- 1State Key Laboratory of Hydroscience and Engineering, Department of Hydraulic Engineering, Tsinghua University, Beijing, China
- 2Department of soil and Water Sciences, College of Land Science and Technology, China Agricultural University, Beijing, China
- 3Cultivated Land and Plant Protection and Plant Inspection Station of Kunshan, Kunshan, China
- 4State Key Laboratory of Pollution Control and Resource Reuse, College of Environmental Science and Engineering, Tongji University, Shanghai, China
Organic cultivation has been considered as an important cultivation approach for sustainable agriculture in the world. Whether organic cultivation can mitigate the negative impact of agriculture on the environment especially in greenhouses is still unclear. The purpose of this study is to investigate the long-term impacts in soil fertility and environment quality through organic cultivation (OC), low-input cultivation (LC) and conventional cultivation (CC) in greenhouses after 15 years of cultivation. We found that the soil organic carbon (SOC) content in the OC treatment was 1.7 times of that in CC, 1.2 times of that in the LC treatments. Vegetable yield and the content of alkali nitrogen, available phosphorus and available potassium in the OC treatment was significantly higher than those in the LC and CC treatments. Due to the high input of organic fertilizers, increased content of heavy metals (Cu, Zn, Pb, Cd, Cr and As) were observed in the OC treatment. In addition, organic cultivation resulted in considerable residue accumulation of tetracycline antibiotics (TCs) and pesticides in the soil. Ecological risk assessment of soil pollutants showed that organic cultivation has the highest ecological risk index. At present, organic partial substitution or low-input cultivation could be a promising approach for the development of sustainable agriculture.
1 Introduction
Industrialized agriculture has been feeding the growing world population, but large-scale land conversion and excessive application of synthetic fertilizers and pesticides have led to considerable adverse environmental impacts and human health, such as soil quality degradation, water eutrophication, groundwater pollution, accumulation of heavy metal, greenhouse gas emissions and biodiversity losses (1, 2). Many studies have shown that application of chemical fertilizers and pesticides reduced soil pH and increased reactive nitrogen in the soil, and the nitrogen oxides produced by nitrogen fertilizer could also damage the ozone layer in the atmosphere (3, 4).
Organic cultivation has been considered to be one of the most important measures for sustainable agriculture in the world, which could provide high quality food while protecting the environment. It improves soil fertility through crop rotation, planting legumes and the application of organic fertilizers, at the same time prohibits the use of any synthetic fertilizers and pesticides to maintain a sustainable agricultural system (5, 6). Long-term organic farming experiments have shown that the organic system improved soil quality. Soil organic carbon (SOC) content is significantly higher than in conventional systems and the nutrient contents were elevated as well (5). Organic cultivation has also been considered an important measure to reduce greenhouse gases because of its potential to sequester SOC (7). Organic cultivation also significantly increased soil microbial diversity by applying organic fertilizers without any synthetic fertilizers and pesticides, which protected the soil from pathogen infection and helped in degrading external pollutants (8).
Greenhouses leads to the decline of soil quality due to high cropping index, large agrochemical input, and closed environment. Agricultural production in greenhouses has become a popular crop production system globally, which provide a suitable environment for crop growth by artificial adjustment of temperature, humidity and light, thus improving yields. 20 million hectares (Mha) of land were used in agricultural production and 700 Mt of vegetables were produced in China in 2014, with 20% of the land and 35% of produced vegetables from greenhouses (9). However, soil acidification and salinization (10), loss of soil organic matter, accumulation of heavy metal (11) and pesticides (12) have severely affected the sustainability of greenhouse production and threatened the ecological environment and human health. Jiao et al. (13) reported that long-term repeated application of chemical fertilizers, metal-containing pesticides and fungicides will gradually increase their levels in agricultural soils. Some studies have shown that the mean concentration of heavy metals (Pb, Cu and Zn) in greenhouse soils is higher than those of local agricultural soils and natural background levels in eastern China (11). Low-input chemical and pesticide cultivation (LC) and organic cultivation (OC) that prohibit any chemicals and pesticides have emerged to alleviate the threats to the ecological environment caused by conventional cultivation (CC) in greenhouses.
The integral consequences on soil fertility and environmental quality in CC, LC, and OC systems have not been systematically investigated yet. This study evaluated results of a 15-year experiment on crop yield, soil fertility quality, soil environmental quality, and ecological risks in CC, LC and OC treatments in greenhouses. We are aiming to evaluate the overall impact of different agricultural systems on soil fertility (nutrient availability) and soil environmental quality (heavy metals, antibiotics and pesticides) in greenhouses, and has implications to the fertilization strategies for improving the soil environmental quality of organic vegetables, and promote the sustainable development of organic vegetable production in greenhouse.
2 Materials and methods
2.1 Site area
The long-term greenhouse production experiment site was established in March 2002 in the Quzhou Experimental Station (36° 52’ N, 115° 01’ E) of China Agricultural University in Quzhou County, Hebei Province, China (Figure 1). The average annual temperature is 13.1°C and the average annual precipitation is 556.2 mm. The experiments were conducted with two crop seasons per year (spring and autumn). The main types of greenhouse vegetables were tomato, celery, cucumber, fennel and eggplant, and the vegetables cultivated each year were shown in Table S1. The salinized cinnamon soil in the greenhouses consists of 54.11% sand, 28.45% silt, and 17.44% clay. Before the long-term experiment, the top soil (0–20 cm depth) contained organic carbon of 9.82 g kg−1, total nitrogen of 1.24 g kg−1, total phosphorus of 1.61 g kg−1, alkaline nitrogen of 108.34 mg kg−1, Olsen phosphorus of 139.13 mg kg−1, and exchangeable potassium of 278.14 mg kg−1.
2.2 Experimental design
This experiment included three treatments: conventional cultivation (CC), low-input cultivation (LC) and organic cultivation (OC), with three replicates for each. The area of each greenhouse is 364 m2 (52 m×7 m). Each greenhouse consisted three plots with an area of 9 m × 7 m, and the plots were placed 4 m apart. The cultivation method and timing are consistent with local production operation in greenhouses. The fertilizations for those treatments include: (1) OC: only organic fertilizer for 115 t ha-1 year-1 without any chemical fertilizer. This fertilizer (N 1.21%, P2O5 0.60%, K2O 1.58%) was derived from agricultural waste, including cow dung, chicken droppings, cotton shell, and crop stalks, which were processed through microbial fermentation and suitable decomposition. The contents of nutrients, heavy metals, antibiotics and pesticide residues in organic fertilizers are shown in Table S2. Pest control relies mainly on physical control, such as manual insect catching, and biological agents. (2) LC: 58 t ha-1 year-1 of organic fertilizer and 4 t ha-1 year-1 of chemical fertilizer. Pest controls are mainly through biological methods. In some severe cases, low-toxic and low-residue chemical pesticides were used. (3) CC: 8 t ha-1 year-1 of chemical fertilizer and 26.8 t ha-1 year-1 of organic fertilizer. The chemical fertilizers include urea (N 46%), calcium superphosphate (P2O5 12%), potassium sulfate (K2O 50%), and diammonium phosphate (N 18%, P2O5 46%). Agricultural antibiotics and chemical pesticides were used to control diseases and pests. The pesticide input and irrigation rates in the three cultivation systems are shown in Table S3.
2.3 Soil sample collection and analysis
Soil samples were collected on May 17, 2017. Soil samples of the 0-20 cm layer were collected at 5-8 locations at each plot following a “S” curve using a 5 cm diameter coring device. The collected fresh soil samples were sent to the laboratory in plastic bags at 4 °C. After removing debris from fresh soil samples and passing through a 2-mm sieve, part of fresh soil was stored at -20 °C for the determination of antibiotic and pesticide residues. The rest of the soil samples were air-dried and used for the determination of SOC and heavy metals after passing through 2-mm and 0.15-mm sieves, respectively. Historical data on soil samples were provided by the experimental station.
Vegetable yields are measured at each plot, with the average values reported for vegetable yields. SOC was measured following the K2Cr2O7 oxidation method. Alkaline hydrolysis nitrogen (AN) was determined using the 0.5M NaOH alkaline hydrolysis diffusion method. Available phosphorus (AP) in soil was determined by processing the samples with 0.5 M NaHCO3, and measured calorimetrically using molybdate. Available potassium (AK) was extracted using ammonium acetate, and then determined by a flame photometry. The total concentrations of heavy metals in the soil (Cu, Zn, Pb, Cd, Cr and As) were determined by microwave-assisted digestion ICP-MS in reference to China National Standard (HJ832-2017). Tetracycline antibiotics (TCs) are a class of widely used antibiotic, including oxytetracycline (OTC), chlortetracycline (CTC), doxycycline (DC) and tetracycline (TC), which are measured in this study. Antibiotics were determined using high performance liquid chromatography in reference to China National Standard (GB/T 32951-2016). As organochlorine pesticides have long been banned, the organic pesticide residues tested in this study are mainly organophosphorus, including dimethoate, chlorpyrifos, chlorfenvinphos and profenofos, which are widely used in the local area. The pesticides were determined by gas chromatography in reference to the China National Standard (GB/T 14552-2003).
2.4 Ecological risk assessment method
The accumulation of heavy metals in the soil was determined by Eq.1 and Eq.2 (14).
Where Ci is the concentration of metals in soil, Si is the initial metals concentration in soil, (Ci/Si)max is the maximum metal accumulation index, Pi is the single factor cumulative index, P is the comprehensive accumulation index, (Ci/Si)ave is the average metal accumulation index. In general, P ≤ 0.7 means safe, 0.7≤P ≤ 1 is the warning value, 1≤P ≤ 2 means slight pollution, 2≤P ≤ 3 is medium pollution, and P≥3 is heavy pollution.
A potential ecological risk index was proposed by Hakanson (15), which was used to evaluate the impact of heavy metals in soil on ecological environment. The single potential ecological risk index (Ei ) and potential ecological risk index of heavy metals (RI ) were determined by Eq.3 and Eq.4, respectively (15).
Where Ei is the single potential ecological risk index, Ci is the concentration of metals determined by experiments, Co is the concentration of metals specified in 《HJ 333-2006 Environmental quality evaluation standard for farmland of greenhouse vegetables production》, Ti is the toxicity coefficient of metals. The toxicity coefficient of Cu, Zn, Pb, Cd, Cr, As and Ni are 5, 1, 5, 30, 2, 10 and 5, respectively. RI is the potential ecological risk index of heavy metals.
The risk quotient (RQ) was calculated according to Eq. (5) - (7) for antibiotic data (16, 17).
Where PNECwater is the ineffective stress concentration of antibiotics in water (μg L-1); EC50 is the concentration for 50% of maximal effect (mg L-1); AF is the evaluation factor; PNECsoil is the ineffective stress concentration of antibiotics in soil (μg kg-1); Kd is the soil-water distribution coefficient (L kg-1); MEC is the measured content of antibiotics (μg kg-1). Considering the worst scenario, the EC50 of the most sensitive species and the maximum mass concentration of antibiotics were used to calculate RQ (18). Algae and bacteria were selected as the sensitive species for TC, DC, OTC and CTC for ecological risk assessment. The toxicity data of algae and Kd of antibiotics were obtained from the ecotoxicology literature (17). Considering the differences between species, AF was set to 1000 based on the lowest acute toxicity data reported in the literature (19). PNECsoilof TCs is shown in Table S4.
The probability risk function (Ф) of pesticides was calculated according to formula (8) - (10) using the pesticide data (20).
HC5 is the 5% of the harmful concentration; xm is the average value after logarithmic transformation of toxicity data; sm is the standard deviation after logarithmic transformation of toxicity data; kl is the extrapolation constant; c is the concentration of pollutants in soil; A is different pesticide residues. Toxicity data of representative organisms were shown in Table S5. Two different confidence levels of HC5 were calculated according to formula (8), as shown in Table S6.
2.5 Data analysis
Relevant data tables were obtained using Microsoft EXCEL (2016). A one-way analysis of variance conducted with the IBM SPSS Statistics 20.0 program (IBM Corporation, USA) were used to study the effects of different treatments on vegetable yield, SOC content, heavy metals, antibiotics and pesticide residues.
3 Results
3.1 Positive effect of long-term organic cultivation on soil fertility
3.1.1 Vegetable yields
After 15 years of cultivation, the yield of vegetables in the organic system was higher than that in the other two systems, both in spring and autumn. The yield of spring vegetables in OC increased by 3.21%-62.28% compared to that in CC, and increased by 2.18%-24.34% compared to that in LC. There were significant differences observed in the years of 2005, 2006, 2013, 2014, 2015 and 2016. The yield of autumn vegetables in OC increased by 4.52% -34.84% and 1.46% -23.59%, compared with that in CC and LC, respectively (Figure 2).
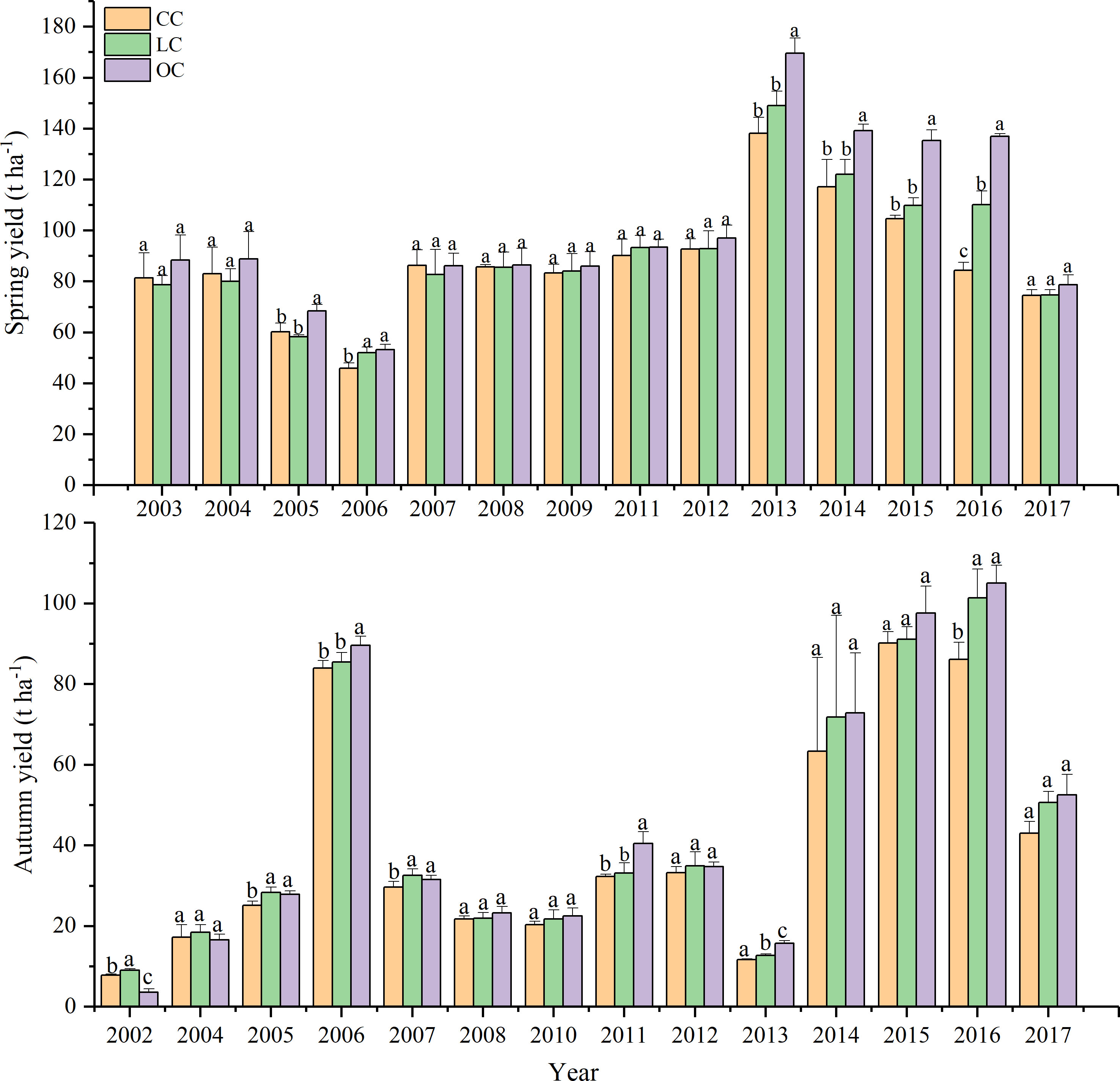
Figure 2 Spring and autumn vegetable yields with the three different cultivation methods (organic cultivation, low-input cultivation, and conventional cultivation) from 2002 to 2017. Lowercase letters indicate significant differences in different treatments in the same year.
3.1.2 Soil organic carbon content
SOC increased over time in all the three systems. In 2017, the SOC in the CC, LC and OC systems were 17.10 g kg-1, 24.81 g kg-1 and 29.80 g kg-1, respectively (Figure 3). After 15 years, the SOC in the OC system was 1.7 times of that in the CC system and 1.2 times of that in the LC system.
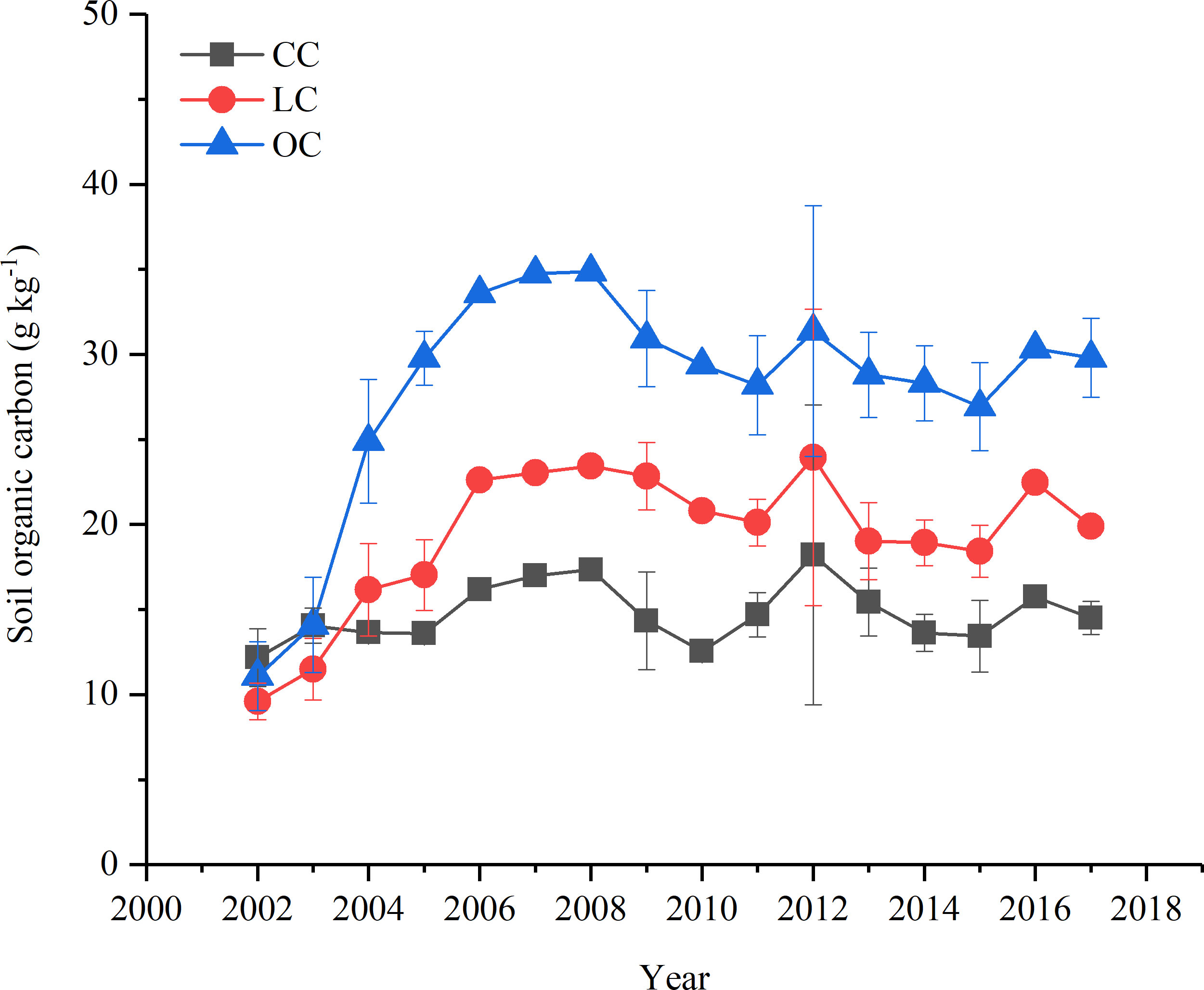
Figure 3 Soil organic carbon content with the three different cultivation methods from 2002 to 2017.
3.1.3 Soil available nutrients content
The available N, P, K in soil increases in all three systems. Compared with CC, AN increased by 26.88%-135.08%, AP increased by 22.25% -210.12%, AK increased by 4.37% -110.87% in OC treatment, whereas in LC AN, AP and AK increased by 10.91%-32.46%, 5.16%-114.89% and 5.56%-62.30%, respectively (Figure 4).
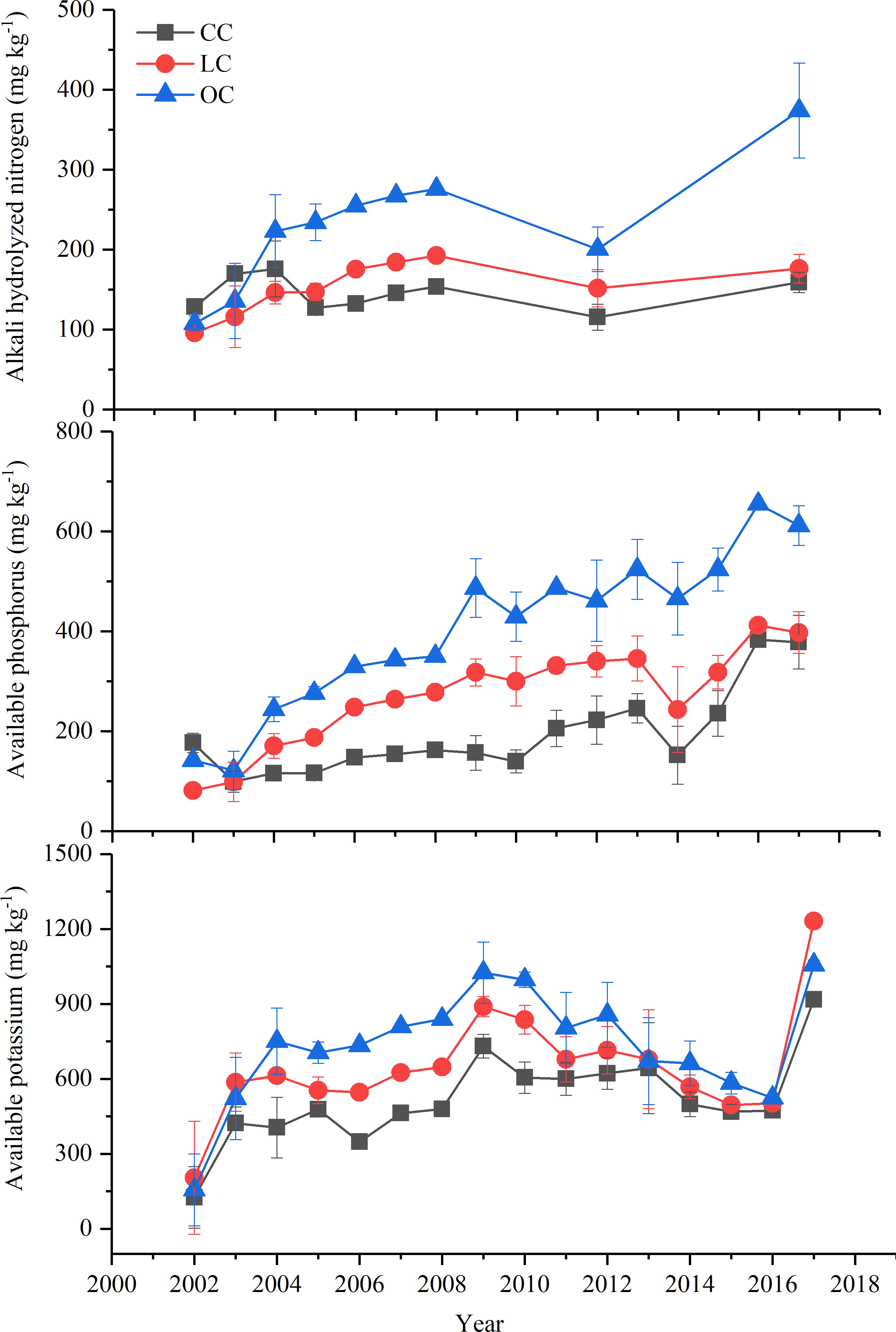
Figure 4 Soil available nutrient content with the three different cultivation methods from 2002 to 2017.
3.2 Negative effects of long-term organic cultivation on soil environmental quality
3.2.1 Heavy metal pollution and ecological risk assessment
After 15 years cultivation, the contents of Zn, Pb, Cr and As in the soil of CC treatment increased significantly. In LC and OC, all the heavy metals increased significantly. The contents of Zn, Cd and Cr in the soil were significantly different among the three treatments in 2017, with the highest concentrations in OC and the lowest in CC. Compared with the initial concentration, Zn content in the soil under the three treatments (OC, LC and CC) increased by 199.80%, 96.64%, and 54.72%, Cd increased by 312.50%, 175.00%, and 100.00%, and Cr increased by 253.74%, 178.46% and 147.70%, respectively (Figure 5). The Cu, Pb and As contents all increased after 15 years of cultivation in three treatments, but there was no significant difference (Figure 5).
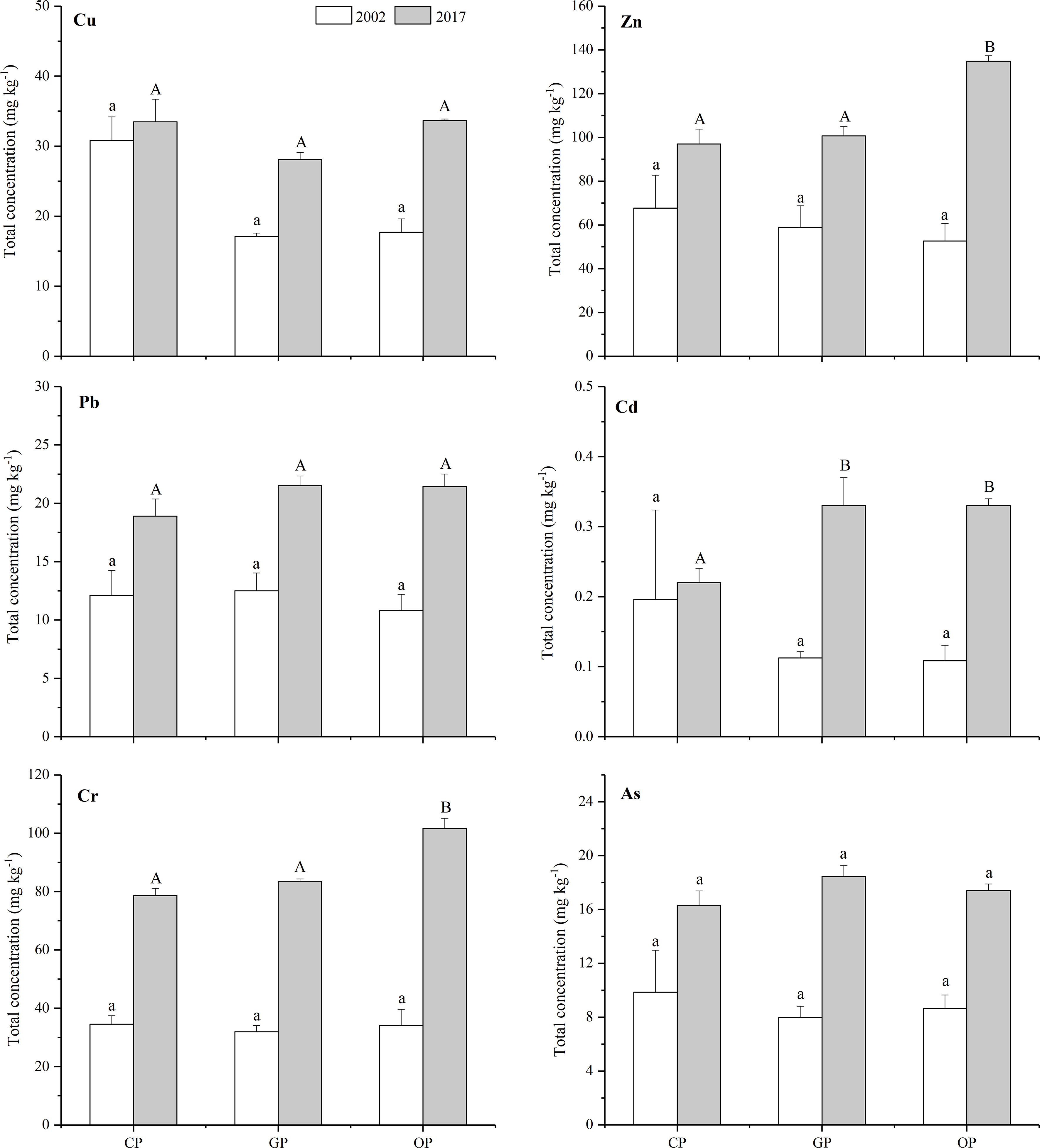
Figure 5 Total concentrations of soil heavy metal under different cultivation in 2002 and 2017. Lowercase letters indicate significant differences among treatments in 2002, capital letters indicate significant differences among treatments in 2017.
Different heavy metals have different accumulation indexes among treatments. Zn, Cd and Cr were strongly accumulated, Cu and Pb were moderately accumulated in the OC treatment, Cd and Cr were moderately accumulated in the LC and CC treatments, and other heavy metals were slightly accumulated (Table S7). The comprehensive accumulation index of soil heavy metal was the highest in OC treatment, which was 3.56, while the comprehensive accumulation index of the LC and CC cultivation were between 2 and 3 (Table S7). The potential ecological risk index (RI) reflects the ecological hazards of soil heavy metal pollution, which was the highest (45.34) in the OC treatment.
3.2.2 Soil antibiotic residues and ecological risk assessment
In this study, 9 soil samples were tested from the three systems, and TCs were detected in 7 soil samples, with a detection ratio of 77.78%. In all soil samples, the detection ratio of OTC was 77.78%, and the highest content reached 563.00 μg kg-1; the detection ratios of DC and TC were both 33.33%, and the highest content of DC and TC were 55.80 μg kg-1 and 20.59 μg kg-1, respectively (Table 1). CTC was not detected. The content of TCs in 5 soil samples exceeded the suggested ecological safety threshold of antibiotic residues in soi (100 μg kg-1) (21).
The content of TC was 15.12 μg kg-1 in OC, while TC was not detected in LC and CC. The content of DC was 34.90 μg kg-1 in OC, which is 691.38% higher than that in CC, and in LC it is not detected. The OTC content was 399.08 μg kg-1 in OC, which was 1536.24% and 44.24% higher than that in LC and CC, respectively. CTC were not detected in any of the treatments. The highest content of TCs (563.00 μg kg-1) was observed in OC treatment, which exceeds the threshold of ecological safety. The remaining three antibiotics in all the samples were<100 μg kg-1, which indicated lower potential ecological risk.
Risk quotient (RQ) evaluates the ecological risk of pollutants according to the ratio of the measured concentration to the predicted ineffective concentration. RQ ≤ 0.1 was low risk, 0.1<RQ<1 was medium risk, and RQ≥1 was high risk (22). The ecological risk of antibiotics residues in soil was assessed with RQ in the three treatments. Both OTC and DC in the soil reached high risk in OC and CC treatments, while TC and CTC were medium risk or low risk in the three treatments (Table 1).
3.2.3 Soil pesticide residues and ecological risk assessment
Commonly used organophosphorus pesticides (OPPs) in agriculture include dimethoate, chlorpyrifos, chlorfenvinphos, and profenofos in China. The results showed that dimethoate was detected with the highest content of 2.40 mg kg-1; while chlorpyrifos, chlorfenvinphos and profenofos were not detected in any soil samples (Table 2). The content of dimethoate in soil was 1.97 mg kg-1 in the OC treatment, which was 688.00% and 169.86% higher than that in LC and CC, respectively. The results showed that the residual amount of OPPs in organic cultivation was the highest.
A probabilistic risk assessment method was used to evaluate the risk of pesticide residues in soil. Since chlorpyrifos, chlorfenvinphos and profenofos were not detected, the probability risk assessment was only done for dimethoate. The total risk quotient of CC, LC and OC were 0.10, 0.04 and 0.21, respectively. The results showed that the ecological risk of OPPs residues in the soil under organic cultivation was higher than that in the other two treatments (Table 2).
3.3 Comprehensive evaluation
We found that organic cultivation had the highest soil fertility but the highest environmental costs.In organic cultivation the highest concentrations of heavy metals and antibiotics are detected which brought high risks to the soil ecosystem. Conventional cultivation had the lowest fertility and high antibiotic residues. Low-input cultivation had intermediate soil fertility and crop yield, high heavy metals, and lower antibiotic residues (Figure 6).
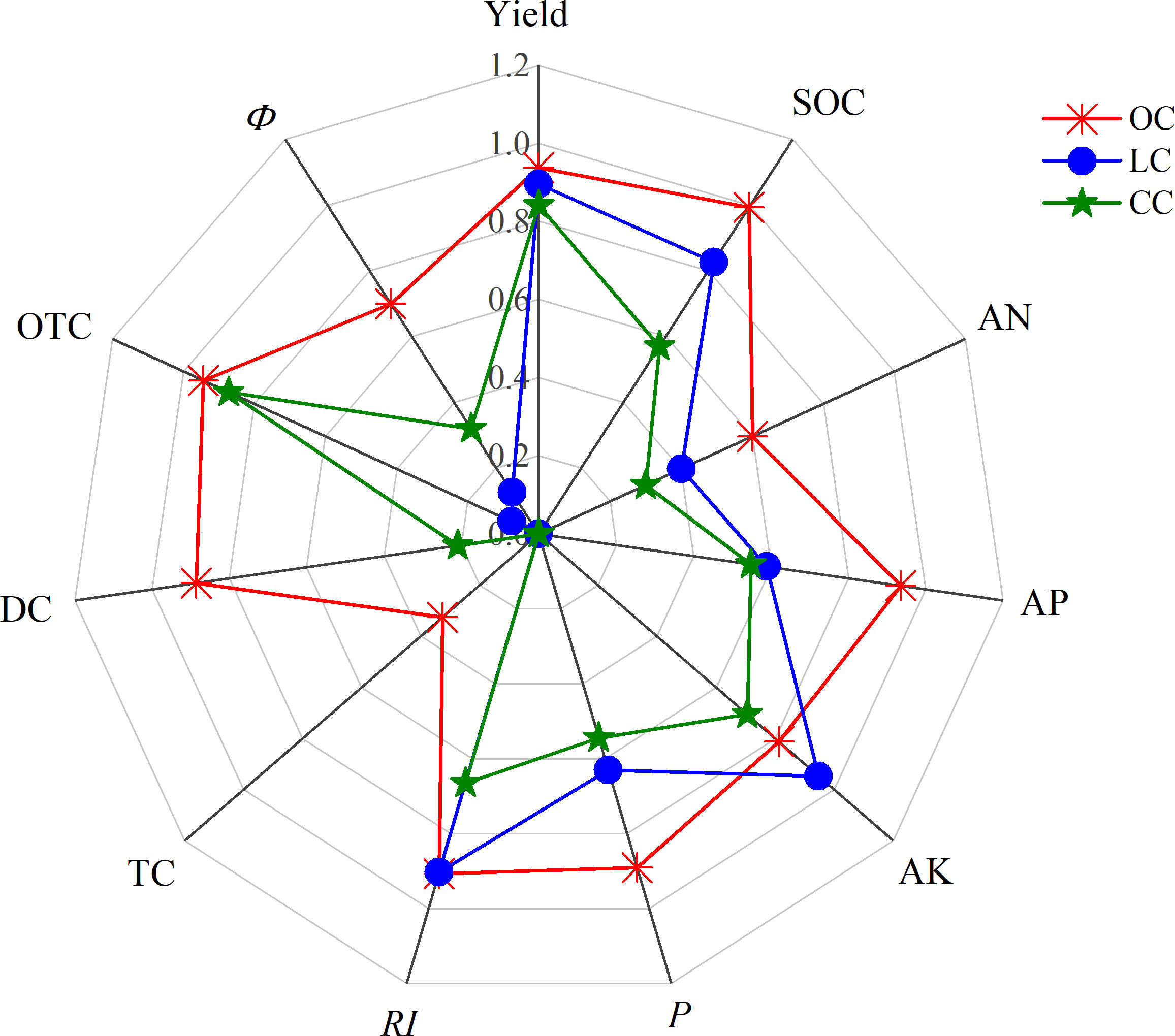
Figure 6 The comprehensive evaluation of soil fertility and soil environmental quality in different cultivation.
4 Discussion
4.1 Organic cultivation benefits soil fertility and crop yield
The impact of organic cultivation on crop yields is widely argued. Several of meta-analysis has concluded that crop yield under organic management were on average 19%~25% lower than that under traditional management (23), and commercial crop yields in the United States showed that the average yield gap between organic and conventional management was 20% (24). The environmental conditions are well-controlled in the greenhouse; therefore the crop production is very different from open field cultivation. For instance, higher temperatures in the greenhouse have significantly increased the total biomass of crop, leading to much higher nutrient demands from soils. Additionally, water management, air humidity and light are better controlled in greenhouses than in open air, providing a more suitable environment for crop growth. Organic vegetable production in greenhouse is characterized by high nutrient demands within short growing periods. Hence, the organic fertilizer in organic cultivation appears to be more easily converted into soil organic matter (SOM) and available nutrients in greenhouses. Due to the high cost of legume crop rotation as the main source of external N, it is rarely used in greenhouses. Therefore, farmers of those organic cultivation in greenhouse prefer to applying large amounts of animal manure and compost to increase vegetable yield. Our experiments showed that the vegetable yield of organic management is higher than that in low-input cultivation and conventional cultivation in greenhouses. The large inputs of organic fertilizer under organic cultivation in greenhouses not only increased soil organic matter content, available nutrient content, but also increased vegetable yield and yield stability.
One of the major benefits of organic cultivation to soil is to increase SOC content, thus improving soil structure and soil fertility. The content of SOM depends on the balance between the input of organic material input and the output of organic material by decomposition. Organic carbon content in soil increased with increasing input of organic fertilizer in organic system (7). Our result confirmed this conclusion. SOC content in organic cultivation was 1.2 and 1.7 times higher than that in the low-input and conventional system, respectively (Figure 3). However, high temperature and humidity will intensify the decomposition of SOM by affecting microbial activity and enzyme activity, resulting in low SOM content in surface soil in greenhouses (25). This may be related to the amount of organic fertilizer applied in the greenhouse. When the input of organic materials is lower than the output of organic materials, it will reduce the content of SOM in greenhouses. The large amount of organic fertilizer input in organic system not only provided a large amount of organic matter to the soil, but also promoted the mineralization of organic matter, thereby increasing soil fertility and vegetable yield.
4.2 The impacts of organic cultivation on soil environmental quality
The accumulations soil heavy metals, antibiotics and pesticide residues are considered as the important soil environmental qualities here and thoroughly discussed in the following context.
4.2.1 Soil heavy metal accumulations
The sources of heavy metal accumulation in soils could be from fertilizer input, irrigation water and atmospheric depositions. Since all of those greenhouses were plastic shed, the atmospheric deposition could be neglected. The water source for irrigation was from ground water, which had very limited contents of heavy metals due to their high stability in the soil (26). Therefore, the major source of heavy metals was the fertilizer inputs. Huang & Jin (27) reported that the application of chemical fertilizers and organic manures lead to high contents of Cd, Cu and Zn in the surface soil in the North China Plain. The average concentration of Pb, Cu and Zn were higher in greenhouse vegetable soils than those in open field soils in eastern China (28). Our results showed that all heavy metals accumulated in organic, low-input and conventional cultivation in greenhouse after 15 years. The accumulation of heavy metals in organic cultivation is heavy and moderate accumulation, while in low-input and conventional cultivation is moderate and slight, indicating that there is a positive correlation between the amount of organic fertilizer and the accumulated amounts of heavy metals.
In organic and low-input cultivation, the inputs of fertilizers were manure and its derived compost. The content of Cu、Zn、Pb、Cd、Cr and As were 31.49 mg kg-1, 209.59 mg kg-1, 8.13 mg kg-1, 0.15 mg kg-1, 16.21 mg kg-1 and 5.10 mg kg-1 in organic manure, respectively. Although the concentrations of these heavy metals in manures are within standard range 《HJ 333-2006 Environmental quality evaluation standard for farmland of greenhouse vegetables production》(Table S8), their concentrations in the soil continue to increase due to long-term repeated application. The comprehensive accumulation index (P) and potential ecological risk index (RI) of soil heavy metals were the highest in organic cultivation, indicating that heavy metal pollution is most serious. In the livestock production industry, a large amount of metal is used in animal feeding operations worldwide to treat animal diseases and promote animal growth (29), which may end up in the manure.
The relatively high concentrations of heavy metals in animal manures were mainly caused by a large number of feeding additives, because of their similar properties to antibiotics and antibacterial to promote animal growth (30). Zhang et al. (31) found that the contents of Cu, Zn, Cr, and As in livestock and poultry manures from typical farms in 7 provinces or municipalities in China were in the range of 1017~1591, 7113~8710, 0~688 and 0.01~65.4 mg kg-1, respectively. Ondoua (32) reported that the content of Cu and Zn in digestates from co-digestion of pig and cow manure was high. Due to the persistence of heavy metals, they accumulate in soils and cannot be degraded. Ecotoxic response of heavy metals is an important index for the evaluation of heavy metal pollution, which is determined by speciation and influenced by soil physical and chemical properties (33, 34). In addition, application of organic waste and pig manure could increase the content of bioavailable heavy metals in the soil (35), which can enter human body through food and affect human health.
4.2.2 Soil antibiotics accumulation
The accumulation of antibiotics is becoming an environmental problem and threaten human health in organic vegetables production (36). However, their residue levels and distribution in greenhouse organic vegetables are still unclear. We found that the content of TC, DC and CTC were<100 μg kg-1 in all three treatments, while the content of OTC was >100 μg kg-1 in organic and conventional cultivation, and reached the highest (563 μg kg-1) in organic cultivation. The content of TCs and the ecological risk of soil TCs were all highest in the organic cultivation systems. This is mainly due to the extensive use of organic fertilizers. Antibiotics are usually derived from organic manure and compost. Tetracyclines are the most frequently reported antibiotic residues and have the highest concentrations in manure. Antibiotic residues were detected in most of the manure, the concentration of oxytetracycline (416.8 mg kg-1) was the highest in chicken manure, and the concentration of ofloxacin (1334.5 mg kg-1) and ciprofloxacin (1717.4 mg kg-1) was the highest in the rice husk compost (37).
In the livestock industry, a large number of antibiotics has been applied to treat animal diseases and promote animal growth every year (29). Collignon & Voss (38) reported that animal husbandry used approximately 97,000 tons of antibiotics in China. The content of chlortetracycline, oxytetracycline and tetracycline in pig manure could be as high as 46 mg kg-1, 29 mg kg-1 and 23 mg kg-1, respectively (39). Tetracyclines are frequently found in soil as well. The application of organic fertilizers with high residual of antibiotics could cause soil ecosystem risks in greenhouses.
Controlling the source and composting process of organic fertilizer is an effective way to reduce antibiotic residues. Ezzariai et al. (40) reported that the process of composting could remove 17-100% of antibiotic residues from sludge and manure. Antibiotic residues were detected in the organic fertilizer even after high-temperature composting process. The content of tetracycline、doxycycline、oxytetracycline and chlortertracycline were 0.80 mg kg-1, 3.34 mg kg-1, 0.05 mg kg-1 and 0.05 mg kg-1 in organic fertilizer, respectively (Table S2). The concentration of oxytetracycline in organic fertilizers was significantly lower than that in soil, which was mainly because the samples were stored for too long leading to degradation of antibiotics. Repeated application of organic fertilizers with antibiotic residues will affect the soil microbial community structure. Kahle & Stamm (41) reported that long-term application of livestock manure could induce 70% increase of antibiotic resistant strains, which has a negative influence on soil biological environment and human health.
4.2.3 Soil pesticide accumulation
The substrates for the organic amendments used in organic cultivation were mostly derived from animal manure and crop residues produced in conventional systems. Therefore, accumulation of pesticide residues in soils of organic cultivation are possible. In the present study, the residual amount of OPPs and the ecological risk of OPPs in organic cultivation soils were the highest. Again, it was mainly from the large amount of organic fertilizer application in organic cultivation. The organic amendments were animal manure, and the pesticide residues may come from the silage consumed by the animals, and the manure after animal digestion of those silage would contain undecomposed pesticides. Zhao et al. (42) found that the organochlorine pesticide residues in fodder and manure were 25.35~65.62 ng g-1 and 33.46~90.89 ng g-1, respectively, indicating that feeding is an important pathway for pesticides to enter manure.
Composting is an important process to degrade organophosphorus pesticide residues (43). Although anaerobic digestates of organic fertilizer can degrade pesticides, and the content of pesticides is within the standard, long-term application can still bring environmental risks to the soil. In addition, researchers argue that some organic pesticides are more hazardous than synthetic pesticides (44). Organic pesticides typically have low toxicity and low persistence in the environment. However, some organic pesticides, such as sulfur and rotenone, have a larger environmental impact because of the higher doses and higher frequency of application required, despite of their lower toxicity (45). It can be seen that the types and the amounts of pesticides in organic fertilizer used for organic agriculture should be strictly controlled. Unfortunately, there are no strong regulations for controlling pesticide residues in organic fertilizers yet.
4. 3 Implications and suggestions
Organic cultivation was considered as a double-edged sword, which can not only improve soil quality (6), but may also cause health risks to soil. The persistent organic pesticides were difficult to be completely decomposed during composting process. Therefore, many studies found that organic cultivation might carry significant amount of pollutants to the soil due to residue accumulations from the long-term application of organic manure, sludge and compost (11, 12, 37). Thus, the impact of organic cultivation on soil depends on the quantity and quality of the applied organic fertilizer. In order to improve the quality of organic fertilizer, it is necessary to develop strict quality management of composting material and environment-friendly new organic composting technology. We still face many challenges in developing high-quality organic fertilizer. In the era of rapid global development, it requires active adjustment of animal husbandry, agricultural production and human lifestyle. At present, organic partial substitution or low input cultivation could be a promising approach for the development of sustainable agriculture.
5 Conclusion
Among the three agricultural systems, organic cultivation significantly increased soil fertility and vegetable yields. Compared with conventional cultivation, organic cultivation increased SOC, alkali-hydrolyzed nitrogen, available phosphorus and potassium by 72.25%-134.17%, 26.88%-135.08%, 22.25% -210.12% and 4.37% -110.87%. Coupled with the ecological risk assessments, we highlight the double-edged sword effect that organic fertilizer has on soil qualities, causing both improvement of soil fertility and accumulation of heavy metals, antibiotics and pesticide residues in soils under organic farming practices. Particularly, after 15 years of organic cultivation, Zn, Cd and Cr concentrations increased by 199.80%, 312.50% and 253.74%, respectively. In addition, organic cultivation leads to the highest content of TCs (563 μg kg-1) and dimethoate (2.40 mg kg-1). Considering both soil fertility improvement and harmful residue accumulation mechanisms will be beneficial for optimizing strategies of organic farming management. Quantitative controls on the amount and composition of the organic fertilizers need to be implemented with caution before large scale of organic cultivation can be applied.
Data availability statement
The original contributions presented in the study are included in the article/Supplementary Material. Further inquiries can be directed to the corresponding author.
Author contributions
LT: Data curation, Investigation, Writing – original draft. JL: Sample collection, Investigation. LZ: Investigation, Formal analysis. SZ: Review. HZ: Review. YL: Review, Funding acquisition. KZ: Review. All authors contributed to the article and approved the submitted version.
Funding
This research is funded by the National Key Research and Development Program of China (Grant No. 2021YFC3201203).
Conflict of interest
The authors declare that the research was conducted in the absence of any commercial or financial relationships that could be construed as a potential conflict of interest.
Publisher’s note
All claims expressed in this article are solely those of the authors and do not necessarily represent those of their affiliated organizations, or those of the publisher, the editors and the reviewers. Any product that may be evaluated in this article, or claim that may be made by its manufacturer, is not guaranteed or endorsed by the publisher.
Supplementary material
The Supplementary Material for this article can be found online at: https://www.frontiersin.org/articles/10.3389/fsoil.2022.1096735/full#supplementary-material
References
1. Smith P, Haberl H, Popp A, Erb KH, Lauk C, Harper R, et al. How much land based greenhouse gas mitigation can be achieved without compromising food security and environmental goals? Glob Chang Biol (2013) 19(8):2285–302. doi: 10.1111/gcb.12160
2. Wang M, Xu S, Yang J, Xu L, Yu Q, Xie X, et al. The effect of organic and conventional management practices on soil macropore structure in greenhouse vegetable production. Eur J Soil Sci (2021) 72(5):2133–49. doi: 10.1111/ejss.13106
3. Goulding K. Nitrate leaching from arable and horticultural land. Soil Use Manag (2006) 16(1):145–51. doi: 10.1111/j.1475-2743.2000.tb00218.x
4. Zhou JY, Gu BJ, Schlesinger WH, Ju XT. Significant accumulation of nitrate in Chinese semi-humid croplands. Sci Rep (2016) 6:25088. doi: 10.1038/srep25088
5. Aulakh CS, Sharma S, Thakur M, Kaur P. A review of the influences of organic farming on soil quality, crop productivity and produce quality. J Plant Nutr (2022) 45(12):1884–905. doi: 10.1080/01904167.2022.2027976
6. Gomiero T, Pimentel D, Paoletti MG. Environmental impact of different agricultural management practices: Conventional vs. organic agriculture. Crit Rev Plant Sci (2011) 30:95–124. doi: 10.1080/07352689.2011.554355
7. Gattinger A, Müller A, Haeni M, Skinner C, Fliessbach A, Buchmann N, et al. Enhanced top soil carbon stocks under organic farming. P Natl (2012) 109(44):18226–31. doi: 10.1073/pnas.1209429109
8. Gömöryová E, Střelcová K, Fleischer P, Gomory D. Soil microbial characteristics at the monitoring plots on windthrow areas of the tatra national park (Slovakia): Their assessment as environmental indicators. Environ Monit Assess (2010) 174:31–45. doi: 10.1007/s10661-010-1755-2
9. Liang L, Ridoutt BG, Lal R, Wang DP, Wu WL, Peng P, et al. Nitrogen footprint and nitrogen use efficiency of greenhouse tomato production in north China. J Clean. Prod (2018) 208(20):285–96. doi: 10.1016/j.jclepro.2018.10.149
10. Wu RY, Sun HW, Xue J, Yan D, Liu Y, Gui D, et al. Acceleration of soil salinity accumulation and soil degradation due to greenhouse cultivation: a survey of farmers practices in China. Environ Monit Assess (2020) 192(6):399. doi: 10.1007/s10661-020-08363-6
11. Hu WY, Chen Y, Huang B, Niedermann S. Health risk assessment of heavy metals in soils and vegetables from a typical greenhouse vegetable production system in China. Hum Ecol Risk Assess (2014) 20:1264–80. doi: 10.1080/10807039.2013.831267
12. Chen B, Ma XX, Liu GQ, Xu XM, Pan FS, Zhang J, et al. An endophytic bacterium acinetobacter calcoaceticus Sasm3-enhanced phytoremediation of nitrate–cadmium compound polluted soil by intercropping sedum alfredii with oilseed rape. Environ Sci pollut R (2015) 22:17625–35. doi: 10.1007/s11356-015-4933-5
13. Jiao WT, Chen WP, Chang AC, Page AL. Environmental risks of trace elements associated with long-term phosphate fertilizers applications: a review. Environ pollut (2012) 168:44–53. doi: 10.1016/j.envpol.2012.03.052
14. Nemerow NL. Stream, lake, estuary, and ocean pollution. New York: Van Nostrand Reinhold, New York (1985).
15. Hakanson L. An ecological risk index for aquatic pollution control.a sedimentological approach. Water Res (1980) 14(8):975–1001. doi: 10.1016/0043-1354(80)90143-8
16. Hernando MD, Mezcua M, Fernández-Alba AR, Barcelo D. Environmental risk assessment of pharmaceutical residues in wastewater effluents, surface waters and sediments. Talanta (2006) 69:334–42. doi: 10.1016/j.talanta.2005.09.037
17. Xiang L, Wu XL, Jiang YN, Yan QY, Li YW, Huang XP, et al. Occurrence and risk assessment of tetracycline antibiotics in soil from organic vegetable farms in a subtropical city, south China. Environ Sci pollut R (2016) 23:13984–95. doi: 10.1007/s11356-016-6493-8
18. Xue BM, Zhang RJ, Wang YH, Liu X, Li J, Zhang G. Antibiotic contamination in a typical developing city in south China: Occurrence and ecological risks in the yongjiang river impacted by tributary discharge and anthropogenic activities. Ecotoxicol. Environ Saf. (2013) 92:229–36. doi: 10.1016/j.ecoenv.2013.02.009
19. Alygizakis NA, Gago-Ferrero P, Borova VL, Pavlidou A, Hatzianestis I, Thomaidis NS. Occurrence and spatial distribution of 158 pharmaceuticals, drugs of abuse and related metabolites in offshore seawater. Sci Total Environ (2016) 541:1097–105. doi: 10.1016/j.scitotenv.2015.09.145
20. Albero B, Tadeo JL, Escario M, Miguel E, Perez R. Persistence and availability of veterinary antibiotics in soil and soil-manure systems. Sci Total Environ (2018) 643:1562–70. doi: 10.1016/j.scitotenv.2018.06.314
21. Karci A, Balcioglu IA. Investigation of the tetracycline, sulfonamide, and fluoroquinolone antimicrobial compounds in animal manure and agricultural soils in Turkey. Sci Total Environ (2009) 407:4652–64. doi: 10.1016/j.scitotenv.2009.04.047
22. Hanna N, Sun P, Sun Q, Li XW, Yang XW, Ji X, et al. Presence of antibiotic residues in various environmental compartments of Shandong province in eastern China: Its potential for resistance development and ecological and human risk. Environ Int (2018) 114:131–42. doi: 10.1016/j.envint.2018.02.003
23. Ponisio LC, M'Gonigle LK, Mace KC, Palomino J, de Valpine P, Kremen C. Diversification practices reduce organic to conventional yield gap. P Roy Soc B-Biol Sci (2015) 282:1799. doi: 10.1098/rspb.2014.1396
24. Kniss AR, Savage SD, Jabbour R. Commercial crop yields reveal strengths and weaknesses for organic agriculture in the united states. PloS One (2016) 11(8):e0161673. doi: 10.1371/journal.pone.0161673
25. Moinet GYK, Hunt JE, Kirschbaum MUF, Morcom CP, Midwood AJ, Millard P. The temperature sensitivity of soil organic matter decomposition is constrained by microbial access to substrates. Soil Biol Biochem (2018) 116:333–9. doi: 10.1016/j.soilbio.2017.10.031
26. Mmolawa KB, Likuku AS, Gaboutloeloe GK. Assessment of heavy metal pollution in soils along major roadside areas in Botswana afr. AJEST (2011) 5(3):186–96. doi: 10.5897/AJEST10.246
27. Huang SW, Jin JY. Status of heavy metals in agricultural soils as affected by different patterns of land use. Environ Monit Assess (2008) 139:317–27. doi: 10.1007/s10661-007-9838-4
28. Chen Y, Huang B, Hu W, Weindorf DC, Liu X, Niedermann S. Assessing the risks of trace elements in environmental materials under selected greenhouse vegetable production systems of China. Sci Total Environ (2014) 470:1140–50. doi: 10.1016/j.scitotenv.2013.10.095
29. Hamscher G, Pawelzick H, Höper H, Nau H. Different behavior of tetracyclines and sulfonamides in sandy soils after repeated fertilization with liquid manure. Environ Toxicol Chem (2005) 24(4):861–8. doi: 10.1897/04-182R.1
30. Xiuling J., Shen Q., Liu F., Xu G., Wang Y., Wu M.. (2012). Antibiotic resistance gene abundances associated with antibiotics and heavy metals in animal manures and agricultural soils adjacent to feedlots in Shanghai; China J Hazard Mater 235–236:178–185. doi: 10.1016/j.jhazmat.2012.07.040
31. Zhang SQ, Zhang FD, Liu XM, Wang YJ, Zou SW, He XS. Determination and analysis on main harmful composition in excrement of scale livestock and poultry feedlots. J Plant Nutr (2005) 11(6):822–9. doi: 10.3321/j.issn:1008-505X.2005.06.019
32. Ondoua R. Agricultural benefits and environmental risks of soil fertilization with anaerobic digestates: A review. Agron Sustain Dev (2013) 34:473–92. doi: 10.1007/s13593-013-0196-z
33. Zhang XK, Qin BQ, Deng JM, Wells M. Whole-cell bioreporters and risk assessment of environmental pollution: a proof-of-concept study using lead. Environ pollut (2017) 229:902–10. doi: 10.1016/j.envpol.2017.07.068
34. Zhang XK, Li BL, Deng JM, Qin BQ, Wells M, Tefsen. B. Regional-scale investigation of dissolved organic matter and lead binding in a large impacted lake with a focus on environmental risk assessment. Water Res (2020) 172:115478. doi: 10.1016/j.watres.2020.115478
35. Zhao BZ, Maeda M, Zhang JB, Zhu AN, Ozaki Y. Accumulation and chemical fractionation of heavy metals in andisols after a different, 6-year fertilization management. Environ Sci pollut R (2006) 13:90–7. doi: 10.1065/espr2005.06.268
36. Hu XG, Zhou QX, Luo Y. Occurrence and source analysis of typical veterinary antibiotics in manure, soil, vegetables and groundwater from organic vegetable bases, northern China. Environ pollut (2010) 158(9):2992–8. doi: 10.1016/j.envpol.2010.05.023
37. Zhang H, Luo Y, Wu L, Huang Y, Christie P. Residues and potential ecological risks of veterinary antibiotics in manures and composts associated with protected vegetable farming. Environ Sci pollut R (2015) 22:5908–18. doi: 10.1007/s11356-014-3731-9
38. Collignon P, Voss A. China, What antibiotics and what volumes are used in food production animals? Antimicrob Resist In (2015) 4:16. doi: 10.1186/s13756-015-0056-5
39. Martínez-Carballo E, González-Barreiro C, Scharf S, Gans O. Environmental monitoring study of selected veterinary antibiotics in animal manure and soils in Austria. Environ pollut (2007) 148(2):570–9. doi: 10.1016/j.envpol.2006.11.035
40. Ezzariai A, Hafidi M, Khadra A, Aemig Q, El Felsa L, Barret M, et al. Human and veterinary antibiotics during composting of sludge or manure: Global perspectives on persistence, degradation, and resistance genes. J Hazard. Mater (2018) 359:465–81. doi: 10.1016/j.jhazmat.2018.07.092
41. Kahle M, Stamm C. Sorption of the veterinary antimicrobial sulfathiazole to organic materials of different origin. Environ Sci Technol (2007) 41(1):132–8. doi: 10.1021/es061198b
42. Zhao L, Dong YH, Wang H. Residues of organochlorine pesticides and polycyclic aromatic hydrocarbons in farm-raised livestock feeds and manures in jiangsu, China. Sci Total Environ (2013) 450:348–55. doi: 10.1016/j.scitotenv.2012.09.017
43. Wei Y.Q., Zhao Y., Lu Q., Cao Z.Y., Wei Z.M.. Organophosphorus-degrading bacterial community during composting from different sources and their roles in phosphorus transformation Bioresour. Technol (2018) 264:277–284. doi: 10.1016/j.biortech.2018.05.088
Keywords: antibiotics, greenhouses, heavy metals, pesticide residues, soil organic carbon
Citation: Tong L, Li J, Zhu L, Zhang S, Zhou H, Lv Y and Zhu K (2022) Effects of organic cultivation on soil fertility and soil environment quality in greenhouses. Front. Soil Sci. 2:1096735. doi: 10.3389/fsoil.2022.1096735
Received: 12 November 2022; Accepted: 13 December 2022;
Published: 23 December 2022.
Edited by:
Xiaokai Zhang, Jiangnan University, ChinaReviewed by:
Qianqian Zhang, Zhejiang Agriculture and Forestry University, ChinaAbhijit Sarkar, Indian Institute of Soil Science (ICAR), India
Copyright © 2022 Tong, Li, Zhu, Zhang, Zhou, Lv and Zhu. This is an open-access article distributed under the terms of the Creative Commons Attribution License (CC BY). The use, distribution or reproduction in other forums is permitted, provided the original author(s) and the copyright owner(s) are credited and that the original publication in this journal is cited, in accordance with accepted academic practice. No use, distribution or reproduction is permitted which does not comply with these terms.
*Correspondence: Yizhong Lv, bHl6QGNhdS5lZHUuY24=; Kun Zhu, a3Vuemh1QGNhdS5lZHUuY24=
†These authors have contributed equally to this work