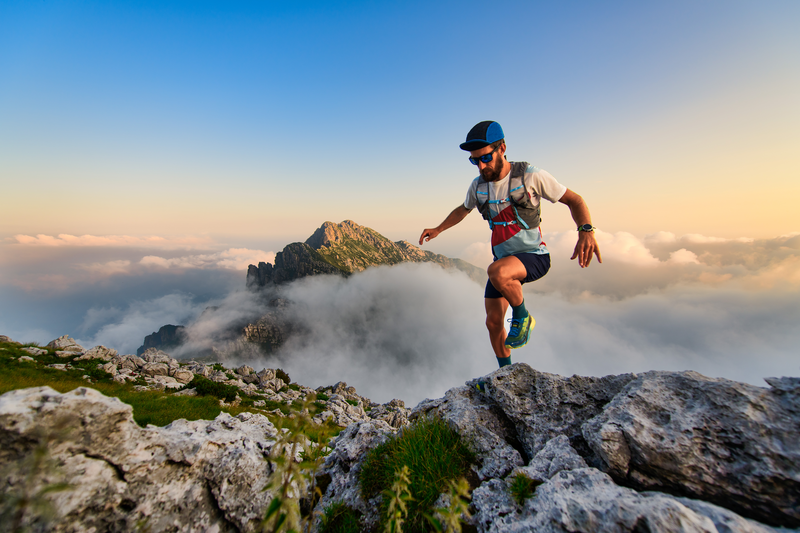
95% of researchers rate our articles as excellent or good
Learn more about the work of our research integrity team to safeguard the quality of each article we publish.
Find out more
REVIEW article
Front. Soil Sci. , 02 August 2022
Sec. Plant-Soil Interactions
Volume 2 - 2022 | https://doi.org/10.3389/fsoil.2022.920142
The hydrogen sulfide (H2S) deposition from oil exploitation occurring since 1969 may potentially affect bacterial communities in acacia and eucalyptus plantations of the Congolese coastal plains. These plantations have been implemented on previous native savannas to use the unsuitable soils for agriculture, provide pulp wood and fuel wood energy, and preserve the natural forests. Increased carbon (C) and nitrogen (N) stocks in stands containing acacia relative to baseline (eucalyptus) stocks have been reported. Phosphorus availability also improved in coarse particulate organic matter (4,000–250 µm) in afforested stands as compared to natural savannas. Investigation of the abundance of bacterial phyla by metabarcoding of the 16S rRNA bacterial gene in different stands of monocultures and mixed-species stands reveals the prevalence of Actinobacteria in all stands. This phylum is generally associated with the presence of sulfur in industrial areas and has a crucial role in organic matter decomposition. This may be linked to improved soil attributes (C, N, and P) and related to oil exploitation in addition to natural processes. This review shows, therefore, how potentially human activities may impact bacterial community composition, which may further change other soil attributes. It also acknowledges that the sustainability of forest plantations on inherently nutrient-poor soils strongly relies on interactions between soil functions, the environment, and human activities driven by soil organisms.
Taking into consideration the potential risk of the endangered savanna ecosystems (1, 2), infertile soils unsuitable for agriculture beneath the tropical savannas in the Congolese coastal plains have been used for fast-growing plantations such as eucalyptus since the 1950s (3, 4). Soils are Arenosols, the most represented soil type in Africa (22%) (https://esdac.jrc.ec.europa.eu/content/soil-map-soil-atlas-africa). They span over 6 million hectares, from the Democratic Republic of the Congo (DRC) through the Republic of the Congo (RoC) to Gabon (5–7) in Central Africa, which is home to the second largest rainforest ecosystem in the world (https://www.onegreenplanet.org/animalsandnature/the-worlds-second-largest-rainforest-congo/). These soils are sandy (>90% of sand) with an inherently nutrient-poor status, i.e., very low soil organic matter (SOM) content (<1% of SOM) (8).
Afforestation or reforestation is a significant forest management practice worldwide (9, 10) to sequester carbon (C) in both soil and biomass, with a potential impact on mitigating climate change, furnishing wood, and restoring land degradation (11–14). Furthermore, afforestation also aims to preserve natural forests and ensure other soil and ecosystem services (10; https://www.conserve-energy-future.com/importance-advantages-afforestation.php). Forest plantations established in the Congolese coastal plains help build healthier soil and enable economic and environmental benefits such as nontimber products, production of pulp (for the industry), and fuel wood energy (for the local population). In fact, 94% of the population relies on them for their energy needs, both on their own and in the neighboring countries of the Congo Basin (11). In addition, when well managed, these forest plantations enhance soil health and ecosystem biodiversity (6, 7, 15).
To improve soil fertility and sustain diminishing forest productivity after the first rotation, since nutrients are exported through wood harvest and poorly replaced with fertilizers (16), nitrogen-fixing trees (NFTs) such as acacias have been introduced in the eucalyptus plantations in the 1990s (17, 18). These mixed-species (acacias and eucalyptus) plantations improved soil health, i.e., soil fertility through increased N status (19–22) and enhanced phosphorus cycling in both forest floor and soil (23–25). However, the decline in available P in stands containing acacia (26) due to symbiotic N2 fixation (27) was reported. These plantations may also have the potential to enhance soil sulfur concentrations, which appear to be two to three higher than commonly observed (28), since although considered an essential plant nutrient, soil S concentration is only about 10% of that of the total N (29). Benefits have also been reported through C sequestration in soil (6, 19) and increased stand wood biomass, i.e., forest productivity (21, 30), that boost the potential to mitigate climate change through increased C stocks (6, 14, 31).
Soil habitat, i.e., its diversity, regulates the conservation and productivity of ecosystems (32). Important functions, such as decomposition of organic residues, nutrient cycling, bioturbation, suppression of soil−borne diseases and pests, and other environmental services (watershed protection, preservation of soil structure, mitigation of greenhouse gas emissions), strongly rely on soil biota (33). Afforestation and the introduction of nitrogen-fixing trees in forest plantations change soil biota, e.g., may lead to shifts in the composition and structure of faunal, microbial, bacterial, and fungal communities (34–38). Peerawat et al. (39) reported that the bacterial and macrofaunal diversity are more resistant to land-use conversion than the fungal diversity. Soil moisture favors positive correlations between mesofaunal abundance and diversity, and it drives ecological interactions between soil mesofauna and microorganisms in acacia plantations in Brazil (40). In addition, Zagatto et al. (41) reported that Acacia mangium litter has exclusive orders in the groups Symphyla, Protura, and Pseudoscorpionida, which are considered indicators of environmental quality. Acacia litter is most favorable to mesofauna compared to eucalyptus due to its high nutritional quality and above-average biomass, which induces high fauna density and diversity.
Anthropogenic activities and biogeochemical processes are obviously linked and act on both terrestrial and aquatic ecosystems and the environment with an undeniable impact on the well-being of living organisms, i.e., plants, animals, and humans (42–45). Hydrogen sulfide (H2S) is naturally present in the atmosphere at low concentrations (0.02 μl L−1) (46–48). Nevertheless, this threshold level may be surpassed in areas with volcanic activity and polluted industrial or livestock production (46, 49), such as the Congolese coastal plains in the Republic of the Congo. Regions harboring higher concentrations of H2S have higher microbial species diversity with the dominance of the Actinobacteria phylum (49), while sulfur stimulates growth and enhances the biomass of Actinobacteria, a gram-positive bacteria (42). Actinobacteria harbor a high ability to decompose a broad range of hydrocarbons, pesticides, and feather waste (50). Increased C and N stocks at 7 years of the first rotation of forest plantations (stands containing acacia, 19, 22) and phosphorus availability (afforested stands, 23) may be related to prevalence of Actinobacteria determined using metabarcoding of the 16S rRNA bacterial gene (31). Besides, H2S resulting from natural processes and enabling the presence of Actinobacteria (48), the prevalence of Actinobaceria may result from human activities (31, 49) and potentially benefit to soil attributes such as C and P dynamics. Sustaining these ecosystems strongly depends, therefore, on interactions between bacterial communities related to other soil functions, the environment, and human activities.
Up to date, few studies to evaluate microbial communities (bacterial or fungal and macrofauna) and soil biodiversity status have been conducted in the forest plantations of the Congolese coastal plains. Enhanced activity of edaphic macroarthropod communities, i.e., the dominance of cockroaches in acacia litter as opposed to ants in eucalyptus, has been reported in the forest plantations of the Congolese coastal plains (17). Albeit there is no difference in Actinobacteria, the prevalent phylum among stands, both its prevalence and strong correlation to sulfur (S) have been noticed at all levels of the taxonomy using metabarcoding of the 16S rRNA bacterial gene. In addition, a shift in the bacterial community composition was evidenced by the dominance of Proteobacteria in eucalyptus monoculture against Firmicutes in stands containing acacia (51). Therefore, further studies are needed to confirm this trend. The current review paper aims to strengthen and confirm our knowledge on (1) how, in addition to natural processes, human activities (H2S deposition from oil exploitation) can modify soil bacterial community composition and soil health; and (2) how the prevalence of Actinobacteria phyla can benefit soil attributes and boost SOM dynamics and soil health through C sequestration and/or land restoration.
Studies reporting the improvement in soil health of sandy soils in forest and agroforestry systems to fight hunger and desertification, boost forest conservation, and ecosystem biodiversity, and mitigation to climate change have been performed in Central Africa (6, 7, 19, 52, 53). Changes occurring in soil processes and functions are driven by soil organisms (54). The role of soil biota in driving and enhancing nutrient dynamics and cycling to improve soil health is crucial, as its interaction with the environment and human activities to ensure soil functions (31, 40, 41, 54). Along with changes in soil organic matter quantity and quality, enhanced activity of edaphic macroarthropod communities through the dominance of ants in eucalyptus against cockroaches in acacia litter has been reported in mixed-species forest plantations of the Congolese coastal plains (17). More than two decades later, bacterial community composition reported Actinobacteria as the dominant phylum in all stands, with Firmicutes as the second prevalent phylum in stands containing acacia and Proteobacteria in monoculture of eucalyptus (51). Anthropogenic activities affect the environment, i.e., both terrestrial and/or marine ecosystems, and may impact the soil microbiome, e.g., its richness and/or diversity and soil health (36, 40, 41, 43, 55, 56). This can be illustrated by the conceptual scheme demonstrating how human activities, the environment, and biogeochemical processes are linked to human well-being through soil health and contribute to four out of the 17 sustainable development goals (SDGs) (Sustainable Development Goals: 17 Goals to Transform our World | United Nations, Figure 1). The figure shows how activities such as oil exploitation, mining, building, and land-use change (e.g., conversion of forest to croplands) may endanger soil health and delay the achievement of these targeted SDGs of the United Nations. Human well-being is linked to SDG 3, i.e., “Good health and well-being,” while food security as the first challenge in Figure 1 is linked to SDG 2 “Zero hunger.” The second challenge, i.e., mitigating climate change, is linked to SDG 13, “Climate action.” Soil sustainable management and co-benefits as a third challenge are linked to SDGs 2 and 15, “No hunger,” and “Life on land,” respectively. The latter SDG, i.e., SGD 15, “Life on land,” is also linked to the fourth challenge, which is the conservation of biodiversity (Figure 1).
Figure 1 Human activities, the environment, and biogeochemical processes are linked to its well-being. Human well-being and challenges are linked to four out of 17 sustainable development goals (SDG) of the United Nations (Sustainable Development Goals: 17 Goals to Transform our WorldUnited Nations): SDG 2: end hunger, achieve food security, improve nutrition, and promote sustainable agriculture; SDG 3: ensure healthy lives and promote well-being for all at all ages; SDG 13: take urgent action to combat climate change and its impacts; and SDG 15: protect, restore, and promote sustainable use of terrestrial ecosystems; sustainably manage forests; combat desertification; halt and reverse land degradation; and halt biodiversity loss.
To highlight and better understand these interactions, some examples from different countries have been reported in Table 1. In the heaps of limestone mines in North-West Russia, high-throughput sequencing of soils revealed significant differences in Proteobacteria, Chloroflexi, Acidobacteria, and Actinobacteria phyla (44, Table 1). The authors noted that Actinobacteria was the only phylum presenting the most profound increase of operational taxonomic units (OTUs) (up to eight times more in 200 years of soil). H2S may become a threat to the surrounding environment and public health, as it may be a source of odors (46, 47). To acknowledge the sulfur bioconversion process in landfill covers and to establish techniques to manage odor pollution, Xia et al. (47) reported both Comamonas and Acinetobacter (Proteobacteria phylum) as genera that have a crucial role in metabolizing H2S in the waste biocover soil. The authors also argued that the decline in the pH values occurring in landfill covers is potentially a result of adsorption, absorption, and biotransformation of H2S. In the forest plantations of the Congolese coastal plains, the H2S, which probably is partially derived from oil exploration (occurring since 1969), may have enhance the prevalence of Actinobacteria phylum sensitive to S concentrations (49, 51). No difference has been noticed regarding the Actinobacteria, which harbors prevalence in all studied stands, i.e., in the monoculture acacia and eucalyptus and mixed-species stands (50% acacia and 50% eucalyptus) (51). This may have further boosted its growth and biomass, as commonly found in industrial areas (49), and enhanced organic material dynamics since Actinobacteria play a key role in decomposing organic materials (50). Increased C stocks reported in stands containing acacia (19, 22) or an increase in available phosphorus in afforested soils (23) reported in mixed-species forest plantations in coastal plains may, therefore, be linked to the prevalence of Actinobacteria phyla in the bacterial community composition of these soils (51).
Table 1 Effects of different activities on soil microbiome, soil C sequestration, and land restoration worldwide.
Other human activities, such as land-use changes, i.e., the establishment of cropping, forest and agroforestry systems, the indirect impact of human activities leading to climate change or land degradation may also change the soil microbiome and soil health (54). It has been reported that the dominant groups of bacterial communities in acid soils are commonly Acidobacteria and Proteobacteria (49, 58). An investigation of the bacterial community structure of monoculture acacia and eucalyptus forest plantations along a depth profile (0 to 800 cm) revealed the dominance of Proteobacteria and Acidobacteria (recurrent in samples between 0 and 300 cm) with the prevalence of Firmicutes and Proteobacteria in pure eucalyptus (36). Nonetheless, acid and sandy soils (pH <4.5 and >90% sand) harboring the bacterial community composition dominated by Actinobacteria, followed by Proteobacteria, Firmicutes, and Acidobacteria contained higher C and N stocks in stands containing acacia relative to eucalyptus in the Congolese coastal plains (19, 51). As oligotrophic bacteria, even in the nondominant phylum, Actinobacteria play an ecophysiological role in carbon cycling, mainly in less-fertile soils due to their relatively larger proportion of genes implicated in plant residue decomposition and crucial involvement in interspecies interactions (57). In a continuous rice cropping system in Northeast China, Yan et al. (43) outlined the dominance of Proteobacteria, Bacteroidetes, Firmicutes, Acidobacteria, and Verrucomicrobia based on their relative abundances, along with an enhanced soil microbial richness; however, the authors found no difference in soil microbial diversity. Hence, the response to the first question is given: it shows how human activities (industrial, agricultural) do change the soil bacterial communities in various ecosystems and do impact soil health and benefits and human well-being through four out of the 17 SDGs of the United Nations (Sustainable Development Goals: 17 Goals to Transform our World | United Nations, Figure 1 and Table 1).
Industrial processes such as the combustion of biomass and fossil fuels, along with livestock production, generate atmospheric H2S in addition to natural sources (46). Investigation revealed the dominance of the Actinobacteria phylum in regions with high concentrations of H2S exhibiting to what extent the sulfur cycle is influenced by the dynamic environmental conditions (49). The Actinobacteria phylum is among the most widely distributed bacterial phyla in soils that harbor a vast capacity to degenerate plant residues in vitro (50, 57, 59). As a matter of fact, Actinobacteria play an essential part in organic matter turnover, the carbon cycle, and humus formation by decomposing organic materials such as cellulose and chitin and filling up the supply of nutrients in the soil (50). In addition, Actinobacteria fix nitrogen, involving an enhanced N availability during microbial-driven plant residue decomposition (60) and the production of antibiotics (57). Both characteristics lead to strengthening their ability to procure carbon sources, preserving them from environmental perturbations (57, 59, 60), potentially enhancing C sequestration and having an impact on both mitigating climate change and restoring degraded lands. Microbial community composition is linked not only to biogeochemical nutrient cycles of N, C, S, C/N, and available P and soil fertility (Figure 2) but also to soil functions, i.e., C sequestration, N, S, O, and P cycles and availability as well as to land reclamation (Table 1). Figure 2 schematizes the fundamental role of soil organisms (e.g., soil microbial communities) within biogeochemical cycles and soil fertility to enable the regulation, conservation, and efficiency of ecosystems (32). Soil organisms are the key parameter in the physical, chemical, and biological improvement of soil fertility, enabling soil functions such as C accumulation or N mineralization. Phylum composition and abundance of the rhizomicrobial communities (Pinus tabuliformis Carr. Forests) were investigated using high-throughput DNA sequencing in North China (55). The authors reported an enhanced Actinobacteria, Proteobacteria, and Firmicutes abundance, even though the Proteobacteria were recognized as keystone organisms for root-driven C accumulation in primary succession and Basidiomycota as the core for root-driven C accumulation in climax communities (55).
Furthermore, changes due to human activities, such as afforestation, through improved soil health, i.e., enhanced soil attributes (C, N, P, S), may be linked to bacterial community composition (prevalence of Actinobacteria phylum) in the Congolese coastal plains. Increased C stocks, i.e., 0.8 t ha−1 in acacia monoculture stands (100%) and 1.9 t ha−1 in the mixed-species (50% acacia and acacia 50% eucalyptus) stands relative to the eucalyptus monoculture (100%) down to 25 cm at the end of the first 7-year rotation were reported (6, 19). Higher N stocks in stands containing acacia, i.e., 1.25 ± 0.02 t ha−1 (acacia monoculture) and 1.28 ± 0.03 t ha−1 (mixed acacia–eucalyptus) relative to 1.19 ± 0.02 t ha−1 (eucalyptus monoculture) stands were also outlined in the same depth (19). In addition, it has also been reported that during the first 2 years of the second rotation, the stands containing acacia harbored higher values of the cumulative net N stocks, i.e., 343 ± 21 kg ha−1 (acacia) and 287 ± 17 kg ha−1 (acacia–eucalyptus) compared to 189 ± 12 kg ha−1 (eucalyptus) (22). This improvement in N status is also revealed through the N concentration in coarse particulate organic matter (POM, 4,000–250 µm), which was 30% higher in acacia (100%) than in eucalyptus (100%) stands at year 2 of the second 7-year rotation at 0–5 cm depth (20). Besides the increase in C and N concentrations in stands containing acacia compared to eucalyptus, available phosphorus (P) was lower in stands containing acacia relative to eucalyptus, while the contrary tendency was observed for S (28). Higher S concentrations were reported in stands containing acacia harboring the higher C stocks relative to eucalyptus monoculture (6, 28). Even though P is needed for the symbiotic atmospheric N2 fixation for the nitrogen-fixing species (27), and a decline in available P was reported in stands containing acacia (26), all afforested stands contained more available P than natural savannas (23). This highlights the targeted ecosystem’s potential for mitigating climate change (C sequestration), contributing to both food security through improved soil fertility (mainly N), sustainable soil management (land restoration), and human welfare, i.e., and four (2, 3, 13, and 15) of the seventeen SDGs of the United Nations (6, 14, 28, 31, 37). Different studies published worldwide confirmed the strong reliance of forest plantation ecosystems, especially those on inherently nutrient-poor soils, such as those of the Congolese coastal plains and the entire Congo Basin (6, 11, 31), on the link between soil functioning (C sequestration, nutrient cycling, etc.), the environment, and human activities driven by soil organisms.
Human activities may shape the environment and affect processes within plants, soils, and the environment driven by soil biota (31, 35, 44, 54). In the review, these impacts have been evaluated mainly at the soil level, especially with regard to its health (37, 40, 41, 52, Figure 3). It is well known that SOM dynamics involve changes in soil health and enhance soil functions, with further impact on ecosystem biodiversity and the environment (32, 35, 61). Soil functions have the potential to influence human health by enhancing or inhibiting some processes that are regulated by soil organisms, leading to secure food, mitigating climate change, and sustaining soil management (land restoration) and ecosystem biodiversity (35, 37, 53). The overall schematic representation of the review is considering soil, especially its health, which relies on the interaction between the environment, soil functions driven by soil organisms, and human activities and well-being. At the very beginning are the human activities that positively or negatively impact soil health. Negative impacts may be direct as those mentioned in this review, i.e., deforestation, building (roads, houses), mining, and oil exploitation. There are also indirect negative impacts, such as those linked to climate change or land degradation initiated by humans.
Figure 3 Impacts of human activities on some direct benefits and disadvantages on soil health. Healthy soil is linked to seven out of 17 sustainable development goals (SDG) of the United Nations (Sustainable Development Goals: 17 Goals to Transform our WorldUnited Nations): SDG 1: end poverty in all its forms everywhere; SDG 2: end hunger, achieve food security, improve nutrition, and promote sustainable agriculture; SDG 3: ensure healthy lives and promote well-being for all at all ages; SDG 6: ensure availability and sustainable management of water and sanitation for all; SDG 7: ensure access to affordable, reliable, sustainable, and modern energy for all; SDG 13: take urgent action to combat climate change and its impacts; and SDG 15: protect, restore, and promote sustainable use of terrestrial ecosystems; sustainably manage forests; combat desertification; halt and reverse land degradation; and halt biodiversity loss.
Positive impacts of human activities may also be direct and indirect. Only direct impacts have been considered in this review (good agricultural practices, agroforestry, afforestation, and reforestation). These human activities foster soil health by enabling goals such as food security, reduction of poverty, adaptation and resilience to climate change, fighting pollution and desertification, restoring degraded lands, preserving water resources, boosting ecosystem biodiversity, and sustaining forest productivity (providing wood and fuel energy and conserving natural forests). These goals may contribute to seven out of the 17 United Nations SDGs (Sustainable Development Goals: 17 Goals to Transform our World | United Nations), i.e., SDG 1 “No poverty,” SDG 2 “Zero hunger,” SDG 3 “Good health and Well-being,” SDG 6–”Clean water and sanitation,” SDG 7 “Affordable and clean energy,” SDG 13 “Climate action,” and SDG 15 “Life on land.” On the contrary, human activities inducing negative impacts on soil health will threaten the mentioned above goals, i.e., the seven out of 17 SDGs (Figure 3).
Carbon sequestration, enhanced soil biodiversity, increased nutrient availability, and soil aggregation are among the benefits resulting from the positive impacts of human activities on soil health. Disadvantages (erosion, compaction loss of biodiversity, loss of chemical fertility, salinity, and acidification) on the opposite lessen the ability of soil to fulfill its functions and meet the seven out of 17 SDGs of the United Nations. It is very important to notice that soil microbial communities, i.e., soil organisms in general, are at the center of this schematic representation (Figures 2, 3). It shows that soil organisms drive and regulate soil functions at the first level and then ecosystem services at the second level through soil functions (31–33, 56). Human activities may shape the environment (42, 43), but the functions of soil, ecosystem, and environment rely strongly on their interaction i.e., the interaction between soil organisms, plants, environment, and human activities.
This review highlights the potential impact of human activities on soil attributes in general and on soil bacterial community composition in particular. These impacts may positively or negatively affect soil attributes and boost or lessen soil health. It is also reported that the sustainability of forest plantations on infertile soils strongly relies on soil organisms that regulate the interaction between soil functions, the environment, and human activities. The current findings will not only help sustain forest productivity and promote soil health at the physical, chemical, and biological levels but also enable population welfare. They will also help to prevent land degradation, mitigate climate change, provide (wood and fuel energy), i.e., leading to preserve natural forests in the entire region of the Congo Basin, and finally, contribute to seven out of the 17 SDG United Nations goals.
Furthermore, the beneficial impact of the predominant phylum Actinobacteria on SOM decomposition is revealed by improved soil attributes (increased C, N stocks, and P availability). The potential impact of human activities (oil exploitation) on the bacterial community of forest ecosystems in the Congolese coastal plains must be confirmed. Further studies must be conducted on the forest plantations located in the coastal plains, areas neighboring Brazzaville, and the northern part of the country. These studies should attest to the strong reliance of forest plantations on the interaction between soil functions, human activities, and the environment. They will also show how the attributes of fragile and inherently infertile soils are strongly linked to the vegetation cover (afforestation) and the environment, determining their development and sustainability as well as the prevention of their degradation.
The author confirms being the sole contributor of this work and has approved it for publication.
L-SK thanks Tamas Onodi for editing and the two reviewers for their suggestions, advice, and commitment to improving the paper.
The author declares that the research was conducted in the absence of any commercial or financial relationships that could be construed as a potential conflict of interest.
All claims expressed in this article are solely those of the authors and do not necessarily represent those of their affiliated organizations, or those of the publisher, the editors and the reviewers. Any product that may be evaluated in this article, or claim that may be made by its manufacturer, is not guaranteed or endorsed by the publisher.
1. Parr CL, Lehmann CE, Bond WJ, Hoffmann WA, Andersen AN. Tropical grassy biomes: Misunderstood, neglected, and under threat. Trends Ecol Evol (2014) 29(4):205–13. doi: 10.1016/j.tree.2014.02.004
2. Silveira FAO, Arruda AJ, Bond W, Durigan G, Fidelis A, Kirkman K, et al. Myth-busting tropical grassy biome restoration. Restor. Ecol (2020) 28(5):1067–73. doi: 10.1111/rec.13202
3. Makany L. La côte atlantique du Congo cadres géographiques et géologiques, leur influence sur la répartition de la végétation et sur les possibilités agricoles du territoire. Symposium Sci (1964), 891–907.
4. Delwaulle JC, Garbaye J, Laplace Y. Ligniculture en milieu tropical: Les reboisements en eucalyptus hybrides de la savane côtière Congolaise. Rev For Francaise (1981) 3:248–55. doi: 10.4267/2042/21511
5. Schwartz D, Namri M. Mapping the total organic carbon in the soils of the Congo. Glob Planet. Change (2002) 33:77–93. doi: 10.1016/S0921-8181(02)00063-2
6. Koutika L-S, Taba K, Ndongo M, Kaonga M. Nitrogen-fixing trees increase organic carbon sequestration in forest and agroforestry ecosystems in the Congo basin. Reg. Environ Change (2021) 21:109. doi: 10.1007/s10113-021-01816-9
7. Koutika L-S, Mabicka Obame R, Kouba Nkouamoussou C, Musadji N-Y. Research priorities for sandy soils in central Africa. Geoderma Regional (2022) 29:e00519. doi: 10.1016/j.geodrs.2022.e00519
8. Mareschal L, Nzila JDD, Turpault MP, M’Bou AT, Mazoumbou JC, Bouillet JP, et al. Mineralogical and physico-chemical properties of ferralic arenosols derived from unconsolidated plio-pleistocenic deposits in the coastal plains of Congo. Geoderma (2011) 162:159–70. doi: 10.1016/j.geoderma.2011.01.017
9. Paquette A, Messier C. The role of plantations in managing the world’s forests in the anthropocene. Front Ecol. Environ (2010) 8:27–34. doi: 10.1890/080116
10. Pérez-Silos I, Álvarez-Martínez JM, Barquín J. Large-Scale afforestation for ecosystem service provisioning: Learning from the past to improve the future. Landscape Ecol (2021) 36:3329–43. doi: 10.1007/s10980-021-01306-7
11. Shure J, Marien JN, de Wasseige C, Drigo R, Salbitano F, Dirou S, et al. Contribution du bois énergie à la satisfaction des besoins énergétiques des populations d’Afrique centrale: Perspectives pour une gestion durable des ressources disponibles. Chap (2012) 5:109–22. doi: 10.2788/48830
12. Bauters M, Ampoorter E, Huygens D, Kearsley E, De Haulleville T, Sellan G, et al. Functional identity explains carbon sequestration in a 77-year-old experimental tropical plantation. Ecosphere (2015) 6(10):198. doi: 10.1890/ES15-00342.1
13. Lal R. Restoring soil quality to mitigate soil degradation. Sustainability (2015) 7:5875–95. doi: 10.3390/su7055875
14. Rumpel C, Amiraslani F, Chenu C, Garcia-Cardenas M, Kaonga M, Koutika LS, et al. The 4p1000 initiative: Opportunities, limitations and challenges for implementing soil organic carbon sequestration as a sustainable development strategy. Ambio (2020) 49(1):350–60. doi: 10.1007/s13280-019-01165-2
15. Lescuyer G, Karsenty A, Eba’a Atyi R. A new tool for sustainable forest management in central Africa: Payments for environmental services. In: Wasseige C, Marcken P, Atyi RE, Nasi R, Mayaux P, editors. The forests of the Congo basin: state of the forest 2008 (2009). CIFOR, DR Congo p. 131–43.
16. Laclau JP, Ranger J, Deleporte P, Nouvellon Y, Saint André L, Marlet S, et al. Nutrient cycling in a clonal stand of eucalyptus and an adjacent savanna ecosystem in congo. 3. Input-output budget and consequences for the sustainability of the plantations. For Ecol Manage (2005) 210:375–91. doi: 10.1016/j.foreco.2005.02.028
17. Bernhard-Reversat F. Dynamics of litter and organic matter at the soil-litter interface of fast-growing tree plantations on sandy ferrallitic soils (Congo). Acta Oecol (1993) 14(2):179–95.
18. Bouillet J-P, Laclau JP, Gonçalves JLM, Voigtlaender M, Gava JL, Leite FP, et al. Eucalyptus and acacia tree growth over entire rotation in single- and mixed-species plantations across five sites in Brazil and Congo. For Ecol Manage (2013) 301:89–101. doi: 10.1016/j.foreco.2012.09.019
19. Koutika L-S, Epron D, Bouillet J-P, Mareschal L. Changes in n and c concentrations, soil acidity and p availability in tropical mixed acacia and eucalypt plantations on a nutrient-poor sandy soil. Plant Soil (2014) 379:205–16. doi: 10.1007/s11104-014-2047-3
20. Koutika L,-S, Tchichelle SV, Mareschal L, Epron D. Nitrogen dynamics in a nutrient-poor soil under mixed-species plantations of eucalypts and acacias. Soil Biol Biochem (2017) 108:84–90. doi: 10.1016/j.soilbio.2017.01.023
21. Tchichelle VS. Production de biomasse et quantification des flux d’azote dans une plantation mixte d’Eucalyptus urophylla x grandis et d’Acacia mangium au Congo. France: Thèse de l’Université de Lorraine (2016). p. 92. Available at: https://scanr.enseignementsup-recherche.gouv.fr/publication/these2016LORR0115.
22. Tchichelle SV, Epron D, Mialoundama F, Koutika LS, Harmand JM, Bouillet JP, et al. Differences in nitrogen cycling and soil mineralization between a eucalypt plantation and a mixed eucalypt and Acacia mangium plantation on a sandy tropical soil. South Forests (2017) 79(1):1–8. doi: 10.2989/20702620.2016.1221702
23. Koutika LS, Mareschal L. Acacia and eucalypt change p, n and c concentrations in POM of arenosols in the Congolese coastal plains. Geoderma Reg (2017) 11:37–43. doi: 10.1016/j.geodrs.2017.07.009
24. Koutika L-S. Afforesting tropical savannas with Acacia mangium and eucalyptus improves soil p availability in arenosols of the Congolese coastal plains. Geoderma Reg (2019) 16:e00207. doi: 10.1016/j.geodrs.2019.e00207
25. Koutika LS, Cafiero L, Bevivino A, Merino A. Organic matter quality of forest floor as a driver of c and p dynamics in acacia and eucalypt plantations established on a ferralic arenosols, congo. for. ecosyst (2020) 7:40. doi: 10.1186/s40663-020-00249-w
26. Koutika L-S, Mareschal L, Epron D. Soil p availability under eucalypt and acacia on ferralic arenosols, republic of the congo. Geoderma Regional (2016) 7(2):153–8. doi: 10.1016/j.geodrs.2016.03.001
27. Binkley D, Cannell MGR, Malcom DC, Robertson PA. Mixtures of N2-fixing and non-N2-fixing tree species. In: The ecology of mixed species stands of trees, vol. 60290. Oxford: Blackwell Scientific Publications (1992). p. 53. Available at: http://www.agris.fao.org/agris-search/search.
28. Koutika L-S, Ngoyi S, Cafiero L, Bevivino A. Soil organic matter quality along rotations in acacia and eucalyptus plantations in the congolese coastal plains. For Ecosyst (2019) 6:39. doi: 10.1186/s40663-019-0197-8
29. Haneklaus S, Schnug E. The global sulphur cycle and its link to plant environment. In: Sulphur in plants. Dordrecht, The Netherlands: Kluwer Academic Publishers (2003). p. 1–28.
30. Epron D, Nouvellon Y, Mareschal L, Moreira RM, Koutika LS, Geneste B, et al. Partitioning of net primary production in Eucalyptus and Acacia stands and in mixed-species plantations: Two case-studies in contrasting tropical environments. For Ecol Manage (2013) 301:102–11. doi: 10.1016/j.foreco.2012.10.034
31. Koutika L-S, Zagatto MRG, Pereira A, Miyittah M, Tabacchioni S, Bevivino A, et al. Does the introduction of N2-fixing trees in forest plantations on tropical soils ameliorate low fertility and enhance carbon sequestration via interactions between biota and nutrient availability? Case studies from central Africa and south America. Front Soil Sci (2021) 1:752747. doi: 10.3389/fsoil.2021.752747
32. Brussaard L, Behan-Pelletier VM, Bignell DE, Brown VK, Didden W, Folgarait P, et al. Biodiversity and ecosystem functioning in soil. Ambio (1997) 26:563−570.
33. Bignell DE, Tondoh J, Dibog L, Huang SP, Moreira F, Nwaga D, et al. Slash−and−burn agriculture: the search for alternatives. New York: Columbia University Press (2005). p. 119−142.
34. Bini D, Figueiredo AF, da Silva MCP, de Figueiredo Vasconcellos RL, Cardoso EJBN. Microbial biomass and activity in litter during the initial development of pure and mixed plantations of of Eucalyptus grandis and Acacia mangium. revue brasilier. Cienca Solo (2012) 37:76–85. doi: 10.1590/S0100-06832013000100008
35. Bini D, dos Santos CA, Bouillet J-PP, Gonçalves JLM, Cardoso EJBN. Eucalyptus grandis and acacia mangium in monoculture and intercropped plantations: evolution of soil and litter microbial and chemical attributes during early stages of plant development. Appl Soil Ecol (2013) 63:57–66. doi: 10.1016/j.apsoil.2012.09.012
36. Pereira AP, de Andrade PA, Bini D, Durrer A, Robin A, Bouillet J-P, et al. Shifts in the bacterial community composition along deep soil profiles in monospecific and mixed stands of eucalyptus grandis and acacia mangium. PloS One (2017) 12(7):e0180371. doi: 10.1371/journal.pone.0180371
37. Pereira APA, Zagatto MRG, Brandani CB, Mescolotti D, Cotta SR, Gonçalves JLM, et al. Acacia changes microbial indicators and increases c and n in soil organic fractions in intercropped eucalyptus plantations. Front Microb (2018) 9:655. doi: 10.3389/fmicb.2018.00655
38. Pereira APA, Santana MC, Zagatto MRG, Brandani CB, Wang J-T, Verma JP, et al. Nitrogen-fixing trees in mixed forest systems regulate the ecology of fungal community and phosphorus cycling. Sci. Total Environ (2020) 758:143711. doi: 10.1016/j.scitotenv.2020.143711
39. Peerawat M, Blaud A, Trap J, Chevallier T, Alonso P, Gay F, et al. Rubber plantation ageing controls soil biodiversity after land conversion from cassava. Agric Ecosyst Environ (2018) 257:92–102. doi: 10.1016/j.agee.2018.01.034
40. Zagatto MRG, Pereira APA, Souza AJ, Pereira RF, Baldesin LF, Pereira CM, et al. Interactions between mesofauna, microbiological and chemical soil attributes in pure and intercropped eucalyptus grandis and acacia mangium plantations. For Ecol Manage (2019) 433:240–7. doi: 10.1016/j.foreco.2018.11.008
41. Zagatto MRG, Pereira APA, Souza AJ, Pereira CM, Baldesin LF, Pereira RF, et al. Acacia mangium increases the mesofauna density and diversity in the litter layer in eucalyptus grandis plantations. Eur J Soil Biol (2019) 94:103100. doi: 10.1016/j.ejsobi.2019.103100
42. Xu Y, Fan J, Ding W, Bol R, Chen Z, Luo J, et al. Stage-specific response of litter decomposition to n and s amendments in a subtropical forest soil. Biol Fertil Soils (2016) 52:711–24. doi: 10.1007/s00374-016-1115-7
43. Yan S, Song J, Fan J, Yan C, Dong S, Ma C, et al. Changes in soil organic carbon fractions and microbial community under rice straw return in northeast China. Global Ecol Conserv (2020) 22:e00962. doi: 10.1016/j.gecco.2020.e00962
44. Abakumov E, Zverev A, Kichko A, Kimeklis A, Andronov E. Soil microbiome of different-aged stages of selfrestoration of ecosystems on the mining heaps of limestone quarry (Elizavetino, Leningrad region). GRUYTER Open Agric (2021) 6:57–66. doi: 10.1515/opag-2020-0207
45. Dontsova K, Balogh-Brunstad Z, Le Roux G. Evaluating the impact and reach of biogeochemical cycles. Eos (2021) 102. doi: 10.1029/2021EO163024
46. Watts SF. The mass budgets of carbonyl sulfide, dimethyl sulfide, carbon disulfide and hydrogen sulfide. Atmos. Environ (2000) 34:761–79. doi: 10.1016/S1352-2310(99)00342-8
47. Xia FF, Zhang HT, Wei XM, Su Y, He R. Characterization of H2S removal and microbial community in landfill cover soils. Environ Sci Pollut Res Int (2015) 18906-17. doi: 10.1007/s11356-015-5070-x
48. Ausma T, De Kok LJ. Atmospheric H2S: Impact on plant functioning. front. Plant Sci (2019) 10:743. doi: 10.3389/fpls.2019.00743
49. Dong Q, Shi H, Liu Y. Microbial character related sulfur cycle under dynamic environmental factors based on the microbial population analysis in sewerage system. Front Microbiol (2017) 8:64. doi: 10.3389/fmicb.2017.00064
50. Anandan R, Dharumadurai D, Manogaran GP. An introduction to actinobacteria. In: Actinobacteria - basics and biotechnological applications. Intech Open Sciences and Open minds (2016). p. pp 3–37. doi: 10.5772/62329
51. Koutika L-S, Fiore A, Tabacchioni S, Aprea G, Pereira APA, Bevivino A. Influence of acacia mangium on soil fertility and bacterial community in eucalyptus plantations in the congolese coastal plains. Sustainability (2020) 12:8763. doi: 10.3390/su12218763
52. Kasongo RK, Van Ranst E, Verdoodt A, Kanyankagote P, Baert G. Impact of Acacia auriculiformis on the chemical fertility of sandy soils on the batéké plateau, D.R. Congo. Soil Use Manage (2009) 25:21–7. doi: 10.1111/j.1475-2743.2008.00188.x
53. Dubiez E, Freycon V, Marien JM, Peltier R, Harmand JM. Long term impact of Acacia auriculiformis woodlots growing in rotation with cassava and maize on the carbon and nutrient contents of savannah sandy soils in the humid tropics (Democratic republic of Congo). Agroforest Syst (2019) 93:1167–78. doi: 10.1007/s10457-018-0222-x
54. Bastida F, Eldridge DJ, García C, Png GK, Bardgett RD, Delgado-Baquerizo M. Soil microbial diversity–biomass relationships are driven by soil carbon content across global biomes. ISME J (2021) 15:2081–91. doi: 10.1038/s41396-021-00906-0
55. Song W, ID Liu Y. Microbial taxa and soil organic carbon accumulation driven by tree roots. Forests (2018) 9:333. doi: 10.3390/f9060333
56. Dong X, Gao P, Zhou R, Li C, Dun X, Niu X. Changing characteristics and influencing factors of the soil microbial community during litter decomposition in a mixed quercus acutissima carruth. and robinia pseudoacacia l. forest in northern China. Catena (2021) 196:104811. doi: 10.1016/j.catena.2020.104811
57. Bao Y, Dolfing J, Guo Z, Chen R, Wu M, Li Z, et al. Important ecophysiological roles of nondominant actinobacteria in plant residue decomposition, especially in less fertile soils. Microbiome (2021) 9:84. doi: 10.1186/s40168-021-01032-x
58. Lauber CL, Hamady M, Knight R, Fierer N. Pyrosequencing-based assessment of soil pH as a predictor of soil bacterial community structure at the continental scale.App. Environ Microb (2009) 75:5111–20. doi: 10.1128/AEM.00335-09
59. van Bergeijk DA, Terlouw BR, Medema MH, van Wezel GP. Ecology and genomics of actinobacteria: new concepts for natural product discovery. Nat Rev Microbiol (2020) 18(10):546–58. doi: 10.1038/s41579-020-0379-y
60. Swarnalakshmi K, Senthilkumar M, Ramakrishnan B. Endophytic actinobacteria: nitrogen fixation, phytohormone production, and antibiosis. In: Subramaniam G, Arumugam S, Rajendran V, editors. Plant growth promoting actinobacteria: a vew avenue for enhancing the productivity and soil fertility of grain legumes. Singapore: Springer Singapore (2016). p. 123–45. doi: 10.1007/978-981-10-0707-1_8
Keywords: human activities, forest plantations, soil bacterial communities, C sequestration, soil attributes (C,N,P, S)
Citation: Koutika L-S (2022) How hydrogen sulfide deposition from oil exploitation may affect bacterial communities and the health of forest soils in Congolese coastal plains? Front. Soil Sci. 2:920142. doi: 10.3389/fsoil.2022.920142
Received: 14 April 2022; Accepted: 07 July 2022;
Published: 02 August 2022.
Edited by:
Bahar S. Razavi, University of Kiel, GermanyReviewed by:
Diogo Paes da Costa, Federal University of Agreste of Pernambuco (UFAPE), BrazilCopyright © 2022 Koutika. This is an open-access article distributed under the terms of the Creative Commons Attribution License (CC BY). The use, distribution or reproduction in other forums is permitted, provided the original author(s) and the copyright owner(s) are credited and that the original publication in this journal is cited, in accordance with accepted academic practice. No use, distribution or reproduction is permitted which does not comply with these terms.
*Correspondence: Lydie-Stella Koutika, bHNfa291dGlrYUB5YWhvby5jb20=
Disclaimer: All claims expressed in this article are solely those of the authors and do not necessarily represent those of their affiliated organizations, or those of the publisher, the editors and the reviewers. Any product that may be evaluated in this article or claim that may be made by its manufacturer is not guaranteed or endorsed by the publisher.
Research integrity at Frontiers
Learn more about the work of our research integrity team to safeguard the quality of each article we publish.