- 1Department of Crop and Soil Sciences, Kwame Nkrumah University of Science and Technology, Kumasi, Ghana
- 2Department of General Agriculture, Sunyani Technical University, Sunyani, Ghana
- 3Department of Theoretical and Applied Biology, Kwame Nkrumah University of Science and Technology, Kumasi, Ghana
- 4Forest and Horticultural Crops Research, University of Ghana, Accra, Ghana
- 5Department of Environmental Science, Kwame Nkrumah University of Science and Technology, Kumasi, Ghana
A better understanding of soil fertility factors that constraint positive crop response to fertilizer inputs will facilitate the improvement of soil nutrient management. In this study, a nutrient omission trial was carried out in a greenhouse condition to identify soil chemical properties limiting in non-responsive soils and to ascertain their effect on soybean (Glycine max) production. The treatments evaluated were control (distilled water), complete nutrient solution (all nutrients), and complete solution with the omission of each of N, P, K, Ca, Mg, and S and micronutrients (Fe, Zn, Cu, Mn, B, and Mo) arranged in a completely randomized design with three replications. After the greenhouse study, the identified limiting nutrients were tested with or without FertiSoil (commercial compost) in a 3 year field experiment. Results of the soil analyses showed low fertility status of the non-responsive soils. The sufficiency quotient index revealed non-responsive soils in all the sites to be predominantly limiting in P and K. The occurrence of other limiting nutrients was also identified: Pishegu (Zn, B), Serekpere (Mg, S), Daffiama Saapare (Ca, Mg, S), and Naaga (Mg, S, Zn, B). The nutrient inputs positively influenced soybean yield response in all the locations. The application of PKZnB with FertiSoil and FertiSoil alone significantly increased soybean grain yields by 585 and 477 kg ha−1, respectively, at Pishegu. Soybean grain yields also increased by 585, 573, and 364 kg ha−1 under the FertiSoil, PKMgS + FertiSoil, and PKMgS applications at Serekpere, respectively. At Daffiama Saapare, the highest (103%) percent increase in soybean grain yield was recorded from the combined application of PKMgSCa and FertiSoil. However, the application of FertiSoil and PKMgSCa singly equally increased soybean grain yield by 77%. Percent soybean grain yield increases of 86, 84, and 74% were observed when PKMgSZnB + FertiSoil, PKMgSZnB, and FertiSoil were applied, respectively, at Naaga. In absolute terms, 83% of the fields had a positive response to mineral fertilizer and 93% to FertiSoil and mineral fertilizer + FertiSoil applications. Organic amendment and/or site-specific fertilizer applications are the best options for alleviating poor or no crop responses to inputs and improve productivity on non-responsive soils.
Introduction
Soil degradation is a major global problem, but more prominent in developing countries where the majority of the populace depend on crop production for their livelihoods (1). Poor land management is the major cause of land degradation among biophysical, socioeconomic, and political factors (2). Continuous cropping with little or no nutrient replenishment has led to soil nutrient mining across sub-Saharan African (SSA) countries especially on smallholder farms (3). Consequently, crop yields obtained from smallholder farms are far below the potential yield resulting in yield gaps. Research efforts to improve soil fertility in SSA over the last three decades have mainly focused on N, P, and K fertilizers (4). However, continuous application of such fertilizers alone has contributed to the depletion of other essential nutrients such as B, Zn, Cu, Fe, and Mn (5), triggering lack of response to future fertilizer applications and reduction in crop yield. Non-responsive soils are soils that do not respond to fertilizer application due to other constraints besides the nutrients contained in the fertilizer (6). Non-responsiveness of a soil to mineral fertilizer application has been attributed to edaphic factors such as soil fertility gradients, nutrient depletion, soil type, low soil organic matter, acidity, and moisture stress and environmental factors (7, 8). Soils that are non-responsive to mineral fertilizer applications are increasingly becoming widespread in SSA. Kihara et al. (9) found 50% of the study sites in Tanzania, Malawi, Kenya, Mali, and Nigeria to be non-responsive to fertilizer application and organic amendments. Zerihun et al. (10) also found over 25% farm sites non-responsive to P fertilizers in western Ethiopia. Agronomical trials implemented by N2Africa project putting nitrogen fixation to work in northern Ghana observed 28% of fields with low crop response to P fertilizers (11). The occurrence of non-responsive soils increases the variability of crop responses to best-fit management practices that worsen the yield gap. To address the non-responsiveness of crops to fertilizer inputs, various diagnostic studies have revealed deficiencies of secondary and micronutrients (Ca, Mg, S, Cu, Zn, B) as a major cause (9, 12, 13). There is, however, lack of systematic and comprehensive knowledge about non-responsive soils, constraints in such soils, and their management in maximizing crop response to fertilizer inputs. In order to optimize crop response and nutrient efficiency, there is a need for proper nutrient management strategies that should be addressed. The Sustainable Development Goal 15.3 by the United Nations aims toward land degradation neutrality (LDN) and land restoration by the year 2030 (14). A credible option for restoring the productive capacity and quality of the soil is to identify the underlying limiting factors and address them. This can be achieved through a site-specific nutrient management with nutrient deficiency diagnosis. The aim of this study was to characterize and identify nutrient deficiencies of non-responsive soils and evaluate the effect of balanced nutrition on crop yield.
Materials and Methods
Selection and Description of Poor Non-responsive Sites
Study sites were purposively selected from N2Africa project area in the Northern, Upper West, and Upper East regions of Ghana for agronomic trials during cropping seasons of 2011 and 2012 (11). Soils non-responsive to P fertilizer application were determined by comparing the grain yield of P treated plots with the control plot, and where the control yields were significantly higher or equal to the P treated plots, the soils were designated as non-responsive (6). Seventy-four (74) fields were identified to be non-responsive to P fertilizer. The 74 fields were within 39 communities in the Northern (14), Upper East (15), and Upper West regions (10), respectively. The Northern region falls within the Guinea savannah, and the Upper East is of the Sudan savannah. The Upper West region is sub-divided into two (2) agro-ecological zones, the southern part being the Guinea savannah and the northern and north eastern part. The Guinea and Sudan Savannah agro-ecological zones are distinguished by a unimodal rainfall season. A 10 year average annual rainfall for Northern, Upper East, and Upper West regions was 1,122, 927, and 817 mm, respectively (15).
Soil Sampling and Analyses
Soil samples from each of the 74 non-responsive soil fields were collected for physico-chemical analyses. In each field, 10 soil samples were randomly taken from a 20 cm depth with an auger and thoroughly mixed to form a composite sample. A sub-sample was then taken and processed for soil physico-chemical properties at the Soil Science laboratory, Kwame Nkrumah University of Science and Technology (KNUST). Soil pH was determined in a 1:2.5 (soil:water) ratio, and total N was determined by the macro Kjeldahl method as described by Bremner (16), available P by the Bray 1 method (17), and organic carbon by the modified Walkley and Black procedure as described by Nelson and Sommers (18). Exchangeable K, Ca, and Mg were extracted by 1 M ammonium acetate (NH4OAc) solution at pH 7 as described by Black (19). Iron, zinc, copper, and manganese were extracted with 0.005 M DTPA (20), and particle size distribution was determined by the hydrometer method (21).
Assessment of Nutrients Limiting in Non-responsive Soils
The double-pot technique (22) was used to identify nutrients in short supply in the selected non-responsive soils. A greenhouse trial was conducted on soils selected from six communities, two from each region. Nutrient solution was prepared according to the modified Hoagland's half strength nutrient solution as described by Paradiso et al. (23) specific to soybean requirement. The rate of ion concentration for the nutrient solution was 7.5, 0.5., 3.0, 2.5, 1.0, 1.0 mM/L (N, P, K, Ca, Mg, S) and 60.0, 7.4, 0.96, 1.04, 7.13, 0.01 μM/L (Fe, Mn, Zn, Cu, B, Mo). The experiment consisted of eight different nutrient solutions and a control arranged in a completely randomized block design and replicated three times (eight nutrient solution × three levels) as presented in Table 1.
Growth and Nutrient Sufficiency
Soybean shoots (one shoot per pot) were measured at 24 and 34 days after sowing (DAS). Regular observations were made to detect visual nutrient deficiency symptoms on foliar parts of plants. At 24 and 34 DAS, measurements were taken to establish the relative growth rate (Rs) and nutrient sufficiency quotients. The relative growth rate measurement was determined by dry matter increase using the pairing method developed by Evans (24) as follows:
where Rs is the relative growth rate (g g−1 day−1), ln is natural logarithm, and W2 and W1 are mean shoot dry weights in grams per pot at times t2 and t1 of growing period.
To measure the availability of a nutrient, the sufficiency quotient (SQ) as an index was used to determine the differences in growth between plants on an omitted nutrient solution and the complete solution (25). When the value of SQ is 1, the availability of that particular nutrient in the soil was considered as high as that in the solution. Nutrients with SQ values of 0–0.25, 0.25–0.5, 0.5–0.75, and >0.75 were most limiting, limiting, moderately limiting, and sufficient, respectively (22, 26). To ease interpretation, results were expressed as percentage, with the complete solution taken as 100%. The SQ is expressed as:
where SQx is the sufficiency quotient for an omitted nutrient, (Rs)C is the relative growth rate of plants growing on a complete solution, and (Rs)-X is the relative growth of plants growing on an omitted nutrient solution.
Application of Mineral Nutrients and Compost to Rehabilitate Non-responsive Soils
Following the results obtained from the nutrient omission trial, a 3 year field experiment was conducted on non-responsive soils in Northern, Upper West, and Upper East regions of Ghana to determine the impact of the identified limiting nutrient applications on the non-responsive soils. The experiment evaluated four (4) treatments; (i) control (no input), (ii) mineral fertilizer as per identified limiting nutrients in each location, (iii) FertiSoil (commercial compost) only, and (iv) mineral fertilizer + FertiSoil. Compost samples were air-dried, milled, and sieved through a 2 mm mesh for analyses (Table 2). Organic C (Walkley and Black wet oxidation method), total N (Kjeldahl method), phosphorus (Bray P 1), and potassium ammonium acetate flame photometry method of the samples were determined. Lignin content was determined using the Acid Detergent Fiber (ADF) method as described by Van Soest and Wine (27) and polyphenol content by the Folin–Denis method (28). Dry matter content was also determined. The compost used for the study was a commercialized product called FertiSoil produced by DeCo! sustainable farming in Tamale, region, Ghana.
The field layout consisted of 12 plots of 5 × 5 m dimension separated by a 1 m buffer. Four treatments were tested in a randomized complete block design with three replications. Mineral fertilizers were applied in bands with triple super phosphate (TSP) at a rate of 30 kg P ha−1, muriate of potash (MOP) at a rate of 20 kg K ha−1, and magnesium sulfate (MgSO4) for Mg and S at a rate of 2.5 and 0.5 kg ha−1, respectively. Calcium sulfate (CaSO4) was applied at a rate of 15 kg Ca ha−1, zinc sulfate (ZnSO4) at a rate of 2.5 kg Zn ha−1, and fertibor at a rate of 0.5 kg B ha−1 at 2 weeks after sowing. FertiSoil was applied at a rate of 5 t ha−1 to the respective plots and mixed with the top soil prior to planting. Soybean cultivar “Jenguma” (TGx series) was sown at two seeds per hill at a distance of 75 cm × 10 cm. A bradyrhizobium inoculant (Nodumax) was applied to the seed at a rate of 5 g kg−1, and sowed after air drying for 30 min. Soybean plants were harvested at full maturity (R8 stage), threshed, and winnowed. Grain yield was then calculated on per hectare basis.
Statistical Analyses
All statistical analyses were conducted with JMP® Pro 13.0.0 (SAS Institution Inc., Cary, NC, 1987–2007). A descriptive statistic was performed on three replicates of each soil sample after laboratory to determine the mean and standard deviation of soil physico-chemical properties. Data in the nutrient omission trial were subjected to analysis of variance (ANOVA), where overall treatment means were significant at p < 0.05 and the means were separated with Tukey HSD test. A mixed effect linear model was performed to assess the effect of nutrient inputs on grain yield. Location and nutrient input were the fixed factors, and the cropping year and replication were the random factors.
Cumulative probability curves of absolute and relative yield response to nutrient application with reference to the control per individual farms were calculated (29).
Results
Nutrient Status of Non-responsive Soils
Table 3 shows the soil physico-chemical properties of the study locations. Soil pH levels revealed soils to be moderately acidic (5.53–6.10) in all the soil samples from the Northern, Upper East, and West regions. In all the sites, the soil organic C (<1%) and total N contents (<0.1%) were very low, whereas available P content (7.51–8.51 mg kg−1) was below the critical value for soybean that is 15 mg kg−1 (30) across regions. Exchangeable K content of the soils in the study locations varied from 0.08 to 0.20 cmol(+) kg−1. The mean values in Northern region were moderate and low in Upper East and West regions. Exchangeable Ca content of all the soil sampled from the Northern, Upper East, and West regions were moderate (2.14–4.13 cmol(+) kg−1). The Mg content in soils of the study locations varied from low to moderate (0.72–1.21 cmol(+) kg−1). Exchangeable Mg levels in the soil samples were found to be low (<1 cmol(+) kg−1) in the Upper East and West regions and moderate (1.21 cmol(+) kg−1) in the Northern region. Extractable Fe concentration in all the soils sampled were found to be low (1.56–2.79 mg kg−1) with very low (<1 mg kg−1) Zn concentrations in Northern, Upper East, and West regions. The textural class of soils experimented on in Northern and Upper East regions was sandy loam with sand contents of 69 and 75%, respectively, while soils from the Upper West had the highest sand percentage (90%).
Assessment of Nutrients Limiting in Non-responsive Soils
Effect of Mineral Nutrient Solutions on Relative Growth Rate (Rs)
Significant variations (p < 0.05) in relative growth rates were recorded from growth analysis of the various treatments across locations except for Daffiama-Dakyea (Table 4). Relative growth rate decreased with each nutrient omitted from the Complete recording the highest 0.153 g g−1 day−1 at Pishegu. At Serekpere, Rs decreased significantly with the omission of macronutrients, except N. A similar observation was made at Daffiama-Sapaare, where significant relative increases of 272 and 188% in Rs were recorded for Complete and Micronutrient omitted nutrient solutions, respectively. The differences in Rs among different nutrient solution compositions were not significantly (p = 0.285) different at Daffiama-Dakyea. The Complete nutrient solution had the highest Rs of 0.159 g g−1 day−1 and was significantly (p < 0.001) different from the omitted nutrient solutions at Naaga.
Nutrient Sufficiency Quotient of Limiting Nutrients From Study Sites
Nutrient sufficiency quotients of nutrients in the study locations are as presented in Table 5 in relation to the Complete nutrient solution. To ease interpretation, the results were expressed in percentages. Nutrients solution without P, K, Micronutrients, and the Control had sufficiency quotient below 50% at Pishegu. At Serekpere, plants grown in the Control, P, K, Mg, and S omitted solutions had lower sufficiency quotients ranging from 30 to 41%. Potassium omitted nutrient solution had the lowest sufficiency quotient of 21% followed by 27% for the control, 33% for Mg, 37% for S, and 38% for P omitted treatments in Daffiama-Saapare. On the contrary, nutrient omitted solutions in Daffiama-Dakyea and the Control were all in the third quartile of 100%. All plants grown on the nutrient omitted solutions including the Control except for N and Ca omitted pots were generally low with <40% in sufficiency quotient at Naaga.
Soybean Responses to Mineral Fertilizers and Organic Amendment Application
Results on the effect of mineral fertilizers and FertiSoil on soybean grain yield are shown in Table 6. Significant (p < 0.05) differences in soybean grain yield were observed among treatments across sites. The application of PKZnB + FertiSoil and FertiSoil significantly (p = 0.002) increased soybean grain yield by 55 and 45% over the control, respectively, at Pishegu. The nutrient inputs significantly (p < 0.001) increased soybean grain yield compared to the control at Serekpere. Addition of PKMgS increased soybean grain yield by 364 kg ha−1, whereas PKMgS + FertiSoil increased grain yield by 573 kg ha−1 and 585 kg ha−1, which was also obtained with FertiSoil application. At Dafiama Saapare, the combined application of PKMgSCa and FertiSoil significantly (p = 0.004) increased soybean grain yield by 103%, while PKMgSCa and FertiSoil separately, equally produced a grain yield increase of 77%. Soybean response to PKMgSZnB and/or FertiSoil also varied significantly (p < 0.001) at Naaga (Table 6). The highest (1,201 kg ha−1) grain yield was produced under the PKMgSZnB + FertiSoil treated plots, followed by PKMgSZnB (1,188 kg ha−1) and FertiSoil (1,122 kg ha−1).
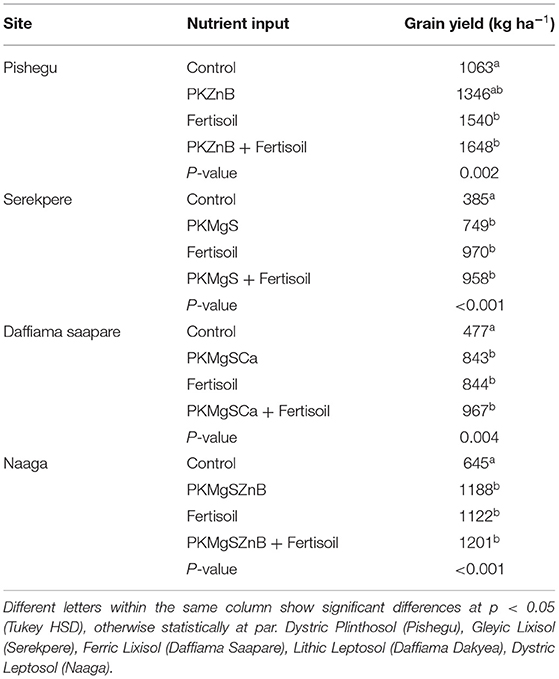
Table 6. Average soybean grain yields response as influenced by mineral fertilizer and FertiSoil in northern Ghana.
The absolute response (treatment yield minus control yield) and relative response (treatment yield minus control yield divided by the control yield, multiplied by 100) are presented in Figures 1A,B. Cumulative soybean yields over the three cropping seasons show a large variation of crop response to mineral fertilizers and fertisoil across farms and within treatments (Figure 1). In absolute terms, 63, 72, and 89% of the fields had a positive response to mineral fertilizer + fertisoil, fertisoil, and mineral fertilizer applications, respectively, in relation to the control (Figure 1A). Fifty percent of the farmers increased their soybean grain yield by 712.50 kg ha−1 or more with fertisoil application. In absolute terms, 54% of farmers increased their soybean grain yields by 715.65 kg ha−1 or more with mineral fertilizer + fertisoil application. Sixty-seven percent of the farmers had absolute increase in soybean grain by 700 kg ha−1 or more with mineral fertilizer application. Gains of over 1,000 kg ha−1 of soybean grain yield were obtained by 11, 28, and 37% fields with mineral fertilizer, fertisoil, and mineral fertilizer + fertisoil application, respectively (Figure 1A). There was a 2% probability chance of achieving a negative response due to the application fertisoil and mineral fertilizer + fertisoil.
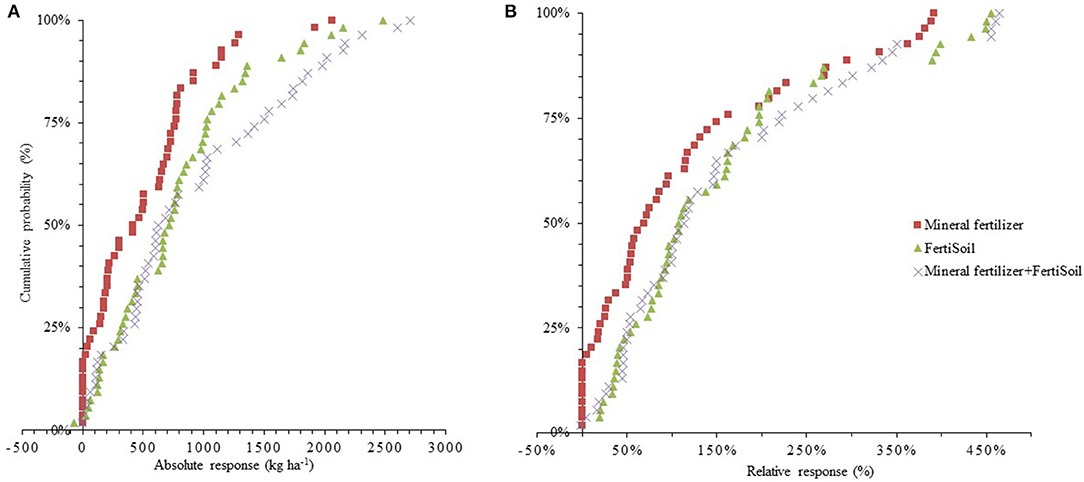
Figure 1. Cumulated probability graph of individual non-responsive farms in northern Ghana (years 1, 2, and 3): (A) soybean absolute response and (B) soybean relative response to mineral fertilizers and/or FertiSoil in comparison to the control.
Farmers regard a 10% or more increase in grain yield by a treatment as the least observable increment. A relative increase of 10% or more occurred on 80, 94, and 96% fields treated with mineral fertilizer, mineral fertilizer + fertisoil, and fertisoil, respectively (Figure 1B).
Discussion
Physical and chemical characteristics of soil are important soil quality indicators that directly or indirectly affect the quality of plant growth and its products. The nutrient levels of non-responsive soils in northern Ghana were generally below critical levels for crop production (Table 3). The result confirms the low inherent fertility status of soils of the Guinea and Sudan savannah agro-ecological zones of Ghana (31). Practices such as intensive cultivation (32), poor farming practices (33), overgrazing (34), and bushfires (35) further deplete the soil of its essential plant nutrients in varying degrees. In a related study, Fermont et al. (36) observed that low soil fertility limited cassava response to N, P, or K fertilizers in East Africa.
All the sites surveyed in northern Ghana were critically low in organic C, N, and P. This is in line with findings of Antwi et al. (37), Asei et al. (38), and Tetteh et al. (39) on soil fertility levels in the Guinea and Sudan savannah zone of Ghana. This is attributable to the dominant soil groups of the region, which are highly weathered with poor soil texture, characterized by low organic matter content and low cation exchange capacity, thereby causing low nutrient levels (40). The low carbon, N, and P contents can also be explained by the low vegetative cover, low input application, biomass removal, regular annual bush fires, erosion, and leaching during rainy seasons (41, 42). The high temperatures of the northern savanna zones further exacerbate the low carbon content by increasing the rate of mineralization, which decreases carbon accumulation (43).
According to Lal (44), an optimum range of soil organic carbon (SOC) is required to improve water and nutrient retention capacities. This is also in line with results obtained by Musinguzi et al. (45) that low fertility fields (<1.2% SOC) had lowest response to mineral fertilizer N application on a Ferralsol. Such low SOC soils are vulnerable to N leaching, and hence, the soils are less responsive to N fertilizers (46). Additionally, Benbi and Brar (47) also found no response to P fertilizer application under low SOC soils and which Bationo et al. (48) attributed to the high P sorption of soils low in SOC.
Exchangeable cations (K+, Ca2+, and Mg2+) were found to be moderate in some communities of the three regions except Mg, which were moderate for all the sites. However, few sites in the Chereponi district of the Northern region had high Mg levels. The results obtained in this study are consistent with results obtained by Tetteh et al. (49) who reported low to moderate nutrient levels and higher values for exchangeable cations in soils of the northern part of Ghana. The substantial differentiation of soil nutrient levels across communities confirms the heterogeneity of smallholder farms in SSA (50).
Iron and Zn levels varied from low to very low across all the study locations. In contrast, Awuni and Reynolds (51) reported medium to optimum levels for Fe and Zn in some sites of northern Ghana attesting to the high variability in Fe and Zn contents of the soils. Copper levels were low in soils in the Northern and Upper West regions except Upper East, which was medium. Previous research by Oluwadare et al. (52) has also shown Cu deficiency in the Guinea savannah soils of Nigeria. Medium levels of Mn were observed in all the soil samples. The soils studied by Boateng et al. (53) also showed moderate Mn levels in the Guinea savannah zone of Ghana. A review by Dimkpa and Bindraban (54) documented that micronutrient deficiencies affect the optimal function of plant metabolism processes of N, P, and K and crop responses.
The pH range (5.5–6.0) observed in the study (Table 3) was within the pH values obtained by Tetteh et al. (49) in a study to evaluate soil suitability indices for cereal production in northern Ghana (5.0–7.5). The soil pH level influences the solubility and availability of nutrients. Ch'ng et al. (55) and Miller (56) reported soil with pH below 6 to be deficient in P, as soil P is adsorbed to Al and Fe oxides, rendering it insoluble and unavailable to plants. The high P fixation in soils having a moderately acidic pH is primarily due to the presence of acid-forming cations associated with H+, Al3+, Fe2+, and Fe3+ and the high levels of sand with low buffering capacity (57). This may partly explain the lack of response to P fertilizers. The texture of the soils was sandy and is mainly accounted for by the parent material (58). The high mean sand percentages observed in this study correspond with the findings of Ziblim et al. (59) and MacCarthy et al. (60). The dominance of sand in the northern savannah agro-ecological zones of Ghana is because the soils are mostly developed from granite and Voltanian sandstone (61). Zheng et al. (62) studied the influence of soil texture on P fertilizer and observed that soils with high sand and low clay content affect P uptake; such soils have lower P supply, available water, and P diffusion rates compared to fine textured soils.
To estimate the availability of a particular nutrient, the sufficiency quotient, which measures the relative growth rate between a seedling on a complete and an omitted nutrient solution, was determined (22, 26). Soils of Pishegu were found to be limiting in P, K, and micronutrients (Zn, B) (Table 5). Essential nutrients like P, K, Mg, and S were found to be limiting in both Serekpere and Daffiama Saapare, with SQ indices ranging from 21 to 41%. However, the index showed nutrient levels in Daffiama-Dakyea to be sufficient, with SQ values >75. It is also worth noting that the Daffiama Dakyea site was within the community a few meters away from the farmer's house. According to Vanlauwe et al. (63), such fields have high soil fertility status and are unlikely to respond to added inputs. Kihara et al. (9) classified such fields as fertile non-responsive soils.
Nutrient sufficiency quotient for all omitted nutrients were below 50% indicating P, K, Mg, S, and Micronutrients (Zn and B) to be limiting at Naaga. Nutrients with SQ of <50 require their application to soils to obtain optimum soybean growth. Foli (64) observed omitted micronutrients, Mg, P, and K nutrient solutions to have SQ values of 14–39%, specifying these nutrients to be deficient, while those above 75% had sufficient availability in the soils of northern Nigeria. The identified secondary and micronutrient (Mg, S, Zn, B) limitations correlated fairly with those of Wortmann et al. (65) and further supported the concept of balanced fertilizer application in SSA (66).
Targeting fertilizer use to variable soil fertility conditions combined with organic amendments is necessary for efficient crop response in smallholder farms in SSA (67). After the three cropping season, the combined application of FertiSoil and mineral fertilizers accounted for the highest improved grain yield at Pishegu, Serekpere, Daffiama Saapare, and Naaga (Table 6). The response of soybean yield to sole organic input or in combination with inorganic fertilizers is consistent with other studies that confirm that the soybean and maize yield increased with the integration of compost and mineral fertilizer application or sole compost application (68–70). Brempong et al. (71) reported improved synergism and synchronization between nutrient release and plant uptake upon the integrated application of organic and inorganic nutrient sources, which resulted in better crop growth and yield. Furthermore, Sileshi et al. (72) also observed through the integrated application of organic amendment and mineral fertilizer that nutrient use efficiency is enhanced resulting in higher crop grain yields. Blanchet et al. (73) also reported highest yield with organic amendment incorporation and attributed it to increasing SOC content and soil biological properties. Additionally, Adeli et al. (74) explained the effect of organic amendment on grain yield increase to improved nutrient availability for plant uptake and enhanced soil physical, chemical, and biological properties leading to improved crop yields. Generally, the improved grain yield to the applied limiting nutrients suggests that these nutrients were hindering crop response to P fertilizer on soils that were previously not responsive to it (75). This observation could be described as the Liebig-synergism where increased yield is as a result of applying most limiting nutrients and resolving deficiencies (76). Soil fertility restoration is necessary to increase agricultural production, food security, economic development, land conservation, and environmental protection (77).
The performance of each nutrient option on non-responsive soils was demonstrated by the cumulative probability curves (Figure 1) and provides the farmer with information on the outcome of maintaining or switching management practices (78).
Conclusions
Non-responsive soils in northern Ghana are widespread, which are characterized by varying nutrient levels low in SOC. The nutrient omission trial revealed P and K to be most limiting in all the soils of the three regions, followed by Mg, S, Zn, and B. In this research, application of balanced fertilization and/or organic input improved soybean grain yield. Nutrient deficiencies are the major causes of poor crop response to fertilizer inputs, which further hinder crop productivity, and addressing these limitations is one of the key mechanisms of bridging crop yield gap. This information will assist knowledge of crop response to fertilizer and implementation of fertilizer recommendation and soil fertility management strategies for optimum and sustainable crop production.
Data Availability Statement
The original contributions presented in the study are included in the article/supplementary material, further inquiries can be directed to the corresponding author.
Author Contributions
RA, RCA, AO, SA-N, and PA-A conceived the research. RA, RCA, and AO set up the experiment. RA collected and analyzed the data. RA drafted the manuscript. RCA, AO, SA-N, and PA-A edited, revised, and made significant contributions. All authors contributed to the article and approved the submitted version.
Funding
This research was funded by the Kwame Nkrumah University of Science and Technology (KNUST) through a sub-agreement with the Alliance for Green Revolution in Africa (AGRA) under the AGRA PhD. Training in Soil Science in West Africa funded by Bill and Melinda Gates Foundation (Training grant 2009 SHP 028).
Conflict of Interest
The authors declare that the research was conducted in the absence of any commercial or financial relationships that could be construed as a potential conflict of interest.
Acknowledgments
We acknowledge the co-operation of smallholder farmers across the Guinea and Sudan Savannah agro-ecological zones of Ghana during the research.
References
1. Tully K, Sullivan C, Weil R, and Sanchez P. The state of soil degradation in Sub-Saharan Africa: baselines, trajectories, and solutions. Sustainability. (2015) 7:6523–52. doi: 10.3390/su7066523
2. Olsson L, Barbosa H, Bhadwal S, Cowie A, Delusca K, Flores-Renteria D, et al. Land degradation. In: Climate Change and Land: An IPCC Special Report on Climate Change, Desertification, Land Degradation, Sustainable Land Management, Food Security, and Greenhouse Gas Fluxes in Terrestrial Ecosystems. Geneva: IPCC (2019). p. 345–436.
3. Kihara J, Tamene LD, Massawe P, and Bekunda M. Agronomic survey to assess crop yield, controlling factors and management implications: a case-study of Babati in Northern Tanzania. Nutr Cycl Agroecosyst. (2015) 102:5–16. doi: 10.1007/s10705-014-9648-3
4. Voortman RL. Micronutrients in agriculture and the world food system-future scarcity and implications (Scarcity of micronutrients in soil, food and mineral resources-background reports). Platform Agriculture, Innovation and Society (LIS) (2012).
5. Moharana PC, Sharma BM, and Biswas DR. Changes in the soil properties and availability of micronutrients after six-year application of organic and chemical fertilizers using STCR-based targeted yield equations under pearl millet-wheat cropping system. J Plant Nutr. (2017) 40:165–76. doi: 10.1080/01904167.2016.1201504
6. Vanlauwe B, Kihara J, Chivenge P, Pypers P, Coe R, and Six J. Agronomic use efficiency of N fertilizer in maize-based systems in Sub-Saharan Africa within the context of integrated soil fertility management. Plant Soil. (2011) 339:35–50. doi: 10.1007/s11104-010-0462-7
7. Vanlauwe B, Descheemaeker K, Giller KE, Huising J, Merckx R, Nziguheba G, et al. Integrated soil fertility management in Sub-Saharan Africa: unravelling local adaptation. Soil. (2015) 1:491–508. doi: 10.5194/soil-1-491-2015
8. Bai Z, Li H, Yang X, Zhou B, Shi X, Wang B, et al. The critical soil P levels for crop yield, soil fertility and environmental safety in different soil types. Plant Soil. (2019) 372:27–37. doi: 10.1007/s11104-013-1696-y
9. Kihara J, Nziguheba G, Zingore S, Coulibaly A, Esilaba A, Kabambe V, et al. Understanding variability in crop response to fertilizer and amendments in sub-Saharan Africa. Agric Ecosyst Environ. (2016) 229:1–2. doi: 10.1016/j.agee.2016.05.012
10. Zerihun A, Alemayo D, and Wolde-Meskel E. On farm yield responses of soybean [Glycine max L. (Merrill)] to fertilizer sources under different soil acidity status in Gobu Sayo district, Western Ethiopia. J Agron. (2015) 14:30–6. doi: 10.3923/ja.2015.30.36
11. Woomer PL, Huising J, Giller KE, Baijukya FB, Kantengwa S, Vanlauwe B, et al. N2Africa final report of the first phase 2009–2013. (2014). p. 138. Available online at: www.N2Africa.org
12. Nziguheba G, Tossah B, Diels J, Franke A, Aihou K, Iwuafor E, et al. Assessment of nutrient deficiencies in maize in nutrient omission trials and long-term field experiments in the West African Savanna. Plant Soil. (2009) 314:143–57. doi: 10.1007/s11104-008-9714-1
13. Njoroge S, Antonius GT, Schut AGT, Giller KE, and Zingore S. Strong spatial-temporal patterns in maize yield response to nutrient additions in African smallholder farms. Field Crops Res. (2017) 214:321–30. doi: 10.1016/j.fcr.2017.09.026
14. Keesstra S, Mol G, de Leeuw J, Okx J, de Cleen M, and Visser S. Soil-related sustainable development goals: four concepts to make land degradation neutrality and restoration work. Land. (2018) 7:133. doi: 10.3390/land7040133
15. Statistics Research Information Directorate (SRID). Agriculture in Ghana. Facts and Figures 2012. Ministry of Food and Agriculture (2016). p. 1–113.
17. Olsen SR, and Sommers LE. Phosphorus. In Page AL, Miller RH, Keeney DR, editors. Methods of Soil Analysis. Part 2. 2nd Edn. Agronomy No. 9. Madison, WI: American Society of Agronomy (1982). p. 403–30. doi: 10.2134/agronmonogr9.2.2ed.c24
18. Nelson DW, and Sommers LE. Total carbon, organic carbon, organic matter. In: Sparks DL, Page AL, Helmke PA, Loeppert RH, Soltanpour PN, Tabatabai MA, editors. Methods of Soil Analysis, Part 2 - Chemical and Microbiological Properties, 2nd Edn. Madison, WI: American Society of Agronomy, Soil Science Society America (1982). p. 539–79. doi: 10.2134/agronmonogr9.2.2ed.c29
19. Black CA. Methods of Soil Analysis. Part 1. Physical and Mineralogical Properties, Including Statistics of Measurement and Sampling. Part II. Chemical I, Microbiological Properties. Agronomy Series. Madison, WI: ASA (1986).
20. Lindsay WL, and Norvell WA. Development of a DTPA soil test for zinc, iron, manganese, and copper. Soil Sci Soc Am J. (1978) 42:421–8. doi: 10.2136/sssaj1978.03615995004200030009x
21. Bouyoucos GJ. Hydrometer method improved for making particle size analyses of soils. Agron J. (1963) 53:464–5. doi: 10.2134/agronj1962.00021962005400050028x
22. Janssen BH. A double-pot technique as a tool in plant nutrition studies. In: Van Beusichen ML, editors. Plant Nutrition-Physiology and Application. Netherlands: Kluwer Academic (1990). p. 759–63. doi: 10.1007/978-94-009-0585-6_127
23. Paradiso R, Buonomo R, De Micco G, Aronne M, Palermo G, Barbieri G, et al. Soybean cultivar selection for bioregenerative life support systems (BLSSs)–hydroponic cultivation. Adv Space Res. (2012) 50:1501–11. doi: 10.1016/j.asr.2012.07.025
24. Evans GC. The Quantitative Analysis of Plant Growth Studies in Ecology. Berkely; Los Angeles, CA: University of California Press (1972).
26. Hatfield AL. Soil test reporting a nutrient index system. Commun Soil Sci Plant Anal. (1972) 3:425–36. doi: 10.1080/00103627209366397
27. Van Soest PJ, and Wine RH. Determination of lignin and cellulose in acid-detergent fiber with permanganate. J Assoc Offic Anal Chemists. (1968) 51:780–5. doi: 10.1093/jaoac/51.4.780
28. Constantinides M, and Fownes JH. Nitrogen mineralization from leaves and litter of tropical plants: relationship to nitrogen, lignin and soluble polyphenol concentrations. Soil Biol Biochem. (1994) 26:49–55. doi: 10.1016/0038-0717(94)90194-5
29. Ronner E, Franke A, Vanlauwe B, Dianda M, Edeh E, Ukem B, et al. Understanding variability in soybean yield and response to P-fertilizer and rhizobium inoculants on farmers' fields in northern Nigeria. Field Crops Res. (2016) 186:133–45. doi: 10.1016/j.fcr.2015.10.023
30. Staton M. Phosphorus and Potassium Fertilizer Recommendations for High-Yielding, Profitable Soybeans. Michigan State University Extension (2014).
31. Ministry of Food Agriculture (MOFA). Investment Guide for the Agriculture Sector in Ghana. Ministry of Food and Agriculture (2018). p. 1–80.
32. Alliance for a Green Revolution in Africa (AGRA). Africa agriculture status report: climate change and smallholder agriculture in Sub-Saharan Africa (Africa agriculture status reports). Nairobi: AGRA (2014).
33. Boakye-Danquah J, Antwi EK, Saito O, Abekoe MK, and Takeuchi K. Impact of farm management practices and agricultural land use on soil organic carbon storage potential in the Savannah ecological zone of Northern Ghana. J Disaster Res. (2014) 9:1–18. doi: 10.20965/jdr.2014.p0484
34. Kombiok JM, Buah SSJ, and Sogbedji JM. Enhancing soil fertility for cereal crop production through biological practices and the integration of organic and in-organic fertilizers in northern savanna zone of Ghana. Soil Fertility. (2012) 1:1–30. doi: 10.5772/53414
35. Bagamsah TT. The Impact of Bushfire on Carbon and Nutrient Stocks as well as Albedo in the Savanna of Northern Ghana (Vol. 25). Göttingen: Cuvillier Verlag (2005).
36. Fermont AM, Tittonell PA, Baguma Y, Ntawuruhunga P, and Giller KE. Towards understanding factors that govern fertilizer response in cassava: lessons from East Africa. Nutr Cycl Agroecosyst. (2010) 86:133–51. doi: 10.1007/s10705-009-9278-3
37. Antwi M, Duker AA, Fosu M, and Abaidoo RC. Geospatial approach to study the spatial distribution of major soil nutrients in the Northern region of Ghana. Cogent Geosci. (2016) 2:1–13. doi: 10.1080/23312041.2016.1201906
38. Asei R, Ewusi-Mensah N, and Abaidoo RC. Response of soybean (Glycine max L.) to rhizobia inoculation and molybdenum application in the northern savannah zones of Ghana. J Plant Sci. (2015) 3:64–70. doi: 10.11648/j.jps.20150302.14
39. Tetteh FM, Ennim SA, Issaka RN, Buri M, Ahiabor BAK, and Fening JO. Chapter 10 fertilizer recommendation for maize and cassava within the breadbasket zone of Ghana. In: Bationo A, Ngaradoum D, Youl S, Lompo F, Fening JO, editors. Improving the Profitability, Sustainability and Efficiency of Nutrients Through Site Specific Fertilizer Recommendations in West Africa Agro-ecosystems. Cham: Springer (2018). p. 161–84. doi: 10.1007/978-3-319-58792-9_10
40. Quesada CA, Lloyd J, Schwarz M, Patiño S, Baker TR, Czimczik C, et al. Variations in chemical and physical properties of Amazon forest soils in relation to their genesis. Biogeosciences. (2010) 7:1515–41. doi: 10.5194/bg-7-1515-2010
41. Chibwana C, Fisher M, and Shively G. Cropland allocation effects of agricultural input subsidies in Malawi. World Dev. (2012) 40:124–33. doi: 10.1016/j.worlddev.2011.04.022
42. Jayne T, Kolavalli S, Debrah K, Ariga J, Brunache P, Kabaghe C, et al. Towards a Sustainable Soil Fertility Strategy in Ghana (Feed the future innovation lab for food security policy research papers 258733) Michigan State University, Department of Agricultural, Food, Resource Economics, Feed the Future Innovation Lab for Food Security (FSP). (2015). doi: 10.22004/ag.econ.258733
43. Dowuona GNN, Atwere P, Dubbin W, Nude PM, Mutala BE, Nartey EK, et al. Characteristics of termite mounds and associated acrisols in the coastal savannah zone of Ghana and impact on hydraulic conductivity. Natur Sci. (2012) 4:423–37. doi: 10.4236/ns.2012.47058
44. Lal R. Soil carbon sequestration impacts on global climate change and food security. Science. (2004) 304:1623–7. doi: 10.1126/science.1097396
45. Musinguzi P, Ebanyat P, Tenywa JS, Basamba TA, Tenywa MM, and Mubiru DN. Critical soil organic carbon range for optimal crop response to mineral fertiliser nitrogen on a ferralsol. Exp Agric. (2016) 52:635–53. doi: 10.1017/S0014479715000307
46. Renck A, and Lehmann J. Rapid water flow and transport of inorganic and organic nitrogen in a highly aggregated tropical soil. Soil Sci. (2004) 169:330–41. doi: 10.1097/01.ss.0000128016.00021.3d
47. Benbi DK, and Brar SPS. Influence of soil organic carbon on the interpretation of soil test P for wheat grown on alkaline soils. Fert Res. (1994) 37:35–41. doi: 10.1007/BF00750671
48. Bationo A, Kihara J, Vanlauwe B, Waswa B, and Kimetu J. Soil organic carbon dynamics, functions and management in West African agro-ecosystems. Agric Syst. (2007) 94:13–25. doi: 10.1016/j.agsy.2005.08.011
49. Tetteh F, Larbi A, Nketia KA, Senayah JF, Hoeschle-Zeledon I, and Abdulrahman N. Suitability of soils for cereal cropping in Northern Ghana (International Institute of Tropical Agriculture (IITA) Report). Ibadan: IITA (2016). p. 19.
50. Vanlauwe B, Bationo A, Chianu J, Giller KE, and Merckx R. Integrated soil fertility management: operational definition and consequences for implementation and dissemination. Outlook Agric. (2010) 39:17–24. doi: 10.5367/000000010791169998
51. Awuni GA, and Reynolds DB. Soybean management with appropriate research and technology (SMART) northern ghana (Soybean Innovation Laboratory (SIL) Value Chain Research Management Research Area (MRA) 3. Feed the Future Soybean Innovation Laboratory (SIL) MRA. 3: Soybean Production and Agronomy. Year 3 Report). Starkville, MS: Mississippi State University (2016). p. 1–41.
52. Oluwadare DA, Voncir N, Mustapha S, and Mohammed GU. Evaluation and enhancement of available micronutrients status of cultivated soil of Nigeria guinea savanna using organic and inorganic amendments. IOSR J Agric Veterinary Sci. (2013) 3:62–8. doi: 10.9790/2380-0356268
53. Boateng E, Gyekye PM, Oppong J, and Akuffo E. Soil and land suitably assessment for agricultural project at Babator, near Bamboi, Northern Region, Ghana (CSIR-SRI, Annual Report). CSIR-SRI (2013). p. 2–8.
54. Dimkpa CO, and Bindraban PS. Fortification of micronutrients for efficient agronomic production: a review. Agron Sustain Dev. (2016) 36:7. doi: 10.1007/s13593-015-0346-6
55. Ch'ng HY, Ahmed OH, and Majid NM. Improving phosphorus availability in an acid soil using organic amendments produced from agroindustrial wastes. Sci World J. (2014) 2014:1–6. doi: 10.1155/2014/506356
56. Miller JO. Soil pH Affects Nutrient Availability. Fact Sheet FS-1054. College Park, MD: University of Maryland, Extension Solutions (2016).p. 1–5.
57. McCauley A, Jones C, and Olson-Rutz K. Soil pH and Organic Matter. Nutrient Management Module No. 8. Bozeman, MT: Montana State University-Bozeman (2017).
58. Bakhshandeh S, Norouzi M, Heidari S, and Bakhshandeh S. The role of parent material on soil properties in sloping areas under tea plantation in Lahijan, Iran. Carpath J Earth Environ Sci. (2014) 9:159–70.
59. Ziblim M, Okai-Anti D, and Asmah EA. Productivity index rating of some soils in the Tolon-Kumbungu district of the Northern region of Ghana. J Soil Sci Environ Manage. (2012) 3:154–63. doi: 10.5897/JSSEM11.124
60. MacCarthy DS, Agyare WA, and Vlek PLG. Evaluation of soil properties of the sudan savannah ecological zone of Ghana for crop production. Ghana J Agric Sci. (2018) 52:95–104.
61. Runge-Metzger A, and Diehl L. Farm household systems in Northern Ghana (Nyankpala Agricultural Research Report, 9). Eschborn: GTZ (1993).
62. Zheng Z, Parent LE, and MacLeod JA. Influence of soil texture on fertilizer and soil phosphorus transformations in gleysolic soils. Can J Soil Sci. (2003) 83:395–403. doi: 10.4141/S02-073
63. Vanlauwe B, Tittonell P, and Mukalama J. Within-farm soil fertility gradients affect response of maize to fertiliser application in Western Kenya. In: Bationo A, Waswa B, Kihara J, Kimetu J, editors. Advances in Integrated Soil Fertility Management in Sub-Saharan Africa: Challenges and Opportunities. Dordrecht: Springer (2007). p. 121–32. doi: 10.1007/978-1-4020-5760-1_10
64. Foli SK. Qualitative and quantitative diagnosis of macro and micronutrient deficiencies in soils across three agro-ecological environments of northern Nigeria using the double-pot technique (MSc. Thesis). Wageningen: Plant Production Systems, Wageningen University (2012).
65. Wortmann CS, Kaizzi KC, Maman N, Cyamweshi A, Dicko M, Garba M, et al. Diagnosis of crop secondary and micronutrient deficiencies in Sub-Saharan Africa. Nutr Cycl Agroecosyst. (2019) 113:127–40. doi: 10.1007/s10705-018-09968-7
66. Chianu JN, Chianu JN, and Mairura F. Mineral fertilizers in the farming systems of Sub-Saharan Africa. A review. Agron Sustain Dev. (2012) 32:545–66. doi: 10.1007/s13593-011-0050-0
67. Giller KE, Rowe EC, de Ridder N, and van Keulen. H. Resource use dynamics and interactions in the tropics: scaling up in space and time. Agric Syst. (2006) 88:8–27. doi: 10.1016/j.agsy.2005.06.016
68. Buah SSJ, Ibrahim H, Derigubah M, Kuzie M, Segtaa JV, Bayala J, et al. Tillage and fertilizer effect on maize and soybean yields in the guinea savanna zone of ghana. Agric Food Secur. (2017) 6:1–11. doi: 10.1186/s40066-017-0094-8
69. Adebayo AR, Akanbi WB, Olaniyi JO, and Kutu FR. Growth and yield attributes of soybean (Glycine max L.) in response to cassava peel compost and inorganic fertilizer. Res Crops. (2018) 18:618–26. doi: 10.5958/2348-7542.2017.00104.8
70. Bello WB, Adejuyigbe CO, Adigun JA, and Dare MO. Soil fertility status, nutrient uptake and maize (Zea mays L.) yield as influenced by animal manure and compost. J Org Agric Environ. (2020) 7:9–16.
71. Brempong MB, Opoku A, Ewusi-Mensah N, and Abaidoo RC. Evaluating added benefits from combined cattle manure and fertilizer application in a maize cropping system. J Environ Sci Eng B. (2017) 6:34–40. doi: 10.17265/2162-5263/2017.01.004
72. Sileshi GW, Jama B, Vanlauwe B, Negassa W, Harawa R, Kiwia A, et al. Nutrient use efficiency and crop yield response to the combined application of cattle manure and inorganic fertilizer in Sub-Saharan Africa. Nutr Cycl Agroecosyst. (2019) 113:181–99. doi: 10.1007/s10705-019-09974-3
73. Blanchet G, Gavazov K, Bragazz L, and Sinaj S. Responses of soil properties and crop yields to different inorganic and organic amendments in a Swiss conventional farming system. Agric Ecosyst Environ. (2016) 230:116–26. doi: 10.1016/j.agee.2016.05.032
74. Adeli A, Sistani KR, Tewolde H, and Rowe DE. Effects of broiler litter applied to no-till and tillage cotton on selected soil properties. Soil Sci Soc Am J. (2007) 71:974–83. doi: 10.2136/sssaj2006.0092
75. Kihara J, Sileshi GW, Nziguheba G, Kinyua M, Zingore S, and Sommer R. Application of secondary nutrients and micronutrients increases crop yields in sub-Saharan Africa. Agron Sustain Dev. (2017) 37:1–14. doi: 10.1007/s13593-017-0431-0
76. Rietra RPJJ, Heinena M, Dimkpab CO, and Bindraban PS. Effects of nutrient antagonism and synergism on yield and fertilizer use. Commun Soil Sci Plant Anal. (2017) 48:1895–920. doi: 10.1080/00103624.2017.1407429
77. Henao J, and Baanante C. Agricultural production and soil nutrient mining in africa: implications for resource conservation and policy development. IFDC Tech Bull. (2006) 1–13.
Keywords: non-responsive soils, site-specific, nutrient sufficiency quotient, fertility, limiting nutrients
Citation: Asei R, Abaidoo RC, Opoku A, Adjei-Nsiah S and Antwi-Agyei P (2021) Use of Limiting Nutrients for Reclamation of Non-responsive Soils in Northern Ghana. Front. Soil Sci. 1:674320. doi: 10.3389/fsoil.2021.674320
Received: 19 March 2021; Accepted: 21 May 2021;
Published: 05 July 2021.
Edited by:
Sudarshan Kumar Dutta, Mohammed VI Polytechnic University, MoroccoReviewed by:
Krishnendu Ray, Ramakrishna Mission Vivekananda Educational and Research Institute, IndiaArtemi Cerdà, University of Valencia, Spain
Copyright © 2021 Asei, Abaidoo, Opoku, Adjei-Nsiah and Antwi-Agyei. This is an open-access article distributed under the terms of the Creative Commons Attribution License (CC BY). The use, distribution or reproduction in other forums is permitted, provided the original author(s) and the copyright owner(s) are credited and that the original publication in this journal is cited, in accordance with accepted academic practice. No use, distribution or reproduction is permitted which does not comply with these terms.
*Correspondence: Rechiatu Asei, cmVjaGlhYXNlaUB5YWhvby5jb20=; orcid.org/0000-0002-7315-9659