Hortic (2020) 261:108863. doi: 10.1016/j.scienta.2019.108863
73. Reis Ribeiro S, Klein B, Machado Ribeiro Q, Duarte dos Santos I, Gomes Genro AL, de Freitas Ferreira D, et al. Chemical composition and oxidative stability of eleven pecan cultivars produced in southern Brazil. Food Res Int (2020) 136:109596. doi: 10.1016/j.foodres.2020.109596
74. Morales-de la Peña M, Rábago-Panduro LM, Martín-Belloso O, Welti-Chanes J. Challenges and benefits of using pecan kernels, derivatives, and byproducts as alternative ingredients in food product development. Food Rev Int (2021). doi: 10.1080/87559129.2021.1961269
75. Bodoira R, Maestri D. Phenolic compounds from nuts: extraction, chemical profiles, and bioactivity. J Agric Food Chem (2020) 68(4):927–42. doi: 10.1021/acs.jafc.9b07160
76. Maestri D, Cittadini MC, Bodoira R, Martínez M. Tree nut oils: Chemical profiles, extraction, stability, and quality concerns. Eur J Lipid Sci Technol (2020) 122(6):1900450. doi: 10.1002/ejlt.201900450
77. Ros E, Mataix J. Fatty acid composition of nuts – implications for cardiovascular health. Br J Nutr (2007) 96(S2):S29–35. doi: 10.1017/BJN20061861
78. Alasalvar C, Bolling BW. Review of nut phytochemicals, fat-soluble bioactives, antioxidant components and health effects. Br J Nutr (2015) 113 Suppl 2:S68–78. doi: 10.1017/s0007114514003729
79. McKay DL, Eliasziw M, Chen CYO, Blumberg JB. A pecan-rich diet improves cardiometabolic risk factors in overweight and obese adults: A randomized controlled trial. Nutrients (2018) 10(3):339. doi: 10.3390/nu1003033947
80. Naghshi S, Sadeghian M, Nasiri M, Mobarak S, Asadi M, Sadeghi O. Association of total nut, tree nut, peanut, and peanut butter consumption with cancer incidence and mortality: A comprehensive systematic review and dose-response meta-analysis of observational studies (2021). Adv Nutr (2021) 12(3):793–808. doi: 10.1093/advances/nmaa152
81. Bielecka J, Puścion-Jakubik A, Markiewicz-żukowska R, Soroczyńska J, Nowakowski P, Grabia M, et al. Assessment of the safe consumption of nuts in terms of the content of toxic elements with chemometric analysis. Nutrients (2021) 13(10):3606. doi: 10.3390/nu13103606
82. NASS-USDA. Noncitrus fruits and nuts summary 2022. Washington DC: US Department of Agriculture, National Agricultural Statistics Service (2022) p. 71–4.
83. Hall GD. Pecan food potential in prehistoric north America. Economic Bot (2000) 54(1):103–12. doi: 10.1007/BF02866604
84. Global Soil Partnership. Global soil organic carbon map – GSOCmap v.1.6. technical report. Rome: FAO (2022).
85. Rohla CT, Smith MW, Maness NO, Reid W. A comparison of return bloom and nonstructural carbohydrates, nitrogen, and potassium concentrations in moderate and severe alternate-bearing pecan cultivars. J Am Soc Hortic Sci (2007) 132(2):172–6. doi: 10.21273/JASHS.132.2.172
86. Wells L. Pecan planting trends in Georgia. HortTechnology (2014) 24(4):475–9. doi: 10.21273/HORTTECH.24.4.475
87. Smith MW, Wood BW. Pecan tree biomass estimates. HortScience (2006) 41(5):1286–91. doi: 10.21273/HORTSCI.41.5.1286
88. Ouedraogo FB, Brorsen BW, Biermacher JT, Rohla CT. Effects of pruning at planting on pecan trunk development and total shoot growth. HortTechnology (2020) 30(2):248–50. doi: 10.21273/HORTTECH04535-19
89. Kellum DS, Shukla MK, Mexal J, Deb S. Greenhouse gas emissions from pecan orchards in semiarid southern new Mexico. HortScience (2018) 53(5):704–9. doi: 10.21273/HORTSCI12773-17
90. FAOSTAT. Reference healthy diets (2022). Available at: www.fao.org/faostat.
91. Urrea Lopez R, Urzúa Esteva E. Cápitulo 1: Capítulo 1 retos y op-ortunidades en la producción de nuez pecanera en méxico. In: Reyes Vazquez N, Urrea Lopez R, editors. Retos y oportunidades para el aprovechamientode la nuez pecanera en méxico. Guadalajara, Jalisco, Mexico: Centro de Investigación y Asistencia en Tecnología y Diseño del Estado de Jalisco, A.C.(CIATEJ) (2016) 1–53.
92. Mexal J, Herrera-Aguirre E. Impactos potenciales del cambio climático en la producción de nuez en la región del noroeste de méxico y suroeste de estados unidos. TECNOCIENCIA Chihuahua (2013) 7(3):163–70.
93. Herrera-Aguirre E, Lòpez Dìaz J. Producción de nuez pecanera a nivel mundial. Revista Nogaleros (2018) 49:50–60.
94. Mexal JG, Herrera E. Servicios ambientales de árboles: énfasis en la industria del nogal pecanero. Tecnociencia Chihuahua (2014) 8(1):39–45.
95. FAO, IFAD, UNICEF, WFP, WHO. The state of food security and nutrition in the world 2022. repurposing food and agricultural policies to make healthy diets more affordable. Rome: FAO (2022). doi: 10.4060/cc0639en
96. Veinticinque N. Brazil Aims to be among the top pecan producers in the next 20 years. Pecan South Magazine Volume (2021) 54(5):6–15.
97. Martins CR, Conte A, Fronza D, Filippini Alba JM, Janner Hamann J, Gonçalves Bilharva M, et al. Situação e perspectiva da nogueira-pecã no brasil. documentos 462. empresa brasileira de pesquisa agropecuária (Embrapa) clima temperado. Pelotas, Rio Grande do Sul, Brasil: Ministério da Agricultura, Pecuária e Abastecimento (2018).
98. Frusso E. El Cultivo de pecán en la Argentina (2020). Available at: https://www.argentinaforestal.com/2020/09/28/el-cultivo-de-pecan-en-la-argentina/ (Accessed 20-09-22).
99. Conti H, Moschini R, y Cazenave G. Zonificación agroclimática del pecan en región pampeana y noreste argentino. Resúmenes la XII Reunión Argent Agrometeorología. (2008), 113–4. Available at: https://inta.gob.ar/sites/default/files/inta_040conti.pdf.
100. Veinticinque N. Argentina’s pecan industry, a thriving and growing sector. Pecan South Magazine Volume (2021) 54(1):30–5.
101. Veinticinque N. Argentina Launches domestic and international pecan marketing campaign. Pecan South Magazine Volume (2022) 55(7):20–7.
102. Fassiolo C, Zoppolo R. Alternativa para la producciòn frutìcola: Nuez pecàn. Revista INIA (2014) 38:37–42.
103. Takata Vidal V, García Pintos V. Caracterización de la nuez pecan ¨Carya illinoinensis¨ y sus perspectivas de comercialización en el Uruguay (2013). Universidad de la República. Available at: https://www.colibri.udelar.edu.uy/jspui/handle/20.500.12008/1778 (Accessed 20-09-22).
104. Kraimer RA, Lindemann WC, Herrera E. Distribution of N15-labeled fertilizer applied to pecan: A case study. [Distribución de fertilizante N15 aplicado al pecán: un estudio de caso]. HortScience (2001) 36:308–12. doi: 10.21273/HORTSCI.36.2.308
105. Secretaría de Agroindustria, Ministerio de Producción y Trabajo. Cadena de nuez pecan – resumen (2019). Available at: https://alimentosargentinos.magyp.gob.ar/HomeAlimentos/Cadenas%20de%20Valor%20de%20Alimentos%20y%20Bebidas/informes/Resumen_Cadena_NUEZ_PECAN_mayo_2019.pdf (Accessed 10-08-22).
106. Lavista Llanos A. Pecan tips: consejos prácticos para el correcto cultivo de la nuez pecán. Dunken, editor. Buenos Airea, Argentina (2020), p. 103.
107. Wells L. ¿Qué son los servicios ecosistémicos de un huerto de pecán? Rev Nuez Sureña (2021) 18–23.
108. Coello Gomez J. Los Sistemas agroforestales modernos: ¿qué son y cuál es su fundamento?, in: Dossier técnico n°99 (2019). Departamento de Agricultura, Ganadería, Pesca y Alimentación de Cataluña (DARP. Available at: https://www.researchgate.net/publication/340538678 (Accessed 05-08-22).
109. Cambareri G. Sistemas silvoarables: Es posible la diversificación del riesgo con laintegración de los frutos secos en los sistemas tradicionales? (2021). Available at: https://www.researchgate.net/publication/353983219_Sistemas_silvoarables_Es_posible_la_diversificacion_del_riesgo_con_la_integracion_de_los_frutos_secos_en_los_sistemas_tradicionales (Accessed Oct 12 2022).
110. Moore B, Johnson M, Rohla C. Pecans and cattle: Are they mutually beneficial? (2018). Available at: https://ageconsearch.umn.edu/record/266788 (Accessed 10-10-22).
111. Rodriguez-Ramos JC, Scott N, Marty J, Kaiser D, Hale L. Cover crops enhance resource availability for soil microorganisms in a pecan orchard. Agricult Ecosyst Environ (2022) 337:108049. doi: 10.1016/j.agee.2022.108049
112. Kremer RJ, Kussman RD. Soil quality in a pecan–kura clover alley cropping system in the Midwestern USA. Agroforestry Syst (2011) 83(2):213–23. doi: 10.1007/s10457-011-9370-y
113. Cabrera-Rodríguez A, Nava-Reyna E, Trejo-Calzada R, García-De la Peña C, Arreola-Ávila JG, Collavino MM, et al. Effect of organic and conventional systems used to grow pecan trees on diversity of soil microbiota. Diversity (2020) 12(11):436. doi: 10.3390/d12110436
114. Wanvestraut RH, Jose S, Nair PK, Brecke BJ. Competition for water in a pecan (Carya illinoensis k. koch)–cotton (Gossypium hirsutum l.) alley cropping system in the southern united states. Agroforestry Syst (2004) 60(2):167–79. doi: 10.1023/B:AGFO.0000013292.29487.7a
115. Zamora DS, Jose S, Nair PKR, Jones JW, Brecke BJ, Ramsey CL. Interspecific competition in a pecan-cotton alley-cropping system in the southern united states: Is light the limiting factor? In: Toward agroforestry design. Dordrecht: Springer (2008). p. 81–95.
116. Yadav RP, Bisht JK, Bhatt JC. Biomass, carbon stock under diferente production systems in the mid hills of Indian himalaya. Trop Ecol (2017) 58(1):15–21.
117. Poore J, Nemecek T. Reducing food’s environmental impacts through producers and consumers. Science (2018) 360(6392):987–92. doi: 10.1126/science.aaq0216
118. Environmental Working Group. Meat eater’s guide to climate change + health. life cycle assessments: Methodology and results (2011). Available at: https://static.ewg.org/reports/2011/meateaters/pdf/methodology_ewg_meat_eaters_guide_to_health_and_climate_2011.pdf.
119. Yadav RP, Bisht JK, Pattanayak A. Biomass production and carbon stock of agroforestry practices in the mid hills of Central Himalaya. Indian Forester (2019) 145(4):409–12.
120. Giuffré L, Romaniuk R, Ríos RP, Zubillaga MM, Rastrelli L. Sustainable management in pecan cultivation in Argentina. Emir J Food Agric (2011) 23(3):243–8.
122. Ares A, Reid W, Brauer D. Production and economics of native pecan silvopastures in central united states. Agroforestry Syst (2006) 66:205–15. doi: 10.1007/s10457-005-8302-0
123. Amorim HC, Ashworth AJ, Zinn YL, Sauer TJ. Soil organic carbon and nutrients affected by tree species and poultry litter in a 17-year agroforestry site. Agronomy (2022) 12(3):641. doi: 10.3390/agronomy12030641
124. Ylagan S, Amorim HC, Ashworth AJ, Sauer T, Wienhold BJ, Owens PR, et al. Soil quality assessment of an agroforestry system following long-term management in the Ozark highlands. Agrosyst Geosci Environ (2021) 4(3):e20194. doi: 10.1002/agg2.20194
125. Cherubin MR, Karlen DL, Franco AL, Tormena CA, Cerri CE, Davies CA, et al. Soil physical quality response to sugarcane expansion in brazil. Geoderma (2016) 267:156–68. doi: 10.1016/j.geoderma.2016.01.004
126. Mungai NW, Motavalli PP, Kremer RJ. Soil organic carbon and nitrogen fractions in temperate alley cropping systems. Commun Soil Sci Plant Anal (2006) 37(7-8):977–92. doi: 10.1080/00103620600584230
127. Mungai NW, Motavalli PP. Litter quality effects on soil carbon and nitrogen dynamics in temperate alley cropping systems. Appl Soil Ecol (2006) 31(1-2):32–42. doi: 10.1016/j.apsoil.2005.04.009
Keywords: pecan (Carya illinoensis), soil organic carbon, nutrition - topics, GHG emission, sustainability
Citation: Cambareri G, Frusso EA, Herrera-Aguirre E, Zoppolo R, Leite FFGD, Beltrán M, Martins C and Mendoza C (2023) Contribution of pecan (Carya illinoinensis [Wangenh.| K. Koch) to Sustainable Development Goal 2 under the dual perspective of carbon storage and human nutrition. Front. Soil Sci. 3:1092003. doi: 10.3389/fsoil.2023.1092003
Received: 07 November 2022; Accepted: 13 March 2023;
Published: 14 April 2023.
Edited by:
Rafael Mattos Dos Santos, University of Guelph, CanadaReviewed by:
Fatima Haque, National Taiwan University, TaiwanMiguel Angel Taboada, Faculty of Agronomy, University of Buenos Aires, Argentina
Joseph Onyango Gweyi, Kenyatta University, Kenya
Copyright © 2023 Cambareri, Frusso, Herrera-Aguirre, Zoppolo, Leite, Beltrán, Martins and Mendoza. This is an open-access article distributed under the terms of the Creative Commons Attribution License (CC BY). The use, distribution or reproduction in other forums is permitted, provided the original author(s) and the copyright owner(s) are credited and that the original publication in this journal is cited, in accordance with accepted academic practice. No use, distribution or reproduction is permitted which does not comply with these terms.
*Correspondence: Gustavo Cambareri, Y2FtYmFyZXJpLmd1c3Rhdm9AaW50YS5nb2IuYXI=