- 1Department of Geography and Environmental Science, School of Archaeology, Geography and Environmental Science, University of Reading, Reading, United Kingdom
- 2Department of Soil Science and Land Management, School of Agriculture and Agricultural Technology, Federal University of Technology, Minna, Nigeria
Introduction
During the 21st century, global mean temperature is expected to rise by 1.5°C to 5.7°C (1). Climate change has already resulted in an overall decrease in the number of cold days and nights, and an increase in the number of warm days and hot nights, across most land areas globally (2). Our changing climate will influence soil ecosystems because soils have a complex interaction with the atmosphere through carbon, nitrogen, and hydrological cycles (3). Soil is the largest terrestrial carbon pool (4–6), but it also provides a habitat for diverse and complex communities of organisms (7). Soil represents a huge potential source of volatile carbon and a potential sink for additional carbon. Soil can therefore buffer CO2 losses into the atmosphere, depending on the balance between photosynthesis, autotrophic respiration, and heterotrophic respiration (2, 8). This balance exerts major controls on the biogeochemical interactions between land and atmosphere leading to the exchange of greenhouse gases like CO2, CH4 and N2O (2), the emissions of which could cause positive feedbacks that warm our climate system (9, 10). While the response of autotrophic respiration to changing climates is relatively well understood, predicting changes to the soil carbon sink due to climate change has been a major source of uncertainty in projections. Although it is known that increasing temperature can stimulate microbial degradation of soil organic carbon and increase the atmospheric concentration of CO2 (10–12), the magnitude of this positive feedback is unclear.
Changes to the Diurnal Temperature Range (DTR)
Much research on the way that ecosystems respond to changing climate is based on the assumption that the future global warming will arise from symmetrical increases in daily maximum (daytime) and daily minimum (night-time) temperature, reflecting a commensurate increase in daily mean temperature. However, evidence from recent studies shows that daily mean temperature increases arise disproportionately due to increases daily minimum (night-time) temperatures (13). Over the last 50 years, negative trends in the Diurnal Temperature Range (DTR) have been observed due to an approximately 0.9°C increase in daily minimum and only 0.6°C increase daily maximum temperature (14). Therefore, while the climate warms, we have a reduction (i.e., narrowing or dampening) of the DTR. The decreasing DTR is attributed to decreases in sunshine duration and increases in the amount of cloud (due to the effects of aerosols), precipitation, and water vapour. However, local deviations from this global trend are expected as different regions will experience different changes in cloud cover, precipitation, and water vapour (15–18). Climate models have predicted that this trend may continue throughout the 21st century (13, 19).
The understanding that increased global annual mean temperature will increase global soil respiration rates is well documented in the literature (20). However, the impact of narrowing or decreasing the DTR on soil microbial community structure and physiological functions (such as respiration) remains largely unknown. Laboratory studies that examine how community level interactions will be influenced by climate change usually incubate mesocosms continuously at constant temperatures that mimic a possible future average temperature at the study location (13, 21). Most of these studies do not account for the influence of diurnal temperature oscillations on the temperature sensitivity of soil respiration, especially in the upper few centimetres of soils (e.g. 22). It is important to consider such temperature oscillations because short term temperature fluctuations can have important influences on processes that are driven by microorganisms like bacteria and fungi (23).
Soil respiration under oscillating temperatures and asymmetric warming
Studies examining the effects of diurnal temperature oscillations on soil respiration are rare. Zhu and Cheng (24) assessed the temperature sensitivity of soil organic carbon decomposition under a constant temperature regime and a diurnally oscillating temperature regime. Their results showed that temperature sensitivity under constant temperatures were consistently higher than those under diurnally oscillating temperatures. A possible mechanistic explanation for this observation is that microorganisms incubated under constant temperatures adapt to optimise metabolism at a particular temperature and this is exponentially faster at higher temperatures, whereas microorganisms incubated at oscillating temperatures are unable to optimise metabolism at the daily maximum temperature and thus exhibit lower temperature sensitivity. Conversely, Uvarov et al. (25) observed similar cumulative respiration between constant and oscillating temperatures in both the field and laboratory. In this case, the mechanistic explanation for this result is that the bioavailability of substrates to microorganisms (which was similar between the constant and oscillating treatments) may have mediated the respiration rate rather than the temperature regime.
In a field experiment, Xia et al. (26) reported that the effect of diurnal warming on soil respiration was not equal to the summed effects of day and night warming, even though night warming showed greater warming-induced respiration than day warming. They also demonstrated that day and night warming, using infrared radiators, influenced the daily mean soil temperature differently; the daily mean soil temperature increased more under night warming than day warming treatments. Adekanmbi et al. (27) undertook a laboratrory incubation experiment and found that the legacy effect of a pre-incubation oscillating between 5°C and 15°C was a significnatly greater respiration rate than soil pre-incubated constantly at 5°C or 10°C and was similar to soil pre-incubated constantly at 15°C, even when respiration was measured at the same temperature in all treatments. A possible mechanistic explanation for this fiding is that, assuming that extracellular depolymerisation is typically the rate limiting step in soil organic matter decomposition (28), the time spent at 15°C in the fluctuating treatment was sufficient to supply sufficient substrate for intracellular decomposition (Figure 1).
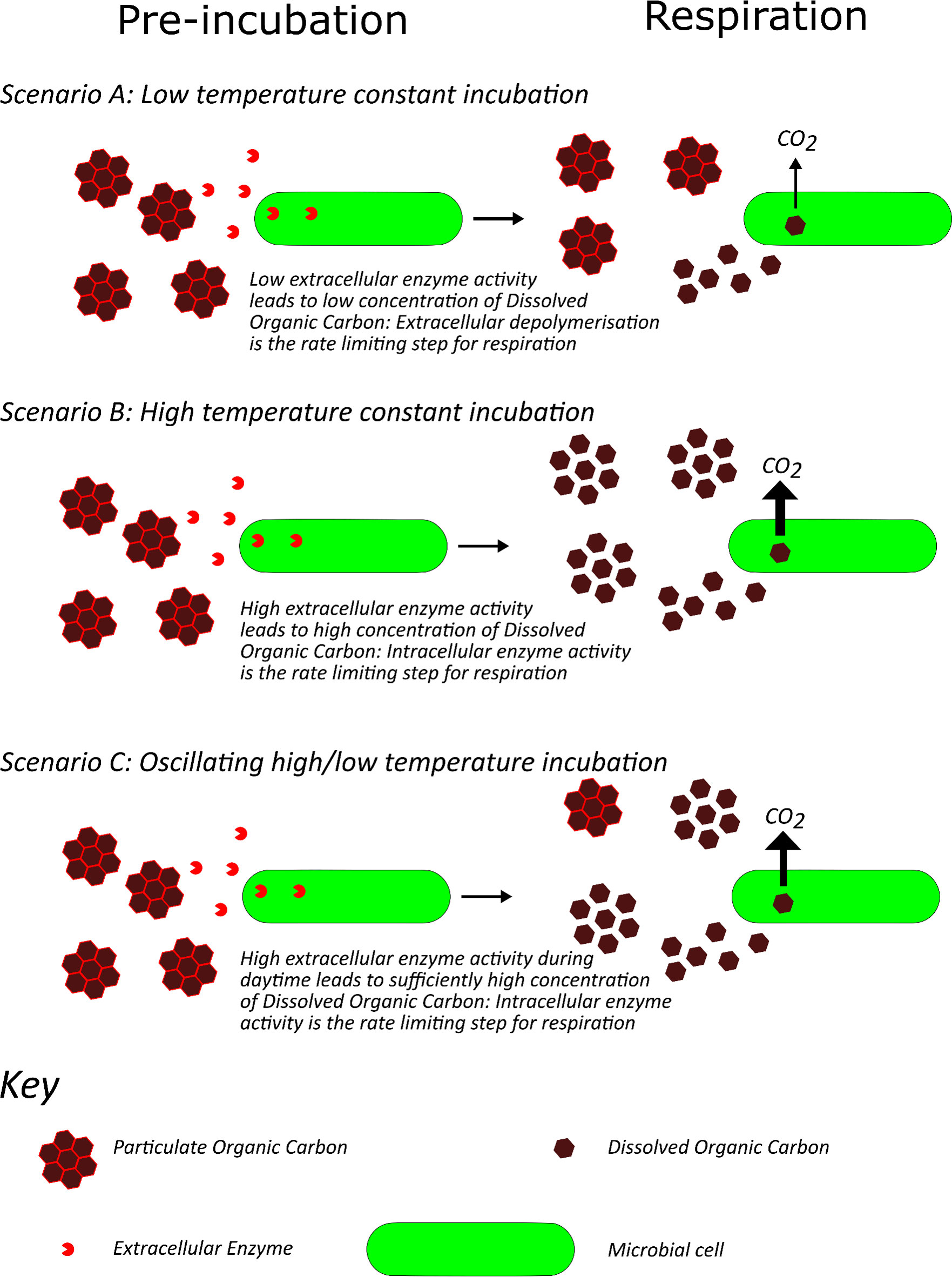
Figure 1 Possible mechanism whereby pre-incubating soils at diurnally oscillating temperatures results in similar respiration rates to pre-incubating constantly at the maximum daily temperature when respiration is measured at a given temperature. When soil is pre-incubated constantly at low temperature (Scenario A) extracellular depolymerisation is the rate limiting step for respiration at a given temperature. When soil is pre-incubated at high temperature (Scenario B) extracellular enzyme activity is sufficient to prevent extracellular depolymerisation being the rate limiting step for respiration at the given temperature. When soil is oscillated between high and low temperature (Scenario C) the time spent at high temperature may be sufficient to prevent extracellular depolymerisation being the rate limiting step for respiration at the given temperature.
Impact of land use change on soil Diurnal Temperature Range (DTR) and respiration
Although a reduction in DTR has been forecasted, due to climate change, soil temperature oscillations can also be influenced by land use and altered by land use change. Wei et al. (29) observed an overall decline in DTR between 2003 and 2013 in the agricultural pastoral ecotone of Northern China across croplands, forests, and land that changed between croplands and grasslands. However, they observed an increase in DTR when land use changed from grassland to forest. The lower DTR under grassland was as a result of both decreasing maximum (daytime) temperature and increasing minimum (night-time) temperatures (29). It has been demonstrated that soil microbes adapt to the temperature of their original site, thus expressing a legacy effect (30). Therefore, while land use change may have (well understood) direct impacts on soil respiration, it also has a poorly understood indirect impact on the temperature sensitivity of respiration due to a change in the temperature regime that soil microorganisms are exposed to. Thus, understanding the impact of diurnal temperature oscillations on the temperature sensitivity of respiration is a pre-requisite for fully understanding the influence of land use change on the soil carbon cycle.
Soil depth as an analogy for soils with different diurnal temperature ranges
The dampening of the DTR with soil depth represents an interesting analogue for the expected pattern in air or surface temperatures forecasted in future climate scenarios. For instance, de Farias et al. (31) assessed hourly, daily and monthly soil temperature from 5, 10, and 20 cm depths and found that, while soil temperatures oscillate diurnally down the profile, a decreasing amplitude in DTR was observed with depth (from 5cm to 20cm) due to higher diurnal temperature fluctuations at the surface, compared to lower layers. Whereas soil respiration is usually measured in the laboratory from samples of soil collected at a defined soil depth range, or in the field as the integrated net flux from the entire soil profile (32), there could be a variation in the temperature sensitivity of soil CO2 flux as a result of thermal diffusivity down the soil profile (33) which is not accounted for in our current understanding. However, the reasons for differential temperature sensitivity with depth also include confounding changes in biotic and abiotic conditions with soil depth. For example, the amplitude of DTR (31), texture, organic carbon (34), abundance and distribution of heterotrophic soil microbial groups (35), and enzyme activities (36) all vary with soil depth. Hicks Pries et al. (22) measured the carbon flux through the whole soil profile in response to 4°C warming and observed similar temperature sensitivity down the profile. Topsoil is where most of the organic carbon and soil microorganisms are concentrated and where the variations in DTR have the greatest impact (37). Nevertheless, soil depth may be a useful analogy for studying the influence of DTR on the temperature sensitivity of soil respiration.
Discussion
It is clear that a lack of experimental observations severely limits our ability to reach a consensus regarding the influence that diurnal oscillations have on the temperature sensitivity of soil respiration. These limitations to our current understanding mean that we are not yet in a position to predict with certainty the influence that a narrowing of the DTR will have on soil respiration. Nevertheless, predictions of the response of soil carbon fluxes to temperature change clearly do need to account for daily temperature oscillations and future changes to the DTR so that they can accurately predict the impact of climate change on positive soil carbon feedbacks to the atmosphere.
Current projections that use only the size of the soil carbon stock and mean daily temperature to predict the response of carbon loss from soil due to warming may not accurately represent the actual mechanisms and may overestimate total carbon losses [e.g. (38)]. This overestimation is because soil organic matter decomposition, carbon stocks, and persistence, are the products of processes that occur locally and there is need for more robust soil biogeochemical models that better represent how historic temperature condition and short term temperature oscillations shape the response of microbial communities and soil organic matter to warming (39). It is imperative to ensure that the next generation of land-surface models adequately simulate the impact of asymmetric warming, including daily temperature extremes, on soil microbial activity and soil heterotrophic respiration. We recommend that future projections and soil biogeochemical model parameterisation should only adopt laboratory data to estimate temperature sensitivity when the laboratory data is collected at temperatures within the typical diurnal range (or predicted diurnal range) in the region where predictions are being made. DTR could be used as an input parameter, alongside daily mean temperature, for parameterising soil carbon models.
To satisfy the next generation of soil carbon models, and their requirement to incorporate the influence of a changing DTR, there is an urgent need for laboratory data on the temperature sensitivity of soil respiration that is collected under realistic environmental conditions, including diurnal oscillations. It is understandable why laboratory incubations are currently undertaken at constant temperatures. Laboratory equipment that allows easy establishment of consistent and controllable oscillation of temperatures in the laboratory is not widely available. There will typically be a lag between a change in the temperature in an incubation chamber and the temperature in the soil incubated in a mesocosm inside the chamber, which means that measurements cannot be directly attributed to the temperature in the mesocosm at the time of the measurement. However, for laboratory experiments to represent useful analogies of phenomena that occur under field conditions, efforts need to be made to bridge the gap between uniform laboratory incubations and the stochasticity of the real-world environment.
Author contributions
TS conceived the idea for the paper. AAA wrote the first draft of the paper. TS provided edits and comments. AAA created subsequent drafts and the final draft of the paper. Both authors contributed to the article and approved the submitted version.
Funding
This work was funded by a PhD scholarship awarded to AAA by the Commonwealth Scholarship Commission. Grant number: NGCS-2017-416.
Conflict of interest
The authors declare that the research was conducted in the absence of any commercial or financial relationships that could be construed as a potential conflict of interest.
Publisher’s note
All claims expressed in this article are solely those of the authors and do not necessarily represent those of their affiliated organizations, or those of the publisher, the editors and the reviewers. Any product that may be evaluated in this article, or claim that may be made by its manufacturer, is not guaranteed or endorsed by the publisher.
References
1. Arias PA, Bellouin N, Coppola E, Jones RG, Krinner G, Marotzke J, et al. Technical summary. In Climate Change 2021: The Physical Science Basis contribution of working group I to the sixth assessment report of the intergovernmental panel on climate change (2021)Masson-Delmotte VP, Zhai A, Pirani SL, Connors C, Péan S, Berger N, et al . United Kingdom and New York, NY, USA:Cambridge University Press, Cambridge, pp. 33–144. doi: 10.1017/9781009157896.002
2. Jia GE, Shevliakova E, Artaxo P, Noblet-Ducoudré N, De Houghton R, House J, et al. Land–climate interactions. In: Shukla PR, Skea J, Buendia EC, Masson-Delmotte V, Pörtner H-O, Roberts DC, editors. Climate change and land: An IPCC special report on climate change, desertification, land degradation, sustainable land management, food security, and greenhouse gas fluxes in terrestrial ecosystems (2019), p. 131–248. https://www.ipcc.ch/site/assets/uploads/sites/4/2021/07/05_Chapter-2-V6.pdf
3. Brevik EC. Soils and climate change: Gas fluxes and soil processes. Soil Horiz (2012) 53:12. doi: 10.2136/sh12-04-0012
4. Smith P, Soussana JF, Angers D, Schipper L, Chenu C, Rasse DP, et al. How to measure, report and verify soil carbon change to realize the potential of soil carbon sequestration for atmospheric greenhouse gas removal. Glob Change Biol (2020) 26:219–41. doi: 10.1111/gcb.14815
5. Lal R, Smith P, Jungkunst HF, Mitsch WJ, Lehmann J, Ramachandran Nair PK, et al. The carbon sequestration potential of terrestrial ecosystems. J Soil Water Conserv (2018) 73:145A–52A. doi: 10.2489/jswc.73.6.145A
6. Lal R. Managing soils for negative feedback to climate change and positive impact on food and nutritional security. Soil Sci Plant Nutr (2020) 66:1–9. doi: 10.1080/00380768.2020.1718548
7. Bardgett RD, van der Putten WH. Belowground biodiversity and ecosystem functioning. Nature (2014) 515:505–11. doi: 10.1038/nature13855
8. Dungait JAJ, Hopkins DW, Gregory AS, Whitmore AP. Soil organic matter turnover is governed by accessibility not recalcitrance. Glob Change Biol (2012) 18:1781–96. doi: 10.1111/j.1365-2486.2012.02665.x
9. Crowther TW, Thomas SM, Maynard DS, Baldrian P, Covey K, Frey SD, et al. Biotic interactions mediate soil microbial feedbacks to climate change. Proc Natl Acad Sci U. S. A. (2015) 112:7033–8. doi: 10.1073/pnas.1502956112
10. Bradford MA, Wieder WR, Bonan GB, Fierer N, Raymond PA, Crowther TW. Managing uncertainty in soil carbon feedbacks to climate change. Nat Clim Change (2016) 6:751–8. doi: 10.1038/nclimate3071
11. Bardgett RD, Kandeler E, Tscherko D, Hobbs PJ, Martijn T, Jones TH, et al. Below-ground microbial community development in a high temperature world. Oikos (1999) 85:193–203. doi: 10.2307/3546486
12. Bardgett RD, Freeman C, Ostle NJ. Microbial contributions to climate change through carbon cycle feedbacks. ISME J (2008) 2:805–14. doi: 10.1038/ismej.2008.58
13. Barton BT, Schmitz OJ. Opposite effects of daytime and nighttime warming on top-down control of plant diversity. Ecology (2018) 99:13–20. doi: 10.1002/ecy.2062
14. Braganza K, Karoly DJ, Arblaster JM. Diurnal temperature range as an index of global climate change during the twentieth century. Geophys Res Lett (2004) 31:2–5. doi: 10.1029/2004GL019998
15. IPCC. Climate change 2001: The scientific basis., contribution of working group I to the third assessment report of the intergovernmental panel on climate change. (2001)Houghton J.T, Ding Y, Griggs DJ, Noguer M, van der Linden PJ, Dai X, et al. (eds.)].United Kingdom and New York, NY, USA: Cambridge University Press, Cambridge, 881pp. doi: 10.1256/004316502320517344
16. Liu B, Xu M, Henderson M, Qi Y, Li Y. Taking china’s temperature: Daily range, warming trends, and regional variations 1955-2000. J Clim (2004) 17:4453–62. doi: 10.1175/3230.1
17. Shen X, Liu B, Li G, Wu Z, Jin Y, Yu P, et al. Spatiotemporal change of diurnal temperature range and its relationship with sunshine duration and precipitation in China. Journal of Geophys Res: Atmos (2014) 119(23):13,163–13,179. doi: 10.1002/2014JD022326
18. Shen X, Liu B, Liu X. Weak cooling of cold extremes vs. continued warming of hot extremes in China during the recent global surface warming hiatus. J Geophy Res: Atmos (2018) 123:4073–87. doi: 10.1002/2017JD027819
19. Davy R, Esau I, Chernokulsky A, Outten S, Zilitinkevich S. Diurnal asymmetry to the observed global warming. Int J Climatol (2017) 37:79–93. doi: 10.1002/joc.4688
20. Raich J.W.J.J.W., Potter CSC, Bhagawati D. Interannual variability in global soil respiration 1980-94. Glob Change Biol (2002) 8:800–12. doi: 10.1046/j.1365-2486.2002.00511.x
21. Thompson RM, Beardall J, Beringer J, Grace M, Sardina P. Means and extremes: Building variability into community-level climate change experiments. Ecol Lett (2013) 16:799–806. doi: 10.1111/ele.12095
22. Hicks Pries CE, Castanha C, Porras R, Torn MS. The whole-soil carbon flux in reswponse to warming. Science (2017) 1319:1–9. doi: 10.1126/science.aal1319
23. Dang CK, Schindler M, Chauvet E, Gessner MO, Dang CK, Schindler M, et al. Temperature oscillation coupled with fungal community shifts can modulate warming effects on litter decomposition. J Ecology (2009) 90:122–31. doi: 10.1890/07-1974.1
24. Zhu B, Cheng W. Constant and diurnally-varying temperature regimes lead to different temperature sensitivities of soil organic carbon decomposition. Soil Biol Biochem (2011) 43:866–9. doi: 10.1016/j.soilbio.2010.12.021
25. Uvarov AV, Tiunov AV, Scheu S. Long-term effects of seasonal and diurnal temperature fluctuations on carbon dioxide efflux from a forest soil. Soil Biol Biochem (2006) 38:3387–97. doi: 10.1016/j.soilbio.2006.05.009
26. Xia J, Han Y, Zhang Z, Zhang Z, Wan S. Effects of diurnal warming on soil respiration are not equal to the summed effects of day and night warming in a temperate steppe. Biogeosciences (2009) 6:1361–70. doi: 10.5194/bg-6-1361-2009
27. Adekanmbi AA, Shu X, Zou Y, Shaw LJ, Sizmur T. Legacy effect of constant and diurnally oscillating temperatures on soil respiration and microbial community structure. bioRxiv (2021). doi: 10.1101/2021.04.12.439414
28. Jan MT, Roberts P, Tonheim SK, Jones DL. Protein breakdown represents a major bottleneck in nitrogen cycling in grassland soils. Soil Biol Biochem (2009) 41:2272–82. doi: 10.1016/j.soilbio.2009.08.013
29. Wei B, Xie Y, Jia X, Wang X, He H, Xue X. Land use/land cover change and it’s impacts on diurnal temperature range over the agricultural pastoral ecotone of northern China. L. Degrad. Dev (2018) 29:3009–20. doi: 10.1002/ldr.3052
30. Hawkes CV, Keitt TH. Resilience vs. historical contingency in microbial responses to environmental change. Ecol Lett (2015) 18:612–25. doi: 10.1111/ele.12451
31. de Farias PDS, da S. Souza L, de Queiroz Paiva A, de Oliveira Á.S., Souza LD, da S. Ledo CA. Hourly, daily, and monthly soil temperature fluctuations in a drought tolerant crop. Rev Bras Cienc do Solo (2018) 42:1–17. doi: 10.1590/18069657rbcs20170221
32. Graf A, Weihermüller L, Huisman JA, Herbst M, Bauer J, Vereecken H. Measurement depth effects on the apparent temperature sensitivity of soil respiration in field studies. Biogeosciences (2008) 5:1175–88. doi: 10.5194/bg-5-1175-2008
33. Subke JA, Bahn M. On the “temperature sensitivity” of soil respiration: Can we use the immeasurable to predict the unknown? Soil Biol Biochem (2010) 42:1653–6. doi: 10.1016/j.soilbio.2010.05.026
34. Fang C, Moncrieff JB. The variation of soil microbial respiration with depth in relation to soil carbon composition. Plant Soil (2005) 268:243–53. doi: 10.1007/s11104-004-0278-4
35. Eilers KG, Debenport S, Anderson S, Fierer N. Digging deeper to find unique microbial communities: The strong effect of depth on the structure of bacterial and archaeal communities in soil. Soil Biol Biochem (2012) 50:58–65. doi: 10.1016/j.soilbio.2012.03.011
36. Schnecker J, Wild B, Takriti M, Eloy Alves RJ, Gentsch N, Gittel A, et al. Microbial community composition shapes enzyme patterns in topsoil and subsoil horizons along a latitudinal transect in Western Siberia. Soil Biol Biochem (2015) 83:106–15. doi: 10.1016/j.soilbio.2015.01.016
37. Michelsen-Correa S, Scull P. The impact of reforestation on soil temperature. Middle States Geographer (2005) 38:39–44.
38. Crowther TW, Todd-Brown KEO, Rowe CW, Wieder WR, Carey JC, MacHmuller MB, et al. Quantifying global soil carbon losses in response to warming. Nature (2016) 540:104–8. doi: 10.1038/nature20150
Keywords: carbon, climate change, global warming, heterotrophic, microbial, feedback, oscillation, DTR
Citation: Adekanmbi AA and Sizmur T (2022) Importance of Diurnal Temperature Range (DTR) for predicting the temperature sensitivity of soil respiration. Front. Soil Sci. 2:969077. doi: 10.3389/fsoil.2022.969077
Received: 14 June 2022; Accepted: 09 August 2022;
Published: 24 August 2022.
Edited by:
Wenting Feng, Institute of Agricultural Resources and Regional Planning (CAAS), ChinaReviewed by:
Xiangjin Shen, Northeast Institute of Geography and Agroecology, (CAS), ChinaCopyright © 2022 Adekanmbi and Sizmur. This is an open-access article distributed under the terms of the Creative Commons Attribution License (CC BY). The use, distribution or reproduction in other forums is permitted, provided the original author(s) and the copyright owner(s) are credited and that the original publication in this journal is cited, in accordance with accepted academic practice. No use, distribution or reproduction is permitted which does not comply with these terms.
*Correspondence: Tom Sizmur, dC5zaXptdXJAcmVhZGluZy5hYy51aw==