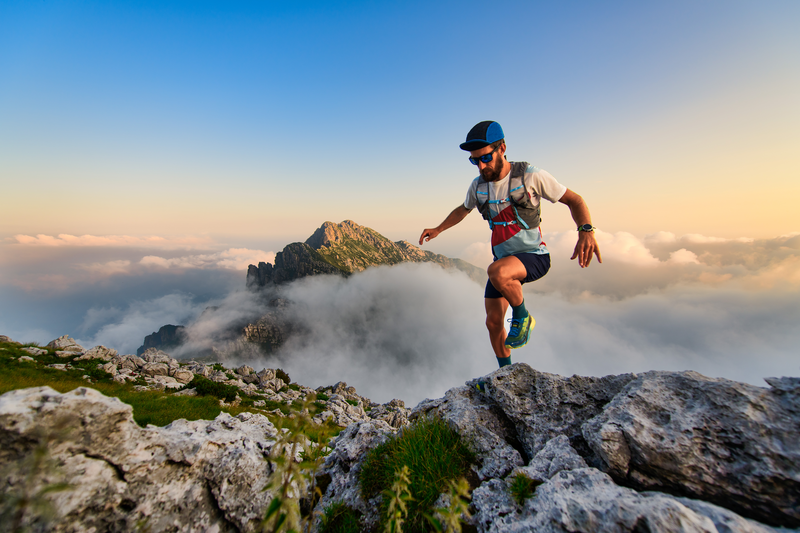
94% of researchers rate our articles as excellent or good
Learn more about the work of our research integrity team to safeguard the quality of each article we publish.
Find out more
MINI REVIEW article
Front. Soft Matter , 02 November 2023
Sec. Polymers
Volume 3 - 2023 | https://doi.org/10.3389/frsfm.2023.1253929
Polymer materials play an important role in various aspects of our lives. Most of them are solid, therefore, the key importance of such polymeric materials is related to their versatility, lightness, economy, durability and strength, corrosion resistance, electrical and thermal insulation (except conductive polymers), appropriate interface between the body and medical devices, and as well as design flexibility. In general, the importance of polymeric materials lies in their versatile properties, cost-effectiveness, and applicability in a variety of industries, including automotive, aerospace, electronics, healthcare, construction, packaging, and more. The purpose of this mini-review is to encourage future authors to submit outstanding contributions related to various aspects and frontiers of polymeric materials in the years to come.
Polymer materials—frequently named plastics, what mean “pliable and easily shaped,” became integrated part of our everyday life. The first synthesis of polymer material, from cellulose and camphor, was done by John Wesley Hyatt in 1869 in order to replace natural ivory for, e.g., preparation of billiard balls. The first fully synthetic polymer material was prepared in 1907 by Leo Baekeland in order to find a replacement for shellac, a natural resin, in electrical insulators and other industrial applications. Bakelite had a significant impact on various industries due to its versatility, durability, and heat-resistant properties. These preliminary discoveries were oriented on polymer materials with specific properties. However, the further developments were oriented on understanding of macromolecular synthesis, structure–property relationship of polymer materials, polymer materials physics, structure, processing and recently on characterization of advanced polymer materials at the molecular level. The polymer materials development during last 100 years was recognized by several Nobel Prices like, e.g., in 1953 to Hermann Staudinger for his pioneering insights into the structure and behavior of macromolecules, in 1963 to Karl Ziegler and Giulio Natta for their independent contributions to the development of the Ziegler-Natta catalyst which revolutionized the field of polymer chemistry, in 2000 to Alan J. Heeger, Alan G. MacDiarmid and Hideki Shirakawa for the discovery and development of conductive polymers, and in 2005 to Robert H. Grubbs for development of the metathesis method in the synthesis of organic molecules.
Polymer synthesis with precise control over the arrangement and sequence of monomer units, resulting in desired polymer structures with tailored properties. Control over polymer structure empowers scientists, engineers, and manufacturers to create materials that address specific needs, improve performance, and contribute to sustainable and innovative solutions across industries. The pioneering development of synthetic approaches for polymer materials by discovering “living polymerization” was done by Michael Szwarc. This Polish origin chemist has done outstanding research in the polymer science which provided a basis for the precise synthesis of polymer materials. Szwarc published in the year 1956 an anionic polymerization of styrene with a sodium naphtalenide under meticulously controlled reaction conditions which enables to maintain chain-end reactivity even after the completion of the polymerization reaction (Szwarc, 1956). This approach allows the precise control of molar mass and polymer dispersity. His contribution has made possible the synthesis of a wide range of functional (co)polymer materials, and was further extended on ring-opening polymerization of some oxacyclic monomers (Jedliński et al., 1991; Adamus et al., 2021).
Krzysztof Matyjaszewski and his group have focused on the development and use of copper based catalysts for Atom Transfer Radical Polymerizations (ATRP) conducted with a halogen as the transferable atom (Wang and Matyjaszewski, 1995). This kind of controlled radical polymerization (CRP) is probably the most robust and efficient, and is currently widely used for preparation of well-defined polymer materials with controlled topology, composition and specific functionality (Szewczyk-Łagodzińska et al., 2023).
The another CRP approach, the reversible addition−fragmentation chain transfer (RAFT) polymerization, was discovered at the Commonwealth Scientific and Industrial Research Organisation (CSIRO) of Australia in 1998 (Cacioli et al., 1986; Chiefari et al., 1998). RAFT process is consisting with a series of reversible addition-fragmentation steps based on the chain transfer converting dormant chains to active propagating radicals. In contrast to ATRP a RAFT polymerization enables controlled progress of molar mass and low dispersity by polymer chains growing at approximately the same time and equal growth of polymer molar mass during polymerization (Semsarilar and Abetz, 2021).
In the year 2022 Barry Sharpless was recognized, among others by his second Nobel Prize in Chemistry, for the contribution to easy synthesis by “Click Chemistry,” which constitutes the convergence of synthetic organic and polymer chemistries (Barry Sharpless, 2023). He introduced the term “Click Chemistry” in 2001 to describe highly efficient and selective reactions that based on carbon-hetero bond formations, and which have the impact on facile approaches to macromolecular synthesis and polymer modification (Kolb et al., 2001). The “click”-based methodologies in polymer science are still the subjects of intensive studies directed toward a major focus on “living polymerization” methods (Devaraj and Finn, 2021).
The above mentioned mile stones in synthesis of polymer materials, recognized mostly by Nobel Prices, constitute only the examples of tremendous discoveries which poses major challenges and opportunities for well-defined synthetic methods of macromolecules. The expectation that polymer materials will exhibit distinctive properties and functions should be however balanced with the social responsibility of their development.
At present, we are only at the beginning of the clean-up after what happened as a result of the turbulent development of classic plastics. The aim of these activities is to identify opportunities and constraints related to future sustainable polymer synthesis and to assess the feasibility of implementing their production in bio-refineries. In order to reduce irreversible threats to the environment, it is important to strive for multiple use of polymeric materials, their economical use as well as to increase the share of renewable sources in obtaining raw materials for their production. Reducing the amount of packaging waste and promoting the circular economy are current challenges. This applies to the entire life cycle of polymer materials, from design and production to consumption, repair, re-use, recycling and putting resources back into the economy.
The ISO 26000:2010 standard contains guidelines on the basic principles of social responsibility, stakeholder engagement, as well as key areas and issues related to it (Social responsibility - Discovering ISO 26000, 2010). In the field of environment, this standard defines social responsibility as the impact of decisions and actions taken on pollution prevention, sustainable use of resources, mitigation and adaptation to climate change, environmental protection, biodiversity and restoration of natural habitats. The European strategy for plastics focuses on adapting EU regulations to the implementation of the principles of the Circular Economy (CE). The list of measures to implement the EU’s Circular Plastics Strategy includes, inter alia, actions in the field of biodegradable and compostable plastics. They concern in particular:
• preparation of harmonized legislation on the definition and labeling of compostable and biodegradable plastics;
• carrying out a life cycle assessment to determine the conditions under which the use of such plastics is beneficial, as well as the criteria for their use;
• starting the process of eliminating polymer materials susceptible to oxidative degradation.
The growing demand for biodegradable polymer materials creates new opportunities, but also challenges. Thus, the European Commission has presented a proposal for a regulation on packaging and packaging waste (PPWR), which will repeal the applicable Directive on packaging and packaging waste (PPWD) and will harmonize the system of packaging waste throughout the EU. Article 8 defines the conditions for recognizing packaging as compostable. This provision also empowers the Commission to adopt delegated acts to amend the list of packaging that must be compostable (Revision of the packaging and packaging waste Directive, 2023). Therefore, it is extremely important to design such packaging materials from bioplastics that would be safe for human health and the environment, and at the same time to responsibly and sustainably indicate new areas where their unique properties could often be used (Kowalczuk, 2016).
Due to the wide spectrum of potential applications of bioplastics (Figure 1), e.g., in medicine, for the production of compostable polymer packaging (such as cosmetics or food), as well as in agrochemical preparations, forensic engineering of advanced polymer materials (FEAPM) can provide basic knowledge and valuable information to prevent future problems. FEAPM is concerned with understanding and evaluating the relationships between the structure, properties and behavior of polymer materials before, during and after practical applications (Kowalczuk, 2017; Samir et al., 2022). Furthermore, in the case of bio-plastics, it is important to understand their structure at the molecular level and to use a polymer material that, having a compostable product certificate, will at the same time retains its functional properties for the assumed period of time.
Figure 1. A new generation of packages, filaments, 3D printed and injected moulded items, prepared from biodegradable polymer materials under POIG.01.03.01-00-018/08 and GREEN-MAP projects.
The understanding of polymer materials structure at the molecular level requires appropriate analytical techniques. The development of soft ionization techniques in mass spectrometry (MS) enables sequence analysis of natural and synthetic macromolecules. It permits the production of gas-phase ions from a wide variety of polymers, with little or no fragmentation during ionization. MS sequence analysis of synthetic macromolecules can be helpful for the specific area of applied research on polymer materials. Multistage mass spectrometry (MSn) enables the structural analysis of mass selected macromolecular ions of (co)polymers and determination of the chemical structure of the end groups (Kowalczuk and Adamus, 2016). Reviewed recently, further developments of polymer MS techniques, facilitate the elucidation of specific structural features in a synthetic polymer, including compositional complexity, primary structure, architecture, topology, and surface properties (Wesdemiotis et al., 2023).
By integrating social responsibility into the production, use, and disposal of polymer materials, it is possible to minimize negative externalities and promote sustainable practices throughout the value chain. It is important for businesses, policymakers, and consumers to recognize the importance of social responsibility when dealing with polymer materials and actively work towards solutions that balance their benefits with environmental and social considerations. Manufacturers and producers of polymer materials can prioritize the use of sustainable raw materials, such as bio-based or recycled polymers, reducing the reliance on fossil fuels and minimizing environmental impacts. Adopting cleaner production processes, minimizing energy consumption, reducing emissions, and implementing waste management strategies are essential for mitigating the environmental footprint associated with polymer manufacturing. Designing polymer products with a focus on durability, reusability, and recyclability can contribute to their responsible use. By considering end-of-life scenarios during the design phase, manufacturers can minimize waste generation and maximize resources efficiency.
Prospects for the development of polymer materials are currently very promising. An increasing number of consumers and governments are beginning to realize the need to reduce the amount of waste, especially plastic, generated by our civilization. As a result, interest in alternative materials such as biodegradable polymers is growing.
There are many types of biodegradable polymer materials on the market today, including polylactide, polyhydroxyalkanoates, lactic acid copolymers, synthetic polybutadienes and many others. Each of these polymers has its own unique properties that allow them to be used in various applications, including packaging, medicine, agriculture and the textile industry. One of the main criteria facing the polymer industry is to develop biodegradable polymer materials that will have a good strength-to-weight ratio and could compete with conventional polymers for the cost of production. Another challenge is the improvement of processing and composting of biodegradable polymer materials to take advantage of the environmental impacts and to minimize the negative effect on the environment that may result from improper disposal. Despite these challenges, the prospects for the development of biodegradable polymer materials are still very productive, many companies are investing in research and development of this technology. It is expected that over the next few years the production and use of biodegradable polymer materials will increase, which will have an impact on our environment (Backer and Leal, 2022).
The development of smart and functional polymers is opening up new possibilities in areas like electronics, sensors, and drug delivery systems. These polymers can respond to external stimuli, such as temperature, pH, light, or electrical signals, enabling them to exhibit specific behaviours or properties. Smart polymers have applications in fields such as smart textiles, controlled drug release, and self-healing materials. Additive manufacturing, also known as 3D printing, is revolutionizing the way polymer materials are used and produced. This technology allows for the rapid and customized fabrication of complex polymer structures with precise control over their geometry and properties. Additive manufacturing enables the production of highly customized products, reduces material waste, and promotes design freedom. Nature-inspired or bioinspired polymers are another area of active research. Scientists are studying natural materials, such as spider silk, mussel adhesives, and lotus leaves, to understand their unique properties and mimic them in synthetic polymers. Bioinspired polymers have the potential to offer new functionalities, such as self-cleaning surfaces, adhesives, and biomedical materials. While polymer materials face challenges related to environmental impact, plastic waste, performance limitations, health and safety concerns, there is hope in the development of sustainable alternatives, improved recycling technologies, and enhanced material properties.
Recently, studies have been carried out on the basis of research on molecular labelling of polymer materials for coding information inside the polymer structure (i.e., at the molecular level). The development of a method for labelling polymer materials will enable their identification based on the molecular recording of information in the polymer structure and their reading using, for example, mass spectrometry (König et al., 2019; Samokhvalova and Lutz, 2023). Basic research conducted with the use of the most modern analytical techniques will allow to read the information from the molecular structure of monodisperse copolymer markers by the so-called sequencing. The combination of these data with physicochemical tests will fill the gap in the existing forensic knowledge and will allow for the design and modification of new, safe, and at the same time biodegradable polymeric materials for advanced applications.
In conclusion, the prospects for the development of polymer materials are vast and exciting. Advancements in performance, sustainability, nanocomposites, smart functionalities, additive manufacturing, recycling, and bioinspired materials are driving the evolution of polymer science and opening up new opportunities in various industries. Embracing the boundless potential of polymer materials by delving into their intricate structures and properties, will pave the way for transformative innovations that will reshape industries and enhance everyday lives. Our contributions to polymer science hold the key to unlocking novel materials with unprecedented functionalities, ushering in a future where imagination is the only limit.
MK: Writing–original draft, Conceptualization, Writing–review and editing.
This research was partly supported by the European Regional Development Fund: Contract no. POIG.01.03.01-00-018/08 “New generation packaging materials made from plastics subject to the organic recycling” in the framework of the Innovative Economy Operational Programme (IE OP), the European Union’s Horizon 2020 research and innovation programme under the Marie Skłodowska-Curie grant agreement No. 872152, project GREEN-MAP and an international project co-financed by the program of the Polish Minister of Science and Higher Education entitled “PMW” in the years 2020–2023; contract No. 5092/H2020/2020/2.
This article is a personal acknowledgement to Dipl.-Ing. Dr. W. Sikorska for the photo provided.
The author declares that the research was conducted in the absence of any commercial or financial relationships that could be construed as a potential conflict of interest.
The author MK declared that he was an editorial board member of Frontiers, at the time of submission. This had no impact on the peer review process and the final decision.
All claims expressed in this article are solely those of the authors and do not necessarily represent those of their affiliated organizations, or those of the publisher, the editors and the reviewers. Any product that may be evaluated in this article, or claim that may be made by its manufacturer, is not guaranteed or endorsed by the publisher.
Adamus, A., Domiński, A., Kowalczuk, M., Kurcok, P., and Radecka, I. (2021). From anionic ring-opening polymerization of β-butyrolactone to biodegradable poly (hydroxyalkanoate)s: our contributions in this field. Polymers 13 (24), 4365. doi:10.3390/polym13244365
Backer, S. A., and Leal, L. (2022). Biodegradability as an off-ramp for the Circular Economy: investigations into biodegradable polymers for home and personal care. Acc. Chem. Res. 55, 2011–2018. doi:10.1021/acs.accounts.2c00336
Barry Sharpless, K. (2023). Nobel prize lecture. NobelPrize.org. Nobel prize outreach AB 2023. Wed. 24 May. Available at: https://www.nobelprize.org/prizes/chemistry/2022/sharpless/lecture/ (Accessed May 29, 2023).
Cacioli, P., Hawthorne, D. G., Laslett, R. L., Rizzardo, E., and Solomen, D. H. (1986). Copolymerization of ω-unsaturated oligo(methyl methacrylate): new macromonomers. J. Macromol. Sci. A 23 (7), 839–852. doi:10.1080/00222338608069476
Chiefari, J., Chong, Y. K., Ercole, F. J., Jeffery, K. J., Le, T. P. T., Mayadunne, R. T. A., et al. (1998). Living free-radical polymerization by reversible Addition−Fragmentation chain transfer: the RAFT process. Macromolecules 31 (16), 5559–5562. doi:10.1021/ma9804951
Devaraj, N. K., and Finn, M. G. (2021). Introduction: click chemistry. Chem. Rev. 121 (12), 6697–6698. doi:10.1021/acs.chemrev.1c00469
Jedliński, Z., Kowalczuk, M., Glowkowski, W., Grobelny, J., and Szwarc, M. (1991). Novel polymerization of β-butyrolactone initiated by potassium naphthalenide in the presence of a crown ether or a cryptand. Macromolecules 24, 349–352. doi:10.1021/ma00002a002
Kolb, H. C., Finn, M. G., and Barry Sharpless, K. (2001). Click chemistry: diverse chemical function from a few good reactions. Angew. Chem. Int. Ed. 40 (11), 2004–2021. doi:10.1002/1521-3773(20010601)40:11<2004::AID-ANIE2004>3.0.CO;2-5
König, N. F., Al Ouahabi, A., Oswald, L., Szweda, R., Charles, L., and Lutz, J.-F. (2019). Photo-editable macromolecular information. Nat. Commun. 10, 3774. doi:10.1038/s41467-019-11566-2
Kowalczuk, M. (2016). New vistas in mass spectrometry for sequence analysis of natural and synthetic biodegradable macromolecules. Chim. Oggi – Chem. Today 34, 12.
Kowalczuk, M., and Adamus, G. (2016). Mass spectrometry for the elucidation of the subtle molecular structure of biodegradable polymers and their degradation products. Mass Spectrom. Rev. 35, 188–198. doi:10.1002/mas.21474
Kowalczuk, M. M. (2017). Forensic engineering of advanced polymeric materials. M. J. Foren. 1 (1), e001.
Revision of the packaging and packaging waste Directive (2023). Briefing EU legislation in progress. Available at: https://www.europarl.europa.eu/RegData/etudes/BRIE/2023/745707/EPRS_BRI(2023)745707_EN.pdf (Accessed May 29, 2023).
Samir, A., Ashour, F. H., Hakim, A. A. A., and Bassyouni, M. O. (2022). Recent advances in biodegradable polymers for sustainable applications. npj Mater. Degrad. 6, 68. doi:10.1038/s41529-022-00277-7
Samokhvalova, S., and Lutz, J.-F. (2023). Macromolecular information transfer. Angew. Chem. Int. Ed. 62, e202300014. doi:10.1002/anie.202300014
Semsarilar, M., and Abetz, V. (2021). Polymerizations by RAFT: developments of the technique and its application in the synthesis of tailored (co)polymers. Macromol. Chem. Phys. 222 (1), 2000311. doi:10.1002/macp.202000311
Social responsibility - Discovering ISO 26000 (2010). Social responsibility - discovering ISO 26000. Available at: https://www.iso.org/publication/PUB100258.html (Accessed May 29, 2023).
Szewczyk-Łagodzińska, M., Plichta, A., Dębowski, M., Kowalczyk, S., Iuliano, A., and Florjańczyk, Z. (2023). Recent advances in the application of ATRP in the synthesis of drug delivery systems. Polymers 15, 1234. doi:10.3390/polym15051234
Wang, J.-S., and Matyjaszewski, K. (1995). Controlled/"living" radical polymerization. Atom Transfer Radical Polymerization in the presence of transition-metal complexes. J. Am. Chem. Soc. 117 (20), 5614–5615. doi:10.1021/ja00125a035
Keywords: macromolecular synthesis, structure-property relationship of polymer materials, biodegradable and functional polymer materials, sequence analysis of synthetic macromolecules, forensic engineering of advanced polymer materials
Citation: Kowalczuk M (2023) Polymer materials—challenges and hope. Front. Soft Matter 3:1253929. doi: 10.3389/frsfm.2023.1253929
Received: 06 July 2023; Accepted: 05 October 2023;
Published: 02 November 2023.
Edited by:
Jerzy Silberring, AGH University of Science and Technology, PolandReviewed by:
Salvatore Sortino, University of Catania, ItalyCopyright © 2023 Kowalczuk. This is an open-access article distributed under the terms of the Creative Commons Attribution License (CC BY). The use, distribution or reproduction in other forums is permitted, provided the original author(s) and the copyright owner(s) are credited and that the original publication in this journal is cited, in accordance with accepted academic practice. No use, distribution or reproduction is permitted which does not comply with these terms.
*Correspondence: Marek Kowalczuk, bWtvd2FsY3p1a0BjbXB3LXBhbi5wbA==
Disclaimer: All claims expressed in this article are solely those of the authors and do not necessarily represent those of their affiliated organizations, or those of the publisher, the editors and the reviewers. Any product that may be evaluated in this article or claim that may be made by its manufacturer is not guaranteed or endorsed by the publisher.
Research integrity at Frontiers
Learn more about the work of our research integrity team to safeguard the quality of each article we publish.