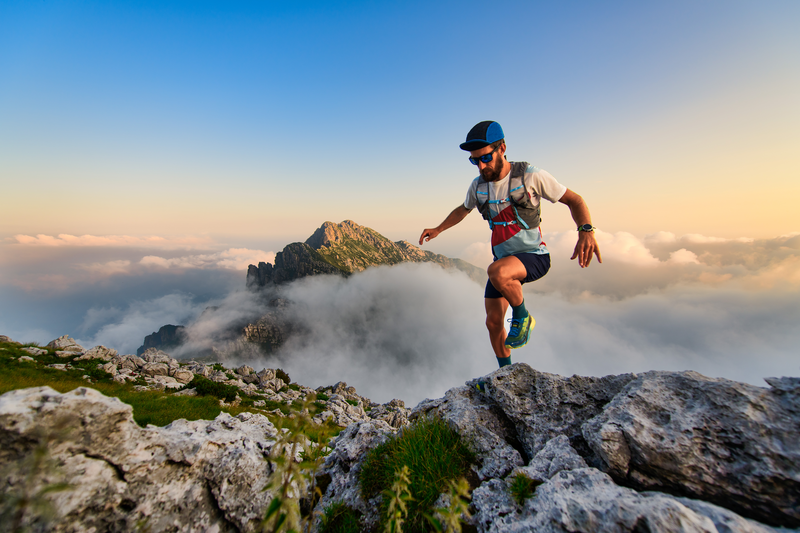
95% of researchers rate our articles as excellent or good
Learn more about the work of our research integrity team to safeguard the quality of each article we publish.
Find out more
REVIEW article
Front. Sleep , 30 March 2023
Sec. Pediatric and Adolescent Sleep
Volume 2 - 2023 | https://doi.org/10.3389/frsle.2023.1127784
This article is part of the Research Topic Novel Technologies in the Diagnosis and Management of Sleep-disordered Breathing View all 22 articles
Purpose: Formal overnight polysomnography (PSG) is required to diagnose obstructive sleep apnea (OSA) in children with sleep disordered breathing (SDB). Most clinical guidelines do not recommend home-based tests for pediatric OSA. However, PSG is limited by feasibility, cost, availability, patient discomfort, and resource utilization. Additionally, the role of PSG in evaluating disease impact may need to be revised. There is a strong need for alternative testing that can stratify the need for PSG and improve the time to diagnosis of OSA. This narrative review aims to evaluate and discuss innovative approaches to pediatric SDB diagnosis.
Findings: Methods to evaluate pediatric SDB outside of PSG include validated questionnaires, single-channel recordings, incorporation of telehealth, home sleep apnea testing (HSAT), and predictive biomarkers. Despite the promise, no individual metric has been found suitable to replace standard PSG. In addition, their use in combination to diagnose OSA diagnosis still needs to be defined.
Summary: When combined with adjunct assessments, HSAT advancements may accurately evaluate SDB in children and thus minimize the need for overnight in-laboratory PSG. Further studies are required to confirm diagnostic validity vis-à-vis PSG as a reference standard.
Sleep-disordered breathing (SDB) in children is characterized by disruption of normal respiration and ventilation cycles during sleep (Gipson et al., 2019), and ranges from mild snoring to obstructive sleep apnea (OSA). Pediatric OSA is associated with lower quality of life (Baldassari et al., 2008), behavior and neurocognitive dysfunction (Landau et al., 2012), impaired growth and development (Nieminen et al., 2000), and greater healthcare utilization (Shehan et al., 2023). Risk factors for OSA in childhood include adenotonsillar hypertrophy (Marcus and Loughlin, 1996), obesity (Mitchell and Kelly, 2007), craniofacial anomalies (Cielo and Marcus, 2015), and neuromuscular disorders (Katz, 2009; Marcus et al., 2012). Prevalence estimates for pediatric OSA range from 1.2 to 5.7% (O'Brien et al., 2003; Bixler et al., 2009; Li et al., 2010), although it approaches 60% in obese children (Verhulst et al., 2008). Pediatric OSA is expected to increase in prevalence with rising childhood obesity (Bryan et al., 2021). Adenotonsillectomy (AT) is the first-line treatment (American Thoracic Society, 1996; Marcus et al., 2012; Mitchell et al., 2019), resulting in resolution or improvement in symptoms in most children. In addition to the obese population, several other cohorts are considered high risk for OSA, with management nuanced by complexity. These include children with Trisomy 21, craniofacial anomalies, craniosynostoses, achondroplasia, and with neuromuscular disorders (ElMallah et al., 2017). Though AT is still considered the first line of treatment, these children have higher rates of residual OSA (Rosen, 2011; Zandieh et al., 2013; Cielo and Marcus, 2015; Moraleda-Cibrián et al., 2015; Thottam et al., 2015; Simpson et al., 2018; Zambon et al., 2022), often requiring additional therapy. Positive pressure may be added to treat residual disease or primarily used in those unsuitable for AT (Muntz, 2012; Nehme et al., 2019). Weight loss (Verhulst et al., 2009), alone or supported by a multidisciplinary team (Roche et al., 2020), has also been beneficial. Additional surgical procedures can also be considered, such as hypoglossal nerve stimulation, uvulopalatopharyngoplasty, inferior turbinate reductions, or lingual tonsillectomy (Ravutha Gounden and Chawla, 2022). Treatment in these cases must be tailored to the child, considering their comorbidities.
Types of PSG are described in Table 1. Currently, overnight, in-laboratory level I polysomnography (PSG) is the only approved technology for diagnosis of pediatric OSA (Marcus et al., 2012; Kirk et al., 2017). Level I PSG is attended by a sleep technician in an accredited facility and includes a minimum of seven parameters: electrooculography (EOG), electroencephalography (EEG), chin electromyography (EMG), airflow, respiratory effort, oxygen saturations, and electrocardiography (ECG) (El Shayeb et al., 2014). Pediatric OSA is diagnosed when the PSG reports an obstructive apnea-hypopnea index (AHI), defined as the frequency of partial or complete reduction in air flow per hour, ≥1 (Mitchell et al., 2019). The most common stratification for mild, moderate, or severe disease is based on AHI thresholds of < 5, 5–9, and ≥10 respectively (Marcus et al., 2013).
Table 1. Types of polysomnography (Collop et al., 2007).
The American Academy of Sleep Medicine (AASM) (Aurora et al., 2011) and the American Academy of Pediatrics (AAP) (Marcus et al., 2012) recommend screening children with SDB with PSG. The American Academy of Otolaryngology—Head and Neck Surgery (AAO–HNS) recommends PSG before AT in children <2 years of age or in those with obesity, craniofacial or neuromuscular disorders, Down syndrome, sickle cell disease, or mucopolysaccharidoses (Mitchell et al., 2019). The AAO–HNS also recommends PSG if the need for surgery is uncertain or if the physical exam does not explain the severity of SDB (Mitchell et al., 2019).
Despite these recommendations, only 10% of children scheduled for AT undergo a PSG (Mitchell et al., 2006). Several barriers exist for level I PSG, e.g., access to a certified sleep laboratory and the limited technical expertise required to diagnose infants and younger children with OSA (Bertoni and Isaiah, 2019). Additionally, the test burden poses unique challenges for children and their families to sleep in an unfamiliar environment while wearing monitoring equipment (Bertoni and Isaiah, 2019). Caregivers must be present during the PSG, which can impact caring for other family members overnight and their productivity the following day. Polysomnography is also expensive, ranging from $1,000 to 4,000 dollars (Bertoni and Isaiah, 2020; Mitchell and Werkhaven, 2020). These issues translate into social and economic burdens for this vulnerable population.
Due to these obstacles, there is a strong need for alternative testing that can approximate PSG results. To date, validated questionnaires (Ahmed et al., 2018; Isaiah et al., 2020; Patel et al., 2020; Wu et al., 2020), single-channel recordings (Kirk et al., 2003; Saito et al., 2007; Álvarez et al., 2017; Hornero et al., 2017; Bertoni et al., 2020), incorporation of telehealth (Paruthi, 2020; Schutte-Rodin, 2020; Castner and D'Andrea, 2022; Griffiths et al., 2022), home-based PSG (Brockmann et al., 2013; Marcus et al., 2014; Ioan et al., 2020; Gao et al., 2021), and biomarkers (Patacchioli et al., 2014; De Luca Canto et al., 2015; Kheirandish-Gozal et al., 2015; Bhattacharjee et al., 2016; Elsharkawi et al., 2017; Teplitzky et al., 2019; Martín-Montero et al., 2022) have been assessed. In this review, we aim to examine innovative approaches to diagnosing pediatric OSA, including exploring combinations of existing technology with the potential for the accurate evaluation of pediatric OSA, thus stratifying the need for traditional overnight PSG.
A narrative review of the relevant literature was performed. Sources were identified through PubMed and Google Scholar searches from September 1, 2022, through January 26, 2023. Search terms were broad and included “pediatric OSA,” “pediatric obstructive sleep apnea,” “pediatric home sleep apnea test,” “sleep questionnaires,” “pediatric sleep questionnaires,” “pediatric OSA diagnosis,” “OSA diagnosis,” “videotaping OSA,” “telehealth pediatric OSA,” “HSAT,” “home diagnosis OSA.” Each article was screened for relevance and quality by the authors and included in the review when deemed appropriate.
The OSA-18 quality of life survey includes 18 symptom-specific questions grouped into five categories: sleep disturbance, physical suffering, emotional distress, daytime problems, and caregiver concerns (Franco et al., 2000). Symptom severity is ranked on an ordinal Likert scale from 1 = none to 7 = all the time. In validation studies of the OSA-18, a total symptom score (TSS) between 60 and 80 correlated with a moderate impact on health-related quality of life. In contrast, a score above 80 indicated a significant impact (Constantin et al., 2010). Ishman et al. (2015) compared OSA-18 responses to PSG metrics. In White children, the specificity and positive predictive value (PPV) of diagnosing OSA using the OSA-18 were 100% when a TSS cut-off of ≥60 and obstructive AHI > 1 were used (Ishman et al., 2015). However, in non-White children, the specificity of these cut-offs was only 67%, with a PPV of 94% (Ishman et al., 2015). The authors also noted a low sensitivity and negative predictive value in all children, indicating an inability to rule out OSA (Ishman et al., 2015). The study concluded that while the OSA-18 questionnaire remains a validated tool to assess the impact of OSA on quality of life, it cannot be used for its diagnosis (Ishman et al., 2015). Constantin et al. (2010) reported that OSA-18 did not accurately diagnose OSA compared to PSG, especially in children with moderate to severe disease. A meta-analysis importantly identified that the OSA-18 could be used as a screening tool for pediatric OSA, though it should not replace PSG for diagnosis (Wu et al., 2020).
The Pediatric Sleep Questionnaire (PSQ) is a validated survey that asks caregivers about the frequency and quality of snoring, breathing problems, mouth breathing, daytime sleepiness, inattention/hyperactivity, and other symptoms (Chervin et al., 2000). Answers to the questions are in a “yes/no/don't know” format (Chervin et al., 2000). Canto et al. (2014) performed a systematic review and meta-analysis of questionnaires to assess for pediatric SDB. The authors noted that the PSQ had sufficient accuracy in screening children for OSA, but it was insufficient to replace PSG (Canto et al., 2014). However, Wu et al. (2020) noted that the PSQ is a sensitive tool for detecting pediatric OSA. The authors also reported that it might be used as a screening tool for OSA. They commented that it could be considered in combination with pulse oximetry in children as an early detection tool (Wu et al., 2020).
Rosen et al. (2015) demonstrated that PSQ symptom scores related to behavior impairment, quality of life, and sleepiness could predict improvement after adenotonsillectomy. Though it could not act as a surrogate for PSG, the authors endorsed its utility as an adjunct to help expect treatment response (Rosen et al., 2015). Patel et al. (2020) studied the predictive accuracy of questionnaires. The sleep-related breathing disorder (SRBD) scale of the PSQ had a sensitivity of 71–84% but a low specificity of 13% (Patel et al., 2020). Also, the area under the receiver operating characteristic (ROC) curve was small, demonstrating poor overall diagnostic accuracy (Patel et al., 2020). They also emphasized the critical role of questionnaires in quantifying the negative impact of SDB on a child's physical and psychological health (Patel et al., 2020). Chervin et al. (2007) demonstrated that the SRBD scale of the PSQ may predict OSA-related neurobehavioral morbidity and response to adenotonsillectomy as well as, and sometimes better than, standard PSG testing. The role of the PSQ may therefore be in screening, assessing SDB-related quality of life, and measuring surgical outcomes in children diagnosed with OSA.
The Children's Sleep Habits Questionnaire (CSHQ) (Owens et al., 2000), is a 45-item questionnaire and includes assessments of parent- and child-reported symptoms among school-aged children aged 4–10 years. Categories of symptoms include bedtime resistance, sleep onset delay, sleep duration, sleep anxiety, night wakening, parasomnias, sleep disordered breathing, and daytime sleepiness (Owens et al., 2000). Items are ranked on a 3-point scale: “usually” if the sleep behavior occurs 5–7 times per week, “sometimes” if it happens 2–4 times/week, and “rarely” for 0–1 times/week. Owens et al. (2000) reported the validity of the CSHQ, concluding it can be used to screen and identify children with sleep disturbances who warrant further testing, though it cannot replace a diagnostic PSG. A summary of validated questionnaires is found in Table 2.
Isaiah et al. (2020) used feature selection algorithms to identify main SDB-related symptoms to predict OSA severity. The authors noted that the original questionnaires were not successful at predicting OSA (Isaiah et al., 2020). However, the selected features eliminated redundancy, resulting in improved prediction performance for OSA severity with a high pre-test probability (Isaiah et al., 2020). Follow-up validation replicated the findings (Kennedy et al., 2022), an essential advance in evaluation for pediatric OSA. This new finding does require further assessment, with multi-institutional validation. However, it holds promise as a reasonable alternative to PSG in resource-limited situations.
The level I polysomnogram measures nine parameters (Bertoni and Isaiah, 2019), of which have been studied in isolation to approximate the overall PSG findings. They are grouped by the system learned; sleep, cardiovascular, oximetry, position, effort, and respiratory (SCOPER) (Collop et al., 2011; Bertoni and Isaiah, 2019, 2020). Of these, cardiovascular, oximetry, position, and respiratory can be measured via single-channel recordings.
Overnight electrocardiogram (ECG) recordings have been evaluated as a potential single-channel recording for pediatric OSA diagnosis. Shouldice et al. (2004) investigated the ability to detect OSA in children based on their ECG recordings during overnight PSG. In this study, obstructive apnea was defined as the absence of airflow with continued chest wall and abdominal movement for ≥2 breaths. Hypopneas were defined as 50% or more decrease in nasal airflow with an associated ≥4% desaturation, an arousal, or both but were only scored if the duration was ≥2 breaths. OSA was diagnosed when the obstructive AHI was ≥1. The authors used a different cutoff system for disease severity, with mild having AHI < 10, moderate AHI 10–15, and severe AHI > 15. The authors created a modified quadratic discriminant analysis classification system, which had a sensitivity of 85% and specificity of 81% to diagnose OSA (Shouldice et al., 2004). However, of the four false negatives identified, three were in cases classified as “mild” OSA, indicating slightly reduced accuracy of this system in children with AHI < 10 (Shouldice et al., 2004). Heart rate variability is an ECG-derived measure that may stratify chronic upper airway obstruction due to the detrimental impact of intermittent hypoxia on autonomic control of the heart (Narkiewicz et al., 1998; Baharav et al., 1999; Teplitzky et al., 2019; Bertoni and Isaiah, 2020). Other studies found predictable changes in heart rate variability in confirmed cases of pediatric OSA (Baharav et al., 1999; Nisbet et al., 2013). Avenues for future research include the integration of cardiac rhythm and other screening methods such as questionnaires or other single channel recordings.
Brouillette et al. (2000) evaluated pulse oximetry's utility for diagnosing pediatric OSA. In this study, when a child was suspected of having OSA, a positive nocturnal oximetry trend had at least 97% PPV of OSA (Brouillette et al., 2000). The authors reported that oximetry could be used for definitive diagnosis in children with SDB and adenotonsillar hypertrophy (Brouillette et al., 2000). Garde et al. (2019) evaluated pulse oximetry as an OSA screen for different AHI thresholds. The authors found that with AHI cut-offs of 1, 5, and 10, the models showed good accuracy, sensitivity, and specificity (Garde et al., 2019). They argue that pulse-oximetry based OSA screening at different AHI cut-offs defined referral thresholds for in-laboratory PSG (Garde et al., 2019). Wu et al. (2020) performed a meta-analysis and determined that the combined use of the PSQ with pulse oximetry can detect OSA in children, though only if PSG is not available. The authors noted that pulse oximetry had a high specificity for screening children without mild OSA and the highest overall specificity when compared to PSQ and OSA-18 (Wu et al., 2020).
Nixon et al. (2004) developed an overnight oximetry data scoring called the McGill Oximetry Score (MOS), which ranges from 1 to 4. A score of 1 indicates a normal/inconclusive OSA study, and additional evaluation for OSA is required (Nixon et al., 2004). A score of 2 designates mild OSA, 3 means moderate OSA, and 4 is severe OSA (Nixon et al., 2004). The scoring is based on the number of drops in arterial oxygen percent saturation (SaO2) < 90, < 85, < 80%, and a number of clusters of desaturation events (Nixon et al., 2004). The authors found that overnight oximetry can estimate OSA severity using this scoring system, allowing prioritization of diagnostic testing and treatment for those with severe OSA (Nixon et al., 2004). The utility of the MOS has been varied in the literature. Chuanprasitkul et al. (2021) evaluated PSG results after children had nocturnal oximetry in the setting of adenotonsillar hypertrophy. The authors found a high rate of OSA in children with inconclusive overnight oximetry, defined as MOS category 1 (Chuanprasitkul et al., 2021). The deficiency of pulse oximetry in isolation was corroborated by Kirk et al. (2003) who found portable oxygen monitoring insufficient to identify OSA in healthy children.
Pavone et al. (2013) studied the role of serial overnight pulse oximetry readings vs. a single night and the ability to diagnose OSA in children. This study identified night-to-night consistent nocturnal pulse oximetry had diagnostic accuracy for OSA (Pavone et al., 2013). The authors argue that two nights of nocturnal pulse oximetry generate an accurate MOS score, which supports a diagnosis of OSA when the category ≥ 2 (Pavone et al., 2013). Similarly, Horwood et al. (2014) evaluated a treatment algorithm based on the MOS. The authors recommended the use of MOS when there is a need to stratify children in resource-limited scenarios (Horwood et al., 2014). Pavone et al. (2017) found that an abnormal pulse oximetry reading predicted the need for AT, supporting its use in contexts where PSG is not readily available (Pavone et al., 2017). Saito et al. (2007) similarly found that pulse oximetry can be used to determine indications for AT. More recently, Hoppenbrouwer et al. (2021) evaluated night-to-night pulse oximetry variability in children by one overnight hospital-based PSG and subsequent home oximetry for two consecutive nights. They found that overall, there was no significant variability between the measurements in the hospital vs. in the home setting (Hoppenbrouwer et al., 2021).
Despite the utility of overnight pulse oximetry as a screening method alone, it remains inferior to PSG. In situations where PSG is feasible, pulse oximetry is not recommended as a substitute. With an improved understanding of optimizing the data from home overnight oximetry and its approximation of PSG data, it holds promise for use in the future as part of a home-based test and potentially as an independent diagnostic tool.
During standard PSG, body position is monitored with apneas, hypopneas, and changes in respiratory patterns (Bertoni and Isaiah, 2019). The most commonly used device is a wrist-worn actigraph (Bertoni and Isaiah, 2019). Less often, body position is detected via hip-worn or in-bed pressure sensors (Bertoni and Isaiah, 2019). Actigraphy helps determine several PSG parameters, including total sleep time (TST), wake after sleep onset (WASO), and sleep efficiency (Bertoni and Isaiah, 2019). There are several actigraphs available, including commercially available wristwatches such as the Fitbit Ultra® (Fitbit, San Francisco, CA) and UP® (Jawbone, San Francisco, CA) (Bertoni and Isaiah, 2019). Sleep-focused actigraphs include the Actiwatch-2® (Phillips Respironics, Amsterdam, The Netherlands) and the Motionlogger® Sleep Watch (Ambulatory Monitoring, Ardsley, NY).
Meltzer et al. (2016) compared the Actiwatch-2® to standard PSG for children with suspected OSA. In this study, the authors found that actigraphy underestimated TST and sleep efficiency, although the sensitivity (0.88) and accuracy (0.84) seemed acceptable (Meltzer et al., 2016). Similar findings were noted when comparing additional brands of actigraphs in children and adolescents (Meltzer et al., 2012), with accurate and sensitive estimations of TST, WASO, and sleep efficiency, though with poor specificity (Toon et al., 2016). The results have been varied, as other studies found commercially available actigraphs poorly approximate sleep compared to PSG (Meltzer et al., 2015). Bertoni et al. (2020) utilized machine learning to generate models which compared overnight PSG parameters to nocturnal actigraphy with the oxygen desaturation index. The goal was to determine if the combination of actigraphy data with oximetry can approximate severe OSA. The authors demonstrated that actigraphy combined with oximetry data could screen for severe OSA (Bertoni et al., 2020), which has implications on postoperative management after AT, as well as a potential diagnostic alternative.
As the data is mixed, further research is needed to help determine the utility of actigraphy in children, particularly in young children who are not yet school age. Given the safety and ease of application, using actigraphy as part of a home sleep evaluation would be beneficial. Their use alone to diagnose OSA is unlikely, though in combination with other single-channel devices shows promise. Further research is needed to assess how to use this technology in the pediatric population effectively.
The AASM suggests that respiratory events be captured using an oronasal thermal airflow sensor or a nasal pressure transducer (Bertoni and Isaiah, 2019). These devices are limited mainly to use in the research setting. There have been studies testing single-channel nasal airflow pressure transducers in diagnosing adult OSA, with some devices having better prediction of OSA than others (Rofail et al., 2010a,b). Small studies have been performed in infants and children regarding the utility and accuracy of using a nasal cannula to detect sleep abnormalities. Trang et al. (2002) evaluated 14 infants to assess the ability of a nasal cannula to detect apneas and hypopneas. The authors found that the nasal cannula was better able to detect hypopneas vs. apneas than a thermistor (Trang et al., 2002). However, an observational study comparing nasal cannula pressure to nasal airflow thermistors in detecting apneas and hypopneas identified that the cannula could detect more events than the thermistor (Serebrisky et al., 2002). This more extensive study in 47 children aged 2–14 years is essential, demonstrating a possible single-channel system for identifying sleep disturbances in children (Serebrisky et al., 2002). However, a recent publication based on 172 children under age 3 demonstrated limited ability for the nasal cannula to detect obstructive events (Jurado et al., 2022). Based on these data, the use of current technology for respiratory monitoring in children is limited. However, its use in combination with other testing metrics or surveys has yet to be evaluated.
The COVID-19 pandemic increased the scope and reach of telehealth services. Sleep medicine is no exception to this movement, demonstrating increased utilization over the pandemic (Paruthi, 2020). Physicians have developed protocols by which to apply telehealth in the evaluation of children with sleep complaints (Witmans et al., 2008). Some pediatric sleep disorders can be adequately evaluated via telehealth appointments, particularly when a physical exam does not alter management. For example, circadian rhythm disorders, insomnia, and sleep-related movement disorders can be managed by telemedicine (Paruthi, 2020). Sleep apnea, however, does require a formal oropharyngeal exam and therefore is not always amenable to telehealth at the initial diagnostic encounter. Though possibly used to screen who requires an in-office exam, telehealth's role in diagnosing pediatric OSA has been challenging. However, incorporating telehealth into HSAT has allowed successful diagnosis in 80% of children around age 10 (Griffiths et al., 2022). This demonstrates an essential caveat to newer technologies, in that study of their use in combination is only just beginning. The use of telehealth as an adjunct during diagnostic testing may prove very beneficial, as demonstrated by Griffiths et al. (2022). Further research is required to assess other benefits of telehealth in pediatric OSA.
The ability of sleep video recordings to approximate PSG findings, or add to home testing, has been studied. Sivan et al. (1996) published the first article describing utility of home video recordings of sleep in screening for OSA. Jacob et al. (1995) similarly noted the value of adding videotape recordings to home testing in children. Lamm et al. (1999) described home videotapes as a useful screening tool in snoring children, though found they cannot distinguish OSA from primary snoring. Given the almost ubiquitous use of smart phones with video technology, Thomas R. J. et al. (2022) recently tested a scoring system for short home sleep videos taken by caregivers during episodes of concerning breathing. The authors found that low scores ruled out moderate-severe OSA, while scores ≥ 3 showed a sensitivity of 100%, specificity of 36%, positive predictive value of 53%, and negative predictive value of 100% for moderate to severe OSA (Thomas R. J. et al., 2022). They concluded that this newly validated clinical scoring system is valuable in triaging children with SDB (Thomas R. J. et al., 2022). Despite successful computer-based analysis of video images in adult OSA (Abad et al., 2016; Muñoz-Ferrer et al., 2019), comparable work is lacking in children. Larger studies are needed to elucidate the role of home videos for the screening and diagnosis of pediatric OSA.
HSAT aims to replicate the results of an in-hospital PSG with increased comfort and accessibility but lower cost and decreased resource utilization (Kirk et al., 2017; Bertoni and Isaiah, 2020). Typically, HSAT is achieved by combining specific PSG channels via portable or wearable sensors (Bertoni and Isaiah, 2020). Once determined to be accessible and feasible (Brockmann et al., 2013; Marcus et al., 2014; Ioan et al., 2020; Lildal et al., 2021), multiple studies have been performed to assess the adequacy of pediatric HSAT.
Gao et al. (2021) performed a systematic review and meta-analysis to determine the diagnostic accuracy of portable, at-home PSG in children. Home testing showed better specificity than sensitivity, indicating appropriate use as a screening method for OSA (Gao et al., 2021). Brunetti et al. (2001) proposed an algorithm to use home testing as a screening tool to help better utilize overnight in-lab testing.
Scalzitti et al. (2017) performed a prospective comparison of home testing to PSG in children aged 2–17. Despite the variability in results, the AHI and lowest oxygen saturation measurements were similar between tests in children aged ≥ 6 years (Scalzitti et al., 2017). These results were echoed by Withers et al. (2022) who compared level I hospital-based PSG to level II home PSG in children aged 5–16. The authors found that level II PSG had an excellent correlation with level I PSG, with the benefit of higher sleep efficiency (Withers et al., 2022). The conclusion of this report was that level II PSG is can be considered for diagnosis in children aged 5–18 (Withers et al., 2022). A study by Alonso-Álvarez et al. (2015) identified home respiratory polygraphy as a potentially helpful and reliable approach for OSA diagnosis in children compared to in-lab PSG. Bhattacharjee et al. (2021) noted acceptable agreement between AHI and oxygen desaturation index between the home and in-laboratory portable monitors, and in-laboratory PSG in 20 adolescents. When installed correctly by trained technicians, home unattended respiratory polygraphic recordings can be used for OSA screening in otherwise healthy children (Ioan et al., 2023). HSAT results may be improved and comparable to in-lab PSG, with the addition of attendance by an online video technician (Green et al., 2022). These results are essential, as they demonstrate a potential population that may be tested at home, creating more availability for children who need PSG for diagnosis. Additionally, the combination of HSAT with screening questionnaires has been beneficial in identifying (Revana et al., 2022), or excluding (Maggio et al., 2021), moderate to severe OSA, further supporting the use of alternate testing methods for improved accuracy.
At this time, the role of HSAT in children is limited to a screening tool, as the AASM does not support the use of HSAT as a replacement for PSG to diagnose OSA in children (Kirk et al., 2017). Difficulty in feasibility, validity, identifying arousals and hypoventilation, issues with use in young children or children with comorbidities, and differences in body sizes are cited as the key limitations (Kirk et al., 2017). The need for home testing is evident, though to date some of the obstacles related to the mechanics of testing have not been overcome. As more studies are performed showing positive outcomes, the guidelines regarding HSAT may eventually evolve.
Untreated OSA has lasting implications on overall health and physiology (Archbold et al., 2012; Marcus et al., 2012; Teplitzky et al., 2019). The study of the systemic impact of OSA has led to evaluation of biomarkers as an alternate means, or adjuncts, for the diagnosis of OSA. From a cardiovascular standpoint, untreated OSA is associated with alterations in left ventricular mass and wall thickness, end diastolic dimensions, and interventricular septal thickness (Amin et al., 2002; Bhattacharjee et al., 2009; Teplitzky et al., 2019). Pediatric OSA can result in right heart failure from pulmonary hypertension, resulting from alveolar hypoventilation from the cyclic apneas, and subsequent pulmonary vasoconstriction (Bhattacharjee et al., 2009; Koc et al., 2012; Teplitzky et al., 2019). For these reasons, pre-operative cardiovascular assessment has been considered, though with debated utility (Li et al., 2008a; Teplitzky et al., 2019; Martín-Montero et al., 2022).
An association between OSA and inflammation was first described by Tauman et al. (2004) who showed that elevated levels of plasma C-reactive protein (CRP), a known marker of inflammation, correlated with AHI, oxygen nadir, and arousal index in some children with OSA (Tauman et al., 2004). This elevation in CRP was also associated with development of cardiovascular disease (Dos Santos et al., 2008), particularly in the setting of obesity (Choi et al., 2013). A large body of evidence has developed evaluating the role of CRP in OSA management. Kheirandish-Gozal et al. (2006) demonstrated elevated CRP levels prior to treatment, with reduction in CRP levels after adenotonsillectomy, adding evidence to support OSA leads to systemic inflammation. This finding has been supported by several authors, noting improvement in systemic inflammation and reduced CRP after OSA treatment (Li et al., 2008b; Ingram and Matthews, 2013; Mutlu et al., 2014; Nachalon et al., 2014; Van Eyck et al., 2014). CRP may also be able to identify residual OSA after adenotonsillectomy (Bhattacharjee et al., 2016). However, the role of CRP in pediatric OSA is nuanced, as other research has noted that CRP in isolation is not predictive of OSA (Kheirandish-Gozal et al., 2015), due to confounding factors such as interindividual variability, environmental, and genetic factors (Kheirandish-Gozal and Gozal, 2017).
Additional metabolic markers have been assessed, given the increasing rates of pediatric obesity (Childhood Obesity Facts, 2022), and concomitant obesity in children with OSA (Bachrach et al., 2022). Comorbid diagnoses to obesity, such as insulin resistance and dyslipidemia, have been evaluated in pediatric OSA (Deboer et al., 2012; Zong et al., 2013; Bhushan et al., 2014; Amini et al., 2017; Siriwat et al., 2020). The use of insulin and lipid levels are not suitable for the diagnosis of OSA, but do denote systemic involvement of the disease, and may serve as an adjunct in global evaluation and work up.
Salivary evaluation has identified OSA biomarkers (Patacchioli et al., 2014; Bencharit et al., 2021), some of which have reportedly acceptable diagnostic accuracy (Canto et al., 2015). Urinary biomarkers have also been studied with mixed results (Biyani et al., 2018). However, some values have the possibility to be used in diagnosis and prediction of OSA severity in children (Villa et al., 2014; Thomas S. et al., 2022). To date, studies on these topics are relatively small in number without reproducibility, limiting their use as a surrogate for PSG. However, their implications on the broad effects of OSA with systemic involvement are apparent. Further studies are needed to better evaluate the role of biomarkers in OSA diagnosis.
High-risk populations include those with Trisomy 21, craniofacial anomalies, and neuromuscular disorders (ElMallah et al., 2017). Alterations in craniofacial measurements, commonly observed in children with syndromes such as Trisomy 21, have been associated with OSA (Katyal et al., 2013; Sutherland et al., 2020). Consideration of body mass index (BMI) and BMI percentile should be assessed in all children to identify those with obesity. Obesity may cause, or worsen OSA, and increases the risk of persistent OSA after AT (O'Brien et al., 2006; Andersen et al., 2016). Obesity is a common comorbidity in children with Trisomy 21, making their OSA more challenging to manage. Children with Trisomy 21 typically are also hypotonic, perpetuating their airway collapse and OSA (Ali Khan, 2022). A multidisciplinary approach between otolaryngology, plastic surgery, pediatric dentistry, and pulmonology/sleep medicine for management of OSA high risk children can improve postoperative AT outcomes and treatment of persistent OSA (DeVries et al., 2020).
Other special populations include children with cleft palate, in whom the underdevelopment and unusual orientation of the palatal musculature increase the propensity for airway collapse (Robison and Otteson, 2011; Muntz, 2012). Surgical procedures designed to repair these anatomic problems are also associated with a higher risk of postoperative upper airway obstruction, requiring a high degree of vigilance following surgery (Rose et al., 2002; Bergeron et al., 2019).
Results of screening tools for OSA in children with Trisomy 21 have been mixed. Several authors have found use of questionnaires unreliable (Grantham-Hill et al., 2020; Skotko et al., 2023). However, Hill et al. (2018) successfully screened for moderate to severe OSA via home pulse oximetry, helping determine which children need formal PSG to confirm OSA. New technologies are emerging to aid in OSA diagnosis (Bassett and Musso, 2017), particularly in these complicated children. Brockmann et al. (2016) studied the feasibility of home PSG in children with Trisomy 21, and obtained a technically successful and acceptable home PSG in 83% of children, concluding that portable home PSG devices may be considered for diagnosis. Ioan et al. (2022) described the utility of pulse transit time (PTT), a technology shown to detect subcortical autonomic arousals, with ventilator polygraphy (PG) to diagnose OSA in children with Trisomy 21. The authors noted a specificity of 1.0 for oAHI >1 event/hour on PTT-PG. When the autonomic arousal index (PTTAI) on PTT-PG is added, the sensitivity for oAHI > 1 is 1.0. The authors concluded that the use of PTT-PG and PTTAI can be diagnostic, though is dependent on signal quality (Ioan et al., 2022). To assess areas of persistent obstruction, cinematic magnetic resonance imaging (“cine MRI”) uses standardized MRI algorithms to localize regions of persistent airway obstruction (Manickam et al., 2016; Isaiah et al., 2018). Another option is drug-induced sleep endoscopy (DISE), with a recent consensus statement on its use in children (Baldassari et al., 2021). In DISE, clinicians can identify potential sites of airway obstruction during sleep using fiberoptic endoscopy to visualize endoluminal upper airway obstruction under anesthesia. The principal uses of cine MRI and DISE are to tailor treatment of upper airway obstruction in children with craniofacial syndromes and potentially in those with recidivism related to OSA. The discussion of OSA in complex children is nuanced and detailed, deserving of its own review. However, it is helpful to address these additional metrics used with PSG, to facilitate personalized approaches for treating pediatric OSA.
Diagnosis of pediatric sleep disordered breathing is limited by access to level I in-lab PSG. Due to the economic burden of this test, patient-family inconvenience, and limited accessibility, additional methods for diagnosis are needed. Though many avenues have been explored, none in isolation has been satisfactory as a replacement to PSG. Further validation efforts are needed to confirm the adequacy of single channel vs. combined channel recordings in the home setting, though data to date are promising. Similarly, adjunct evaluations such as questionnaires and biomarkers, may prove effective when used in conjunction with some of the SCOPER technologies. Newer studies on pediatric HSAT show promise and may eventually be a reasonable option in certain populations. Additional large volume studies are required, but with the great and persistent need for more accessible diagnostic testing, continued research will hopefully identify acceptable and accurate alternatives to level I PSG.
TT, AZ, and AI: manuscript drafting and revision and final manuscript approval accountable for all aspects of the work.
Research reported in this publication was supported by the National Heart Lung Blood Institute of the National Institutes of Health under award number R01HL167012.
AI receives patent-related royalties from University of Maryland for inventions related to sleep apnea diagnosis and treatment. These are not discussed in the manuscript.
The remaining authors declare that the research was conducted in the absence of any commercial or financial relationships that could be construed as a potential conflict of interest.
All claims expressed in this article are solely those of the authors and do not necessarily represent those of their affiliated organizations, or those of the publisher, the editors and the reviewers. Any product that may be evaluated in this article, or claim that may be made by its manufacturer, is not guaranteed or endorsed by the publisher.
The content is solely the responsibility of the authors and does not necessarily represent the official views of the National Institutes of Health.
Abad, J., Muñoz-Ferrer, A., Cervantes, M. Á., Esquinas, C., Marin, A., Martínez, C., et al. (2016). Automatic video analysis for obstructive sleep apnea diagnosis. Sleep 39, 1507–1515. doi: 10.5665/sleep.6008
Ahmed, S., Hasani, S., Koone, M., Thirumuruganathan, S., Diaz-Abad, M., Mitchell, R., et al. (2018). “An empirical study of questionnaires for the diagnosis of pediatric obstructive sleep apnea,” in Annual International Conference of the IEEE Engineering in Medicine and Biology Society. IEEE Engineering in Medicine and Biology Society. Annual International Conference 2018 (Honolulu, HI).
Ali Khan, I. (2022). Role of adenotonsillectomy and tonsillectomy in children with down syndrome who develop obstructive sleep apnea by obesity as a risk factor. Int. J. Pediatr. 2022, 8074094. doi: 10.1155/2022/8074094
Alonso-Álvarez, M. L., Terán-Santos, J., Carbajo, E. O., Cordero-Guevara, J. A., Navazo-Egüia, A. I., Kheirandish-Gozal, L., et al. (2015). Reliability of home respiratory polygraphy for the diagnosis of sleep apnea in children. Chest 147, 1020–1028. doi: 10.1378/chest.14-1959
Álvarez, D., Alonso-Álvarez, M. L., Gutiérrez-Tobal, G. C., Crespo, A., Kheirandish-Gozal, L., Hornero, R., et al. (2017). Automated screening of children with obstructive sleep apnea using nocturnal oximetry: an alternative to respiratory polygraphy in unattended settings. J. Clin. Sleep Med. 13, 693–702. doi: 10.5664/jcsm.6586
American Thoracic Society (1996). Standards and indications for cardiopulmonary sleep studies in children. Am. J. Respir. Crit. Care Med. 153, 866–878. doi: 10.1164/ajrccm.153.2.8564147
Amin, R. S., Kimball, T. R., Bean, J. A., Jeffries, J. L., Willging, J. P., Cotton, R. T., et al. (2002). Left ventricular hypertrophy and abnormal ventricular geometry in children and adolescents with obstructive sleep apnea. Am. J. Respir. Crit. Care Med. 165, 1395–1399. doi: 10.1164/rccm.2105118
Amini, Z., Kotagal, S., Lohse, C., Lloyd, R., Sriram, S., and Kumar, S. (2017). Effect of obstructive sleep apnea treatment on lipids in obese children. Children 4, 44. doi: 10.3390/children4060044
Andersen, I. G., Holm, J.-.C., and Homøe, P. (2016). Obstructive sleep apnea in obese children and adolescents, treatment methods and outcome of treatment - A systematic review. Int. J. Pediatr. Otorhinolaryngol. 87, 190–197. doi: 10.1016/j.ijporl.2016.06.017
Archbold, K. H., Vasquez, M. M., Goodwin, J. L., and Quan, S. F. (2012). Effects of sleep patterns and obesity on increases in blood pressure in a 5-year period: report from the tucson children's assessment of sleep apnea study. J. Pediatr. 161, 26–30. doi: 10.1016/j.jpeds.2011.12.034
Aurora, R. N., Zak, R. S., Karippot, A., Lamm, C. I., Morgenthaler, T. I., Auerbach, S. H., et al. (2011). Practice parameters for the respiratory indications for polysomnography in children. Sleep 34, 379–388. doi: 10.1093/sleep/34.3.379
Bachrach, K., Danis, D. O., Cohen, M. B., and Levi, J. R. (2022). The relationship between obstructive sleep apnea and pediatric obesity: a nationwide analysis. Ann. Otol. Rhinol. Laryngol. 131, 520–526. doi: 10.1177/00034894211028489
Baharav, A., Kotagal, S., Rubin, B. K., Pratt, J., and Akselrod, S. (1999). Autonomic cardiovascular control in children with obstructive sleep apnea. Clin. Auton. Res. 9, 345–351. doi: 10.1007/BF02318382
Baldassari, C. M., Lam, D. J., Ishman, S. L., Chernobilsky, B., Friedman, N. R., Giordano, T., et al. (2021). Expert consensus statement: pediatric drug-induced sleep endoscopy. Otolaryngol. Head Neck Surg. 165, 578–591. doi: 10.1177/0194599820985000
Baldassari, C. M., Mitchell, R. B., Schubert, C., and Rudnick, E. F. (2008). Pediatric obstructive sleep apnea and quality of life: a meta-analysis. Otolaryngol. Head Neck Surg. 138, 265–273. doi: 10.1016/j.otohns.2007.11.003
Bassett, E. C., and Musso, M. F. (2017). Otolaryngologic management of Down syndrome patients: what is new? Curr. Opin. Otolaryngol. Head Neck Surg. 25, 493–497. doi: 10.1097/MOO.0000000000000415
Bencharit, S., Redenz, R. G., Brody, E. R., and Chiang, H. (2021). Salivary biomarkers associated with obstructive sleep apnea: a systematic review. Exp. Rev. Mol. Diagnost. 21, 223–233. doi: 10.1080/14737159.2021.1873132
Bergeron, M., Cohen, A. P., Maby, A., Babiker, H. E., Pan, B. S., and Ishman, S. L. (2019). The effect of cleft palate repair on polysomnography results. J. Clin. Sleep Med. 15, 1581–1586. doi: 10.5664/jcsm.8016
Bertoni, D., and Isaiah, A. (2019). Towards patient-centered diagnosis of pediatric obstructive sleep apnea—A review of biomedical engineering strategies. Exp. Rev. Med. Devices 16, 617–629. doi: 10.1080/17434440.2019.1626233
Bertoni, D., and Isaiah, A. (2020). Home-based screening for obstructive sleep apnea in children. US Respir. Pulm. Dis. 5:38–42. doi: 10.17925/USPRD.2020.5.1.38
Bertoni, D., Sterni, L. M., Pereira, K. D., Das, G., and Isaiah, A. (2020). Predicting polysomnographic severity thresholds in children using machine learning. Pediatr. Res. 88, 404–411. doi: 10.1038/s41390-020-0944-0
Bhattacharjee, R., Benjafield, A., Blase, A., Dever, G., Celso, J., Nation, J., et al. (2021). The accuracy of a portable sleep monitor to diagnose obstructive sleep apnea in adolescent patients. J. Clin. Sleep Med. 17, 1379–1387. doi: 10.5664/jcsm.9202
Bhattacharjee, R., Kheirandish-Gozal, L., Kaditis, A. G., Verhulst, S. L., and Gozal, D. (2016). C-reactive protein as a potential biomarker of residual obstructive sleep apnea following adenotonsillectomy in children. Sleep 39, 283–291. doi: 10.5665/sleep.5428
Bhattacharjee, R., Kheirandish-Gozal, L., Pillar, G., and Gozal, D. (2009). Cardiovascular complications of obstructive sleep apnea syndrome: evidence from children. Prog. Cardiovasc. Dis. 51, 416–433. doi: 10.1016/j.pcad.2008.03.002
Bhushan, B., Maddalozzo, J., Sheldon, S. H., Haymond, S., Rychlik, K., Lales, G. C., et al. (2014). Metabolic alterations in children with obstructive sleep apnea. Int. J. Pediatr. Otorhinolaryngol. 78, 854–859. doi: 10.1016/j.ijporl.2014.02.028
Bixler, E. O., Vgontzas, A. N., Lin, H. M., Liao, D., Calhoun, S., Vela-Bueno, A., et al. (2009). Sleep disordered breathing in children in a general population sample: prevalence and risk factors. Sleep 32, 731–736. doi: 10.1093/sleep/32.6.731
Biyani, S., Benson, M. J., DeShields, S. C., Cunningham, T. D., and Baldassari, C. M. (2018). Urinary leukotriene E4 levels in children with sleep-disordered breathing. Otolaryngol. Head Neck Surg. 158, 947–951. doi: 10.1177/0194599818760281
Brockmann, P. E., Damiani, F., Nuñez, F., Moya, A., Pincheira, E., Paul, M. A., et al. (2016). Sleep-disordered breathing in children with Down syndrome: usefulness of home polysomnography. Int. J. Pediatr. Otorhinolaryngol. 83, 47–50. doi: 10.1016/j.ijporl.2016.01.030
Brockmann, P. E., Perez, J. L., and Moya, A. (2013). Feasibility of unattended home polysomnography in children with sleep-disordered breathing. Int. J. Pediatr. Otorhinolaryngol. 77, 1960–1964. doi: 10.1016/j.ijporl.2013.09.011
Brouillette, R. T., Morielli, A., Leimanis, A., Waters, K. A., Luciano, R., and Ducharme, F. M. (2000). Nocturnal pulse oximetry as an abbreviated testing modality for pediatric obstructive sleep apnea. Pediatrics 105, 405–412. doi: 10.1542/peds.105.2.405
Brunetti, L., Rana, S., Lospalluti, M. L., Pietrafesa, A., Francavilla, R., Fanelli, M., et al. (2001). Prevalence of obstructive sleep apnea syndrome in a cohort of 1,207 children of Southern Italy. Chest 120, 1930–1935. doi: 10.1378/chest.120.6.1930
Bryan, S., Afful, J., Carroll, M., Te-Ching, C., Orlando, D., Fink, S., et al. (2021). NHSR 158. National Health and Nutrition Examination Survey 2017–March 2020 Pre-pandemic Data Files. Available online at: https://stacks.cdc.gov/view/cdc/106273 (accessed November 18, 2022).
Canto, G. D., Pachêco-Pereira, C., Aydinoz, S., Major, P. W., Flores-Mir, C., and Gozal, D. (2015). Diagnostic capability of biological markers in assessment of obstructive sleep apnea: a systematic review and meta-analysis. J. Clin. Sleep Med. 11, 27–36. doi: 10.5664/jcsm.4358
Canto, G. D., Singh, V., Major, M. P., Witmans, M., El-Hakim, H., Major, P. W., et al. (2014). Diagnostic capability of questionnaires and clinical examinations to assess sleep-disordered breathing in children: a systematic review and meta-analysis. J. Am. Dent. Assoc. 145, 165–178. doi: 10.14219/jada.2013.26
Castner, L. M., and D'Andrea, L. A. (2022). Telehealth sleep labs: bringing pediatric polysomnography home. J. Clin. Sleep Med. 18, 1727–1728. doi: 10.5664/jcsm.10046
Chervin, R. D., Hedger, K., Dillon, J. E., and Pituch, K. J. (2000). Pediatric sleep questionnaire (PSQ): validity and reliability of scales for sleep-disordered breathing, snoring, sleepiness, and behavioral problems. Sleep Med. 1, 21–32. doi: 10.1016/S1389-9457(99)00009-X
Chervin, R. D., Weatherly, R. A., Garetz, S. L., Ruzicka, D. L., Giordani, B. J., Hodges, E. K., et al. (2007). Pediatric sleep questionnaire: prediction of sleep apnea and outcomes. Arch. Otolaryngol. Head Neck Surg. 133, 216–222. doi: 10.1001/archotol.133.3.216
Childhood Obesity Facts (2022). Overweight and Obesity. CDC. Available online at: https://www.cdc.gov/obesity/data/childhood.html (accessed November 18, 2022).
Choi, J., Joseph, L., and Pilote, L. (2013). Obesity and C-reactive protein in various populations: a systematic review and meta-analysis. Obes. Rev. 14, 232–244. doi: 10.1111/obr.12003
Chuanprasitkul, C., Veeravigrom, M., Sunkonkit, K., Tansrirattanawong, S., and Sritippayawan, S. (2021). Incidence/predictors of pediatric obstructive sleep apnea with normal oximetry. Pediatr. Int. 63, 1376–1380. doi: 10.1111/ped.14663
Cielo, C. M., and Marcus, C. L. (2015). Obstructive sleep apnoea in children with craniofacial syndromes. Paediatr. Respir. Rev. 16, 189–196. doi: 10.1016/j.prrv.2014.11.003
Collop, N. A., Anderson, W. M., Boehlecke, B., Claman, D., Goldberg, R., Gottlieb, D. J., et al. (2007). Clinical guidelines for the use of unattended portable monitors in the diagnosis of obstructive sleep apnea in adult patients. Portable Monitoring Task Force of the American Academy of Sleep Medicine. J. Clin. Sleep Med. 3, 737–747. doi: 10.5664/jcsm.27032
Collop, N. A., Tracy, S. L., Kapur, V., Mehra, R., Kuhlmann, D., Fleishman, S. A., et al. (2011). Obstructive sleep apnea devices for out-of-center (OOC) testing: technology evaluation. J. Clin. Sleep Med. 7, 531–548. doi: 10.5664/JCSM.1328
Constantin, E., Tewfik, T. L., and Brouillette, R. T. (2010). Can the OSA-18 quality-of-life questionnaire detect obstructive sleep apnea in children? Pediatrics 125, e162–168. doi: 10.1542/peds.2009-0731
De Luca Canto, G. D. L., Pachêco-Pereira, C., Aydinoz, S., Major, P. W., Flores-Mir, C., and Gozal, D. (2015). Biomarkers associated with obstructive sleep apnea: a scoping review. Sleep Med. Rev. 23, 28–45. doi: 10.1016/j.smrv.2014.11.004
Deboer, M. D., Mendoza, J. P., Liu, L., Ford, G., Yu, P. L., and Gaston, B. M. (2012). Increased systemic inflammation overnight correlates with insulin resistance among children evaluated for obstructive sleep apnea. Sleep Breath. 16, 349–354. doi: 10.1007/s11325-011-0499-8
DeVries, J. K., Nation, J. J., Nardone, Z. B., Lance, S. H., Stauffer, J. A., Abichaker, G. M., et al. (2020). Multidisciplinary clinic for care of children with complex obstructive sleep apnea. Int. J. Pediatr. Otorhinolaryngol. 138, 110384. doi: 10.1016/j.ijporl.2020.110384
Dos Santos, M. G., Pegoraro, M., Sandrini, F., and Macuco, E. C. (2008). Risk factors for the development of atherosclerosis in childhood and adolescence. Arq. Bras. Cardiol. 90, 301–308. doi: 10.1590/S0066-782X2008000400012
El Shayeb, M., Topfer, L.-A., Stafinski, T., Pawluk, L., and Menon, D. (2014). Diagnostic accuracy of level 3 portable sleep tests versus level 1 polysomnography for sleep-disordered breathing: a systematic review and meta-analysis. CMAJ 186, E25–E51. doi: 10.1503/cmaj.130952
ElMallah, M., Bailey, E., Trivedi, M., Kremer, T., and Rhein, L. M. (2017). Pediatric obstructive sleep apnea in high-risk populations: clinical implications. Pediatr. Ann. 46, e336–e339. doi: 10.3928/19382359-20170815-01
Elsharkawi, I., Gozal, D., Macklin, E. A., Voelz, L., Weintraub, G., and Skotko, B. G. (2017). Urinary biomarkers and obstructive sleep apnea in patients with Down syndrome. Sleep Med. 34, 84–89. doi: 10.1016/j.sleep.2017.02.005
Franco, R. A., Rosenfeld, R. M., and Rao, M. (2000). Quality of life for children with obstructive sleep apnea. Otolaryngol. Head Neck Surg. 123, 9–16. doi: 10.1067/mhn.2000.105254
Gao, X., Li, Y., Xu, W., and Han, D. (2021). Diagnostic accuracy of level IV portable sleep monitors versus polysomnography for pediatric obstructive sleep apnea: a systematic review and meta-analysis. Sleep Med. 87, 127–137. doi: 10.1016/j.sleep.2021.08.029
Garde, A., Hoppenbrouwer, X., Dehkordi, P., Zhou, G., Rollinson, A. U., Wensley, D., et al. (2019). Pediatric pulse oximetry-based OSA screening at different thresholds of the apnea-hypopnea index with an expression of uncertainty for inconclusive classifications. Sleep Med. 60, 45–52. doi: 10.1016/j.sleep.2018.08.027
Gipson, K., Lu, M., and Kinane, T. B. (2019). Sleep-disordered breathing in children. Pediatr. Rev. 40, 3–13. doi: 10.1542/pir.2018-0142
Grantham-Hill, S., Evans, H. J., Tuffrey, C., Sanders, E., Elphick, H. E., Gringras, P., et al. (2020). Psychometric properties and predictive value of a screening questionnaire for obstructive sleep apnea in young children with Down syndrome. Front. Psychiatry 11, 285. doi: 10.3389/fpsyt.2020.00285
Green, A., Nagel, N., Kemer, L., and Dagan, Y. (2022). Comparing in-lab full polysomnography for diagnosing sleep apnea in children to home sleep apnea tests (HSAT) with an online video attending technician. Sleep Biol. Rhythms 20, 397–401. doi: 10.1007/s41105-022-00384-7
Griffiths, A., Mukushi, A., and Adams, A.-M. (2022). Telehealth-supported level 2 pediatric home polysomnography. J. Clin. Sleep Med. 18, 1815–1821. doi: 10.5664/jcsm.9982
Hill, C. M., Elphick, H. E., Farquhar, M., Gringras, P., Pickering, R. M., Kingshott, R. N., et al. (2018). Home oximetry to screen for obstructive sleep apnoea in Down syndrome. Arch. Dis. Child. 103, 962–967. doi: 10.1136/archdischild-2017-314409
Hoppenbrouwer, X. L. R., Rollinson, A. U., Dunsmuir, D., Ansermino, J. M., Dumont, G., Nijeweme-d'Hollosy, W. O., et al. (2021). Night to night variability of pulse oximetry features in children at home and at the hospital. Physiol. Meas. 42, 104003. doi: 10.1088/1361-6579/ac278e
Hornero, R., Kheirandish-Gozal, L., Gutiérrez-Tobal, G. C., Philby, M. F., Alonso-Álvarez, M. L., Álvarez, D., et al. (2017). Nocturnal oximetry–based evaluation of habitually snoring children. Am. J. Respir. Crit. Care Med. 196, 1591–1598. doi: 10.1164/rccm.201705-0930OC
Horwood, L., Brouillette, R. T., McGregor, C. D., Manoukian, J. J., and Constantin, E. (2014). Testing for pediatric obstructive sleep apnea when health care resources are rationed. JAMA Otolaryngol. Head Neck Surg. 140, 616–623. doi: 10.1001/jamaoto.2014.778
Ingram, D. G., and Matthews, C. K. (2013). Effect of adenotonsillectomy on c-reactive protein levels in children with obstructive sleep apnea: a meta-analysis. Sleep Med. 14, 172–176. doi: 10.1016/j.sleep.2012.11.011
Ioan, I., Renard, E., Da Mota, S., Bonabel, C., Tiotiu, A., Franco, P., et al. (2023). Unattended home sleep studies for the diagnosis of obstructive sleep apnea in a population of French children. Sleep Med. 102, 117–122. doi: 10.1016/j.sleep.2023.01.001
Ioan, I., Weick, D., Schweitzer, C., Guyon, A., Coutier, L., and Franco, P. (2020). Feasibility of parent-attended ambulatory polysomnography in children with suspected obstructive sleep apnea. J. Clin. Sleep Med. 16, 1013–1019. doi: 10.5664/jcsm.8372
Ioan, I., Weick, D., Sevin, F., Sanlaville, D., De Fréminville, B., Schweitzer, C., et al. (2022). Pulse transit time as a diagnostic test for OSA in children with Down syndrome. J. Clin. Sleep Med. 18, 119–128. doi: 10.5664/jcsm.9510
Isaiah, A., Kiss, E., Olomu, P., Koral, K., and Mitchell, R. B. (2018). Characterization of upper airway obstruction using cine MRI in children with residual obstructive sleep apnea after adenotonsillectomy. Sleep Med. 50, 79–86. doi: 10.1016/j.sleep.2017.10.006
Isaiah, A., Shikara, M., Pereira, K. D., and Das, G. (2020). Refining screening questionnaires for prediction of sleep apnea severity in children. Sleep Breath 24, 1349–1356. doi: 10.1007/s11325-019-01964-7
Ishman, S. L., Yang, C. J., Cohen, A. P., Benke, J. R., Meinzen-Derr, J. K., Anderson, R. M., et al. (2015). Is the OSA-18 predictive of obstructive sleep apnea: comparison to polysomnography. Laryngoscope 125, 1491–1495. doi: 10.1002/lary.25098
Jacob, S. V., Morielli, A., Mograss, M. A., Ducharme, F. M., Schloss, M. D., and Brouillette, R. T. (1995). Home testing for pediatric obstructive sleep apnea syndrome secondary to adenotonsillar hypertrophy. Pediatr. Pulmonol. 20, 241–252. doi: 10.1002/ppul.1950200407
Jurado, M. J., Sampol, G., Quintana, M., Romero, O., Cambrodí, R., Ferré, A., et al. (2022). Nasal cannula use during polysomnography in children aged under three with suspected sleep apnea. Sleep Med. 99, 41–48. doi: 10.1016/j.sleep.2022.07.009
Katyal, V., Pamula, Y., Martin, A. J., Daynes, C. N., Kennedy, J. D., and Sampson, W. J. (2013). Craniofacial and upper airway morphology in pediatric sleep-disordered breathing: systematic review and meta-analysis. Am. J. Orthod. Dentofac. Orthop. 143, 20–30.e3. doi: 10.1016/j.ajodo.2012.08.021
Katz, S. L. (2009). Assessment of sleep-disordered breathing in pediatric neuromuscular diseases. Pediatrics 123(Suppl. 4), S222–225. doi: 10.1542/peds.2008-2952E
Kennedy, C. L., Onwumbiko, B. E., Blake, J., Pereira, K. D., and Isaiah, A. (2022). Prospective validation of a brief questionnaire for predicting the severity of pediatric obstructive sleep apnea. Int. J. Pediatr. Otorhinolaryngol. 153, 111018. doi: 10.1016/j.ijporl.2021.111018
Kheirandish-Gozal, L., Capdevila, O. S., Tauman, R., and Gozal, D. (2006). Plasma C-reactive protein in nonobese children with obstructive sleep apnea before and after adenotonsillectomy. J. Clin. Sleep Med. 02, 301–304. doi: 10.5664/jcsm.26589
Kheirandish-Gozal, L., Gileles-Hillel, A., Alonso-Álvarez, M. L., Peris, E., Bhattacharjee, R., Terán-Santos, J., et al. (2015). Effects of adenotonsillectomy on plasma inflammatory biomarkers in obese children with obstructive sleep apnea: a community-based study. Int. J. Obes. 39, 1094–1100. doi: 10.1038/ijo.2015.37
Kheirandish-Gozal, L., and Gozal, D. (2017). Pediatric OSA syndrome morbidity biomarkers: the hunt is finally on! Chest 151, 500–506. doi: 10.1016/j.chest.2016.09.026
Kirk, V., Baughn, J., D'Andrea, L., Friedman, N., Galion, A., Garetz, S., et al. (2017). American academy of sleep medicine position paper for the use of a home sleep apnea test for the diagnosis of OSA in children. J. Clin. Sleep Med. 13, 1199–1203. doi: 10.5664/jcsm.6772
Kirk, V. G., Bohn, S. G., Flemons, W. W., and Remmers, J. E. (2003). Comparison of home oximetry monitoring with laboratory polysomnography in children. Chest 124, 1702–1708. doi: 10.1378/chest.124.5.1702
Koc, S., Aytekin, M., Kalay, N., Ozcetin, M., Burucu, T., Ozbek, K., et al. (2012). The effect of adenotonsillectomy on right ventricle function and pulmonary artery pressure in children with adenotonsillar hypertrophy. Int. J. Pediatr. Otorhinolaryngol. 76, 45–48. doi: 10.1016/j.ijporl.2011.09.028
Lamm, C., Mandeli, J., and Kattan, M. (1999). Evaluation of home audiotapes as an abbreviated test for obstructive sleep apnea syndrome (OSAS) in children. Pediatr. Pulmonol. 27, 267–272. doi: 10.1002/(SICI)1099-0496(199904)27:4<267::AID-PPUL7>3.0.CO;2-X3.0.CO;2-X
Landau, Y. E., Bar-Yishay, O., Greenberg-Dotan, S., Goldbart, A. D., Tarasiuk, A., and Tal, A. (2012). Impaired behavioral and neurocognitive function in preschool children with obstructive sleep apnea. Pediatr. Pulmonol. 47, 180–188. doi: 10.1002/ppul.21534
Li, A. M., Au, C. T., Sung, R. Y. T., Ho, C., Ng, P. C., Fok, T. F., et al. (2008a). Ambulatory blood pressure in children with obstructive sleep apnoea: a community based study. Thorax 63, 803–809. doi: 10.1136/thx.2007.091132
Li, A. M., Chan, M. H. M., Yin, J., So, H. K., Ng, S. K., Chan, I. H. S., et al. (2008b). C-reactive protein in children with obstructive sleep apnea and the effects of treatment. Pediatr. Pulmonol. 43, 34–40. doi: 10.1002/ppul.20732
Li, A. M., So, H. K., Au, C. T., Ho, C., Lau, J., Ng, S. K., et al. (2010). Epidemiology of obstructive sleep apnoea syndrome in Chinese children: a two-phase community study. Thorax 65, 991–997. doi: 10.1136/thx.2010.134858
Lildal, T. K., Bertelsen, J. B., and Ovesen, T. (2021). Feasibility of conducting type III home sleep apnoea test in children. Acta Otolaryngol. 141, 707–713. doi: 10.1080/00016489.2021.1930152
Maggio, A. B. R., Beghetti, M., Van, H. C., Salomon, C. G., Barazzone-Argiroffo, C., and Corbelli, R. (2021). Home respiratory polygraphy in obstructive sleep apnea syndrome in children: Comparison with a screening questionnaire. Int. J. Pediatr. Otorhinolaryngol. 143, 110635. doi: 10.1016/j.ijporl.2021.110635
Manickam, P. V., Shott, S. R., Boss, E. F., Cohen, A. P., Meinzen-Derr, J. K., Amin, R. S., et al. (2016). Systematic review of site of obstruction identification and non-CPAP treatment options for children with persistent pediatric obstructive sleep apnea. Laryngoscope 126, 491–500. doi: 10.1002/lary.25459
Marcus, C. L., Brooks, L. J., Draper, K. A., Gozal, D., Halbower, A. C., Jones, J., et al. (2012). Diagnosis and management of childhood obstructive sleep apnea syndrome. Pediatrics 130, 576–584. doi: 10.1542/peds.2012-1671
Marcus, C. L., and Loughlin, G. M. (1996). Obstructive sleep apnea in children. Semin. Pediatr. Neurol. 3, 23–28. doi: 10.1016/S1071-9091(96)80025-8
Marcus, C. L., Moore, R. H., Rosen, C. L., Giordani, B., Garetz, S. L., Taylor, H. G., et al. (2013). A randomized trial of adenotonsillectomy for childhood sleep apnea. N. Engl. J. Med. 368, 2366–2376. doi: 10.1056/NEJMoa1215881
Marcus, C. L., Traylor, J., Biggs, S. N., Roberts, R. S., Nixon, G. M., Narang, I., et al. (2014). Feasibility of comprehensive, unattended ambulatory polysomnography in school-aged children. J. Clin. Sleep Med. 10, 913–918. doi: 10.5664/jcsm.3970
Martín-Montero, A., Gutiérrez-Tobal, G. C., Kheirandish-Gozal, L., Vaquerizo-Villar, F., Álvarez, D., Campo, F. D., et al. (2022). Heart rate variability as a potential biomarker of pediatric obstructive sleep apnea resolution. Sleep 45, zsab214. doi: 10.1093/sleep/zsab214
Meltzer, L. J., Hiruma, L. S., Avis, K., Montgomery-Downs, H., and Valentin, J. (2015). Comparison of a commercial accelerometer with polysomnography and actigraphy in children and adolescents. Sleep 38, 1323–1330. doi: 10.5665/sleep.4918
Meltzer, L. J., Walsh, C. M., Traylor, J., and Westin, A. M. L. (2012). Direct comparison of two new actigraphs and polysomnography in children and adolescents. Sleep 35, 159–166. doi: 10.5665/sleep.1608
Meltzer, L. J., Wong, P., Biggs, S. N., Traylor, J., Kim, J. Y., Bhattacharjee, R., et al. (2016). Validation of actigraphy in middle childhood. Sleep 39, 1219–1224. doi: 10.5665/sleep.5836
Mitchell, M., and Werkhaven, J. A. (2020). Cost-effectiveness of polysomnography in the management of pediatric obstructive sleep apnea. Int. J. Pediatr. Otorhinolaryngol. 133, 109943. doi: 10.1016/j.ijporl.2020.109943
Mitchell, R. B., Archer, S. M., and Ishman, S. L. (2019). Clinical practice guideline: tonsillectomy in children. Otolaryngol. Head Neck Surg. 160, 187–205. doi: 10.1177/0194599818801757
Mitchell, R. B., and Kelly, J. (2007). Outcome of adenotonsillectomy for obstructive sleep apnea in obese and normal-weight children. Otolaryngol. Head Neck Surg. 137, 43–48. doi: 10.1016/j.otohns.2007.03.028
Mitchell, R. B., Pereira, K. D., and Friedman, N. R. (2006). Sleep-disordered breathing in children: survey of current practice. Laryngoscope 116, 956–958. doi: 10.1097/01.MLG.0000216413.22408.FD
Moraleda-Cibrián, M., Edwards, S. P., Kasten, S. J., Buchman, S. R., Berger, M., and O'Brien, L. M. (2015). Obstructive sleep apnea pretreatment and posttreatment in symptomatic children with congenital craniofacial malformations. J. Clin. Sleep Med. 11, 37–43. doi: 10.5664/jcsm.4360
Muñoz-Ferrer, A., Cervantes, M.-Á., Garcia-Olivé, I., Vicente, I., Folgado, C., Ruiz-Manzano, J., et al. (2019). In-home diagnosis of obstructive sleep apnea using automatic video analysis. Arch. Bronconeumol. 56, 704–709 (2020). doi: 10.1016/j.arbres.2019.11.027
Muntz, H. R. (2012). Management of sleep apnea in the cleft population. Curr. Opin. Otolaryngol. Head Neck Surg. 20, 518–521. doi: 10.1097/MOO.0b013e3283585685
Mutlu, M., Vuralkan, E., Yardim Akaydin, S., Akin, I., and Miser, E. (2014). Effects of adenoid/tonsillectomy on inflammatory response in snoring children with witnessed apnoea. Clin. Otolaryngol. 39, 266–271. doi: 10.1111/coa.12289
Nachalon, Y., Lowenthal, N., Greenberg-Dotan, S., and Goldbart, A. D. (2014). Inflammation and growth in young children with obstructive sleep apnea syndrome before and after adenotonsillectomy. Mediat. Inflamm. 2014, e146893. doi: 10.1155/2014/146893
Narkiewicz, K., Montano, N., Cogliati, C., van de Borne, P. J., Dyken, M. E., and Somers, V. K. (1998). Altered cardiovascular variability in obstructive sleep apnea. Circulation 98, 1071–1077. doi: 10.1161/01.CIR.98.11.1071
Nehme, J., LaBerge, R., Pothos, M., Barrowman, N., Hoey, L., Kukko, M., et al. (2019). Treatment and persistence/recurrence of sleep-disordered breathing in children with Down syndrome. Pediatr. Pulmonol. 54, 1291–1296. doi: 10.1002/ppul.24380
Nieminen, P., Tolonen, U., and Löppönen, H. (2000). Snoring and obstructive sleep apnea in children: a 6-month follow-up study. Arch. Otolaryngol. Head Neck Surg. 126, 481–486. doi: 10.1001/archotol.126.4.481
Nisbet, L. C., Yiallourou, S. R., Nixon, G. M., Biggs, S. N., Davey, M. J., Trinder, J., et al. (2013). Nocturnal autonomic function in preschool children with sleep-disordered breathing. Sleep Med. 14, 1310–1316. doi: 10.1016/j.sleep.2013.07.010
Nixon, G. M., Kermack, A. S., Davis, G. M., Manoukian, J. J., Brown, K. A., and Brouillette, R. T. (2004). Planning adenotonsillectomy in children with obstructive sleep apnea: the role of overnight oximetry. Pediatrics 113, e19–e25. doi: 10.1542/peds.113.1.e19
O'Brien, L. M., Holbrook, C. R., Mervis, C. B., Klaus, C. J., Bruner, J. L., Raffield, T. J., et al. (2003). Sleep and neurobehavioral characteristics of 5- to 7-year-old children with parentally reported symptoms of attention-deficit/hyperactivity disorder. Pediatrics 111, 554–563. doi: 10.1542/peds.111.3.554
O'Brien, L. M., Sitha, S., Baur, L. A., and Waters, K. A. (2006). Obesity increases the risk for persisting obstructive sleep apnea after treatment in children. Int. J. Pediatr. Otorhinolaryngol. 70, 1555–1560. doi: 10.1016/j.ijporl.2006.04.003
Owens, J. A., Spirito, A., and McGuinn, M. (2000). The Children's Sleep Habits Questionnaire (CSHQ): psychometric properties of a survey instrument for school-aged children. Sleep 23, 1043–1051. doi: 10.1093/sleep/23.8.1d
Paruthi, S. (2020). Telemedicine in pediatric sleep. Sleep Med. Clin. 15, e1–e7. doi: 10.1016/j.jsmc.2020.07.003
Patacchioli, F. R., Tabarrini, A., Ghiciuc, C. M., Dima-Cozma, L. C., Prete, A., Bianchini, C., et al. (2014). Salivary biomarkers of obstructive sleep apnea syndrome in children. Pediatr. Pulmonol. 49, 1145–1152. doi: 10.1002/ppul.22972
Patel, A. P., Meghji, S., and Phillips, J. S. (2020). Accuracy of clinical scoring tools for the diagnosis of pediatric obstructive sleep apnea. Laryngoscope 130, 1034–1043. doi: 10.1002/lary.28146
Pavone, M., Cutrera, R., Verrillo, E., Salerno, T., Soldini, S., and Brouillette, R. T. (2013). Night-to-night consistency of at-home nocturnal pulse oximetry testing for obstructive sleep apnea in children. Pediatr. Pulmonol. 48, 754–760. doi: 10.1002/ppul.22685
Pavone, M., Ullmann, N., Verrillo, E., De Vincentiis, G., Sitzia, E., and Cutrera, R. (2017). At-home pulse oximetry in children undergoing adenotonsillectomy for obstructive sleep apnea. Eur. J. Pediatr. 176, 493–499. doi: 10.1007/s00431-017-2868-1
Ravutha Gounden, M., and Chawla, J. K. (2022). Management of residual OSA post adenotonsillectomy in children with Down Syndrome: a systematic review. Int. J. Pediatr. Otorhinolaryngol. 152, 110966. doi: 10.1016/j.ijporl.2021.110966
Revana, A., Vecchio, J., Guffey, D., Minard, C. G., and Glaze, D. G. (2022). Clinical application of home sleep apnea testing in children: a prospective pilot study. J. Clin. Sleep Med. 18, 533–540. doi: 10.5664/jcsm.9650
Robison, J. G., and Otteson, T. D. (2011). Increased prevalence of obstructive sleep apnea in patients with cleft palate. Arch. Otolaryngol. Head Neck Surg. 137, 269–274. doi: 10.1001/archoto.2011.8
Roche, J., Isacco, L., Masurier, J., Pereira, B., Mougin, F., Chaput, J.-P., et al. (2020). Are obstructive sleep apnea and sleep improved in response to multidisciplinary weight loss interventions in youth with obesity? A systematic review and meta-analysis. Int. J. Obes. 44, 753–770. doi: 10.1038/s41366-019-0497-7
Rofail, L. M., Wong, K. K. H., Unger, G., Marks, G. B., and Grunstein, R. R. (2010a). The role of single-channel nasal airflow pressure transducer in the diagnosis of OSA in the sleep laboratory. J. Clin. Sleep Med. 6, 349–356. doi: 10.5664/jcsm.27876
Rofail, L. M., Wong, K. K. H., Unger, G., Marks, G. B., and Grunstein, R. R. (2010b). Comparison between a single-channel nasal airflow device and oximetry for the diagnosis of obstructive sleep apnea. Sleep 33, 1106–1114. doi: 10.1093/sleep/33.8.1106
Rose, E., Staats, R., Thissen, U., Otten, J.-E., Schmelzeisen, R., and Jonas, I. (2002). Sleep-related obstructive disordered breathing in cleft palate patients after palatoplasty. Plast. Reconstr. Surg. 110, 392–396. doi: 10.1097/00006534-200208000-00002
Rosen, C. L., Wang, R., Taylor, H. G., Marcus, C. L., Katz, E. S., Paruthi, S., et al. (2015). Utility of symptoms to predict treatment outcomes in obstructive sleep apnea syndrome. Pediatrics 135, e662–671. doi: 10.1542/peds.2014-3099
Rosen, D. (2011). Management of obstructive sleep apnea associated with Down syndrome and other craniofacial dysmorphologies. Curr. Opin. Pulm. Med. 17, 431–436. doi: 10.1097/MCP.0b013e32834ba9c0
Saito, H., Araki, K., Ozawa, H., Mizutari, K., Inagaki, K., Habu, N., et al. (2007). Pulse-oximetery is useful in determining the indications for adeno-tonsillectomy in pediatric sleep-disordered breathing. Int. J. Pediatr. Otorhinolaryngol. 71, 1–6. doi: 10.1016/j.ijporl.2006.08.009
Scalzitti, N., Hansen, S., Maturo, S., Lospinoso, J., and O'Connor, P. (2017). Comparison of home sleep apnea testing versus laboratory polysomnography for the diagnosis of obstructive sleep apnea in children. Int. J. Pediatr. Otorhinolaryngol. 100, 44–51. doi: 10.1016/j.ijporl.2017.06.013
Schutte-Rodin, S. (2020). Telehealth, telemedicine, and obstructive sleep apnea. Sleep Med. Clin. 15, 359–375. doi: 10.1016/j.jsmc.2020.05.003
Serebrisky, D., Cordero, R., Mandeli, J., Kattan, M., and Lamm, C. (2002). Assessment of inspiratory flow limitation in children with sleep-disordered breathing by a nasal cannula pressure transducer system. Pediatr. Pulmonol. 33, 380–387. doi: 10.1002/ppul.10096
Shehan, J. N., Kakarlapudi, S., Soh, H. H., Uppalapati, A. V., and Levi, J. R. (2023). Age criteria for polysomnography and inpatient management for adenotonsillectomy. Am. J. Otolaryngol. 44, 103670. doi: 10.1016/j.amjoto.2022.103670
Shouldice, R. B., O'Brien, L. M., O'Brien, C., de Chazal, P., Gozal, D., and Heneghan, C. (2004). Detection of obstructive sleep apnea in pediatric subjects using surface lead electrocardiogram features. Sleep 27, 784–792. doi: 10.1093/sleep/27.4.784
Simpson, R., Oyekan, A. A., Ehsan, Z., and Ingram, D. G. (2018). Obstructive sleep apnea in patients with down syndrome: current perspectives. Nat. Sci. Sleep 10, 287–293. doi: 10.2147/NSS.S154723
Siriwat, R., Wang, L., Shah, V., Mehra, R., and Ibrahim, S. (2020). Obstructive sleep apnea and insulin resistance in children with obesity. J. Clin. Sleep Med. 16, 1081–1090. doi: 10.5664/jcsm.8414
Sivan, Y., Kornecki, A., and Schonfeld, T. (1996). Screening obstructive sleep apnoea syndrome by home videotape recording in children. Eur. Respir. J. 9, 2127–2131. doi: 10.1183/09031936.96.09102127
Skotko, B. G., Flores, A. G., Elsharkawi, I., Patsiogiannis, V., McDonough, M. E., Verda, D., et al. (2023). Validation of a predictive model for obstructive sleep apnea in people with Down syndrome. Am. J. Med. Genet. A 191, 518–525. doi: 10.1002/ajmg.a.63055
Sutherland, K., Weichard, A. J., Davey, M. J., Horne, R. S., Cistulli, P. A., and Nixon, G. M. (2020). Craniofacial photography and association with sleep-disordered breathing severity in children. Sleep Breath. 24, 1173–1179. doi: 10.1007/s11325-019-01928-x
Tauman, R., Ivanenko, A., O'Brien, L. M., and Gozal, D. (2004). Plasma C-reactive protein levels among children with sleep-disordered breathing. Pediatrics 113, e564–e569. doi: 10.1542/peds.113.6.e564
Teplitzky, T. B., Pereira, K. D., and Isaiah, A. (2019). Echocardiographic screening in children with very severe obstructive sleep apnea. Int. J. Pediatr. Otorhinolaryngol. 126, 109626. doi: 10.1016/j.ijporl.2019.109626
Thomas, R. J., Dalton, S., Harman, K., Thacker, J., Horne, R. S. C., Davey, M. J., et al. (2022a). Smartphone videos to predict the severity of obstructive sleep apnoea. Arch. Dis. Child. 107, 148–152. doi: 10.1136/archdischild-2020-320752
Thomas, S., Patel, S., Gummalla, P., Tablizo, M. A., and Kier, C. (2022b). You cannot hit snooze on OSA: sequelae of pediatric obstructive sleep apnea. Children 9, 261. doi: 10.3390/children9020261
Thottam, P. J., Trivedi, S., Siegel, B., Williams, K., and Mehta, D. (2015). Comparative outcomes of severe obstructive sleep apnea in pediatric patients with Trisomy 21. Int. J. Pediatr. Otorhinolaryngol. 79, 1013–1016 (2015). doi: 10.1016/j.ijporl.2015.04.015
Toon, E., Davey, M. J., Hollis, S. L., Nixon, G. M., Horne, R. S. C., and Biggs, S. N. (2016). Comparison of commercial wrist-based and smartphone accelerometers, actigraphy, and PSG in a clinical cohort of children and adolescents. J. Clin. Sleep Med. 12, 343–350. doi: 10.5664/jcsm.5580
Trang, H., Leske, V., and Gaultier, C. (2002). Use of nasal cannula for detecting sleep apneas and hypopneas in infants and children. Am. J. Respir. Crit. Care Med. 166, 464–468. doi: 10.1164/rccm.2110114
Van Eyck, A., Van Hoorenbeeck, K., De Winter, B. Y., Ramet, J., Van Gaal, L., De Backer, W., et al. (2014). Sleep-disordered breathing and C-reactive protein in obese children and adolescents. Sleep Breath 18, 335–340. doi: 10.1007/s11325-013-0890-8
Verhulst, S. L., Franckx, H., Van Gaal, L., Backer, D. E., and Desager, W. (2009). The effect of weight loss on sleep-disordered breathing in obese teenagers. Obesity 17, 1178–1183. doi: 10.1038/oby.2008.673
Verhulst, S. L., Van Gaal, L., Backer, D. E., and Desager, W. (2008). The prevalence, anatomical correlates and treatment of sleep-disordered breathing in obese children and adolescents. Sleep Med. Rev. 12, 339–346. doi: 10.1016/j.smrv.2007.11.002
Villa, M. P., Supino, M. C., Fedeli, S., Rabasco, J., Vitelli, O., Pozzo, M. D., et al. (2014). Urinary concentration of 8-isoprostane as marker of severity of pediatric OSAS. Sleep Breath. 18, 723–729. doi: 10.1007/s11325-013-0934-0
Withers, A., Maul, J., Rosenheim, E., O'Donnell, A., Wilson, A., and Stick, S. (2022). Comparison of home ambulatory type 2 polysomnography with a portable monitoring device and in-laboratory type 1 polysomnography for the diagnosis of obstructive sleep apnea in children. J. Clin. Sleep Med. 18, 393–402. doi: 10.5664/jcsm.9576
Witmans, M. B., Dick, B., Good, J., Schoepp, G., Dosman, C., Hawkins, M. E., et al. (2008). Delivery of pediatric sleep services via telehealth: the Alberta experience and lessons learned. Behav. Sleep Med. 6, 207–219. doi: 10.1080/15402000802371312
Wu, C.-R., Tu, Y.-K., Chuang, L.-P., Gordon, C., Chen, N.-H., Chen, P.-Y., et al. (2020). Diagnostic meta-analysis of the pediatric sleep questionnaire, OSA-18, and pulse oximetry in detecting pediatric obstructive sleep apnea syndrome. Sleep Med. Rev. 54, 101355. doi: 10.1016/j.smrv.2020.101355
Zambon, A. A., Trucco, F., Laverty, A., Riley, M., Ridout, D., Manzur, A. Y., et al. (2022). Respiratory function and sleep disordered breathing in pediatric duchenne muscular dystrophy. Neurology 99, e1216–e1226. doi: 10.1212/WNL.0000000000200932
Zandieh, S. O., Padwa, B. L., and Katz, E. S. (2013). Adenotonsillectomy for obstructive sleep apnea in children with syndromic craniosynostosis. Plast. Reconstr. Surg. 131, 847–852. doi: 10.1097/PRS.0b013e3182818f3a
Keywords: sleep apnea, pediatric OSA, PSG (polysomnography), update, pediatric OSA diagnosis
Citation: Teplitzky TB, Zauher A and Isaiah A (2023) Evaluation and diagnosis of pediatric obstructive sleep apnea—An update. Front. Sleep 2:1127784. doi: 10.3389/frsle.2023.1127784
Received: 20 December 2022; Accepted: 16 February 2023;
Published: 30 March 2023.
Edited by:
David Ingram, Children's Mercy Kansas City, United StatesReviewed by:
Mirja Quante, University of Tübingen, GermanyCopyright © 2023 Teplitzky, Zauher and Isaiah. This is an open-access article distributed under the terms of the Creative Commons Attribution License (CC BY). The use, distribution or reproduction in other forums is permitted, provided the original author(s) and the copyright owner(s) are credited and that the original publication in this journal is cited, in accordance with accepted academic practice. No use, distribution or reproduction is permitted which does not comply with these terms.
*Correspondence: Amal Isaiah, YWlzYWlhaEBzb20udW1hcnlsYW5kLmVkdQ==
Disclaimer: All claims expressed in this article are solely those of the authors and do not necessarily represent those of their affiliated organizations, or those of the publisher, the editors and the reviewers. Any product that may be evaluated in this article or claim that may be made by its manufacturer is not guaranteed or endorsed by the publisher.
Research integrity at Frontiers
Learn more about the work of our research integrity team to safeguard the quality of each article we publish.