- 1Department of Respiratory and Sleep Medicine, Royal North Shore Hospital, Sydney, NSW, Australia
- 2Charles Perkins Centre, Faculty of Medicine and Health, University of Sydney, Sydney, NSW, Australia
Mandibular advancement splint (MAS) therapy emerged as an effective therapy for obstructive sleep apnoea (OSA) in the mid 1990s, and is now the leading treatment alternative for OSA. Since its inception, the field has seen a suite of revisions and advances in relation to design and customisation, fabrication, titration methods, response prediction models and the integration of data collection technology. This paper reviews these current and emerging innovations in MAS therapy and their impact upon sleep apnoea management.
1. Background: Mechanism of action and treatment response
MAS is an oral appliance which protrudes the mandible in relation to the maxilla, causing movement of soft tissues (tongue and soft palate) to increase the calibre of the upper airway and reduce its collapsibility. Although continuous positive airway pressure (CPAP) therapy is more effective than MAS at lowering the apnoea hypopnoea index (AHI) (Luz et al., 2022), CPAP acceptance and compliance rates may be low, leading to reduced overall efficacy in eliminating the burden of OSA (Grote et al., 2000; Schwartz et al., 2018). MAS therapy improves blood pressure, daytime somnolence, driving risk and quality of life to the same extent as CPAP, including in patients with severe OSA (Lim et al., 2006; Phillips et al., 2013). Therefore, it is hypothesised that the reduced efficacy of MAS when compared to CPAP may be offset by improved patient tolerance and adherence with MAS therapy (Schwartz et al., 2018), leading to similar benefits in neuro-behavioural and cardiovascular outcomes. However, one of the key barriers to wider uptake has been the variability of patient response, since up to 70% of patients will experience a partial or complete treatment response (Sutherland et al., 2015), leaving around 30% without a beneficial therapeutic outcome. In addition, since a MAS device relies on dental adherence in order to remain in situ, patients with inadequate dentition are ineligible and have been excluded from research studies. Prediction tools for a favourable MAS treatment response are an area of ongoing research.
2. Patient selection and prediction of response: Endotypes
Traditionally, demographic and anthropometric characteristics have been explored as markers of MAS treatment response. For example, younger age, less obesity, female gender, milder OSA, and supine-dependent OSA have variously been associated with treatment success, though these have all been weakly predictive (Sutherland et al., 2015). Endotypic profiling has been gaining recognition in Sleep Medicine generally as a means by which to advance precision medicine for OSA patients, and can be applied to MAS treatment response.
An endotype refers to a disease subtype with a distinct functional or patho-biological mechanism (Edwards et al., 2019). OSA endotypes include: arousal threshold (degree of ventilatory drive required to trigger an arousal from sleep), loop gain (instability in ventilatory control in response to a disturbance), pharyngeal collapsibility and compensatory airway muscle responsiveness. Traditionally, these endotypic traits have been determined in a highly controlled research laboratory setting using invasive measurement techniques (Edwards et al., 2016; Bamagoos et al., 2019b). However more recently, methods have been developed to impute endotypic traits from data accessible from routine clinical polysomnography (PSG). For example, Terrill et al. have developed a mathematical method to reliably calculate loop gain from the rise in ventilatory drive that follows an obstructive respiratory event (Terrill et al., 2015). The same group has also developed algorithms for the estimation of pharyngeal collapsibility and compensatory muscle responsiveness from the changes in ventilation and ventilatory drive seen on PSG (Sands et al., 2018). These advances pave the way for more accurate MAS prediction models unencumbered by the need for invasive laboratory studies. In a group of 93 patients with, on average, moderate OSA, greater MAS efficacy was associated with 5 endotypic traits derived using algorithms applied to clinical polysomnographic data: lower loop gain, higher arousal threshold, lower ventilatory response to arousal, moderate pharyngeal collapsibility and weaker muscle compensation (Bamagoos et al., 2019a). The association of lower loop gain and MAS response has also been confirmed in other studies (Edwards et al., 2016; Op de Beeck et al., 2021). These findings may improve prediction models for MAS response, and also raise questions for future research. For example, future studies on combination therapy with MAS plus a carbonic anhydrase inhibitor to facilitate loop gain reduction are warranted (Hedner and Zou, 2022).
Characteristics which act as direct or surrogate markers for the site of airway collapse have also been studied as predictors of response to MAS therapy. For example, the level and specific type of airway collapse observed on drug-induced sleep endoscopy (DISE) has been associated with response to MAS. Tongue-base collapse predicts a favourable response, whereas complete concentric collapse or complete latero-lateral oropharyngeal collapse are seen in those less likely to respond (Op de Beeck et al., 2019). Complete anteroposterior epiglottic collapse predicted an unfavourable response to maxillomandibular advancement surgery (Zhou et al., 2021); however MAS therapy was equally effective in patients with or without epiglottic collapse (Van de Perck et al., 2022). A posteriorly positioned tongue with a less collapsible airway is a positive predictor for MAS therapy (Marques et al., 2019). Further, certain “airflow shapes”, once again derived from routine PSG, have been used to predict the site of airway collapse and thereby response to MAS. Increased drop in airflow during respiratory events as well as a “pinched” expiratory flow shape (indicative of palatal prolapse) is associated with the poorest response to MAS therapy (Vena et al., 2020).
3. MAS titration technology
Traditionally, MAS devices are manually titrated under the supervision of a dentist. Various titration methods have been used, for example titrating to a percentage of maximal mandibular advancement, titrating on the basis of symptoms such as the alleviation of snoring or daytime somnolence, or titrating to an improvement in hypoxic burden which may be measured at home on overnight oximetry. Optimal titration is important to maximise the therapeutic benefits of the MAS device. An advancement of at least 50% of maximum mandibular protrusion is required to have a potential therapeutic outcome while minimising adverse side effects (Aarab et al., 2010; de Ruiter et al., 2020). However, manual titration of a MAS remains inefficient in terms of time to achieve optimal therapeutic outcomes (Sharma et al., 2013; Fleury and Lowe, 2014; Kuna, 2014). International guidelines recommend a progress diagnostic sleep study following titration to assess the efficacy of the device (Ramar et al., 2015).
Novel MAS titration techniques such as the use of a remote-controlled mandibular positioner (RCMP) to determine the therapeutic level of mandibular advancement during a single night PSG have been proposed to overcome the inefficiency barriers to MAS therapy (Pételle et al., 2002; Tsai et al., 2004; Dort et al., 2006; Remmers et al., 2013). Single night PSG titration enables reasonable prospective prediction of MAS therapy success as demonstrated by Remmers et al. (2013). However, RMCP is resource intensive, and requires the use of a sleep laboratory and trained and experienced staff.
A feedback-controlled mandibular positioner (FCMP) was recently developed to enable titration of a MAS device outside the laboratory setting (Remmers et al., 2017). The FMCP combines the use of a level 3 home sleep apnoea test (HSAT), the mechanism of the RCMP and machine learning algorithms to analyse the frequency of sleep disordered breathing and automatically titrate the mandibular advancement device accordingly to resolve sleep disordered breathing (Remmers et al., 2017). An early iteration of the FCMP demonstrated a sensitivity and specificity of 85 and 93% respectively for the prediction of therapeutic success, defined as an oxygen desaturation index (ODI) < 10/hr with the device in situ (Remmers et al., 2017). A subsequent iteration improved the sensitivity and specificity to 91 and 100% respectively, with 93% prediction accuracy (Mosca et al., 2022). This finding highlights the potential for future use of an auto-titrating mandibular advancement device to efficiently identify the therapeutic mandibular position. One small crossover pilot study (n = 10) found no difference in optimal MAS positioning using three titration methods: (1) subjective titration, (2) PSG-guided titration using a remotely controlled mandibular positioner (RCMP) and (3) DISE-assisted titration using RCMP (Kazemeini et al., 2022). Larger studies will be required to confirm the accuracy of remote titration methods compared with in-laboratory titration.
4. Advances in mandibular advancement splint fabrication
The European Respiratory Society recommends a custom-made titratable MAS device as preferable over non-custom devices (Ramar et al., 2015). Recent implementation of digital technologies to dentistry has transformed dental workflows for custom MAS devices (Tallarico, 2020; Alauddin et al., 2021). The use of intraoral scanners and computer aided design/computer aided manufacturing (CAD/CAM) techniques have streamlined the delivery of dental care (Tallarico, 2020; Alauddin et al., 2021). Benefits in patient preference, time savings and elimination of physical storage make digital workflows superior to conventional dental workflows (Mangano et al., 2017). Accuracy of both intraoral scanners and conventional impression methods are also comparable (Afrashtehfar et al., 2022; Hashemi et al., 2022). Device fabrication via CAD/CAM methods allows for the time efficient production of dental devices (van Noort, 2012). The digital technology also provides more accurate measures of tooth and jaw position, improving the quality of device fabrication, as well as facilitating superior observation and monitoring of potential dental side effects from these appliances.
Studies comparing CAD/CAM manufactured MAS devices and conventionally manufactured MAS devices are limited. One study demonstrated a significant increase of 40% in oropharyngeal airway volume in patients who used a CAD/CAM MAS device (Kerbrat et al., 2021). Similar findings were also observed in oral appliance treatment success rates of 63% (Kerbrat et al., 2021) and 80% (Vecchierini et al., 2016) in patients using CAD/CAM devices. In addition, therapy compliance and patient preference favoured the CAD/CAM oral appliances (Vecchierini et al., 2016; Kerbrat et al., 2021). The authors attributed these findings due to the differences in material, shape, and magnitude of vertical opening between devices (Vecchierini et al., 2016).
5. Data collection and remote monitoring
5.1. Adherence data
The collection of efficacy and adherence data is routine for CPAP therapy in clinical practice, and is now available for MAS therapy. The American Academy of Dental Sleep Medicine defines adequate compliance with oral appliance (OA) as a minimum of ≥80% of total sleep time per night, starting when the OA is placed in the mouth and ending when the OA is removed from the mouth, ≥5 nights per week (Radmand et al., 2021). A number of small studies have looked at objectively recorded MAS compliance over an initial 3 month period, and most have found average compliance rates to be in excess of 6 h per night (Sutherland et al., 2021b). In order to objectively assess compliance data, a temperature sensitive sensor microchip may be embedded within, or attached to, the device. When the temperature lies within a certain range (generally 31.5–39.2°C), it is inferred that the device is in situ within the oral cavity, and therefore in use. The device may store from 100 days to many months' worth of compliance information which is available for download via a base-station at the time of patient review. There are a number of models available, with sampling intervals ranging from 5 to 15 min. One study compared the accuracy of three commercially available microsensors under in vitro and in vivo, conditions, and all were found to be highly reliable (Kirshenblatt et al., 2018). Data analysis and display varies according to brand and software. One brand (Dentitrac, Braebon Ltd.) additionally collects and reports positional data (supine vs. non-supine sleep) (Sutherland et al., 2021b). Cluster analysis has identified three main MAS adherence patterns identified over 60 days of objective adherence data recording: “Consistent Users” (48.3%), “Inconsistent Users,” (32.8%) and “Non-Users” (19.0%). These usage patterns can be identified within the first 20 days of therapy, providing an early opportunity for intervention for those patients with sub-therapeutic adherence (Sutherland et al., 2021a).
Development of device-imbedded compliance chips within MAS devices opens up the possibility of remote, real-time monitoring of patient compliance. Integration of device recorded compliance data to cloud-based and patient engagement platforms may improve patient compliance to OSA therapies in some patients. For example, the use of cloud-based monitoring for CPAP therapy has demonstrated an extra hour of CPAP use per night (Hwang et al., 2018; Malhotra et al., 2018). Furthermore, the inclusion of a patient engagement tool which provides coaching and the ability for patients to view CPAP use to remote monitoring further improves device usage (Hostler et al., 2017; Hwang et al., 2018; Woehrle et al., 2018). Additionally, the adherence rate was higher compared to usual care without remote monitoring and patient engagement tools (Hostler et al., 2017; Hwang et al., 2018). While platforms for remote monitoring of MAS compliance are currently limited, integration of these platforms and telehealth modes in future oral appliances may increase the uptake of MAS devices and further improve long term adherence rates for some patients. A recent study evaluated the objective compliance with remote monitoring and therapy feedback to patients for MAS devices (Kwon et al., 2022). Similar trends were noted to that of remote monitoring and therapy feedback for CPAP in that, objective compliance to MAS therapy can be increased with remote monitoring and therapy feedback to patients (Kwon et al., 2022).
5.2. Biological signals
Another recent advance for MAS devices is the integration of buccal oximetry sensors. Evidence on their performance is mixed with very early success with these sensors (Rogers and Gan, 1997), but more recent work suggesting these sensors do not provide accurate oxygen saturation (SpO2) measurement and further technological work was needed to determine if it is the site, the sensors or both which is the issue (De Jong et al., 2011). In contrast, a very recent study by Nabavi et al. (2020) successfully developed a smart MAS that monitors cardiorespiratory parameters intraorally (Nabavi et al., 2020). The device comprised of a flexible hybrid wireless monitoring platform integrated within a MAS, that acquires intraoral photo-plethysmography (PPG) signals. Their results showed that the PPG signals captured intraorally are highly correlated with the conventional PPG signals received and therefore enabled the collection of heart rate (HR), respiratory rate (RR), and SpO2. The estimated values of HR, RR, and SpO2 from the intraoral PPG signals show an accuracy of over 96% with reference to PSG. Further, PPG has been combined in a single device together with positional data and breathing route (mouth vs. nose) (Nabavi and Bhadra, 2021). These developments have exciting potential clinical applications and may translate into a smart MAS device which can facilitate home-based MAS efficacy studies, home-based MAS titration studies as well as capturing data on combination therapy with MAS plus positional devices. Further, the inclusion of physiological sensors highlights the potential for the development of future FCMP devices which can more accurately auto-titrate oral appliance during sleep (Remmers et al., 2017).
Martinot et al. (2019) and Pépin et al. (2020) have shown that mandibular movements measured using midsagittal mounted magnetic sensors on the chin and the forehead, successfully differentiated obstructive and central events (Martinot et al., 2019) and when the signals were combined with machine learning could successfully discriminate controls from apnoea patients (RDI ≥ 5) with an area under the receiver operating characteristic curve (AUC-ROC) of 0.95 (Pépin et al., 2020). More recent work by the same group (Le-Dong et al., 2021) demonstrated that machine learning also enabled accurate sleep staging to be performed with an AUC for wake of 0.98, N1/N2 sleep of 0.86, N3 sleep of 0.97, and REM sleep of 0.96. Additionally, mandibular jaw movements (MJM) can be used as a surrogate measure for nocturnal respiratory effort (RE), since the slight protrusions of the mandible during sleep are reflective of respiratory drive (Martinot et al., 2022). Respiratory effort measured via MJM was a stronger predictor of prevalent hypertension than AHI (Martinot et al., 2022). Such sensors potentially could be embedded within MAS devices to allow capture of compliance data and cardiovascular risk profiling. European Respiratory Society guidelines have highlighted the need for rigorous validation studies for such diagnostic devices which use intelligent sensors, including the need for appropriate power calculations as well as side effect and failure rate profiling. Importantly, it is noted that since the diagnostic algorithms for such devices are not published, the sleep stages and event scoring cannot be manually reviewed or altered as they can be for level 1–4 diagnostic devices (Riha et al., 2023).
At least one manufacturer has recently recognised the potential benefits of instrumenting MAS, announcing the development of a smart oral appliance prototype hardware and software that provides a smart oral appliance with sensors to monitor efficacy and compliance1, see Figure 1.
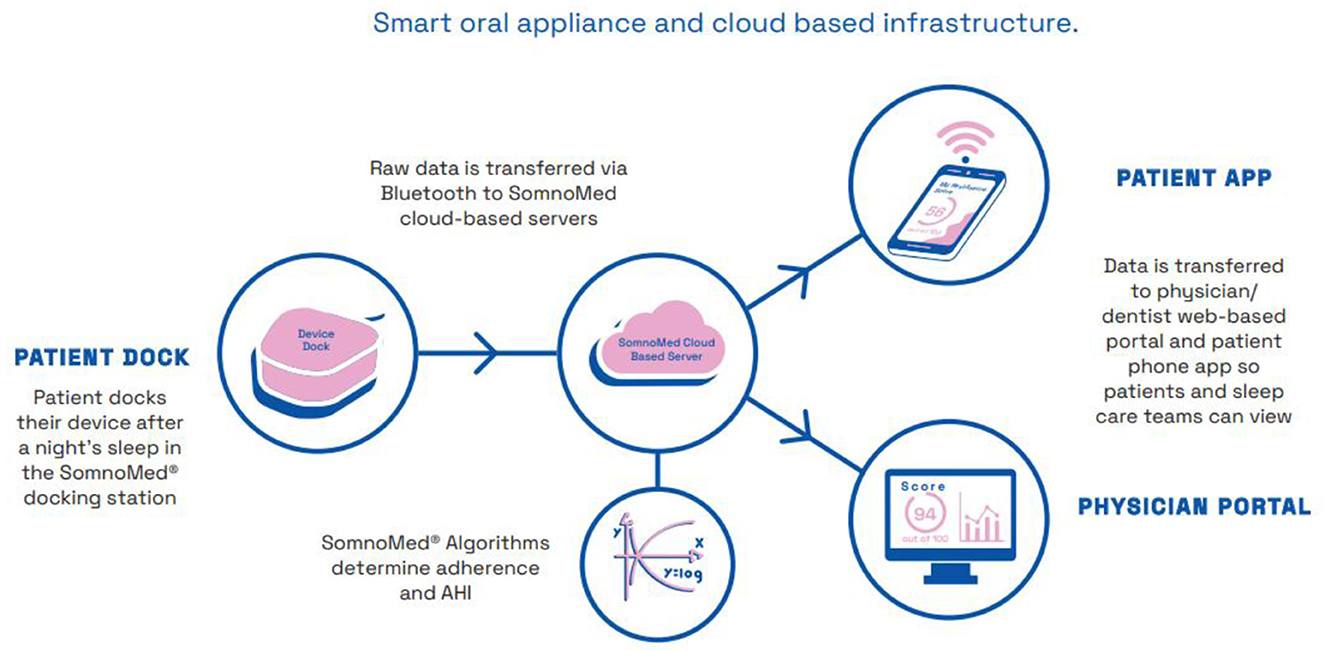
Figure 1. Schematic diagram illustrating mandibular movement sensor technology for the collection, storage, analysis and display of sleep data; as used in a commercial application. Image courtesy of Somnomed.
6. Conclusion
Like many areas of medicine, OSA therapy has now entered the age of personalisation. Advances in MAS therapy discussed here will contribute to personalisation of MAS therapy at the level of patient selection, titration, improved adherence and monitoring of treatment. The use of endo-phenotypes for MAS response prediction models is likely to become more refined and thereby more accurate, allowing for targeted patient selection and combination treatment strategies. New MAS prototypes can incorporate a suite of physiological sensors that support clinical decision making with regards to titration, compliance and efficacy of the device. In particular, mandibular movement sensors have emerged which have diagnostic and treatment applications, though these require rigorous validation studies. MAS compliance monitoring and cloud-based platforms will continue to be integrated into clinical practice to improve patient engagement and compliance. These combined advances will increase the quality and safety of MAS therapy, making it available to increasing groups of patients using a targeted therapeutic approach.
Author contributions
AM, BT, and PDC each wrote sections of the first draft of the manuscript. AM drafted the final version. All authors contributed to the scope and structure of the manuscript. All authors contributed to manuscript revisions and approved the submitted version.
Conflict of interest
AM reports in-kind support from Resmed Pty Ltd., Australia and Somnomed Australia for previous researcher-initiated studies; and has received honoraria from Somnomed Australia for presenting at educational meetings. PC has an appointment to an endowed academic Chair at the University of Sydney that was created from ResMed funding. He has received research support from ResMed, SomnoMed, Zephyr Sleep Technologies, and Bayer. He is a consultant/adviser to Zephyr Sleep Technologies, ResMed, SomnoMed, and Signifier Medical Technologies. He has a pecuniary interest in SomnoMed related to a previous role in R&D (2004). PDC holds an endowed academic chair at the University of Sydney, established through funding from ResMed and has received research support from ResMed, SpaceLabs, and Somnomed.
The remaining author declares that the research was conducted in the absence of any commercial or financial relationships that could be construed as a potential conflict of interest.
Publisher's note
All claims expressed in this article are solely those of the authors and do not necessarily represent those of their affiliated organizations, or those of the publisher, the editors and the reviewers. Any product that may be evaluated in this article, or claim that may be made by its manufacturer, is not guaranteed or endorsed by the publisher.
Footnotes
References
Aarab, G., Lobbezoo, F., Hamburger, H. L., and Naeije, M. (2010). Effects of an oral appliance with different mandibular protrusion positions at a constant vertical dimension on obstructive sleep apnea. Clin. Oral Invest. 14, 339–345. doi: 10.1007/s00784-009-0298-9
Afrashtehfar, K. I., Alnakeb, N. A., and Assery, M. K. M. (2022). Accuracy of intraoral scanners versus traditional impressions: a rapid umbrella review. J. Evid. Based Dent. Pract. 22, 101719. doi: 10.1016/j.jebdp.2022.101719
Alauddin, M. S., Baharuddin, A. S., and Mohd Ghazali, M. I. (2021). The modern and digital transformation of oral health care: a mini review. Healthcare (Basel) 9, 118. doi: 10.3390/healthcare9020118
Bamagoos, A. A., Cistulli, P. A., Sutherland, K., Madronio, M., Eckert, D. J., Hess, L., et al. (2019a). Polysomnographic endotyping to select patients with obstructive sleep apnea for oral appliances. Ann. Am. Thorac. Soc. 16, 1422–1431. doi: 10.1513/AnnalsATS.201903-190OC
Bamagoos, A. A., Cistulli, P. A., Sutherland, K., Ngiam, J., Burke, P. G. R., Bilston, L. E., et al. (2019b). Dose-dependent effects of mandibular advancement on upper airway collapsibility and muscle function in obstructive sleep apnea. Sleep 42. doi: 10.1093/sleep/zsz049
De Jong, M. J., Schmelz, J., Evers, K., Bradshaw, P., Mcknight, K., and Bridges, E. (2011). Accuracy and precision of buccal pulse oximetry. Heart Lung 40, 31–40. doi: 10.1016/j.hrtlng.2009.12.002
de Ruiter, M. H. T., Aarab, G., De Vries, N., Lobbezoo, F., and De Lange, J. (2020). A stepwise titration protocol for oral appliance therapy in positional obstructive sleep apnea patients: proof of concept. Sleep Breath 24, 1229–1236. doi: 10.1007/s11325-020-02045-w
Dort, L. C., Hadjuk, E., and Remmers, J. E. (2006). Mandibular advancement and obstructive sleep apnoea: a method for determining effective mandibular protrusion. Eur. Respir. J. 27, (1003). doi: 10.1183/09031936.06.00077804
Edwards, B. A., Andara, C., Landry, S., Sands, S. A., Joosten, S. A., Owens, R. L., et al. (2016). Upper-airway collapsibility and loop gain predict the response to oral appliance therapy in patients with obstructive sleep apnea. Am. J. Respir. Crit. Care Med. 194, 1413–1422. doi: 10.1164/rccm.201601-0099OC
Edwards, B. A., Redline, S., Sands, S. A., and Owens, R. L. (2019). More Than the Sum of the Respiratory Events: Personalized Medicine Approaches for Obstructive Sleep Apnea. Am. J. Respir. Crit. Care Med. 200, 691–703. doi: 10.1164/rccm.201901-0014TR
Fleury, B., and Lowe, A. A. (2014). ORal Appliance Network for Global Effectiveness Group. Current barriers and study needs for oral appliance therapy: the personal perspective of a physician and dentist. J. Dental Sleep Med. 1, 123–127. doi: 10.15331/jdsm.4138
Grote, L., Hedner, J., Grunstein, R., and Kraiczi, H. (2000). Therapy with nCPAP: incomplete elimination of Sleep Related Breathing Disorder. Eur. Respir. J 16, 921–927. doi: 10.1183/09031936.00.16592100
Hashemi, A. M., Hashemi, H. M., Siadat, H., Shamshiri, A., Afrashtehfar, K. I., and Alikhasi, M. (2022). Fully digital versus conventional workflows for fabricating posterior three-unit implant-supported reconstructions: a prospective crossover clinical trial. Int. J. Environ. Res. Public Health 19, 11456. doi: 10.3390/ijerph191811456
Hedner, J., and Zou, D. (2022). New pharmacologic agents for obstructive sleep apnoea: what do we know and what can we expect? Curr. Opin. Pulm. Med. 28, 522–528. doi: 10.1097/MCP.0000000000000920
Hostler, J. M., Sheikh, K. L., Andrada, T. F., Khramtsov, A., Holley, P. R., and Holley, A. B. (2017). A mobile, web-based system can improve positive airway pressure adherence. J. Sleep Res. 26, 139–146. doi: 10.1111/jsr.12476
Hwang, D., Chang, J. W., Benjafield, A. V., Crocker, M. E., Kelly, C., Becker, K. A., et al. (2018). Effect of Telemedicine Education and Telemonitoring on Continuous Positive Airway Pressure Adherence. The Tele-OSA Randomized Trial. Am. J. Respir. Crit. Care Med. 197, 117–126. doi: 10.1164/rccm.201703-0582OC
Kazemeini, E., Op de Beeck, S., Vroegop, A., Van Loo, D., Willemen, M., Verbraecken, J., et al. (2022). A pilot study on comparison of subjective titration versus remotely controlled mandibular positioning during polysomnography and drug-induced sleep endoscopy, to determine the effective protrusive position for mandibular advancement device therapy. Sleep Breath 26, 1837–1845. doi: 10.1007/s11325-022-02569-3
Kerbrat, A., Vinuesa, O., Lavergne, F., Aversenq, E., Graml, A., Kerbrat, J. B., et al. (2021). Clinical impact of two types of mandibular retention devices - A CAD/CAM design and a traditional design - On upper airway volume in obstructive sleep apnea patients. J. Stomatol. Oral Maxillofac. Surg. 122, 361–366. doi: 10.1016/j.jormas.2021.06.002
Kirshenblatt, S., Chen, H., Dieltjens, M., Pliska, B., and Almeida, F. R. (2018). Accuracy of thermosensitive microsensors intended to monitor patient use of removable oral appliances. J. Can. Dent. Assoc. 84, i2.
Kuna, S. (2014). Looking through the glass ceiling. J. Dental Sleep Med. 1, 129–130. doi: 10.15331/jdsm.4140
Kwon, J.-S., Jung, H.-J., Yu, J.-H., Bae, S.-Y., Park, Y., Cha, J. Y., et al. (2022). Effectiveness of remote monitoring and feedback on objective compliance with a mandibular advancement device for treatment of obstructive sleep apnea. J. Sleep Res. 31, e13508. doi: 10.1111/jsr.13508
Le-Dong, N. N., Martinot, J. B., Coumans, N., Cuthbert, V., Tamisier, R., Bailly, S., et al. (2021). Machine learning-based sleep staging in patients with sleep apnea using a single mandibular movement signal. Am. J. Respir. Crit. Care Med. 204, 1227–1231. doi: 10.1164/rccm.202103-0680LE
Lim, J., Lasserson, T. J., Fleetham, J., and Wright, J. (2006). Oral appliances for obstructive sleep apnoea. Cochrane Datab. Syst. Rev. 2006, Cd004435. doi: 10.1002/14651858.CD004435.pub3
Luz, G. P., Badke, L., Nery, L. E., Silva, L. O., Guimarães, T. M., Coelho, G., et al. (2022). Effect of CPAP vs. mandibular advancement device for excessive daytime sleepiness, fatigue, mood, sustained attention, and quality of life in patients with mild OSA. Sleep Breath. doi: 10.1007/s11325-022-02694-z. [Epub ahead of print].
Malhotra, A., Crocker, M. E., Willes, L., Kelly, C., Lynch, S., and Benjafield, A. V. (2018). Patient engagement using new technology to improve adherence to positive airway pressure therapy: a retrospective analysis. Chest 153, 843–850. doi: 10.1016/j.chest.2017.11.005
Mangano, F., Gandolfi, A., Luongo, G., and Logozzo, S. (2017). Intraoral scanners in dentistry: a review of the current literature. BMC Oral Health 17, 149. doi: 10.1186/s12903-017-0442-x
Marques, M., Genta, P. R., Azarbarzin, A., Taranto-Montemurro, L., Messineo, L., Hess, L. B., et al. (2019). Structure and severity of pharyngeal obstruction determine oral appliance efficacy in sleep apnoea. J. Physiol. 597, 5399–5410. doi: 10.1113/JP278164
Martinot, J. B., Le-Dong, N. N., Cuthbert, V., Denison, S., Borel, J. C., Gozal, D., et al. (2019). Respiratory mandibular movement signals reliably identify obstructive hypopnea events during sleep. Front. Neurol. 10, 828. doi: 10.3389/fneur.2019.00828
Martinot, J. B., Le-Dong, N. N., Malhotra, A., and Pépin, J. L. (2022). Respiratory effort during sleep and prevalent hypertension in obstructive sleep apnoea. Eur Respir J. 61, 1486. doi: 10.1183/13993003.01486-2022
Mosca, E. V., Bruehlmann, S., Zouboules, S. M., Chiew, A. E., Westersund, C., Hambrook, D. A., et al. (2022). In-home mandibular repositioning during sleep using MATRx plus predicts outcome and efficacious positioning for oral appliance treatment of obstructive sleep apnea. J. Clin. Sleep Med. 18, 911–919. doi: 10.5664/jcsm.9758
Nabavi, S., and Bhadra, S. (2021). Smart Mandibular Advancement Device for Intraoral Monitoring of Cardiorespiratory Parameters and Sleeping Postures. IEEE Trans. Biomed. Circuits Syst. 15, 248–258. doi: 10.1109/TBCAS.2021.3065824
Nabavi, S., Debbarma, S., and Bhadra, S. (2020). “A smart mandibular advancement device for intraoral cardiorespiratory monitoring,” in 42nd Annual International Conference of the IEEE Engineering in Medicine & Biology Society (EMBC) 2020. 4079–4084. doi: 10.1109/EMBC44109.2020.9176520
Op de Beeck, S., Dieltjens, M., Azarbarzin, A., Willemen, M., Verbraecken, J., Braem, M. J., et al. (2021). Mandibular advancement device treatment efficacy is associated with polysomnographic endotypes. Ann. Am. Thorac. Soc. 18, 511–518. doi: 10.1513/AnnalsATS.202003-220OC
Op de Beeck, S., Dieltjens, M., Verbruggen, A. E., Vroegop, A. V., Wouters, K., Hamans, E., et al. (2019). Phenotypic labelling using drug-induced sleep endoscopy improves patient selection for mandibular advancement device outcome: a prospective study. J. Clin. Sleep Med. 15, 1089–1099. doi: 10.5664/jcsm.7796
Pépin, J. L., Letesson, C., Le-Dong, N. N., Dedave, A., Denison, S., Cuthbert, V., et al. (2020). Assessment of mandibular movement monitoring with machine learning analysis for the diagnosis of obstructive sleep apnea. JAMA Netw. Open 3, e1919657. doi: 10.1001/jamanetworkopen.2019.19657
Pételle, B., Vincent, G., Gagnadoux, F., Rakotonanahary, D., Meyer, B., and Fleury, B. (2002). One-night mandibular advancement titration for obstructive sleep apnea syndrome. Am. J. Respir. Crit. Care Med. 165, 1150–1153. doi: 10.1164/ajrccm.165.8.2108056
Phillips, C. L., Grunstein, R. R., Darendeliler, M. A., Mihailidou, A. S., Srinivasan, V. K., Yee, B. J., et al. (2013). Health outcomes of continuous positive airway pressure versus oral appliance treatment for obstructive sleep apnea: a randomized controlled trial. Am. J. Respir. Crit. Care Med. 187, 879–887. doi: 10.1164/rccm.201212-2223OC
Radmand, R., Chiang, H., Di Giosia, M., Galang-Boquiren, M. T., Rohatgi, R., Silk, D., et al. (2021). Defining and measuring compliance with oral appliance therapy. J. Dent. Sleep Med. 8, 7204. doi: 10.15331/jdsm.7204
Ramar, K., Dort, L. C., Katz, S. G., Lettieri, C. J., Harrod, C. G., Thomas, S. M., et al. (2015). Clinical practice guideline for the treatment of obstructive sleep apnea and snoring with oral appliance therapy: an update for 2015. J. Clin. Sleep Med. 11, 773–827. doi: 10.5664/jcsm.4858
Remmers, J., Charkhandeh, S., Grosse, J., Topor, Z., Brant, R., Santosham, P., et al. (2013). Remotely controlled mandibular protrusion during sleep predicts therapeutic success with oral appliances in patients with obstructive sleep apnea. Sleep, 36, 1517-25a. doi: 10.5665/sleep.3048
Remmers, J. E., Topor, Z., Grosse, J., Vranjes, N., Mosca, E. V., Brant, R., et al. (2017). A feedback-controlled mandibular positioner identifies individuals with sleep apnea who will respond to oral appliance therapy. J. Clin. Sleep Med. 13, 871–880. doi: 10.5664/jcsm.6656
Riha, R. L., Celmina, M., Cooper, B., Hamutcu-Ersu, R., Kaditis, A., Morley, A., et al. (2023). ERS technical standards for using type III devices (limited channel studies) in the diagnosis of sleep disordered breathing in adults and children. Eur. Respir. J. 61. doi: 10.1183/13993003.00422-2022
Rogers, S. R., and Gan, T. J. (1997). Pulse oximeter probe sheath for buccal use. Anesth. Analg. 85, 1414. doi: 10.1213/00000539-199712000-00052
Sands, S. A., Edwards, B. A., Terrill, P. I., Taranto-Montemurro, L., Azarbarzin, A., Marques, M., et al. (2018). Phenotyping pharyngeal pathophysiology using polysomnography in patients with obstructive sleep apnea. Am. J. Respir. Crit. Care Med. 197, 1187–1197. doi: 10.1164/rccm.201707-1435OC
Schwartz, M., Acosta, L., Hung, Y. L., Padilla, M., and Enciso, R. (2018). Effects of CPAP and mandibular advancement device treatment in obstructive sleep apnea patients: a systematic review and meta-analysis. Sleep Breath 22, 555–568. doi: 10.1007/s11325-017-1590-6
Sharma, S., Essick, G., Schwartz, D., and Aronsky, A. J. (2013). Sleep Medicine Care Under One Roof: A Proposed Model for Integrating Dentistry and Medicine. J. Clin. Sleep Med. 09, 827–833. doi: 10.5664/jcsm.2934
Sutherland, K., Almeida, F. R., Kim, T., Brown, E. C., Knapman, F., Ngiam, J., et al. (2021a). Treatment usage patterns of oral appliances for obstructive sleep apnea over the first 60 days: a cluster analysis. J. Clin. Sleep Med. 17, 1785–1792. doi: 10.5664/jcsm.9288
Sutherland, K., Dalci, O., and Cistulli, P. A. (2021b). What do we know about adherence to oral appliances? Sleep Med. Clin. 16, 145–154. doi: 10.1016/j.jsmc.2020.10.004
Sutherland, K., Takaya, H., Qian, J., Petocz, P., Ng, A. T., and Cistulli, P. A. (2015). Oral appliance treatment response and polysomnographic phenotypes of obstructive sleep apnea. J. Clin. Sleep Med. 11, 861–868. doi: 10.5664/jcsm.4934
Tallarico, M. (2020). Computerization and digital workflow in medicine: focus on digital dentistry. Materials 13, 2172. doi: 10.3390/ma13092172
Terrill, P. I., Edwards, B. A., Nemati, S., Butler, J. P., Owens, R. L., Eckert, D. J., et al. (2015). Quantifying the ventilatory control contribution to sleep apnoea using polysomnography. Eur. Respir. J. 45, 408–418. doi: 10.1183/09031936.00062914
Tsai, W. H., Vazquez, J. C., Oshima, T., Dort, L., Roycroft, B., Lowe, A. A., et al. (2004). Remotely controlled mandibular positioner predicts efficacy of oral appliances in sleep apnea. Am. J. Respir. Crit. Care Med. 170, 366–370. doi: 10.1164/rccm.200310-1446OC
Van de Perck, E., Dieltjens, M., Vroegop, A. V., Verbraecken, J., Braem, M., and Vanderveken, O. M. (2022). Mandibular advancement device therapy in patients with epiglottic collapse. Sleep Breath 26, 1915–1920. doi: 10.1007/s11325-021-02532-8
van Noort, R. (2012). The future of dental devices is digital. Dental Mater. 28, 3–12. doi: 10.1016/j.dental.2011.10.014
Vecchierini, M.-F., Attali, V., Collet, J.-M., D'ortho, M.-P., El Chater, P., Kerbrat, J.-B., et al. (2016). A custom-made mandibular repositioning device for obstructive sleep apnoea–hypopnoea syndrome: the ORCADES study. Sleep Med. 19, 131–140. doi: 10.1016/j.sleep.2015.05.020
Vena, D., Azarbarzin, A., Marques, M., Op de Beeck, S., Vanderveken, O. M., Edwards, B. A., et al. (2020). Predicting sleep apnea responses to oral appliance therapy using polysomnographic airflow. Sleep 43, zsaa004. doi: 10.1093/sleep/zsaa004
Woehrle, H., Arzt, M., Graml, A., Fietze, I., Young, P., Teschler, H., et al. (2018). Effect of a patient engagement tool on positive airway pressure adherence: analysis of a German healthcare provider database. Sleep Med. 41, 20–26. doi: 10.1016/j.sleep.2017.07.026
Zhou, N., Ho, J. T. F., De Vries, N., Bosschieter, P. F. N., Ravesloot, M. J. L., and De Lange, J. (2021). Evaluation of drug-induced sleep endoscopy as a tool for selecting patients with obstructive sleep apnea for maxillomandibular advancement. J. Clin. Sleep Med. 18, 1073–1081. doi: 10.5664/jcsm.9802
Keywords: oral appliance, mandibular advancement splint, mandibular advancement device, sleep apnoea, obstructive sleep apnoea
Citation: Mohammadieh A, Tong B, De Chazal P and Cistulli PA (2023) Innovations in mandibular advancement splint therapy for obstructive sleep apnoea. Front. Sleep 2:1144327. doi: 10.3389/frsle.2023.1144327
Received: 14 January 2023; Accepted: 24 February 2023;
Published: 23 March 2023.
Edited by:
Ding Zou, University of Gothenburg, SwedenReviewed by:
Marie Marklund, Umeå University, SwedenMarijke Dieltjens, University of Antwerp, Belgium
Copyright © 2023 Mohammadieh, Tong, De Chazal and Cistulli. This is an open-access article distributed under the terms of the Creative Commons Attribution License (CC BY). The use, distribution or reproduction in other forums is permitted, provided the original author(s) and the copyright owner(s) are credited and that the original publication in this journal is cited, in accordance with accepted academic practice. No use, distribution or reproduction is permitted which does not comply with these terms.
*Correspondence: Anna Mohammadieh, amoh2259@uni.sydney.edu.au