- Farmed Landscapes Research Centre, School of Agriculture and Environment, Massey University, Palmerston North, New Zealand
Over half of Aotearoa New Zealand’s (NZ’s) land area is under agriculture or forestry production. Long term monitoring has shown declines in freshwater quality in regions with the most intensive agriculture. The New Zealand government has historically focused on reducing the impact of agriculture on water quality through its Resource Management Act 1991. Lack of improvement in freshwater quality has resulted in the 2020 Essential Freshwater package of reforms which will require all pastoral farms >20 ha in size and all arable farms > 5 ha in size to develop a Freshwater Farm Plan (FFP) by a certified Freshwater Farm Planner. As far as we are aware, New Zealand is the first country in the world to mandate compulsory FFPs. This paper describes the key geological, soil, and landscape factors that need to be considered in an FFP for it to be successful in meeting the 2020 Essential Freshwater objectives. We argue that a greater emphasis should be placed on understanding a farm’s natural resources, as they provide the physical interface between the farming system and both the freshwater and atmospheric ecosystems. Documenting our learning in this area could assist other countries considering Freshwater Farm Planning as a strategy to reduce the impact of agriculture on freshwater quality.
Introduction
Forty-eight billion dollars’ worth of export revenue was generated from agriculture in Aotearoa New Zealand (NZ) in 2020, making it one of the country’s largest export earners. Dairy (42%), meat and wool (22%), and horticulture (13.5%), are the dominant industries (1) with over half of NZs entire land area under agriculture or forestry production. Sheep, beef, and dairy farming make up the majority of this production area (11.2 million ha, 81%) (2). Half of NZ’s river length drainage catchments are described as having a ‘pastoral land cover class’ (3) and are defined as having < 15% urban land use and > 25% of land under pastoral agriculture, which is predominantly grazed by dairy, beef, and sheep. Other significant land uses within this land cover class include horticulture and arable crops.
New Zealand has more than 70 major rivers, with streams and rivers covering a total length of 425,000 km, half of which are headwater streams (4). Rivers are generally characterised as being fast flowing, comparatively short and generally unstable (4), whilst lakes are commonly volcanic or glacial in origin. Nationally important waterways in New Zealand have been classified in to 7 biogeographic regions based on physical disturbances (Last Glacial Maximum, volcanic eruptions and seismic activity), biotic distributions and genetic similarity between populations (5). National water quality monitoring has shown that the concentration of nitrogen (N), phosphorus (P), sediment, and E coli contaminants have increased, and ecological health indicators have decreased, as the percentage of pastoral and urban land cover in upstream catchments has increased (6). As a result, the quality of freshwater in New Zealand is of significant concern to the New Zealand public, with water related issues rated as the most important environmental issue facing NZ, along with a perception that agriculture is one of the three main causes of freshwater degradation (7). In response to this societal and political pressure, the NZ Government has historically used policies such as its Resource Management Act 1991 as an instrument to protect freshwater and freshwater ecosystems. This Act states that the responsibility of protecting freshwater and freshwater ecosystems sits with NZs regional governing bodies (Regional Councils). However, improvement of freshwater quality has not met societal expectations for clean water suitable for recreational activities, due to challenges associated with regulatory limitations, lack of landscape/catchment approaches, and the difficulty of addressing more diffuse forms of contaminant loss that are typical of agriculture. A new suite of regulations, the Essential Freshwater Package, was introduced in 2020 which places the wellbeing of water as the highest guiding principle (Te Mana o te Wai) and acknowledges and values the role of Māori (the indigenous people of Aotearoa) in its decision making.
A key policy instrument in these new reforms is the compulsory requirement for all pastoral farms >20 ha in size, and all arable farms > 5 ha in size, to develop a Freshwater Farm Plan (FFP) by a certified Freshwater Farm Planner, starting in 2022. These plans have the specific purpose “….to better control adverse effects of farming on freshwater and freshwater ecosystems within specified districts, regions, or parts of New Zealand through the use of certified freshwater farm plans.” (8). Although soil and water conservation plans, whole farm plans, and farm environment plans, have long been used in NZ (9–11) to minimise soil erosion and reduce agricultural impacts on the surrounding environment, this is the first time farm plans will be specifically used to protect NZ freshwater. As far as we are aware, NZ is the first country in the world to mandate compulsory FFPs (11, 12).
Comprehensive Freshwater Farm Planning requires an understanding of the farmer’s values and goals for their farm, business, and family. To address Te Mana o te Wai, it is critical that the plan takes account of the farm’s location within the catchment, understands the catchment’s cultural and community values, the waterways interacting with the farm, and prioritises the freshwater issues of greatest concern. Freshwater Farm Plans will also be used by the government as a tool to demonstrate compliance with national regulatory requirements, such as meeting its Nitrogen Fertiliser cap policy and Intensive Winter Grazing requirements (13). To meet the primary objective of controlling or reducing the adverse effects of farming on freshwater and freshwater ecosystems, these plans need a comprehensive approach to 1) understanding the geology, soil, landscape resources, and long term climate patterns, and how these are likely to influence the spatial and seasonal changes in the types and pathways of contaminant loss, 2) understanding the farm system and particular management practices which increase the likelihood of contaminant loss to freshwater, and 3) integrating the farm’s physical resources with farm management practices to identify and prioritise risk, and to recommend mitigation strategies which are practical and effective. These recommendations need to consider the farmer’s economic and human resources, and then need to be prioritised and costed to allow the farmer to address issues sequentially and pragmatically. The role of the farmer in this process cannot be understated, as targeted on-farm actions to mitigate contaminant loss risk must result in measurable improvements in freshwater outcomes for the Essential Freshwater policy to be effective, a challenge also recognised internationally (14).
To be effective in meeting freshwater quality and freshwater ecological objectives, FFPs need to do more than just meet industry-defined Best Management Practice (BMP). For some farms in some catchments, best practice will not be sufficient, since BMPs are often generalised and are not specific enough to target the unique physical resource and management aspects of the farm being surveyed. For example, intensively grazed farms with well drained soils and high rainfall may have a high risk of nitrate leaching and may not be able to meet the Essential Freshwater Package objectives by just meeting BMPs associated with N fertiliser use or grazing management. More specific and targeted mitigation strategies are likely to be needed on these farms. Freshwater farm planners who can confidently identify the contaminant of highest risk, the likely pathway for its loss, and the natural resource and climatic factors contributing to and exacerbating this risk, will play a critical role in the successful implementation of the Essential Freshwater policy and its longer-term objectives in improving NZs freshwater outcomes.
The Essential Freshwater objectives, national standards, and timeframes have now been written into NZ’s legislative framework, and this has created an urgent and critical need to train rural professionals to develop the multidisciplinary and integrative skills required to deliver FFPs to a high standard, and thus resulting in tangible improvements in freshwater outcomes throughout the country. Through the accumulation and synthesis of academic research, combined with decades of institutional experience in the fields of geology, soil and landscape patterns, and their interaction with agricultural management practices influencing contaminant loss processes, we are well placed to contribute to the current debate regarding which essential elements an FFP should encompass to achieve successful freshwater outcomes. This paper aims to provide a perspective to aid policy planners and prospective Freshwater Farm Planners to help identify those key aspects which need to be addressed in an FFP to reduce risks to freshwater quality from agricultural production systems.
Geology
Geology is often overlooked when contaminant loss from farms is considered because it is often not visible and requires specialist training to interpret its role. However, we argue that geology is one of the more important resources to identify and interpret in an FFP as it has a significant influence over both the contaminant types lost from farms and the pathways by which they are lost. For example, the geology underlying a farm influences the likelihood of soil erosion and sediment loss to freshwater by influencing erosion type and severity, primarily via the combination of slope and the susceptibility of the rock to weathering. Geology also dictates the type and stability of soils developing above the rock. Over the last 25 million years, New Zealand has experienced rapid uplift, due to subduction processes between the Pacific and Indo-Australian tectonic plates. Today, this uplift has exposed many areas of quartzo-feldspathic marine sedimentary rocks. These consist of relatively unconsolidated mudstones and sandstones which are susceptible to erosion under New Zealand’s rainfall and temperature regime, and which overlie older and much more consolidated mudstones and sandstones (argillites and greywackes, respectively). These consolidated marine sediments have become more frequently exposed in higher uplift areas parallel to the plate boundary. Subduction arc volcanism has added complexity to NZ’s base marine sedimentary geology with volcanic tephra deposits such as pumices, and andesitic and rhyolitic ashfalls (15). Soils developing directly from the consolidated marine sedimentary rocks tend to be more stable and resistant to erosion, whilst those developing from the unconsolidated mudstone and sandstone rock types or are forming from unconformable airfall material such as loess and volcanic airfall deposition, are more susceptible to erosion processes. Underlying aggradational alluvial gravels (sourced from very high erosion rates of greywacke rock during global cold climate cycles) often have a well-established hydraulic connection to ground and surface water bodies. Thus, field recognition and inclusion of geological features is crucial in terms of understanding potential ‘hidden’ pathways of nutrient loss and erosion processes. A farm with underlying alluvial gravels located adjacent to a river with a significant nitrate water quality issue may need more aggressive nitrogen management to reduce the risk to that freshwater body. In contrast, finer soil texture, drainage class, and a finer-textured underlying rock type can influence the biogeochemistry of the sub surface environment, creating redox conditions which facilitate the denitrification of nitrate to inert N gas and reduce the risk of nitrate loss to ground and, eventually, to surface water (16).
Most of NZ’s public domain geological data is available at a scale of 1:250000, is presented as lithological and stratigraphic units rather than susceptibility to weathering, and often ignores important (in the context of this discussion) loosely consolidated overburden material such as loess and tephra deposits. However, this dataset does provide the FPP planner with some sense of rock types that are more likely to be encountered when undertaking a farm survey. A separate (and potentially more useful) public domain database is NZ’s Land Use Capability (LUC) dataset containing rock types nominally mapped, in terms of weatherability, at a scale of about 1:50000. Despite the availability of these datasets, geological mapping is not at a scale sufficient to meet the farm-scale analysis (~1:10000) required for the formulation of robust FFPs.
Soils
Soil types are a product of the weathering process of a farm’s underlying geology and are influenced by the five key soil forming factors of climate, vegetation, topography, parent material, and time. In combination, these factors control the physical, biological, and chemical weathering processes that influence soil properties such as texture (the percentage of sand, silt, and clay size fractions), nutrient adsorption properties, hydraulic conductivity, water storage buffering under dry conditions, and structural susceptibility to animal pugging, compaction, erosion, and resilience to cultivation. The appropriate scale of spatial distribution and correct identification of soil types are paramount to achieve an accurate assessment of contaminant loss risk in Freshwater Farm Planning. New Zealand soils have historically been mapped at a regional scale (1:50000), with their chemical and physical properties and spatial distribution publicly accessible through a central data base called Smap online (17). However, this scale is often too coarse to accurately define soil types and boundaries at the farm scale (18) which is required for the formulation of robust FFPs which requires analysis of data at the farm scale.
Accurate identification of soil types and soil properties is a key component of contaminant loss risk and pathway identification. For example, soils which are shallow, coarse-textured, and have a low P sorption capacity, are very susceptible to P leaching and potentially surface runoff loss (19). Such soils represent an extreme risk of contaminant loss to freshwater if they are receiving surface applications of animal effluent (20). If regional scale soil mapping suggests the soil type receiving this effluent application has a high P sorption capacity when, in fact, farm scale mapping shows that the soil has low P sorption and is at risk of P leaching and surface runoff loss, the objectives of the FFP in terms of “… to better control adverse effects of farming on freshwater and freshwater ecosystems.” will be compromised and improved freshwater outcomes will not be achieved. A particular example is the complex of low P sorption Gley (Sulphaquepts, Aquepts or Aquents, Aquox) and high P sorption Allophanic (Aquands, Cryands and Udands) soils (21, 27) found in parts of the Waikato region of NZ where intensive dairying (with its associated high rainfall (> 1000 mm/pa) and high P fertiliser application rates) dominates agricultural systems.
It is essential that Freshwater Farm planners have the necessary skills to ground-truth and validate soil types and boundaries on the farm to undertake contaminant loss risk assessments using appropriate information mapped at the farm scale. A survey on farm planning practices conducted in 16 regional council bodies in NZ between 2001 and 2004 found that only one of the 20 different plan types involved developing a farm scale soil map (10). Manderson and Palmer (18) argue that the provision of appropriately scaled soil data is economically feasible and in line with practices used in other strong agricultural countries within Europe and the United States of America.
Landscape classification and sustainable production
Land Resource Inventory and Land Use Capability
NZ’s Land Resource Inventory (LRI) and LUC (22) is a land use classification system derived from that used in the United States of America (9). The LRI database (23) contains five physical factors (rock type, soil type, slope, erosion type and severity, vegetative cover) which have been regionally mapped at 1:50000 scale to objectively identify and define landscape polygons in terms of their erosion susceptibility. Each polygon is then assessed in terms of its capability for long-term sustainable production [classed from 1 (most versatile) through to 8 (severe limitations)], the major limiting factor for sustainable production (erosion, wetness, soil properties, climate), and the suite of management practices required to ensure long term sustainable land use. These three assessments are encapsulated in the polygon’s LUC code. Whilst the LUC assessment of a landscape unit (or polygon) is somewhat subjective and may differ to some degree between LUC assessors, its greatest value lies with the associated LRI database which is an objective measurement of the five physical factors influencing erosion type and severity. New Zealand’s agricultural land area has been regionally mapped in this manner and is publicly available. Although the mapping scale is not ideal for the farm-scale analysis required for robust FFPs, the LRI database does provide very useful indicators regarding the approximate type and spatial distribution of rock and soil types, slope, erosion types and severity, and vegetative cover on and adjacent to the farm. Slope information can be enhanced by the increasing availability of very high resolution (metre-scale) digital elevation models (DEMs) which now cover significant areas of NZ, and can be processed, using a suitable GIS package, to produce high quality slope, aspect, and waterflow pathway maps.
The LRI/LUC system was first developed in 1969, primarily to address increasing erosion severity observed in NZ since the 1930s and does not consider nutrient loss risk (particularly nitrate). However, it is still relevant in terms of minimising the adverse effects of agriculture on freshwater quality, primarily in terms of the objective measurement of erosion type and severity which contribute significantly to the sediment loads (and thus freshwater quality) of several NZ waterways (24). This assessment allows a targeted response to erosion type and severity, increasing the likelihood of successful mitigation strategies and reducing sediment loads to waterways.
Land management units
To help identify and assess risk to freshwater quality, freshwater farm planners need to bring together their understanding of geology, soil type, and landscape features, and overlay this with an understanding of farm management practices, to enable identification of different land management units (LMU) within the farm. Land management units are important, as they group areas of the farm that respond in a similar way to similar management practices, thus simplifying the process of identifying the farm’s risk to freshwater quality. For example, areas of the farm which have flat topography and are poorly drained, may be prone to animal pugging damage, especially when grazed by heavier stock classes, increasing the risk of sediment, nutrient, and pathogen loss, via surface runoff (Figure 1). Identification of these areas then allows planners to target specific management or mitigation strategies to individual LMUs to minimise risk to freshwater.
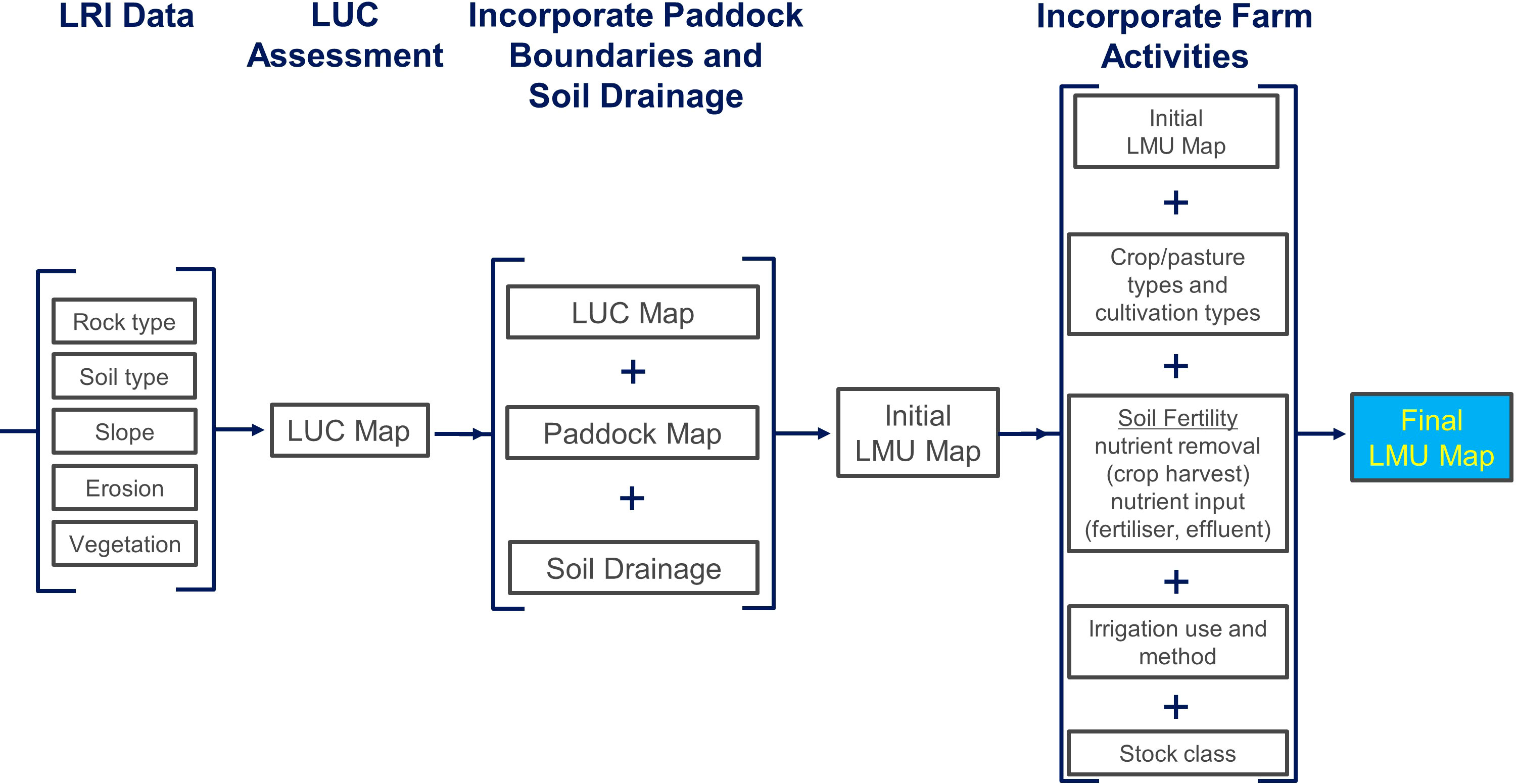
Figure 1 Example of how a farm can be separated into different land management units (LMU) to help identify the likely risks to freshwater and thus target mitigation strategies. LRI: Land Resource Inventory, LUC: Land Use Classification.
Determining risk to freshwater
Identifying the risk to freshwater that each farm LMU poses requires a detailed understanding of sediment, nutrient, and pathogen sources, nutrient cycling within a farm system, and loss processes operating at the farm scale, a topic which is not the subject of this paper. Determining risk can be subjective as the planner will need to draw on their own knowledge and experience to assess and rank nutrient and farm management with respect to long term seasonal climate patterns and the farm’s natural resources. This process can be assisted using farm scale contaminant loss models, but these are not always reliable or do not cover the range of contaminants of concern. Freshwater Farm planners also need to understand the importance of contaminant connectivity when assessing risk. For example, eroded soil which travels across a gently sloping 100m long paddock consisting of soils with good drainage properties will pose a lower risk than soils (with similar drainage properties) eroding from a steep hill side located 5 m above a flowing stream. The importance of identifying critical source areas (CSAs) within the farm have been well documented (25, 26) and planners must correctly identify and rank CSAs in terms of their contaminant loss risk and their connectivity to ground or surface water.
This risk assessment highlights the importance of ensuring that Freshwater Farm Planners have the highest level of knowledge and integrative skillsets to understand how underlying geology influences soil properties and nutrient transport processes and attenuation, how climate and landscape features influence when and how contaminants will be lost and, finally, how the complex farm system may interact with these features to exacerbate the risk of loss, and which management strategies will best mitigate this loss. It is hoped that the NZ government’s proposed Freshwater Farm Planning certification scheme will be sufficiently stringent to ensure that the Essential Freshwater policy is delivered by highly skilled and experienced planners and that the auditing process serves as a robust means by which FFP recommendations are implemented by the farmer.
Conclusion
This paper provides a perspective drawn from the authors’ experience in soil science research and in teaching, both at the undergraduate and postgraduate level, and in the delivery of professional short courses related to the topics of sustainable nutrient management and freshwater farm planning. With the recent introduction of the Essential Freshwater Package into NZ’s legislative framework and the requirement that most farms will be required to develop an FFP, there is an immediate need to provide the training and education of professionals interested in becoming FFP planners.
These relevant skill sets require the ability to integrate both farm management practices, and knowledge of farm systems with an understanding of the processes associated with the farm’s physical resources. A robust FFP will require analysis at the farm scale. Whilst information and skills associated with the farm system and management practices is often readily available at this scale, the mapping scale of physical resource data is not, and is often only available at a regional scale. Coupled with this is the scarcity of people who are comfortable with soil and geological processes relative to those familiar with farming systems and the use of nutrient budgeting models. We feel that a stronger emphasis needs to be placed on the importance of geology, soil, and landscape knowledge in freshwater farm planning to achieve measurable improvements in freshwater outcomes for Aotearoa New Zealand.
Data availability statement
The original contributions presented in the study are included in the article/supplementary material. Further inquiries can be directed to the corresponding author.
Author contributions
This paper was written jointly by LB and MB. All authors read and approved the final manuscript.
Acknowledgments
We would like to acknowledge Alan Palmer for inspiring us with his long serving passion and dedication to the importance of geology and soil science in whole farm planning. We also acknowledge Mike Hedley for starting us on this journey of professional development education.
Conflict of interest
The authors declare that the research was conducted in the absence of any commercial or financial relationships that could be construed as a potential conflict of interest.
Publisher’s note
All claims expressed in this article are solely those of the authors and do not necessarily represent those of their affiliated organizations, or those of the publisher, the editors and the reviewers. Any product that may be evaluated in this article, or claim that may be made by its manufacturer, is not guaranteed or endorsed by the publisher.
References
1. Ministry for Primary Industries. Situation and outlook for primary industries-2021 (2021). Wellington, New Zealand: Ministry for Primary Industries. Available at: https://www.mpi.govt.nz/dmsdocument/45451-Situation-and-Outlook-for-Primary-Industries-SOPI-June-2021 (Accessed May 25, 2022).
2. Beef and Lamb NZ. Compendium of new Zealand farm facts, in: Beef and lamb NZ (2020). Wellington, New Zealand. Available at: https://beeflambnz.com/sites/default/files/data/files/Compendium-2020.pdf (Accessed May 25, 2022).
3. Ministry for the Environment and StatsNZ. Our freshwater 2020 (2020). Ministry for the Environment and StatsNZ. Available at: https://environment.govt.nz/publications/our-freshwater-2020/ (Accessed May 10, 2022).
4. Department of Conservation. Overview of new Zealand rivers (2022). Available at: https://www.doc.govt.nz/about-us/statutory-and-advisory-bodies/nz-conservation-authority/publications/protecting-new-zealands-rivers/02-state-of-our-rivers/overview-of-new-zealand-rivers/6 (Accessed August 2, 2022).
5. Leathwick JR, Collier K, Chadderton L. Identifying freshwater ecosystems with nationally important natural heritage values: development of a biogeographic framework (2007). Department of Conservation. Available at: https://www.doc.govt.nz/globalassets/documents/science-and-technical/sfc274.pdf (Accessed August 2, 2022).
6. Larned S, Brooker D, Dudley B, Moores J, Monaghan R, Baillie B, et al. Land-use impacts on freshwater and marine environments in new Zealand (2018). National Institute of Water and Atmospheric Research Ltd. Available at: https://environment.govt.nz/assets/Publications/Files/land-use-impacts-on-freshwater-and-marine-environments-.pdf (Accessed May 19, 2022).
7. Hughey KFD, Kerr GN, Cullen R. Public perceptions of new zealand’s environment. EOS ecology (2019). Christchurch: Lincoln University. Available at: https://research.lincoln.ac.nz/resources/general/Public-Perceptions-of-New-Zealands-Environment-2019_2021-01-25-032756.pdf (Accessed May 15, 2022).
8. Ministry for the Environment. Resource management act 1991. part 9A: inserted, on 1 July 2020, by section 64 of the resource management amendment act 2020 (2020 no 30) (2020). Wellington: Ministry for the Environment. Available at: https://www.legislation.govt.nz/act/public/1991/0069/latest/whole.html?search=sw_096be8ed81c12031_freshwater+farm+plan_25_se&p=1 (Accessed May 15, 2022).
9. McCaskill LW. Hold this land: a history of soil conservation in new Zealand. Wellington: A H & A W Reed (1973).
10. Manderson AK, Mackay AD, Palmer AP. Environmental whole farm management plans: Their character, diversity, and use as agri-environmental indicators in new Zealand. J Environ Man (2007) 82:319–31. doi: 10.1016/j.jenvman.2005.05.020
11. Stokes S, Macintosh KA, McDowell RW. Reflecting on the journey of environmental farm planning in new Zealand. New Z J Agric Res (2021) 64:1–8. doi: 10.1080/00288233.2021.1876108
12. Macintosh KA, McDowell RW, Wright-Stow AE, Depree C, Robinson GM. National-scale implementation of mandatory freshwater farm plans: a mechanism to deliver water quality improvement in productive catchments in new Zealand? Nutt Cycl. Agroecosys. (2021) 120:121–9. doi: 10.1007/s10705-021-10146-5
13. Ministry for the Environment. Freshwater farm plans-how freshwater farm plans fit with the wider regulatory system (2022). Wellington, New Zealand. Available at: https://environment.govt.nz/acts-and-regulations/freshwater-implementation-guidance/freshwater-farm-plans/ (Accessed May 10, 2022).
14. Smithers J, Furman M. Environmental farm planning in Ontario: exploring participation and the endurance of change. Land Use Policy (2003) 20:343–56. doi: 10.1016/S0264-8377(03)00055-3
15. Ballance PF, Cotterall L. New Zealand geology: An illustrated guide. Geosci Soc New Z (2017) 148:397.
16. Rivas A, Singh R, Horne D, Roygard J, Matthews A, Hedley MJ. Denitrification potential in the subsurface environment in the manawatu river catchment, new Zealand: Indications from oxidation-reduction conditions, hydrogeological factors, and implications for nutrient management. J Environ Man (2017) 197:476–89. doi: 10.1016/j.jenvman.2017.04.015
17. Manaaki Whenua. S-map online (2022). Manaaki Whenua. Available at: https://smap.landcareresearch.co.nz/ (Accessed May 19, 2022).
18. Manderson A, Palmer A. Soil information for agricultural decision making: a new Zealand perspective. Soil Use Man (2006) 22:393–400. doi: 10.1111/j.1475-2743.2006.00048.x
19. McDowell RW, Condron LM. Chemical nature and potential mobility of phosphorus in fertilized grassland soils. Nutt Cyc. Agroecosys. (2000) 57:225–33. doi: 10.1023/A:1009838424935
20. McDowell RW, Gray CW, Cameron KC, Di HJ, Pellow R. The efficacy of good practice to prevent long-term leaching losses of phosphorus from an irrigated dairy farm. Agric Ecosys. Environ (2019) 273:86–94. doi: 10.1016/j.agee.2018.12.007
21. Hewitt A, Dymond J. Survey of new Zealand soil orders. Ecosys. Serv New Zealand: cond. Trends (2013) 1(10):121–31.
22. Lynn IH, Manderson AK, Page MJ, Harmsworth GR, Eyles GO, Douglas GB, et al. Land use capability survey handbook – a new Zealand handbook for the classification of land. Lower Hutt: AgResearch Hamilton; Landcare Research, Lincoln; GNS Science (2009).
23. Anderson JR, Hardy EE, Roach JT, Witmer RE. A land use and land cover classification system for use with remote sensor data. United States Geol. Surv. Prof Pap. (1976), 964. doi: 10.3133/pp964
24. Hicks DM, Shankar U, McKerchar AI, Basher L, Lynn I, Page M, et al. Suspended sediment yields from new Zealand rivers. J Hydrol. (New Zealand) (2011) 50:81–142. doi: 10.3316/informit.p315190637227597
25. Gburek WJ, Sharpley AN, Folmar GJ. Critical areas of phosphorus export from agricultural watersheds. Boca Raton, FL: Lewis Publishers (2000).
26. Pionke HB, Gburek WJ, Sharpley AN. Critical source area controls on water quality in an agricultural water-shed located in the Chesapeake basin. Ecol Eng (2000) 14:325–35. doi: 10.1016/S0925-8574(99)00059-2
Keywords: farm environment plan, whole farm plan, agriculture, sustainable agriculture, water quality, policy
Citation: Burkitt L and Bretherton M (2022) The importance of incorporating geology, soil, and landscape knowledge in freshwater farm planning in Aotearoa New Zealand. Front. Soil Sci. 2:956692. doi: 10.3389/fsoil.2022.956692
Received: 30 May 2022; Accepted: 16 August 2022;
Published: 02 September 2022.
Edited by:
Amin Soltangheisi, University of Reading, United KingdomReviewed by:
Silvia Maribel Contreras Ramos, CONACYT Centro de Investigación y Asistencia en Tecnología y Diseño del Estado de Jalisco (CIATEJ), MexicoCopyright © 2022 Burkitt and Bretherton. This is an open-access article distributed under the terms of the Creative Commons Attribution License (CC BY). The use, distribution or reproduction in other forums is permitted, provided the original author(s) and the copyright owner(s) are credited and that the original publication in this journal is cited, in accordance with accepted academic practice. No use, distribution or reproduction is permitted which does not comply with these terms.
*Correspondence: Lucy Burkitt, TC5CdXJraXR0QG1hc3NleS5hYy5ueg==