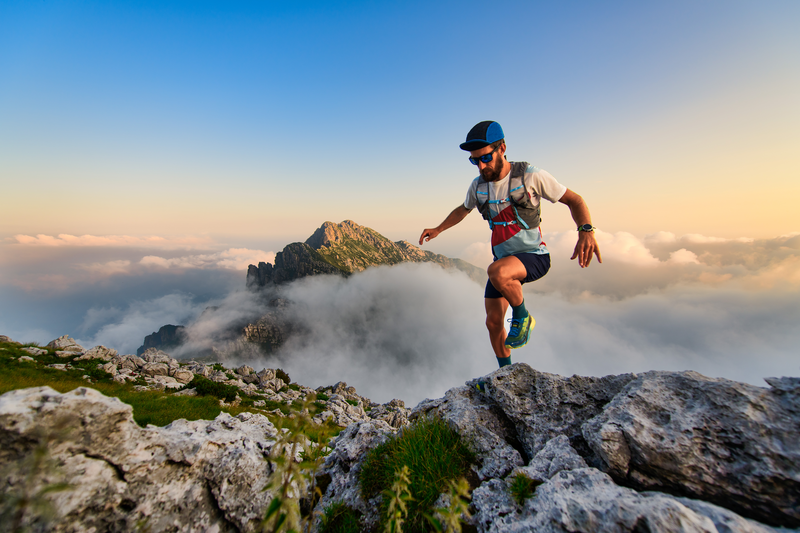
94% of researchers rate our articles as excellent or good
Learn more about the work of our research integrity team to safeguard the quality of each article we publish.
Find out more
ORIGINAL RESEARCH article
Front. Soil Sci. , 08 September 2022
Sec. Soil Management
Volume 2 - 2022 | https://doi.org/10.3389/fsoil.2022.960426
Inorganic and organic fertilizers have been widely used to maintain crop yields. However, several studies have demonstrated that the dissolution of carbonates in agricultural soils by the acidification induced by N-fertilizers can result in their total or partial loss in the tilled layer of some agricultural soils. The effect of inorganic and organic fertilization on carbonates in calcareous semiarid Mediterranean soils has been less studied and is still unclear. Based on a 25-year field experiment, we investigated the effects of different fertilization strategies on the soil pH, inorganic C content, and in the proportion of pedogenic carbonates in the topsoil (0-30 cm) of a carbonate-rich soil in Navarre (N Spain). Five treatments were compared: no amendments as a control (SC), mineral fertilization (MF), and the application of sewage sludge at different doses (80 Mg ha-1 every year (80-1), 40 Mg ha-1 every year (40-1) and 40 Mg ha-1 every four years (40-4). Results showed a decrease in soil pH values by increasing the amount of sewage sludge and a small alteration in the calcite structure particularly in 40-4. However, no significant differences between treatments were found in the total content of carbonates nor in the proportion of pedogenic carbonates. The high concentration of total carbonates in the soil (16.09 ± 0.48%), and of the proportion of pedogenic carbonates (40.21 ± 1.29%, assuming a δ13C of primary carbonates = 0 ‰) seemed elevated enough to compensate for the observed acidification in the studied soil. In the case of MF, no changes were observed in the pH values, nor in the carbonate content (total and pedogenic). This suggests that the use of sewage sludge could induce changes in the future at a faster rate and of greater dimension than the use of mineral fertilizers such as the ones used in this field (46% urea, superphosphate and ClK).
Soils are the largest terrestrial C pool and the third greatest C reservoir in the world, following oceans and fossil fuels (1). The soil C pool is formed by organic and inorganic C (SOC and SIC), which cannot be considered completely independent from each other. SOC has been studied in much detail since long ago due to its importance in chemical, biological and physical processes in soils, and playing an essential role in agricultural soil functioning (2). In contrast, the SIC pool (mainly formed by carbonate minerals, and especially, calcite) has not been carefully studied because its long average residence time (millennia), has led to the general assumption that agricultural practices cannot alter the global content or characteristics of SIC at the same pace than those of SOC. However, several studies have shown that agricultural management activities can indeed generate changes in the dynamics of SIC (3–5). These alterations can be reflected in the amounts, mineralogy and morphology of carbonates of carbonates in superficial soil layers in relatively short time lapses (6–9). This is so because agricultural management normally implies changes in the chemistry of the soil solution through for example fertilization, incorporation of amendments such as lime, and irrigation with water of different quality (3, 6, 10–13). Furthermore, agricultural practices change the soil biological activity (i.e. greater or lesser activity of roots and microorganisms), and therefore of the partial pressure of CO2 (6), which all can alter the SIC cycle. Considering that, calcareous soils account for approximately 50% of the Earth´s surface including approximately 9 billion hectares of arable land worldwide (14), the actual relevance of these processes in agricultural soils needs to be quantified for a better understanding of the consequences of agricultural management on the global soil C budget.
This is especially important in arid and semiarid soils developed on calcareous materials, where the largest SIC stocks are commonly found as a consequence of the non-percolating water regime, which hinders the possibility of complete carbonates dissolution and leaching during pedogenesis (1). As such, in many semi-arid Mediterranean soils, a significant proportion of the total soil mass is formed by primary (lithogenic) and secondary (pedogenic) carbonates (15). However, there is a general lack of data on the proportion of both types of carbonates, because it is difficult to analytically distinguish between them, especially when carbonates are found in the fine fraction of the soil, and the parent material is calcareous (16). This represents a major knowledge gap, despite the relevance of total and pedogenic carbonates and their formation pace in several major soil processes. First, carbonates have been regarded as stabilizers of SOC, which can preserve highly humidified organic matter (2). In fact, in semi-arid zones of Spain, calcareous soils have been seen to contain more SOC than neutral to weakly acidic soils under similar management regimes (17). Second, carbonates are relevant due to their influence in the total C cycle (18). Third, carbonates play a recognized role in stabilizing soil structure (19, 20). Finally, carbonates can have an important influence in soil fertility and agricultural productivity. Therefore, understanding the SIC cycle and the influence of agricultural management (including fertilization) on it in Mediterranean calcareous soils is crucial.
A very relevant management strategy in this sense is fertilization. Nitrogen fertilizers are widely used around the world and their use is expected to increase in the coming decades (21). According to FAO, the total fertilizer nutrient demand was 185,063 million tons in 2016 and this demand was forecast to grow until 200,919 million tons in 2022 (22). The application of inorganic fertilizers produce changes in the chemical and physical properties of agricultural soils. This is so because the use of N-fertilizers can induce soil acidification. Acidification of calcareous soils results in the dissolution of carbonates, increasing CO2 emissions and the leaching of Ca2+. Zamanian etal. (9), have estimated that at least 0.41 Gt C were released irrecoverably as CO2 to the atmosphere from SIC over the past 50 years due to the application of N fertilization. In the same direction Raza etal. (23) have accounted for 7 million ha of cropland becoming carbonate-free in the last 40 years in China. Another recent example is the article of Tao etal. (24), which has concluded that Chinese croplands have lost 27–38% of their inorganic carbon density from the 0–40 cm soil layer, and that the soil pH has decreased by 0.53 units over the past 30 years. In that study, N fertilization was one of the major drivers leading to dramatic losses soil carbonates, so that five provinces of China have already lost more than 75% of their SIC stocks from the upper 40 cm soil layer during the last four decades (23). In the same way, Ortiz etal. (25) have concluded that irrigation waters and fertilizers load can dissolve SIC.
In addition to the use of inorganic fertilizers, the use of organic amendments and fertilizers such as sewage sludge (SS) can also cause changes in the SIC content. Sewage sludge has been applied for decades as a soil amendment in many areas. From a circular economy perspective, utilizing SS in agriculture closes the product life cycle (26) and therefore can be one of the most economically sustainable disposal methods. Because of this, many researchers have studied the potential of SS as a source of nutrients for crops and as a fertilizer, because SS can have considerable quantities of nutrients (0.5-0.7% phosphorus and 2.4-5.0% nitrogen according to Gherghel etal. (27)). However, SS can also contain organic and inorganic pollutants and pathogens, which present a risk for the environment (28–30). In addition, the application of SS can also induce changes in some chemical and physical properties of the soils (31, 32). For example, a decrease in soil pH values have been reported by many researchers after the application of SS at high doses and/or over relatively long periods of time (31–36). As with the application of inorganic fertilizers, the decrease in pH values by employing SS can cause carbonate dissolution (14).
Organic and inorganic fertilization can therefore change the inorganic carbon cycle of agricultural soils. Thus, taking into account that the presence of carbonates in soils is crucial for various processes such as acidity buffering, aggregates formation, organic matter stabilization, microbial and enzyme activities, nutrient cycling and plant productivity (23), the study of SIC content should be a priority, especially in areas with high organic or inorganic N fertilization.
In this framework, the hypothesis of this work was that the application of SS or inorganic fertilizers in a calcareous agricultural soil for a relatively long period of time changes the soil pH and, as a consequence, also modifies the SIC stock and typology i.e. lithogenic and pedogenic carbonate types. Therefore, this work aimed to determine the extent of changes in the soil pH, and in the SIC pool in terms of quantity and typology in the tilled layer (0-30 cm) of a calcareous Mediterranean soil after the application of SS and inorganic fertilizers for 25 years. In order to confirm this initial hypothesis, the methodological approach consisted in a two-step process:
i. First, a mineralogical characterization of the soil samples after the application of SS or inorganic fertilizers for 25 years using X Ray Diffraction (XRD), coupled to the quantification of soil pH and SIC.
ii. Second, a study of the type of carbonates (lithogenic versus pedogenic), from the quantification of pedogenic carbonates using isotopic determinations.
The study was carried out in a long-term experimental field in Arazuri, Navarre, Spain. The experimental field was setup in 1992 to assess the effect of the continuous application of SS as an agricultural amendment in a Calcaric Cambisol (37). The soil has a silty-clay-loam texture (31% clay, 30% silt, 39% sand) and around 16% carbonates in the upper layer (0-30 cm). The main physical-chemical characteristics of this soil layer are shown in Table 1 (30, 32).
The experimental design consists of a random factorial block design with three blocks (n = 3) where each block includes five experimental plots corresponding to the five treatments studied. These were: (1) application of 40 Mg ha-1 of SS every year (40-1, corresponding to the average applied dose in production fields), (2) application of 40 Mg ha-1 of SS every four years (40-4, the lowest dose studied), (3) application of 80 Mg ha-1 of SS every year (80-1 as the highest dose), (4) application of mineral fertilization at the agronomic rate every year (MF) and (5) control plot (SC, no SS or other fertilizers were applied). The individual plots per block, corresponding to the different treatments, had a surface area of 35 m2 (10 m x 3.5 m).
The crops used corresponded to the most frequent ones in rainfed conditions in the area, in a rotation of 3 years (cereal–cereal–sunflower (Helianthus annuus L.)), managed with annual tillage with a 30 cm deep moldboard plow, and application of phytosanitary products according to the crops’ needs each year. The cereal crops used were wheat (Triticum aestivum L.) and barley (Hordeum vulgare L.). Sewage sludge was produced in the urban wastewater treatment plant from the city of Pamplona (population 330,000), with primary and secondary treatments, stabilized through anaerobic digestion and mechanical dewatering (Table 2). The SS was applied in September, 3 to 4 weeks before sowing the cereal crops, using a 3.5 m wide spreader trailer, followed by moldboard plowing down to 30 cm. Finally, mineral fertilization was done with commercial fertilizers at the recommended dose (180 kg N ha-1 year-1 for wheat, 160 kg N ha-1 year-1 for barley and 100 kg N ha-1 year-1 for sunflower). Nowadays, 46% urea and superphosphate are used for wheat and barley and 46% urea, superphosphate and ClK is used for sunflower. However, other types of mineral fertilizers, such as ammonium nitrate, were used in the past.
Table 2 Chemical Properties of the Sewage sludge from the urban wastewater treatment plant of Pamplona.
Soil sampling was carried out 25 years after the onset of the experiment, in September, at each treatment and replicate at 0-30 cm, corresponding to the tilled depth, after the crop cycle was completed, before the application of SS and at the furthest moment in time from previous soil alterations. Samples were collected using an Edelman-type auger (Ø = 5 cm). Three subsamples were collected per plot and combined to obtain a composite sample. The samples were then air-dried and ground to pass a 2 mm sieve.
Homogenized samples, finely ground by using an agate mortar were analyzed by powder X-ray diffraction (XRD). The mineralogical composition of the samples was resolved on a PANalytical X’Pert PRO X-ray diffractometer (Malvern, Panalytical, United Kingdom) fitted with a Cu anode. The operating conditions were 40 mA, 45 kV, divergence slit of 0.5°, and 0.5 mm reception slits. The samples were scanned with a step size of 0.0167° (2θ) and 150 ms per step. The characterization of samples was carried out using the random power method operating from 5° to 80° (2θ) (38).
In addition, bulk samples were analyzed for their total carbonate concentration by a modified Bernard’s calcimeter after quantifying the CO2 produced after treating a soil sample ground to a powdery consistence with HCl 6M (39).
Finally, taking into the account that changes in SIC are affected by soil pH (40), pH values were analyzed in a soil:water suspension at 1:2.5 after overnight shaking in an orbital shaker at 125 rpm, using an electrode CRISON GLP 22 calibrated with standard solutions of pH 4, 7 and 10.
Soil samples ground to 2 mm were treated following two different protocols: (1) carbonate removal by acid fumigation for measuring the δ13C of SOC (41), and (2) organic matter removal by heating at 550°C during 3 hours for measuring the δ13C of SIC.
The isotopic composition of SIC and SOC were measured by Thermo KIEL IV (Thermo Fisher Scientific). The precision is 0.05‰ for δ13C. This isotopic signature was used to determine the type of carbonates (lithogenic vs. pedogenic). The proportion of pedogenic carbonates (f) can be estimated using the equation proposed by Salomons & Mook (42).
where δ13CcarbTOT, δ13CcarbPRIM and δ13CcarbPEDOGEN are the isotopic signature of total, primary or lithogenic and pedogenic carbonates, respectively.
Such a proportion cannot be directly calculated in bulk soil samples nor for any given fraction, because (i) pedogenic carbonates cannot be physically isolated from primary carbonates, which implies that data on δ13CcarbPEDOGEN were not available, and (ii) δ13CcarbPRIM is unknown. The determination of theoretical δ13CcarbPEDOGEN values is however possible from the isotopic signature of the original soil CO2 from which they were precipitated, assuming that the isotopic composition of respired CO2 is equivalent to that of SOC (43), and that respired CO2 accounts for most of the soil atmosphere CO2. According to this approach, the isotopic signature of pedogenic carbonates would be that of the respired CO2 plus an enrichment factor due to lower diffusion rates of 13CO2 than 12CO2 (+4.4 ‰, 44), as well as a temperature-dependent factor accounting for isotopic discrimination during carbonates precipitation from CO2. Following Bughio etal. (6), the estimated value of the latter was of +10.43‰ in our site (for an average annual temperature of 15 °C).
Common values of δ13CcarbPRIM found in the literature for lithogenic carbonates range between -2 to 2 ‰ (45). Therefore, a sensitivity analysis was performed by calculating the proportion of pedogenic carbonates within this range of δ13CcarbPRIM, as previously used in other works (46–48).
The three different zones locating each block (n = 3) that groups the five studied plots of 35 m2 (10 m x 3.5 m) were randomly chosen in the experimental field. In each of the three blocks, the five treatments were randomly assigned too. In this way, the statistical model used takes into account that there may be a random effect of the block in addition to the fixed effect of the treatment.
The effect of the different treatments was analyzed for three variables: the concentration of total carbonates, the proportion of pedogenic carbonates (calculated using a δ13CcarbPRIM value of 0.0‰), and the pH values. The treatment effect for each variable was analyzed with a linear mixed model (LMM) that includes the random effect of the block, and the fixed effect of the treatment. Since the different values of the proportion of pedogenic carbonates calculated for different δ13CcarbPRIM values (-2,0, 2 ‰) are linearly related, the conclusions obtained from the model for treatment effects on f calculated for pedogenic carbonates with δ13CcarbPRIM = 0 ‰ are also valid for the other values. When the LMM observed significant differences, 95% confidence intervals were used for pairwise comparison of mean values between treatments.
According to XRD results, the minerals identified in the studied soil were quartz, calcite, Ca/Na feldspar and minerals from the phyllosilicate and mica groups (Figure 1). No differences were detected overall in the mineralogical composition of the samples from the different treatments (Figure 1). However, a small displacement of the d-spacing calcite peak (3.03 Å) was observed by XRD in the plots in which SS were applied (Figure 2).
Figure 1 Mineralogical composition of the studied plots by XRD. (Application of 40 Mg ha-1 of SS every year (40-1), application of 40 Mg ha-1 of SS each four years (40-4), application of 80 Mg ha-1 of SS every year (80-1), application of mineral fertilization every year (MF) and control plot (SC).
Figure 2 Displacement of the calcite peak by XRD (Application of 40 Mg ha-1 of SS every year (40-1), application of 40 Mg ha-1 of SS each four years (40-4), application of 80 Mg ha-1 of SS every year (80-1), application of mineral fertilization every year (MF) and control plot (SC).
No differences were observed in the values of pH between the control treatment without any fertilization (SC), and MF (Figure 3). Howerer, a decrease in the pH of the samples after the application of SS for 25 years was observed (Figure 3). The greatest reduction in pH values occurred for the highest dose of SS applied to the soil. The LMM confirmed that this effect was statistically significant (p-value= 0.0005). A post hoc (95% family-wise confidence level) analysis showed that the plots without SS addition (SC and MF) had indeed higher pH values than those treated with SS at high doses (40-1 and 80-1) (Figure 3).
Figure 3 Mean pH values ± se(mean) of the soil samples. (Application of 40 Mg ha-1 of SS every year (40-1), application of 40 Mg ha-1 of SS each four years (40-4), application of 80 Mg ha-1 of SS every year (80-1), application of mineral fertilization every year (MF) and control plot (SC). Different letters mean differences between groups (p< 0.05).
Figure 4 shows the results of the concentration of total carbonates, and the proportion of pedogenic carbonates in the studied treatments. In relation to the total concentration of carbonates, the samples presented a mean value of 16.09 ± 0.48% (mean ± se(mean)) of carbonates (Figure 4A). No significant differences were found between treatments (p-value > 0.05 corresponding to the fixed effect of the treatment in the considered LMM). Similar statistical results were found for the proportion of pedogenic carbonates f. In this case, the mean value of f was 40.2 ± 1.29% for δ13CcarbPRIM =0 ‰ (Figure 4B).
Figure 4 Mean values of total content of carbonates ± se(mean) in the soil samples (0-30cm). (A) concentration of total carbonates. (B): Mean values of proportion of pedogenic carbonates. (Application of 40 Mg ha-1 of SS every year (40-1), application of 40 Mg ha-1 of SS each four years (40-4), application of 80 Mg ha-1 of SS every year (80-1), application of mineral fertilization every year (MF) and control plot (SC).
No important changes in soil mineralogy were detected by XRD (Figure 1). Nevertheless, a small displacement of the d-spacing calcite peak (3.03 Å) was observed in the plots in which SS were applied (Figure 2). This small displacement of the d-spacing calcite peak suggests the incorporation of Mg in the calcite structure of pedogenic carbonates (49, 50), indicating that carbonates dissolution/re-precipitation is an active process in SS-treated soils.
This displacement was not appreciated in the MF and SC plots (Figure 2), which means that dolomite or other soluble minerals with Mg were not present in the soil (Figure 1) and the only source of Mg in this experiment is the SS used in the 80-1, 40-1 and 40-4 plots (Table 2) (30). Furthermore, the MF plot showed a narrower calcite peak, suggesting a higher crystallinity than in the other treatments. This fact can be explained because some fertilizers applied in MF plots in the past contained sand-size carbonate grains in their composition (7). Carbonate minerals are intentionally added to some N- fertilizers to reduce their explosive properties and to facilitate their manipulation (51).
In relation to pH values (Figure 3), the decrease in the pH values after the application of SS for 25 years (especially for the highest dose) could be explained by nitrification of the ammonium contained in SS and/or the production of organic acids during organic matter decomposition (52). The relatively high amounts of N added with SS in the treatments with the highest doses can therefore be related to the observed decrease in pH. It has to be noted that despite this statistically significant differences of pH values, the soil pH remained above 8.0 in all treatments, due to the buffer capacity of the high initial content of carbonates (16.09 ± 0.48%), as recently summarized by Raza etal. (23).
In contrast, the pH values did not change after 25 years of applying mineral fertilizers (MF). An explanation can be the intentional addition of carbonates in some fertilizers to reduce their explosive properties and to facilitate their manipulation (51). The added carbonates in the MF plot were most probably in fine/loose particles leading to fast reaction, as they were at the place where acidity generated, for example around the fertilizer granules. Another explanation is the rate of acidity generation by organic fertilizers in the treatments using SS. The rate of acidity of SS is relatively slower than that of the mineral fertilizers but the process takes over longer periods, depending on decomposition rates.
When comparing the pH results of the MF plot with data from other parts of the world, an opposite behavior was observed, since a general drop in soil pH values has recently been reported in China after the application of inorganic fertilizers (23, 24). An explanation of this different response of soils is the differences in the type of fertilizer and doses used. There are several types of commercial N-fertilizers such as anhydrous ammonia, aqua ammonia, ammonium nitrate, ammonium sulfate, N solutions, urea, NH4+-phosphates, and mixed fertilizers (53). And the magnitude of the expectable soil pH decrease related to their use is different depending on the type (54, 55) and on the N dose (55). In this study, the average N fertilizer input in MF was 157 kg N ha-1 year-1, which is smaller than the average N input used in China during 2011-2017 [240 kg N ha-1 year-1, even reaching values of 500 kg N ha-1 year-1 in orchards and greenhouse vegetables crops (23)]. A lesser impact of mineral N fertilization on soil pH in the studied soil than in other soils receiving higher N doses seems therefore expectable.
In relation to the type of N fertilizer, its acidifying power cannot explain the difference of our results from others reporting pH drops after long-term mineral N fertilization. The potential of H+ release by each type of nitrogenous fertilizer is different depending on its composition. For example, (NH4)3PO4 produce the highest amount of H+ release, followed by and (NH4)2HPO4 + KCl (NPK) but urea (CONH2)2, NH4NO3, and NH4HCO3 have a smaller acidifying power (23). In the MF plot, commercial fertilizers with different acidifying power have been used during these 25 years like in China where changes in the pH values of the soils have been reported after the use of several nitrogenous fertilizers (23, 24). This means that in this case, the type of N-fertilizer is not a key factor and the most likely explanation for the different behavior observed is the dose used. Also, as explained above, the soil of this study had a high initial carbonate content (16.09 ± 0.48%), and therefore an important buffer capacity, thus, the high initial content of soil carbonates can counteract to a great extent the increase of possible H+ inputs from nitrogenous fertilizers (14). This suggests that the use of reasonable doses of mineral fertilizers may be enough to mitigate acidification from fertilization in calcareous soils.
Unlike expected, no significant differences were found in the concentration of total carbonates between treatments (Figure 4A), despite the reduction in the pH values of the soil (Figure 3) after the application of SS. Although soil acidification due to the application of fertilizers has the potential to accelerate carbonate dissolution rates (3), the high concentration of carbonates in the study soil (16.09 ± 0.48%), seems elevated enough not to be sensitive to detect these changes in the bulk samples. This suggests that the initial carbonate content is a key factor for observing and detecting these changes in soil bulk samples. Soil carbonates have a well-known buffering capacity, which helps to stabilize pH when an acidic element is added to the soil. Therefore, the changes in the total and pedogenic carbonates content in carbonate-rich soils like the one used in this study seem to be not as rapid as those seen in low-carbonate soils. This result is consistent with the findings of other researchers. For instance, Wu etal. (56), observed that the decline in topsoil pH in noncalcareous croplands was almost twice that observed in the calcareous cropland soils of a region in China. In another article about soil acidification in China, the results revealed a significant acidification of all topsoils except in the highest-pH soils, which represented only a small percentage of the Chinese cultivated soil (57). In both cases, N fertilizer application was the most important factor inducing soil acidification of croplands.
In relation to the content of pedogenic carbonates, the soil of study also had an important proportion of pedogenic carbonates (Figure 4B). This high content of pedogenic carbonates suggests that the dissolution and re-precipitation of carbonates is indeed a relevant process in the studied soil depth. The relatively low precipitation-to-evapotranspiration ratio in the area would limit the leaching of dissolved carbonates, and can explain this accumulation of pedogenic carbonates at the topsoil, as explained for the in situ models for soil carbonates formation and accumulation described by Zamanian etal. (1).
In this sense, as in the case of the total carbonate content, the proportion of pedogenic carbonates (48.68 ± 0.63 to 28.40 ± 0.82%) seemed elevated enough not to be sensitive to detect changes related to the observed decrease in soil pH, when comparing the results of the control plot (SC), with the other treatments (organic and inorganic fertilizers). This observation suggests that the dissolution of carbonates and the acceleration of the formation of pedogenic carbonates in response to the use of acidifying amendments may be modulated by the buffering capacity of the soil associated with its original carbonate content, which seems a crucial factor in this process.
It is also possible that, although no changes in the carbonate content of the bulk samples were detected, changes in some soil size-fractions would occur, as observed in other soils of the region after the adoption of irrigation (7, 47). Therefore, new studies should consider the study of pedogenic carbonates in all the soil size-fractions, considering that other researchers have observed changes in the content of pedogenic carbonates after some agricultural management. For example, Bughio etal. (6), observed annual accumulation rates between 0.23 and 0.12 Mg ha-1 of C in the form of pedogenic carbonates in an irrigated Fluvic Cambisol in a semi-humid region of China under different fertilization systems (organic and mineral) at medium and low doses, over 39 years.
The use of organic or inorganic N fertilization did not result in carbonate losses in the studied calcareous soil after 25 years, indicating that the changes in this soil component were not as rapid as those seen in low-carbonate soils. This suggests that the buffering capacity of soil carbonates can modulate the dissolution of carbonates and the acceleration of the formation of pedogenic carbonates in response to the use of acidifying amendments. In this sense, a high soil initial carbonate content could prevent detecting changes in the decadal scale in the total and pedogenic carbonate contents because of acidification. Therefore, the initial soil carbonates concentration seems a crucial factor to this respect. However, a decrease in soil pH values and a slight change in the crystalline structure of calcite in the tilled layer was detected after the application of high doses of sewage sludge for 25 years, but not with mineral fertilization, indicating that the use of sewage sludge could induce changes in the dynamics of soil inorganic carbon in the future at a faster rate and of greater dimension than the use of mineral fertilizers used in this study. The continued use of organic fertilizers should be studied in detail in relation to its acidifying potential in calcareous soils.
The datasets presented in this article are not readily available because they are subjected to funders’ regulations. Requests to access the datasets should be directed to aXNhYmVsc29uc29sZXMuZGVzb3RvQHVuYXZhcnJhLmVz.
IV and AE were responsible for the conception and design of the study. IDS and IV organized the database and performed formal analysis. HU performing statistical analysis. KZ has done the isotopic analysis. IDS and IV wrote the first draft of the manuscript and AE, HU, and KZ contributed to discussion of the results, review and editing. All authors have read and approved the submitted version.
This project has received funding from the National Institute for Agricultural and Food Research and Technology (INIA) through the RTA2017-00088-C03-01 project, from the Universidad Pública de Navarra through the PJUPNA1905 and PRO-UPNA (6164) projects and from the German Research Foundation (DFG) through the project ZA 1068/4-1.
We are grateful for the services and disposition of the Commonwealth of Pamplona, which contributed the experimental plot and the sludge from the Arazuri treatment plant, and INTIA, who manages the experimental field. We also thank Dr. P. Barré (ENS-Paris, France) and Dr. T. Chevallier (IRD-Montpellier, France) for their support in raising funds for this study, and for fruitful discussions on soil inorganic C storage and cycling. C. Gonzaléz from the Soil Science Laboratory of UPNA is thanked for her assistance in field and lab work. Dr. R García, from Universidad Autónoma de Madrid is thanked for X-ray analysis support.
The authors declare that the research was conducted in the absence of any commercial or financial relationships that could be construed as a potential conflict of interest.
All claims expressed in this article are solely those of the authors and do not necessarily represent those of their affiliated organizations, or those of the publisher, the editors and the reviewers. Any product that may be evaluated in this article, or claim that may be made by its manufacturer, is not guaranteed or endorsed by the publisher.
1. Zamanian K, Pustovoytov K, Kuzyakov Y. Pedogenic carbonates: Forms and formation processes. Earth-Science Rev (2016) 157:1–17. doi: 10.1016/j.earscirev.2016.03.003
2. Romanyà J, Rovira P. An appraisal of soil organic c content in Mediterranean agricultural soils. Soil Use Manage (2011) 27(3):321–32. doi: 10.1111/j.1475-2743.2011.00346.x
3. Sanderman J. Can management induced changes in the carbonate system drive soil carbon sequestration? a review with particular focus on Australia. Agricult. Ecosyst Environ (2012) 155:70–7. doi: 10.1016/j.agee.2012.04.015
4. Plaza-Bonilla D, Arrúe JL, Cantero-Martínez C, Fanlo R, Iglesias A, Álvaro-Fuentes J. Carbon management in dryland agricultural systems. A review. Agron Sustain Dev (2015) 35(4):1319–34. doi: 10.1007/s13593-015-0326-x
5. Wang J, Wang X, Zhang J. ‘Land use impacts on soil organic and inorganic carbon and their isotopes in the yanqi basin’. In: Wang X, editor. Carbon cycle in the changing arid land of China. (Singapore: Springer Nature Singapore Pte Ltd.) (2018). p. 69–88.
6. Bughio MA, Wang P, Meng F, Chen Q, Kuzyakov Y, Wang X, et al. Neoformation of pedogenic carbonates by irrigation and fertilization and their contribution to carbon sequestration in soil. Geoderma (2016) 262:12–9. doi: 10.1016/j.geoderma.2015.08.003
7. de Soto IS, Virto I, Barré P, Fernández-Ugalde O, Antón R, Martínez I, et al. A model for field-based evidences of the impact of irrigation on carbonates in the tilled layer of semi-arid Mediterranean soils. Geoderma (2017) 297:48–60. doi: 10.1016/j.geoderma.2017.03.005
8. Garcia-Franco N, Walter R, Wiesmeier M, Colocho Hurtarte LC, Berauer BJ, Buness V, et al. Biotic and abiotic controls on carbon storage in aggregates in calcareous alpine and prealpine grassland soils. Biol Fertil. Soils (2020) 57:203–18. doi: 10.1007/s00374-020-01518-0
9. Zamanian K, Zhou J, Kuzyakov Y. Soil carbonates: The unaccounted, irrecoverable carbon source. Geoderma (2021) 384:114817. doi: 10.1016/j.geoderma.2020.114817
10. Lal R, Kimble JM. Pedogenic carbonates and the global carbon cycle. In: Lal R, Kimble JM, Eswaran H, Stewart BA, editors. Global change and pedogenic carbonates. Boca Raton, FL, USA: Lewish Publishers (2000). p. 65–85.
11. Entry JA, Sojka RE. Irrigation increase inorganic carbon in agricultural soils. Environ Manage (2004) 33(1):S309–17. doi: 10.1007/s00267-003-9140-3
12. Wu L, Wood Y, Jiang P, Li L, Pan G, Lu J, et al. Carbon sequestration and dynamics of two irrigated agricultural soils in California. Soil Sci Soc America J (2008) 72:808–14. doi: 10.2136/sssaj2007.0074
13. Hannan KD, Kehila D, Millard P, Midwood AK, Neilsen D, Neilsen GH, et al. Bicarbonates in irrigation water contribute to carbonate formation and CO2 production in orchard soils under drip irrigation. Geoderma (2016) 266:120–6. doi: 10.1016/j.geoderma.2015.12.015
14. Raza S, Zamanian K, Ullah S, Kuzyakov Y, Virto I, Zhou J. Inorganic carbon losses by soil acidification jeopardize global efforts on carbon sequestration and climate change mitigation. J Cleaner Production (2021) 315:128036. doi: 10.1016/j.jclepro.2021.128036
15. Mermut AR, Amundosn R, Cerling TE. ‘The use of stable isotopes in studying carbonate dynamics in soils’. In: Lal R, Kimble JM, Eswaran H, Stewart BA, editors. Global change and pedogenic carbonates. (Boca Raton, FL USA: Lewish Publishers) (2000). p. 65–85.
16. Salehi MH, Khademi H, Eghbal MK, Mermut AR. Stable isotope geochemistry of carbonates and organic carbon in selected soils from chaharmahal bakhtiari province, Iran. Commun Soil Sci Plant Anal (2004) 35(11–12):1681–97. doi: 10.1081/CSS-120038562
17. Calvo de Anta R, Luís E, Febrero-Bande M, Galiñanes J, Macías F, Ortíz R, et al. Soil organic carbon in peninsular Spain: Influence of environmental factors and spatial distribution. Geoderma (2020) 370:114365. doi: 10.1016/j.geoderma.2020.114365
18. Baldock JA, Skjemstad JO. Role of the soil matrix and minerals in protecting natural organic materials against biological attack. Organic Geochem. (2000) 31:697–710. doi: 10.1016/S0146-6380(00)00049-8
19. Fernández-Ugalde O, Virto I, Barré P, Gartzia-Bengoetxea N, Enrique A, Imaz MJ, et al. Effect of carbonates on the hierarchical model of aggregation in calcareous semi-arid Mediterranean soils. Geoderma (2011) 164(3–4):203–14. doi: 10.1016/j.geoderma.2011.06.008
20. Rowley MC, Grand S, Verrecchia É.P. Calcium-mediated stabilisation of soil organic carbon. Biogeochemistry. (2018) 137:27–49. doi: 10.1007/s10533-017-0410-1
21. Ma R, Zou J, Han Z, Yu K, Wu S, Li Z, et al. Global soil-derived ammonia emissions from agricultural nitrogen fertilizer application: A refinement based on regional and crop-specific emission factors. Global Change Biol (2021) 27(4):855–67. doi: 10.1111/gcb.15437
22. FAO. World fertilizer outlook and trends to 2022, world fertilizer trends and outlook to 2022 (2019). Available at: https://www.fao.org/3/ca6746en/ca6746en.pdf.
23. Raza S, Miao M, Wang P, Ju X, Chen Z, Zhou J, et al. Dramatic loss of inorganic carbon by nitrogen-induced soil acidification in Chinese croplands. Global Change Biol (2020) 26(6):3738–51. doi: 10.1111/gcb.15101
24. Tao J, Raza S, Zhao M, Cui J, Wang P, Sui Y, et al. Vulnerability and driving factors of soil inorganic carbon stocks in Chinese croplands. Sci Total Environ (2022) 825:154087. doi: 10.1016/j.scitotenv.2022.154087
25. Ortiz AC, Jin L, Ogrinc N, Kaye J, Krajnc B, Ma L. Dryland irrigation increases accumulation rates of pedogenic carbonate and releases soil abiotic CO2. Sci Rep (2022) 12(1):1–12. doi: 10.1038/s41598-021-04226-3
26. Aleisa E, Alsulaili A, Almuzaini Y. Recirculating treated sewage sludge for agricultural use: Life cycle assessment for a circular economy’. Waste Manage (2021) 135:79–89. doi: 10.1016/j.wasman.2021.08.035
27. Gherghel A, Teodosiu C, De Gisi S. A review on wastewater sludge valorisation and its challenges in the context of circular economy. J Cleaner Production (2019) 228:244–63. doi: 10.1016/j.jclepro.2019.04.240
28. Prasad M. N.V, de Campos Favas P. J, Vithanage M, Mohan S. V. ‘8-sanitary and environmental aspects of sewage sludge management’. Industrial and Municipal Sludge Emerging Concerns and Scope for Resource Recovery In: Prasad MNV, et al, editors. (India: Butterworth-Heinemann) (2019). p. 155–80. doi: 10.1016/B978-0-12-815907-1.00008-8
29. Buta M, Hubeny J, Zieliński W, Harnisz M, Korzeniewska E. Sewage sludge in agriculture – the effects of selected chemical pollutants and emerging genetic resistance determinants on the quality of soil and crops – a review. Ecotoxicol Environ Saf (2021) 214:112070. doi: 10.1016/j.ecoenv.2021.112070
30. Zaragüeta A, Enrique A, Virto I, Antón R, Urmeneta H, Orcaray L. Effect of the long-term application of sewage sludge to a calcareous soil on its total and bioavailable content in trace elements, and their transfer to the crop. Minerals (2021) 11(4):356. doi: 10.3390/min11040356
31. Dhanker R, Chaudhary S, Goyal S, Garg V. Influence of urban sewage sludge amendment on agricultural soil parameters. Environ Technol Innovation (2021) 23:101642. doi: 10.1016/j.eti.2021.101642
32. Simoes-Mota A, Poch RM, Enrique A, Orcaray L, Virto I. Soil quality assessment after 25 years of sewage sludge vs. mineral fertilization in a calcareous soil. Land (2021) 10(7):1–20. doi: 10.3390/land10070727
33. Huang CC, Chen ZS. Carbon and nitrogen mineralization of sewage sludge compost in soils with a different initial pH. Soil Sci Plant Nutr (2009) 55(5):715–24. doi: 10.1111/j.1747-0765.2009.00410.x
34. Singh RP, Agrawal M. Effect of different sewage sludge applications on growth and yield of vigna radiata l. field crop: Metal uptake by plant. Ecol Eng (2010) 36(7):969–72. doi: 10.1016/j.ecoleng.2010.03.008
35. Tamir G, Shenker M, Heller H, Bloom PR, Fine P, Bar-Tal A. Organic n mineralization and transformations in soils treated with animal waste in relation to carbonate dissolution and precipitation’. Geoderma (2013) 209–210:50–6. doi: 10.1016/j.geoderma.2013.05.028
36. Eid EM, Shaltout K, Alamri S, Alrumman S, Hussain A, Sewelam N, et al. Monitored sewage sludge application improves soil quality, enhances plant growth, and provides evidence for metal remediation by sorghum bicolor l. J Soil Sci Plant Nutr (2021) 21(3):2325–38. doi: 10.1007/s42729-021-00524-x
37. FAO. Soil map of the world (2021). Available at: https://www.fao.org/soils-portal/data-hub/soil-maps-and-databases/faounesco-soil-map-of-the-world/en/.
38. Moore M, Reynolds RC. X-Ray diffraction and the identification and analysis of clay minerals. 2nd ed. Oxford, UK: Oxford University Press (1997).
39. Sherrod LA, Dunn G, Peterson GA, Kolberg RL. Inorganic carbon analysis by modified pressure-calcimeter method. Soil Sci Soc America J (2002) 66(1):299–305. doi: 10.2136/sssaj2002.2990
40. An H, Wu X, Zhang Y, Tang Z. Effects of land-use change on soil inorganic carbon: A meta-analysis. Geoderma (2019) 353:273–82. doi: 10.1016/j.geoderma.2019.07.008
41. Ramnarine R, Voroney RP, Wagner-Riddle C, Dunfield KE. Carbonate removal by acid fumigation for measuring the δ13c of soil organic carbon. Can J Soil Sci (2011) 91(2):247–50. doi: 10.4141/cjss10066
42. Salomons W, Mook WG. Isotope geochemistry of carbonate dissolution and reprecipitat on in soil. Soil Sci (1976) 1(1):15–24. doi: 10.1097/00010694-197607000-00003
43. Nordt LC, Wilding LP, Drees LR. ‘Pedogenic carbonate transformations in leaching soil systems: implications for the global c cycle’. In: Lal R, Kimble JM, Eswaran H, Stewart B, editors. Global change and pedogenic carbonates. Boca Raton, FL USA.: Lewish Pub (2000). p. 43–64.
44. Rovira P, Vallejo VR. Changes in δ13C composition of soil carbonates driven by organic matter decomposition in a Mediterranean climate: A field incubation experiment. Geoderma (2008) 144(3–4):517–34. doi: 10.1016/j.geoderma.2008.01.006
45. Ryskov YA, Borisov AV, Oleinik SA, Ryskova EA, Demkin VA. ‘The relationship between lithogenic and pedogenic carbonate fluxes in steppe soils, and regularities of their profile dynamics for the last four millennia’. In: Lal R, Kimble JM, Eswaran H, Stewart B, editors. Global change and pedogenic carbonates. Boca Raton, FL USA: Lewish Pub (2000). p. 121–33.
46. Bughio MA, Wang P, Meng F, Chen Q, Li J, Shaikh A. Neoformation of pedogenic carbonate and conservation of lithogenic carbonate by farming practices and their contribution to carbon sequestration in soil. J Plant Nutr Soil Sci (2017) 180:454–63. doi: 10.1002/jpln.201500650
47. de Soto IS, Virto I, Barré P, Enrique A. Effect of irrigation on carbonate dynamics in a calcareous soil using isotopic determinations. Spanish J Soil Sci (2019) 9(2):142–7. doi: 10.3232/SJSS.2019.V9.N2.06
48. Lu T, Wang X, Xu M, Yu Z, Luo Y, Smith P. Dynamics of pedogenic carbonate in the cropland of the north China plain: Influences of intensive cropping and salinization. Agricult. Ecosyst Environ (2020) 292:106820. doi: 10.1016/j.agee.2020.106820
49. Khalaf FI, Al-Zamel A. Petrography, micromorphology and genesis of Holocene pedogenic calcrete in Al-jabal Al-akhdar, sultanate of Oman. Catena (2016) 147:496–510. doi: 10.1016/j.catena.2016.07.044
50. Landi A, Mermut AR, Anderson DW. Origin and rate of pedogenic carbonate accumulation in Saskatchewan soils, Canada. Geoderma (2003) 117(1–2):43–156. doi: 10.1016/S0016-7061(03)00161-7
51. Torres M. ‘Fertilizantes químicos y minerales’. In: Melgar R, Díaz M, editors. Fertilización de cultivos y pasturas. Buenos Aires: Editorial Hemisferio Sur (2005). p. 101–28.
52. Urra J, Alkorta I, Mijangos I, Epelde L, Garbisu C. Application of sewage sludge to agricultural soil increases the abundance of antibiotic resistance genes without altering the composition of prokaryotic communities. Sci Total Environ (2019) 647:1410–20. doi: 10.1016/j.scitotenv.2018.08.092
53. Russel DA. ‘Conventional nitrogen fertilizers’. In: Hauck RD, editor. Nitrogen in crop production. Madison, WI: American Society of Agronomy, Inc (1984). p. 183–94.
54. Ginés I, Mariscal-Sancho I. Incidencia de los fertilizantes sobre el pH del suelo. Fertiberia (2002), 1–9.
55. Fageria NK, Dos Santos AB, Moraes MF. Influence of urea and ammonium sulfate on soil acidity indices in lowland rice production. Commun Soil Sci Plant Anal (2010) 41(13):1565–75. doi: 10.1080/00103624.2010.485237
56. Wu Z, Sun X, Sun Y, Yan J, Zhao Y, Chen J. Soil acidification and factors controlling topsoil pH shift of cropland in central China from 2008 to 2018. Geoderma (2022) 408:115586. doi: 10.1016/j.geoderma.2021.115586
Keywords: pedogenic carbonates, soil inorganic carbon, sewage sludge, inorganic fertilizers, semi-arid land
Citation: de Soto IS, Zamanian K, Urmeneta H, Enrique A and Virto I (2022) 25 years of continuous sewage sludge application vs. mineral fertilizers on a calcareous soil affected pH but not soil carbonates. Front. Soil Sci. 2:960426. doi: 10.3389/fsoil.2022.960426
Received: 02 June 2022; Accepted: 19 August 2022;
Published: 08 September 2022.
Edited by:
Carlos Cantero-Martinez, Universitat de Lleida, SpainReviewed by:
Silvia Maribel Contreras Ramos, CONACYT Centro de Investigación y Asistencia en Tecnología y Diseño del Estado de Jalisco (CIATEJ), MexicoCopyright © 2022 de Soto, Zamanian, Urmeneta, Enrique and Virto. This is an open-access article distributed under the terms of the Creative Commons Attribution License (CC BY). The use, distribution or reproduction in other forums is permitted, provided the original author(s) and the copyright owner(s) are credited and that the original publication in this journal is cited, in accordance with accepted academic practice. No use, distribution or reproduction is permitted which does not comply with these terms.
*Correspondence: Iñigo Virto, aW5pZ28udmlydG9AdW5hdmFycmEuZXM=
Disclaimer: All claims expressed in this article are solely those of the authors and do not necessarily represent those of their affiliated organizations, or those of the publisher, the editors and the reviewers. Any product that may be evaluated in this article or claim that may be made by its manufacturer is not guaranteed or endorsed by the publisher.
Research integrity at Frontiers
Learn more about the work of our research integrity team to safeguard the quality of each article we publish.