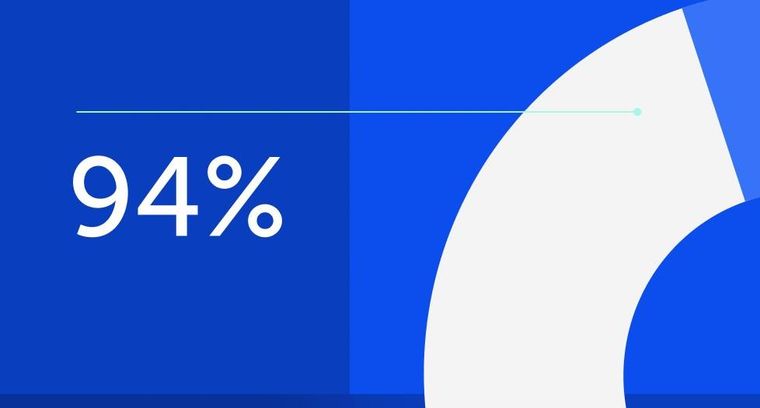
94% of researchers rate our articles as excellent or good
Learn more about the work of our research integrity team to safeguard the quality of each article we publish.
Find out more
REVIEW article
Front. Soil Sci., 13 September 2021
Sec. Soils and Human Health
Volume 1 - 2021 | https://doi.org/10.3389/fsoil.2021.732971
Soil is an important source of resources required for human health and well-being. Soil is also a major environmental reservoir of pathogenic organisms. This may include viruses like the severe acute respiratory syndrome coronavirus-2 (SARS-CoV-2), which through 2020 and 2021 created dramatic catastrophes worldwide as the causative agent of the coronavirus disease of 2019 (COVID-19). So, soil has both positive and negative impacts on human health. One of the major positive impacts is the transfer of nutrients from soil to plants, and from there to humans through their diet. Biofortification is able to enhance the levels of nutrients essential to human health in the crops we consume and represents a sustainable solution to address malnutrition, which in turn may strengthen the human immune system against COVID-19. This nutrient transfer works better when we have healthy soils. Therefore, soils and biofortification have important roles to play in combatting the COVID-19 pandemic. However, several questions still remain, such as what are the expected environmental impacts of COVID-19 on soil? Can SARS-CoV-2 be transmitted through soil, and under what conditions? Which soil processes and properties influence SARS-CoV-2 survival rates and times, as well as transmission? What are the specific links between soil health and COVID-19? What are the projected soil management scenarios in response to COVID-19? Questions such as these deserve more attention as the world seeks to recover from its most recent pandemic.
As a major source of food, feed, fiber, fuel, and biodiversity, soil is considered an important resource not only for agriculture, but also for human health and the environment [(1, 2); Figure 1]. Soils contain the nutrients, or elements, that are essential for human and plant life functions. Thus, soil has a very strong link to human health [e.g., (3, 4)], agriculture-based economies (5, 6), food security (7, 8), and air and water quality (9). Furthermore, the health of soil is a pivotal global issue for producing healthy plants, which are crucial for human health (10). However, the health of soil is under great threat across the globe. These threats may include salinization (11, 12), climate change (12), erosion (13), compaction (14), nutrient depletion (15), contamination with toxic levels of pollutants such as heavy metals or pesticides (16), human assisted migration of soil-borne pests (4), and overgrazing (17).
Figure 1. There are direct and indirect as well as positive and negative relationships between soil and human health. For example, soil is the main source of several antibiotics derived from soil organisms, while at the same time soil hosts many pathogens.
The relationship between soils, human health, and the coronavirus disease of 2019 (COVID-19) pandemic is very complicated and interesting (18, 19). Soil is a major host and transmission pathway for several pathogenic microbes (18, 20) because it is one of the most diverse and dense microbial habitats on our planet (21, 22). Spread of the COVID-19 pandemic may be controlled by several factors like atmospheric temperature and average daylight hours (23), wind speed (24), atmospheric stability (25), flooding (26), relative and absolute humidity (27), contaminated wastes (28), and different environmental media (29). Soils are currently considered a black box by many in microbiology. Soil microorganisms can be divided into categories such as those beneficial to plants or humans and pathogenic to plants or humans (19, 30). Thus, healthy ecosystems and healthy humans depend on healthy soils, as confirmed by the one health concept (19, 31).
Soil nutrient bioavailability problems for cultivated plants may result in malnutrition for people who rely on those plants as a source of food. Supplying necessary nutrients for cultivated plants through fertilization or other approaches is called biofortification, and is a vital process for human health (32). Biofortification has been used with many important food crops like wheat (33), rice (34), maize (35), cassava (36), sweet potato (37), pear (38), strawberry (39), and pulse crops (40). Nutrients that have been used for biofortification include boron (41), copper (42), iron (36), iodine (43), calcium (38), selenium (44, 45), and zinc (46). In addition to nutrients, edible crops can be biofortified with vitamins such as vitamin B1 (thiamine), B2 (riboflavin), B3 (e.g., niacin), B5 (pantothenate), B6 (e.g., pyridoxine), B7 (biotin), B9 (e.g., folates and their derivatives), B12 (cobalamin), vitamin C (ascorbate), vitamin E (tocopherol), and carotenoids (32, 47–49).
Therefore, this review seeks to highlight biofortification and human health opportunities and challenges related to soil under the COVID-19 pandemic. Major issues addressed include: (a) the current status of our knowledge about severe acute respiratory syndrome coronavirus-2 (SARS-CoV-2) in the environment, particularly soil, (b) the expected environmental impacts of COVID-19 on soil health, and (c) ways that soils and biofortification may help alleviate the COVID-19 pandemic.
One might not immediately think “soil” when thinking about the spread of viral diseases. Viruses are biologically active microscopic parasites that require a host to replicate. Although aerosols and droplets are the most common methods for viruses to spread, it is widely recognized that transmission can also occur via the fecal-oral route, which may include soils. As a result, to prevent the spread of infection, all environmental compartments (i.e., soil, water, and air) the virus can affect must be monitored (50). Recent studies have established that the transmission of SARS-CoV-2, the virus that causes COVID-19, may occur through air, water, soil, cold-chain, biota, and surface contact (51). As compared to other environmental compartments, the interactions between soil and viruses have not received much attention (52). However, many research papers that addressed links between the COVID-19 outbreak and the environment were published over the last year. This included links between the outbreak and soil that addressed several issues, such as:
1. How to handle soil samples to measure or quantify SARS-CoV-2. Some studies have also investigated the detection and quantification of SARS-CoV-2 in wastewater and sewage sludge (53) as well as soils (54).
2. Why do we need to study SARS-CoV-2 in relation to soils? These studies emphasize how soils are an important component of the environment that receive this and other viruses when wastewater is used to irrigate cultivated plants, infected manure is applied as a nutrient source, or when soils are used for on-site sewage disposal, which may transfer the virus through the food chain (53–56).
3. There is currently no established procedure for detecting and quantifying SARS-CoV-2 in soil or soil-related liquid samples, although there have been attempts to develop procedures to detect this and other pathogenic viruses in liquid-soil samples (53, 54, 54).
4. What role can soil play in mitigating the effects of COVID-19 over the short- and long-term? The existence, survival, and behavior of SARS-CoV-2 in the soil is still not well-known (21). It is known that the survival of viruses (like SARS-CoV-2) mainly depended on soil properties (e.g., soil temperature, pH, moisture content, exchangeable Al) and virus characteristics (e.g., presence or absence of a viral envelop, adsorption rate of the virus on soil), and some projections for SARS-CoV-2 survival in soil have been made based on similar viruses (18, 21).
5. The positively charged form of coronavirus can attach such viruses to the negative-charge sites on soil clays, which may at least slow down their spread in soil (57). Are soil minerals able to react with and adsorb this virus, slowing or neutralizing its spread?
6. Adsorption by soil can have a significant impact on the ability of viruses to infect host organisms. Negative and positive charges on clay particles are able to absorb more than 90% of the viruses in clay-rich soils. Based on the survival rate of similar viruses, it has been concluded that the survival of corona virus in soil may be just a few days. However, no studies to date have focused specifically on the survival of SARS-CoV-2 in soil media (18, 29, 56).
7. The actual rates of transmissivity and survival of SARS-CoV-2 in soil and their relationships with soil properties and other environmental conditions are still open questions that need to be evaluated (18, 21). As vaccines are increasingly administered a critical point has been reached in the COVID-19 outbreak. How can we build a broader awareness about the importance of soil to human health and how we can manage soil to enhance the health of people, animals, and plants in the post-COVID-19 world (58)?
Dietary malnutrition is thought to be a key cause of health deterioration in developing countries. This includes deterioration of the immune system. Around 75% of the world's population is deficient in micronutrients, namely Zn, Cu, I, and Fe, resulting in several health issues. As a result, providing these micronutrients in a balanced human diet has gained a lot of attention across the world. These micronutrients are often supplied to humans through dietary fortification. While this provides a fast way to avoid illnesses caused by nutritional shortage, it is an artificial method that requires special attention and is not socially acceptable to broad parts of the community. Instead, enrichment or biofortification of food crops throughout the crop growth and development stages has the potential to naturally supply essential nutrients to everyone, particularly people without access to expensive commercially available fortified meals or supplements (59).
Biofortification is a sustainable process that can increase the vitamin or mineral nutrient contents in cultivated plants or animals via agronomic practices, plant breeding or transgenic techniques (60). The production of biofortified staple crops mainly depends on the content of available soil nutrients for plant and then human health and nutrition. Soil properties including chemical (pH, salinity, CEC, SOM, Eh, nutrient interactions in soil, etc.), physical (soil texture, structure, moisture content, aeration, etc.) and biological (soil microbial populations and activities, macro-organism populations and activities, enzyme activities, etc.) are the primary factors that control the bioavailability of nutrients for cultivated plants. Promising results have been obtained using biofortification of different nutrients or vitamins for many crops under different climatic conditions in several countries (Table 1). Therefore, the right biofortification process should start with an analysis of the soil and its properties, then move to selection of the right edible plant or crop, which should fit the soil and climatic conditions. For example, soil or foliar agronomic Se-biofortification (up to 80 g ha−1) of rice is a promising strategy that could be adopted in tropical soils in Brazil to produce rice grains rich in Se for human consumption (34). In Se or Zn deficient regions, the biofortification of soybean seeds by spiking the soil with deficient elements during the flowering phase may improve the human nutrition value of this crop. Experiments have achieved 25-fold Se and 190-fold Zn increases in soybean seeds (61). Under the acidic (pH 4.6) soil conditions in Brazil, agronomic Zn-biofortification (25 mg Zn kg−1 soil) on 29 genotypes of cowpea (Vigna unguiculata L.) was confirmed by increased Zn and soluble storage protein and decreased phytic acid in cowpea grains (62).
Table 1. A survey of some biofortification studies published in 2020–2021, including the place of study, targeted nutrient and crop, and a summary of the important findings of these studies.
Soil and foliar fertilization of iodine (up to 7.5 kg I ha−1) was investigated under field conditions (pH 5.5) in Germany to produce biofortified strawberry with higher iodine content in fruits. Higher iodine content was achieved using foliar application either as a single treatment shortly before harvest or when repeatedly sprayed during the flowering period (39). Different Zn-biofortification strategies, including application method and rates (0.3–10 and 1.28 kg ha−1, for soil and foliar application res.), were studied under Mediterranean climatic and field conditions (pH 8.1 and CaCO3 410 g kg−1) in Spain to produce wheat (70). They found that foliar applications at and after the early booting stage was a promising approach for producing both Zn-biofortified durum and bread wheat. Different programs also have been applied to overcome malnutrition problems by producing biofortified food crops such as pulses and legumes to enhance human nutrition (40, 71). Therefore, some of the great challenges facing the planet include understanding the properties of soil and cultivated plants (72). Agronomic biofortification success stories have been reported using Se-fertilization in Finland, Zn-fertilization in Turkey, and iodine-fertilization in China, among many others. Biofortification, in turn, can increase human resistance to diseases such as COVID-19, thus helping combat the current epidemic (73).
Soil health was described by Li et al. (74) as “the continued capacity of soil to function as a vital living ecosystem that sustains plants, animals, and humans,” which is in-line with the definition offered by USDA (75). Creating or maintaining healthy soil is a pivot approach to achieve both the sustainability of ecosystems and production of healthy food (76). Several studies have reported on the relationship between soil health and human health [e.g., (3, 52, 58, 74, 77)]. Soils are the main source of food production for humanity, and their health is essential to ensure a biodiverse and healthy environment, among other functions. The role of soils in food production during the COVID-19 pandemic was maximized because the disease threatened food availability in many places all over the world due to lack of workforce, the disruption of food chains, closed borders, and national lockdowns (78). There are several important ways that soil can strengthen local food production systems, enhance food resilience, and create a circular economy based on soil restoration. This restoration may be achieved through C-sequestration, cycling of nutrients on-farm, minimizing pollution of the environment, and minimizing contamination of foods (21). The sustainable management of soil resources is essential to recover from the COVID-19 pandemic because such management strengthens food supply chains and improves environmental quality while addressing interconnected issues such as the COVID-19 pandemic, global warming, hunger and malnutrition, water scarcity and renewability, and dwindling biodiversity (21).
Improving human health may include providing people with enough safe nutrients to avoid malnutrition and allow the immune system to fight against multiple diseases such as COVID-19 (73, 79). The COVID-19 pandemic has a strong relationship with other outbreaks such as severe acute respiratory syndrome (SARS) and Middle East respiratory syndrome (MERS). These are also respiratory diseases with similar infection stages (i.e., cough and fever) caused by coronaviruses that resulted in significant mortality among people who did not have a strong immune system (80). The immune system is supported by adequate and safe nutrition, whereas malnutrition or contaminated foods (e.g., through heavy metals or organic chemicals) may make individuals more vulnerable to such infections (81, 82). This immunity could be strengthened through factors like physical exercises (83), consumption of functional food ingredients like probiotics, flavonoids, carotenoids, curcumin, and omega-3 fatty acids supplements (84) and food rich in vitamins such as vitamins A, C, D, and E (80). Appropriate intake of vitamins and bioactive lipids could increase human resistance to viruses like SARS-CoV-2 (85, 86). As previously discussed, these can be supplied through the soil-crop system using biofortification.
Industrial agriculture is the dominant mode of food production used to create adequate amounts of safe food products in the modern world (87). However, industrial agriculture has several potentially negative aspects. These include loss of biodiversity, soil degradation, erosion, and the intensive use of herbicides, pesticides, and synthetic chemical fertilizers that may have deleterious effects on soil health, all of which can negatively impact soil and food security and human health (87, 88). In some instances, industrial agriculture has also introduced pollutants such as heavy metals to the soil environment (88). With the COVID-19 pandemic sweeping the world, food insecurity increased for millions of people (89) in several countries (90–95) and in large urban school districts (96). Understanding how COVID-19 impacts food insecurity, and the role that soils can play in minimizing this, is critical.
It has been reported that all forms of malnutrition might increase drastically due to the COVID-19 pandemic, which could increase the possibility of a malnutrition epidemic (97). Biofortified crops can provide needed nutrients that can combat malnutrition and improve resistance to respiratory disorders (36).
The current global pandemic has caused challenges such as indirect effects on soil quality (98), including negative effects on nutrient balances in soil (22). Food production is a major challenge with a pandemic sweeping the world, and food insecurity increased around the world. For example, it was recently estimated that COVID-19 is significantly aggravating already existing food insecurity in the U.S., with more than 54 million people being food insecure after COVID-19 (87).
Food insecurity is strongly related to soil health and nutrient budgets in the soil. The instant negative impact of the COVID-19 pandemic on food security and food supply systems, particularly in developing countries, has been widely reported [e.g., (21, 89)]. This impact included several obstacles that restricted the movement of goods and people between and within countries, which prevented access to markets, particularly in the agricultural sector (99). The future may include decreases in global food demand, a large loss in markets and employment, and growing concerns about international cooperation due to COVID-19 (100). Therefore, all countries need strategies to protect food and nutrition security, particularly for their poor, by focusing on the prioritization of diversification for production and markets (89). The mitigation strategies for COVID-19 as a global risk should also be linked with climate change, because both climate change and COVID-19 have rapidly expanded to all areas of the world (101) and they create some similar problems.
Many open questions need to be answered to provide sustainable biofortification under COVID-19. These include: (1) how can the world overcome the expected crises in global food security, trade, the economy, sustainable development, food waste management, agricultural production, and climate change? (2) How can countries fight malnutrition and food insecurity, particularly under the food production and distribution crisis during and post-COVID-19? (3) How can countries build resilient food systems amidst COVID-19? (4) To what extent we can depend on potential inhibitors (baricitinib, chloroquine, dexamethasone, favipiravir, fedratinib, remdesivir, vaccination, biofortification etc.) to manage COVID-19? (5) Can biofortification practices be effectively implemented in the global agricultural economy given the challenges presented by COVID-19?
There is no denying that COVID-19 is one of the most challenging pandemics to face humanity in the modern era. As common natural phenomena, a number of viruses are able to transfer between animals, plants, humans, and environmental compartments such as water or soil. It is important that we understand these dynamics, and the frequency of disease epidemics and pandemics has been increasing (19). The COVID-19 pandemic affected virtually all our life spheres, including the health, education, economic, political, and agricultural sectors. Several public health safety measures were implemented to restrict SARS-CoV-2 transmission, such as regular hand washing, social distancing, border closures, and restricted internal movements and lockdowns. However, the contributions that agriculture and soils might make to address the COVID-19 pandemic were rarely raised in the public discourse. Human immunity depends on nutritional status, which in turn is largely dependent on foods consumed, the nutrients they derived from soil, and a lack of pollutants in that soil. Biofortified crops that produce foods rich in functional food components can help improve general health, boost the immune system, and consequently might be useful in preventing COVID-19 transmission or improving outcomes for those who contract COVID-19. Due to the current disruptions in supply chains and demand on foods, the impacts of COVID-19 may provide the scientific community an opportunity to focus on agricultural innovations, including the development and delivery of biofortified crops and improving the transfer of nutrients from soil to crop. This represents one way that the agricultural and soil communities can assist with the current COVID-19 crisis and prepare food systems for future crises. However, several questions remain, such as what are the expected environmental impacts of COVID-19 on soil? Can SARS-CoV-2 be transmitted through soil, and under what conditions? Which soil processes and properties influence SARS-CoV-2 survival rates and times, as well as transmission? What are the specific links between soil health and COVID-19? What are the projected soil management scenarios in response to COVID-19? Questions such as these deserve more attention as the world seeks to recover from its most recent pandemic. These questions are critical to understanding soil and human health connections in the post-COVID-19 world.
HE-R: writing of original draft and supervision. All authors: conceptualization, methods, writing review, editing, and approval of submitted version.
This work was financed and supported by the Central Department of Mission, Egyptian Ministry of Higher Education (Mission 19/2020) in a grant to HE-R.
The authors declare that the research was conducted in the absence of any commercial or financial relationships that could be construed as a potential conflict of interest.
All claims expressed in this article are solely those of the authors and do not necessarily represent those of their affiliated organizations, or those of the publisher, the editors and the reviewers. Any product that may be evaluated in this article, or claim that may be made by its manufacturer, is not guaranteed or endorsed by the publisher.
1. El-Ramady H, Alshaal T, Omara AE-D, Elsakhawy T, Fawzy ZF. Soils and human creation in the holy quran from the point of view of soil science. Env Biodiv Soil Secur. (2019) 3:1–9. doi: 10.21608/jenvbs.2019.7856.1052
2. Brevik EC, Pereg L, Pereira P, Steffan JJ, Gedeon CI. Shelter, clothing, and fuel: often overlooked links between soils, ecosystem services, and human health. Sci Total Environ. (2019) 651(Pt 1):134–42. doi: 10.1016/j.scitotenv.2018.09.158
3. Kong F, Chen Y, Huang L, Yang Z, Zhu K. Human health risk visualization of potentially toxic elements in farmland soil: a combined method of source and probability. Ecotoxicol Environ Saf. (2021) 211:111922. doi: 10.1016/j.ecoenv.2021.111922
4. Fierer N, Wood SA, de Mesquita CPB. How microbes can, and cannot, be used to assess soil health. Soil Biol Biochem. (2021) 153:108111. doi: 10.1016/j.soilbio.2020.108111
5. De Corato U. Agricultural waste recycling in horticultural intensive farming systems by on-farm composting and compost-based tea application improves soil quality and plant health: a review under the perspective of a circular economy. Sci Total Environ. (2020) 738:139840. doi: 10.1016/j.scitotenv.2020.139840
6. Mahroof K, Omar A, Rana NP, Sivarajah U, Weerakkody V. Drone as a service (DaaS) in promoting cleaner agricultural production and Circular Economy for ethical Sustainable Supply Chain development. J Clean Product. (2021) 287:125522. doi: 10.1016/j.jclepro.2020.125522
7. Pozza LE, Field DJ. The science of soil security and food security. Soil Secur. (2020) 1:100002. doi: 10.1016/j.soisec.2020.100002
8. Mukhopadhyay R, Sarkar B, Jat HS, Sharma PC, Bolan NS. Soil salinity under climate change: challenges for sustainable agriculture and food security. J Environ Manage. (2021) 280:111736. doi: 10.1016/j.jenvman.2020.111736
9. Che Z, Wang J, Li J. Effects of water quality, irrigation amount and nitrogen applied on soil salinity and cotton production under mulched drip irrigation in arid Northwest China. Agric Water Manag. (2021) 247:106738. doi: 10.1016/j.agwat.2021.106738
10. Hirt H. Healthy soils for healthy plants for healthy humans: how beneficial microbes in the soil, food and gut are interconnected and how agriculture can contribute to human health. EMBO Rep. (2020) 21:e51069. doi: 10.15252/embr.202051069
11. Daliakopoulos IN, Tsanis IK, Koutroulis A, Kourgialas NN, Varouchakis AE, Karatzas GP, et al. The threat of soil salinity: a European scale review. Sci Total Environ. (2016) 573:727–39. doi: 10.1016/j.scitotenv.2016.08.177
12. Eswar D, Karuppusamy R, Chellamuthu S. Drivers of soil salinity and their correlation with climate change. Curr Opin Environ Sustain. (2021) 50:1–9. doi: 10.1016/j.cosust.2020.10.015
13. Fenta AA, Tsunekawa A, Haregeweyn N, Tsubo M, Yasuda H, Kawai T, et al. Agroecology-based soil erosion assessment for better conservation planning in Ethiopian river basins. Environ Res. (2021) 195:110786. doi: 10.1016/j.envres.2021.110786
14. Jimenez KJ, Rolim MM, Gomes IF, de Lima RP, Berrío LLA, Ortiz PFS. Numerical analysis applied to the study of soil stress and compaction due to mechanised sugarcane harvest. Soil Tillage Res. (2021) 206:104847. doi: 10.1016/j.still.2020.104847
15. Castillo P, Serra I, Townley B, Aburto F, López S, Tapia J, et al. Biogeochemistry of plant essential mineral nutrients across rock, soil, water and fruits in vineyards of Central Chile. Catena. (2021) 196:104905. doi: 10.1016/j.catena.2020.104905
16. Peng J, Chen Y, Xia Q, Rong G, Zhang J. Ecological risk and early warning of soil compound pollutants (HMs, PAHs, PCBs and OCPs) in an industrial city, Changchun, China. Environ Pollut. (2021) 272:116038. doi: 10.1016/j.envpol.2020.116038
17. Cai Y, Yan Y, Xu D, Xu X, Wang C, Wang X, et al. The fertile island effect collapses under extreme overgrazing: evidence from a shrub-encroached grassland. Plant Soil. (2020) 448:201–12. doi: 10.1007/s11104-020-04426-2
18. Steffan JJ, Derby JA, Brevik EC. Soil pathogens that may potentially cause pandemics, including severe acute respiratory syndrome (SARS) coronaviruses. Curr Opin Environ Sci Health. (2020) 17:35–40. doi: 10.1016/j.coesh.2020.08.005
19. Qian H, Zhang Q, Lu T, Peijnenburg WJGM, Penuelas J, Zhu Y-G. Lessons learned from COVID-19 on potentially pathogenic soil microorganisms. Soil Ecol Lett. (2021) 3:1–5. doi: 10.1007/s42832-020-0068-9
20. Bandala ER, Kruger BR, Cesarino I, Leao AL, Wijesiri B, Goonetilleke A. Impacts of COVID-19 pandemic on the wastewater pathway into surface water: a review. Sci Total Environ. (2021) 774:145586. doi: 10.1016/j.scitotenv.2021.145586
21. Lal R. Home gardening and urban agriculture for advancing food and nutritional security in response to the COVID-19 pandemic. Food Secur. (2020) 12:871–6. doi: 10.1007/s12571-020-01058-3
22. Lal R, Brevik EC, Dawson L, Field D, Glaser B, Hartemink AE, et al. Managing soils for recovering from the COVID-19 pandemic. Soil Syst. (2020) 4:46. doi: 10.3390/soilsystems4030046
23. Iqbal MM, Abid I, Hussain S, Shahzad N, Waqas MS, Iqbal MJ. The effects of regional climatic condition on the spread of COVID-19 at global scale. Sci Total Environ. (2020) 739:140101. doi: 10.1016/j.scitotenv.2020.140101
24. Coccia M. How do low wind speeds and high levels of air pollution support the spread of COVID-19? Atmos Pollut Res. (2021) 12:437–45. doi: 10.1016/j.apr.2020.10.002
25. Coccia M. The effects of atmospheric stability with low wind speed and of air pollution on the accelerated transmission dynamics of COVID-19. Int J Environ Stud. (2020) 1802937. doi: 10.1080/00207233.2020.1802937
26. Han J, He S. Urban flooding events pose risks of virus spread during the novel coronavirus (COVID-19) pandemic. Sci. Total Environ. (2021) 755:142491. doi: 10.1016/j.scitotenv.2020.142491
27. Kumar S. Effect of meteorological parameters on spread of COVID-19 in India and air quality during lockdown. Sci Total Environ. (2020) 745:141021. doi: 10.1016/j.scitotenv.2020.141021
28. Behera BC. Challenges in handling COVID-19 waste and its management mechanism: a review. Environ Nanotechnol Monit Manag. (2021) 15:100432. doi: 10.1016/j.enmm.2021.100432
29. Muthuraman Y, Lakshminarayanan I. A review of the COVID-19 pandemic and its interaction with environmental media. Environ Challenges. (2021) 3:100040. doi: 10.1016/j.envc.2021.100040
30. Mendes R, Garbeva P, Raaijmakers J. The rhizosphere microbiome: significance of plant beneficial, plant pathogenic, and human pathogenic microorganisms. FEMS Microbiol Rev. (2013) 37:34–63. doi: 10.1111/1574-6976.12028
31. van Bruggen AHC, Goss EM, Havelaar A, van Diepeningen AD, Finckh MR, Morris JG Jr. One health - cycling of diverse microbial communities as a connecting force for soil, plant, animal, human and ecosystem health. Sci Total Environ. (2019) 664:927–37. doi: 10.1016/j.scitotenv.2019.02.091
32. Tiozon RJr N, Fernie AR, Sreenivasulu N. Meeting human dietary vitamin requirements in the staple rice via strategies of biofortification and post-harvest fortification. Trends Food Sci Technol. (2021) 109:65–82. doi: 10.1016/j.tifs.2021.01.023
33. Shi Y, Li J, Sun Z. Success to iron biofortification of wheat grain by combining both plant and microbial genetics. Rhizosphere. (2020) 15:100218. doi: 10.1016/j.rhisph.2020.100218
34. de Lima Lessa JH, Raymundo JF, Corguinha APB, Martins FAD, Araujo AM, Santiago FEM, et al. Strategies for applying selenium for biofortification of rice in tropical soils and their effect on element accumulation and distribution in grains. J Cereal Sci. (2020) 96:103125. doi: 10.1016/j.jcs.2020.103125
35. Cheah ZX, O'Hare TJ, Harper SM, Kochanek J, Bell MJ. Zinc biofortification of immature maize and sweetcorn (Zea mays L.) kernels for human health. Sci Hortic. (2020) 272:109559. doi: 10.1016/j.scienta.2020.109559
36. Okwuonu IC, Narayanan NN, Egesi CN, Taylor NJ. Opportunities and challenges for biofortification of cassava to address iron and zinc deficiency in Nigeria. Glob Food Secur. (2021) 28:100478. doi: 10.1016/j.gfs.2020.100478
37. Siwela M, Pillay K, Govender L, Lottering S, Mudau FN, Modi AT, et al. Biofortified crops for combating hidden hunger in South Africa: availability, acceptability, micronutrient retention and bioavailability. Foods. (2020) 9:815. doi: 10.3390/foods9060815
38. Pessoa CC, Lidon FC, Coelho ARF, Caleiro JC, Marques AC, Luís IC, et al. Calcium biofortification of Rocha pears, tissues accumulation and physicochemical implications in fresh and heat-treated fruits. Sci Hortic. (2021) 277:109834. doi: 10.1016/j.scienta.2020.109834
39. Budke C, Straten ST, Mühling KH, Broll G, Daum D. Iodine biofortification of field-grown strawberries–Approaches and their limitations. Sci Hortic. (2020) 269:109317. doi: 10.1016/j.scienta.2020.109317
40. Jha AB, Warkentin TD. Biofortification of pulse crops: status and future perspectives. Plants. (2020) 9:73. doi: 10.3390/plants9010073
41. Hussain M, Mehboob N, Naveed M, Shehzadi K, Yasir TA. Optimizing boron seed coating level and boron-tolerant bacteria for improving yield and biofortification of Chickpea. J Soil Sci Plant Nutr. (2020) 20:2471–8. doi: 10.1007/s42729-020-00313-y
42. Grujcic D, Yazici AM, Tutus Y, Cakmak I, Singh BR. Biofortification of silage maize with zinc, iron and selenium as affected by nitrogen fertilization. Plants. (2021) 10:391. doi: 10.3390/plants10020391
43. Dobosy P, Kröpfl K, Óvári M, Sandil S, Németh K, Engloner A, et al. Biofortification of green bean (Phaseolus vulgaris L.) and lettuce (Lactuca sativa L.) with iodine in a plant-calcareous sandy soil system irrigated with water containing KI. J Food Compos Analy. (2020) 88:103434. doi: 10.1016/j.jfca.2020.103434
44. El-Ramady H, Faizy SED, Abdalla N, Taha H, Domokos-Szabolcsy É, Fari M, et al. Selenium and nano-selenium biofortification for human health: opportunities and challenges. Soil Syst. (2020) 4:57. doi: 10.3390/soilsystems4030057
45. González-García Y, Cárdenas-Álvarez C, CadenasPliego G, Benavides-Mendoza A, Cabrera-de-la-Fuente M, Sandoval-Rangel A, et al. Effect of three nanoparticles (Se, Si and Cu) on the bioactive compounds of bell pepper fruits under saline stress. Plants. (2021) 10:217. doi: 10.3390/plants10020217
46. Pal V, Singh G, Dhaliwal SS. A new approach in agronomic biofortification for improving zinc and iron content in Chickpea (Cicer arietinum L.) grain with simultaneous foliar application of zinc sulphate, ferrous sulphate and urea. J Soil Sci Plant Nutr. (2021) 21:883–96. doi: 10.1007/s42729-021-00408-0
47. Viscardi S, Marileo L, Barra PJ, Duran P, Inostroza-Blancheteau C. From farm to fork: it could be the case of Lactic Acid Bacteria in the stimulation of folates biofortification in food crops. Curr Opin Food Sci. (2020) 34:1–8. doi: 10.1016/j.cofs.2020.08.002
48. Zheng X, Kuijer HNJ, Al-Babili S. Carotenoid biofortification of crops in the CRISPR era. Trends Biotechnol. (2020) 39:857–60. doi: 10.1016/j.tibtech.2020.12.003
49. Jiang L, Strobbe S, Van Der Straeten D, Zhang C. Regulation of plant vitamin metabolism: backbone of biofortification for the alleviation of hidden hunger. Mol Plant. (2021) 14:40–60. doi: 10.1016/j.molp.2020.11.019
50. Anand U, Bianco F, Suresh S, Tripathi V, Núñez-Delgado A, Race M. SARS-CoV-2 and other viruses in soil: an environmental outlook. Environ Res. (2021) 198:111297. doi: 10.1016/j.envres.2021.111297
51. Shao L, Ge S, Jones T, Santosh M, Silva LF, Cao Y, et al. The role of airborne particles and environmental considerations in the transmission of SARS-CoV-2. Geosci Front. (2021) 12:101189. doi: 10.1016/j.gsf.2021.101189
52. Leonel LP, Tonetti AL. Wastewater reuse for crop irrigation: crop yield, soil and human health implications based on giardiasis epidemiology. Sci Total Environ. (2021) 775:145833. doi: 10.1016/j.scitotenv.2021.145833
53. Conde-Cid M, Arias-Estevez M, Núnez-Delgado A. SARS-CoV-2 and other pathogens could be determined in liquid samples from soils. Environ Pollut. (2021) 273:116445. doi: 10.1016/j.envpol.2021.116445
54. Conde-Cid M, Arias-Estevez M, Núnez-Delgado A. How to study SARS-CoV-2 in soils? Environ Res. (2021) 198:110464. doi: 10.1016/j.envres.2020.110464
55. Núnez-Delgado A. What do we know about the SARS-CoV-2 coronavirus in the environment? Sci Total Environ. (2020) 727:138647. doi: 10.1016/j.scitotenv.2020.138647
56. Núnez-Delgado A. SARS-CoV-2 in soils. Environ Res. (2020) 190:110045. doi: 10.1016/j.envres.2020.110045
57. Mishra BB, Roy R, Singh SK, Sharma SK, Prasad BD, Choudhary SK, et al. Preventive measures against transmission and multiplication of COVID-19 following the simple natural laws with soil, clay, and biodiversity. Open Acc J Envi Soi Sci. (2020) 5:622–32. doi: 10.32474/OAJESS.2020.05.000210
58. Brevik EC, Steffan JJ, Rodrigo-Comino J, Neubert D, Burgess LC, Cerdà A. Connecting the public with soil to improve human health. Eur J Soil Sci. (2019) 70:898–910. doi: 10.1111/ejss.12764
59. Aziz MZ, Yaseen M, Abbas T, Naveed M, Mustafa A, Hamid Y, et al. Foliar application of micronutrients enhances crop stand, yield and the biofortification essential for human health of different wheat cultivars. J Integr Agric. (2019) 18:1369–78. doi: 10.1016/S2095-3119(18)62095-7
60. Bouis HE, Saltzman A. Improving nutrition through biofortification: a review of evidence from HarvestPlus, 2003 through 2016. Glob Food Secur. (2017) 12:49–58. doi: 10.1016/j.gfs.2017.01.009
61. Dai H, Wei S, Twardowska I. Biofortification of soybean (Glycine max L.) with Se and Zn and enhancing its physiological functions by spiking these elements to soil during flowering phase. Sci Total Environ. (2020) 740:139648. doi: 10.1016/j.scitotenv.2020.139648
62. Silva VM, Nardeli AJ, Mendes NAC, de Moura Rocha M, Wilson L, Young SD, et al. Agronomic biofortification of cowpea with zinc: variation in primary metabolism responses and grain nutritional quality among 29 diverse genotypes. Plant Physiol Biochem. (2021) 162:378–87. doi: 10.1016/j.plaphy.2021.02.020
63. Trippe RC III, Pilon-Smits EAH. Selenium transport and metabolism in plants: phytoremediation and biofortification implications. J Hazard Mater. (2021) 404:124178. doi: 10.1016/j.jhazmat.2020.124178
64. Izydorczyk G, Ligas B, Mikula K, Witek-Krowiak A, Moustakas K, Chojnack K. Biofortification of edible plants with selenium and iodine–a systematic literature review. Sci Total Environ. (2021) 754:141983. doi: 10.1016/j.scitotenv.2020.141983
65. Roda FA, Marques I, Batista-Santos P, Esquível MG, Ndayiragije A, Lidon FC, et al. Rice biofortification with zinc and selenium: a transcriptomic approach to understand mineral accumulation in flag leaves. Front Genet. (2020) 11:543. doi: 10.3389/fgene.2020.00543
66. Yadav R, Ror P, Rathore P, Ramakrishna W. Bacteria from native soil in combination with arbuscular mycorrhizal fungi augment wheat yield and biofortification. Plant Physiol Biochem. (2020) 150:222–33. doi: 10.1016/j.plaphy.2020.02.039
67. Ye Y, Qu J, Pu Y, Rao S, Xu F, Wu C. Selenium biofortification of crop food by beneficial microorganisms. J Fungi. (2020) 6:59. doi: 10.3390/jof6020059
68. Van Der Straeten D, Bhullar NK, De Steur H, Gruissem W, MacKenzie D, Pfeiffer W, et al. Multiplying the efficiency and impact of biofortification through metabolic engineering. Nature Comm. (2020) 11:5203. doi: 10.1038/s41467-020-19020-4
69. Sohrabi M, Mehrjerdi MZ, Karimi S, Tavallali V. Using gypsum and selenium foliar application for mineral biofortification and improving the bioactive compounds of garlic ecotypes. Ind Crops Prod. (2020) 154:112742. doi: 10.1016/j.indcrop.2020.112742
70. Sánchez-Rodríguez AR, Marín-Paredes M, González-Guzmán A, Méndez JM, Sánchez-Parra M, Sacristán D, et al. Zinc biofortification strategies for wheat grown on calcareous Vertisols in southern Spain: application method and rate. Plant Soil. (2021) 462:125–40. doi: 10.1007/s11104-021-04863-7
71. Kumar S, Pandey G. Biofortification of pulses and legumes to enhance nutrition. Heliyon. (2020) 6:e03682. doi: 10.1016/j.heliyon.2020.e03682
72. Maqbool A, Abrar M, Bakhsh A, Çalişkan S, Khan HZ, Aslam M, et al. Biofortification under climate change: the fight between quality and quantity. In: Fahad S, Hasanuzzaman M, Alam M, Ullah H, Saeed M, Ali Khan I, Adnan M, et al. editors. Environment, Climate, Plant and Vegetation Growth. Cham: Springer Nature Switzerland AG (2020). p. 173–227. doi: 10.1007/978-3-030-49732-3_9
73. Gastélum-Estrada A, Serna-Saldívar SO, Jacobo-Velázquez DA. Fighting the COVID-19 pandemic through biofortification: innovative approaches to improve the immunomodulating capacity of foods. ACS Food Sci Technol. (2021) 1:480–6. doi: 10.1021/acsfoodscitech.1c00019
74. Li Z, Lun F, Liu M, Xiao X, Wang C, Wang L, et al. Rapid diagnosis of agricultural soil health: a novel soil health index based on natural soil productivity and human management. J Environ Manage. (2021) 277:111402. doi: 10.1016/j.jenvman.2020.111402
75. USDA. Soil Health. (2021) Available online at: https://www.nrcs.usda.gov/wps/portal/nrcs/main/soils/health/ (accessed June 15, 2021).
76. Nunes MR, Karlen DL, Veum KS, Moorman TB, Cambardella CA. Biological soil health indicators respond to tillage intensity: a US meta-analysis. Geoderma. (2020) 369:114335. doi: 10.1016/j.geoderma.2020.114335
77. Friedrichsen CN, Hagen-Zakarison S, Friesen ML, McFarland CR, Tao H, Wulfhorst JD. Soil health and well-being: redefining soil health based upon a plurality of values. Soil Secur. (2021) 2:100004. doi: 10.1016/j.soisec.2021.100004
78. Poch RM, dos Anjos LHC, Attia R, Balks M, Benavides-Mendoza A, Bolaños-Benavides MM, et al. Soil: the great connector of our lives now and beyond COVID-19. Soil. (2020) 6:541–7. doi: 10.5194/soil-6-541-2020
79. El-Ramady H, Abdalla N, Elbasiouny H, Elbehiry F, Elsakhawy T, Omara AE, et al. Nano-biofortification of different crops to immune against COVID-19: a review. Ecotoxicol Environ Saf. (2021) 222:112500. doi: 10.1016/j.ecoenv.2021.112500
80. Das UN. Can bioactive lipids inactivate coronavirus (COVID-19)? Arch Med Res. (2020) 51:282–6. doi: 10.1016/j.arcmed.2020.03.004
81. Khadke S, Ahmed N, Ahmed N, Ratts R, Raju S, Gallogly M, et al. Harnessing the immune system to overcome cytokine storm and reduce viral load in COVID-19: a review of the phases of illness and therapeutic agents. Virol J. (2020) 17:154. doi: 10.1186/s12985-020-01415-w
82. Gao G, Zhu Z, Fan L, Ye S, Huang Z, Shi Q, et al. Absent immune response to SARS-CoV-2 in a 3-month recurrence of coronavirus disease 2019 (COVID-19) case. Infection. (2021) 49:57–61. doi: 10.1007/s15010-020-01485-6
83. da Silveira MP, Fagundes KKS, Bizuti MR, Starck É, Rossi RC, Silva DTR. Physical exercise as a tool to help the immune system against COVID-19: an integrative review of the current literature. Clin Exp Med. (2021) 21:15–28. doi: 10.1007/s10238-020-00650-3
84. Singh P, Tripathi MK, Yasir M, Khare R, Tripathi MK, Shrivastava R. Potential inhibitors for SARS-CoV-2 and functional food components as nutritional supplement for COVID-19: a review. Plant Foods Hum Nutr. (2020) 75:458–66. doi: 10.1007/s11130-020-00861-9
85. Han S, Roy PK, Hossain MI, Byun K-H, Choi C, Ha S-D. COVID-19 pandemic crisis and food safety: Implications and inactivation strategies. Trends Food Sci Technol. (2021) 109:25–36. doi: 10.1016/j.tifs.2021.01.004
86. Capodice JL, Chubak BM. Traditional Chinese herbal medicine-potential therapeutic application for the treatment of COVID-19. Chin Med. (2021) 16:24. doi: 10.1186/s13020-020-00419-6
87. Stark JC. Food production, human health and planet health amid Covid-19. Explore. (2021) 17:179–80. doi: 10.1016/j.explore.2020.12.009
88. Brevik EC, Slaughter L, Singh BR, Steffan JJ, Collier D, Barnhart P, et al. Soils and human health: current status and future needs. Air Soil Water Res. (2020) 13:1–23. doi: 10.1177/1178622120934441
89. Heck S, Campos H, Barker I, Okello JJ, Baral A, Boy E, et al. Resilient agri-food systems for nutrition amidst COVID-19: evidence and lessons from food-based approaches to overcome micronutrient deficiency and rebuild livelihoods after crises. Food Secur. (2020) 12:823–30. doi: 10.1007/s12571-020-01067-2
90. Bidisha SH, Mahmood T, Hossain MB. Assessing food poverty, vulnerability and food consumption inequality in the context of COVID-19: a case of Bangladesh. Soc Indic Res. (2021) 155:187–210. doi: 10.1007/s11205-020-02596-1
91. Mishra K, Rampal J. The COVID-19 pandemic and food insecurity: a viewpoint on India. World Dev. (2020) 135:105068. doi: 10.1016/j.worlddev.2020.105068
92. Dabone C, Mbagwu I, Muray M, Ubangha L, Kohoun B, Etowa E, et al. Global food insecurity and African, Caribbean, and Black (ACB) populations during the COVID-19 pandemic: a rapid review. J Racial Ethn Health Disparities. (2021) 1–16. doi: 10.1007/s40615-021-00973-1
93. Chiwona-Karltun L, Amuakwa-Mensah F, Wamala-Larsson C, Amuakwa-Mensah S, Hatab AA, Made N, et al. COVID-19: from health crises to food security anxiety and policy implications. Ambio. (2021) 50:794–811. doi: 10.1007/s13280-020-01481-y
94. Pakravan-Charvadeh MR, Mohammadi-Nasrabadi M, Gholamrezai S, Vatanparast H, Flora C, Nabavi-Pelesaraei A. The short-term effects of COVID-19 outbreak on dietary diversity and food security status of Iranian households (a case study in Tehran province). J Clean Product. (2021) 281:124537. doi: 10.1016/j.jclepro.2020.124537
95. Payán DD, Rios LKD, Ramírez AS, Young M-EDT. Structural barriers influencing food insecurity, malnutrition, and health among latinas during and after COVID-19: considerations and recommendations. J Acad Nutr Dietet. (2021) 121:837–43. doi: 10.1016/j.jand.2021.01.005
96. McLoughlin GM, McCarthy JA, McGuirt JT, Singleton CR, Dunn CG, Gadhoke P. Addressing food insecurity through a health equity lens: a case study of large urban school districts during the COVID-19 pandemic. J Urban Health. (2020) 97:759–75. doi: 10.1007/s11524-020-00476-0
97. Littlejohn P, Finlay BB. When a pandemic and an epidemic collide: COVID-19, gut microbiota, and the double burden of malnutrition. BMC Med. (2021) 19:31. doi: 10.1186/s12916-021-01910-z
98. Mohan M, Rue HA, Bajaj S, Galgamuwa GP, Adrah E, Aghai MM, et al. Afforestation, reforestation and new challenges from COVID-19: thirty-three recommendations to support civil society organizations (CSOs). J Environ Manag. (2021) 9:112277. doi: 10.1016/j.jenvman.2021.112277
99. Ilesanmi FF, Ilesanmi OS, Afolabi AA. The effects of the COVID-19 pandemic on food losses in the agricultural value chains in Africa: the Nigerian case study. Public Health Pract. (2021) 2:100087. doi: 10.1016/j.puhip.2021.100087
100. Wolfe MT, Patel PC. Everybody hurts: self-employment, financial concerns, mental distress, and well-being during COVID-19. J Bus VenturInsights. (2021) 15:e00231. doi: 10.1016/j.jbvi.2021.e00231
Keywords: environmental pollution, corona virus, SARS-CoV-2, soil health, soil quality
Citation: El-Ramady H, Brevik EC, Elbasiouny H, Elbehiry F, El-Henawy A, Faizy SE-D, Elsakhawy T, Omara AE-D, Amer M and Eid Y (2021) Soils, Biofortification, and Human Health Under COVID-19: Challenges and Opportunities. Front. Soil Sci. 1:732971. doi: 10.3389/fsoil.2021.732971
Received: 29 June 2021; Accepted: 18 August 2021;
Published: 13 September 2021.
Edited by:
Shahid Hussain, Bahauddin Zakariya University, PakistanReviewed by:
Daniel A. Jacobo-Velázquez, Monterrey Institute of Technology and Higher Education (ITESM), MexicoCopyright © 2021 El-Ramady, Brevik, Elbasiouny, Elbehiry, El-Henawy, Faizy, Elsakhawy, Omara, Amer and Eid. This is an open-access article distributed under the terms of the Creative Commons Attribution License (CC BY). The use, distribution or reproduction in other forums is permitted, provided the original author(s) and the copyright owner(s) are credited and that the original publication in this journal is cited, in accordance with accepted academic practice. No use, distribution or reproduction is permitted which does not comply with these terms.
*Correspondence: Eric C. Brevik, ZXJpYy5icmV2aWtAc2l1LmVkdQ==
Disclaimer: All claims expressed in this article are solely those of the authors and do not necessarily represent those of their affiliated organizations, or those of the publisher, the editors and the reviewers. Any product that may be evaluated in this article or claim that may be made by its manufacturer is not guaranteed or endorsed by the publisher.
Research integrity at Frontiers
Learn more about the work of our research integrity team to safeguard the quality of each article we publish.