- 1Respiratory Epidemiology and Clinical Research Unit, Centre for Outcomes Research and Evaluation, Research Institute of the McGill University Health Centre, Montréal, QC, Canada
- 2Department of Medicine, McGill University, Montréal, QC, Canada
- 3Division of Respiratory Medicine, Department of Medicine, McGill University Health Centre, Montréal, QC, Canada
- 4Montreal Chest Institute, McGill University Health Centre, Montréal, QC, Canada
Background: The increasing interest in remote patient monitoring technologies in patients with chronic obstructive pulmonary disease (COPD) requires a phased and stepwise investigative approach, which includes high-risk clinical subgroups who stand to benefit most from such innovations.
Methods: Patients aged > 40 with spirometry-confirmed COPD presenting with a current acute exacerbation (ECOPD) were recruited from a tertiary centre Day Hospital in this prespecified feasibility study. Heart rate, respiratory rate, oxygen saturation, skin temperature, and daily activity and overnight sleep quality parameters were collected remotely by a wearable biometric wristband and ring for 21 consecutive days. “Total ambulatory wear time” and “percent of useable data” for eligible vital sign parameters were calculated. Correlation and agreement between cardiorespiratory vital sign data were performed using Spearman’s correlation rho and the Bland-Altman test, respectively. User experience was measured with end-of-study System Usability Scale (SUS) questionnaires.
Results: Nine participants (mean age 66.8 ± 8.4 years, 22% female, mean FEV1 1.4L (34.1% predicted), with “severe” (56%) or “very severe” (44%) COPD) experiencing a current ECOPD were included. Wear time was 94% (wristband) and 88.2% (ring) of the total ambulatory study period. Wristband-obtained data (every 1 min, artefact-free) revealed 99.2% and 98.6% of all heart rate and temperature data, respectively, was useable, whereas only 17.6% of all respiratory rate data was useable. Ring-obtained data (every 5 min, “average” and “good” quality) revealed 84.5% of all heart rate data was useable. Cross-sectional analyses with nurse-obtained vital signs revealed correlation coefficients of 0.56 (p = 0.11) and 0.81 (p = 0.0086) for wristband-obtained and ring-obtained heart rate, respectively, and only 0.15 (p = 0.74) for wristband-obtained respiratory rate, without evidence of systematic/proportional bias. Longitudinal heart rate and respiratory rate inter-device analyses demonstrated correlations of 0.86 (p < 0.001) and 0.65 (p < 0.001), respectively. Finally, end-of-study SUS scores were 86.4/100 (wristband) and 89.2/100 (ring).
Conclusion: Older adults with severe/very severe COPD experiencing a current ECOPD were capable of autonomous physiological data collection/upload/transmission from their home environment over several weeks using sophisticated wearable biometric technology, with favourable user experiences. Cross-sectional and longitudinal comparative results call into question the paradigm of single sets of infrequent/interval vital sign checks as the current “gold-standard” in frontline clinical practice.
1 Introduction
Chronic obstructive pulmonary disease (COPD) is a highly prevalent and progressive lung disease. The natural history of COPD is characterized by persistent airflow obstruction, breathlessness and exercise intolerance in the chronic phase, as well as by discrete, episodic events, exacerbations of COPD (ECOPDs) in acute phases marked by an increase in airway inflammation and sudden severe shortness of breath (Celli et al., 2021; GOLD, 2024). COPD is now the third leading global cause of death (Safiri et al., 2022; GOLD, 2024). Notably, the worldwide prevalence of COPD is only expected to increase, and is projected to approach 600 million cases (a relative growth of 23%) by 2050 (Boers et al., 2023). Despite advances in chronic disease management, including therapies to decrease the future risk of ECOPDs, these exacerbation events continue to account for one of the single highest rates of hospital admissions and re-hospitalization in adults among all major chronic illnesses (Ford, 2015; Amegadzie et al., 2023), and the severity and frequency of ECOPDs are a clear prognostic factor for mortality (Soler-Cataluña et al., 2005). There is an urgent need for health system models around the world to adapt and restructure in order to accommodate the substantial ongoing challenges in the delivery of chronic and acute COPD care.
The conventional operational diagnostic and classification criteria for ECOPDs, in which these acute events were classified retrospectively largely based on how they were ultimately treated (Rodriguez-Roisin, 2000; Celli et al., 2021), have been associated with important limitations in advancing exacerbation research and innovations to clinical care. Furthermore, many ECOPDs go unreported altogether in part by limitations in patient self-recognition (Seemungal et al., 1998; Wedzicha and Seemungal, 2007) as the individual-level perception of exacerbation symptoms is remarkably heterogeneous between patients (Laue et al., 2017; Scioscia et al., 2017). The new diagnostic and classification criteria put forth by the Rome Proposal, which has subsequently been incorporated into the 2023 and 2024 editions of the Global Strategy for the Diagnosis, Management and Prevention of COPD (GOLD) Reports, now classify exacerbations by more “objective” cardiorespiratory physiological parameter criteria including changes in respiratory rate, heart rate, and oxygen saturation. This new ECOPD classification framework is very timely given that simultaneous advances in technology have made the once ambitious prospect of near-continuous accurate remote patient physiologic monitoring now more feasible than ever before. Furthermore, the important shortcomings in ensuring appropriate COPD care during the COVID-19 pandemic era, which included both public health interventions (social distancing, lockdowns) as well as healthcare service closures (respiratory clinics, pulmonary rehabilitation programs and pulmonary function testing labs) have further demonstrated the immediate clinical relevance of remote patient monitoring platform development (Dechman et al., 2020; Stanojevic et al., 2022).
Sophisticated wearable biometric devices (“wearables”) can be worn for prolonged periods and are capable of accurately measuring physiological parameters (Aliverti, 2017). With well over a decade of improvements and innovations in wearable technologies, in terms of both hardware and software, remote patient monitoring through the use of wearable devices has become increasingly attractive as a chronic disease management tool in COPD (GOLD, 2024) given that near-continuous accurate ambulatory monitoring would allow for the early detection of abnormal objective parameters in deteriorating patients (Soon et al., 2020). In patients with COPD, studies which predated modern wearables demonstrated that subtle increases in respiratory rate, detected from monitors installed onto domiciliary oxygen supply systems in home oxygen recipients or from non-invasive ventilation machine downloads, were present and detectable up to 5 days before an ECOPD hospitalization, offering an opportunity for early intervention (Yañez et al., 2012; Borel et al., 2015). Subsequent studies have found that the monitoring of additional physiological parameters such as oxygen saturation and heart rate along with respiratory rate, as presented in the Rome Proposal, may be cumulatively applied towards the early subclinical detection of ECOPDs (Al Rajeh and Hurst, 2016). Recent qualitative and quantitative feasibility studies in the COPD patient population have demonstrated that, in concert with the development of this technology, patients themselves who are living with COPD are willing and keen to wear wearables such as smartwatches and vests capable of passively monitoring vital signs and activity (Rubio et al., 2017; Wu et al., 2018; Liaqat et al., 2019; Hawthorne et al., 2022).
Prior to the actual incorporation of novel wearables-based remote patient monitoring platforms into the conventional COPD health delivery model, however, more evidence is needed to support the capacity for effective, autonomous, sustained remote operation of platform components in high-risk COPD patient subpopulations. Potential technology illiteracy and discomfort with technology use (both software and hardware) is prevalent and may be even more common in older patients with COPD (Jiang et al., 2023), those with advanced chronic disease, and those with frequent exacerbations. Paradoxically, these same patients, who stand most likely to benefit from such innovations in management, are at risk of being excluded altogether in studies which investigate technological innovations to care (Western et al., 2021; Navathe et al., 2022; Coutu et al., 2023). The primary objective of this study was to determine the capacity of patients with severe COPD who are experiencing a current exacerbation to operate wearable technology and to autonomously collect and transmit physiological data as outpatients over several consecutive weeks. The secondary objectives of this study were to compare the cardiorespiratory vital sign data collected from the wearable devices with the prevailing gold standard of a single set of vital signs obtained by a clinical nurse, as well as with one another, and finally to explore the COPD patient user experience with wearables-based remote patient monitoring technology. We hypothesized that patients with severe COPD experiencing an acute exacerbation would demonstrate a high ambulatory device total wear time, and that a high proportion of “useable” physiological data would be obtained through this wearables-based remote monitoring system. We also hypothesized close correlation and agreement in the cardiorespiratory parameters and favourable patient usability scores.
2 Materials and methods
2.1 Study design and population
This single-center prospective pre-specified non-interventional pilot and feasibility study, embedded within clinicaltrials.gov study NCT05776654 and approved by the Research Ethical Board (2023-8851), was conducted at the McGill University Health Centre (MUHC). Males and females aged >40 who were former or current smokers with COPD confirmed by spirometry (FEV1/FVC <0.7) and presenting with and receiving treatment for a current exacerbation were eligible for recruitment. Participants were excluded if they received four or more days of consecutive corticosteroid and/or antibiotic therapy at the time of initial presentation. Additional exclusion criteria included an inability to provide informed consent, no pre-existing diagnosis of COPD, and the presence of cardiac arrhythmia and/or presence of pacemaker/defibrillator. While in the larger prospective study patients are eligible for recruitment from the from the Montreal Chest Institute (MCI) Day Hospital, outpatient clinics, and inpatient respiratory ward as well as from the MUHC Emergency Department, with no predefined cutoff in airflow obstruction severity as determined by FEV1 (GOLD 1–4), in the present pilot and feasibility study only patients with GOLD 3 (“severe”) and GOLD 4 (“very severe”) COPD were included, and were only recruited from the MCI Day Hospital where each patient underwent triage vital signs on the day of their ECOPD presentation by a respiratory clinical nurse.
Eligible participants were approached for in-person recruitment by a study team member. Demographics, clinical history, and comorbidities were obtained from information provided by participants and were confirmed in the medical records. Spirometry data and participant vitals, including heart rate, respiratory rate, body temperature, and oxygen saturation taken by the Day Hospital nurse were collected from the medical chart.
2.2 Study protocol and equipment
Each participant was provided with a biometric wristband (EmbracePlus, Empatica Inc.), a biometric ring (Oura Gen III, Oura Health Oy) fitted based on patient finger size, and a study tablet. All device fitting, provision of study equipment and accessories (chargers), and training regarding the proper wear, charging, and upload/transmission of wearable device data via the tablet interface was completed at the bedside at one single in-person study visit, which occurred along with recruitment on the same day as the initial patient ECOPD presentation to the Day Hospital. Participants were instructed to wear the wristband and the ring at all times, except when showering or charging the devices, for a total of 21 consecutive days. While a printed instruction sheet covering the training session was provided to each participant, and while participants were notified that they could reach a study team member during the ambulatory follow-up period on an as-needed basis in case of troubleshooting issues, they were otherwise tasked with independent, autonomous operation of the wearable devices throughout the 21-day study period from their home environment.
Respiratory rate, heart rate, oxygen saturation and body temperature were recorded in the chart by the Day Hospital nurse as part of standard vital signs measurement on the day of recruitment. The collection of these parameters, and additional parameters, by the combination of the wristband and the ring, commenced following the in-person recruitment and training study visit on study Day 1 in the Day Hospital. Data collection continued following the participant’s return home that same day, for a total of 21 consecutive days and nights from the participant’s own home environment. Data was captured by device wear in the home environment and was then wirelessly transmitted in a remote manner to the study team for offline analysis.
Both devices contain photoplethysmography (PPG) sensors, temperature sensors, and 3-dimensional accelerometers and gyroscopes. Each device is also capable of sensing and reporting when it is, and is not, being worn. The vital sign parameters that are obtained from the wristband include respiratory rate, heart rate (and variability), and skin temperature. Activity level including step count, acceleration, activity count/intensity, sleep detection and body position, and electrodermal activity are also collected as wristband physiological parameters. Raw data for each of these parameters are averaged and made available in 1-min increments throughout the day and night from the wristband, and measurements commence right from the time of initial wear.
The vital sign parameters that are obtained from the ring in a near-continuous fashion (5-min increments) throughout the day and night are limited to heart rate (and variability) alone, whereas respiratory rate (and variation) and oxygen saturation are averaged each night and are made available as one single nightly average from the ring. Likewise, nightly sleep quality metrics (including time spent asleep/awake, sleep efficiency, sleep latency, sleep stages, and duration of REM, deep, and light sleep) and daily activity metrics (including step count, movement, total and “active” calorie consumption, and metabolic equivalents) are made available as single nightly and single daily averaged values, respectively. While heart rate measurements from the ring commence right from the time of initial wear, nightly averaged data from the ring is not available on the very first night of wear (due to a mandatory device-specific run-in period) and therefore these parameters were only available from the second night onwards during the study period.
An end-of-study System Usability Scale (SUS) questionnaire, composed of 10 questions answered on a Likert scale and used in previous studies as a practical, reliable, and versatile tool to assess the user experience with technology (Lewis et al., 2009; Liang et al., 2018; Keogh et al., 2020), was administered on the last day of the study to determine the COPD participant experience and usability with each device. A separate questionnaire was administered for the wristband and for the ring.
2.3 Study outcomes and statistical analysis
The co-primary outcomes for this multi-week feasibility study, “total ambulatory wear time” and “percent of useable data”, served as the descriptive markers for the capacity of study participants to successfully collect and transmit outpatient physiological data remotely in an autonomous manner. “Total ambulatory wear time” was calculated by first summing the total daily and nightly time that the wearable was sensed and reported as being worn for each participant, and by then dividing this value by the total number of study days/nights for each participant over the observation period. The “percent of useable data” analyses were limited to vital sign parameters with data available in non-daily/non-nightly averaged increments (i.e., if the vital sign parameter data was available every 1–5 min rather than as one single daily or nightly average) given that parameters made available as single daily or nightly averages provide little information on the actual underlying quantity of acceptable-quality sampling of physiological data over such long observation periods.
During the data recording process artefactual data is handled and reported in distinct ways by each device. Regarding data quality monitoring by the wristband, the 1-min average for a given parameter is either provided, meaning that the value is deemed by the device to be reliable, or is simply not provided as an intentional missing value with an accompanying reason (ex. “worn during motion”, “device not worn correctly”, “worn with low signal quality”) depending on whether the threshold for poor quality for a given parameter was reached or not. Regarding data quality monitoring by the ring, conversely, the 5-min heart rate value is always provided regardless of the level of quality deemed by the ring, however each reported value is accompanied by a descriptive marker for the quality of that incremental measurement (“good”, “average”, “bad”). “Percent of useable data” for each eligible parameter on each device was therefore calculated by filtering and summing the total number of 1-min increments (wristband) for which data of reliable quality was reported, and filtering and summing the total number of 5-min increments (ring) for which data was of “average” or “good” quality, and by then dividing those values by the total eligible number of time increments during which the wearable device was sensed as being worn for each participant over the entire ambulatory study period.
The secondary outcomes were the Day 1 correlation and agreement between cardiorespiratory vital signs obtained by the Day Hospital nurse with those obtained by the wearable devices on the study recruitment day (cross-sectional analyses); the correlation between cardiorespiratory vital signs between the wristband and ring over the entire study period (longitudinal analyses); and the end-of-study SUS scores (device-specific user experience). Because the earliest respiratory rate and oxygen saturation data available from the ring was from the night of study Day 2, cross-sectional comparison with Day 1 nurse-obtained vital signs was not possible. Likewise, non-overlap of certain vital sign parameters (oxygen saturation and temperature) precluded these longitudinal inter-device comparisons.
Baseline characteristic and demographic data were presented as means and standard deviations (SD) for continuous variables and as absolute frequencies (n) and percentages for categorical variables, unless otherwise indicated. The co-primary outcomes were reported as percentages. Correlation and agreement analyses were performed using Spearman’s correlation rho and the Bland-Altman test, respectively. While the strength of correlation was determined following standard interpretation guidelines (i.e., 0.1 < r ≤ 0.3 = weak, 0.3 < r ≤ 0.5 = moderate, and r > 0.5 = strong) (Cohen, 1988; Eden et al., 2022), the detection of the presence of systematic differences between measurements (i.e., fixed bias) or of possible outliers was determined by constructing Bland-Altman plots (Karun and Puranik, 2021). A p-value of <0.05 was considered to be indicative of reaching statistical significance. SUS scores were scored out of 100 and were reported descriptively. All statistical analyses were performed in R Statistical Software, version 4.3 (R project, 2023).
3 Results
3.1 Participant characteristics
A total of nine patients who met all eligibility criteria were included in the present feasibility study. Baseline demographics and lung function are presented in Table 1. Baseline vital signs obtained by the Day Hospital nurse and from the biometric wristband (Day 1) and ring (Day 1 and Day 2 overnight) are presented in Table 2. Two (22%) participants were female and seven (78%) were male. All participants had either “severe” or “very severe” COPD and were older adults (mean age 66.8 ± 8.4 years).
3.2 Co-primary outcomes
The co-primary outcomes, total ambulatory wear time for each device and the percentage of useable data for each eligible device-obtained vital sign, are presented in Table 3. Out of the 21-day observation period in the nine study participants, the wristband was worn for 94% of the total ambulatory study time, while the ring was worn for 88.2% of the total ambulatory study time.
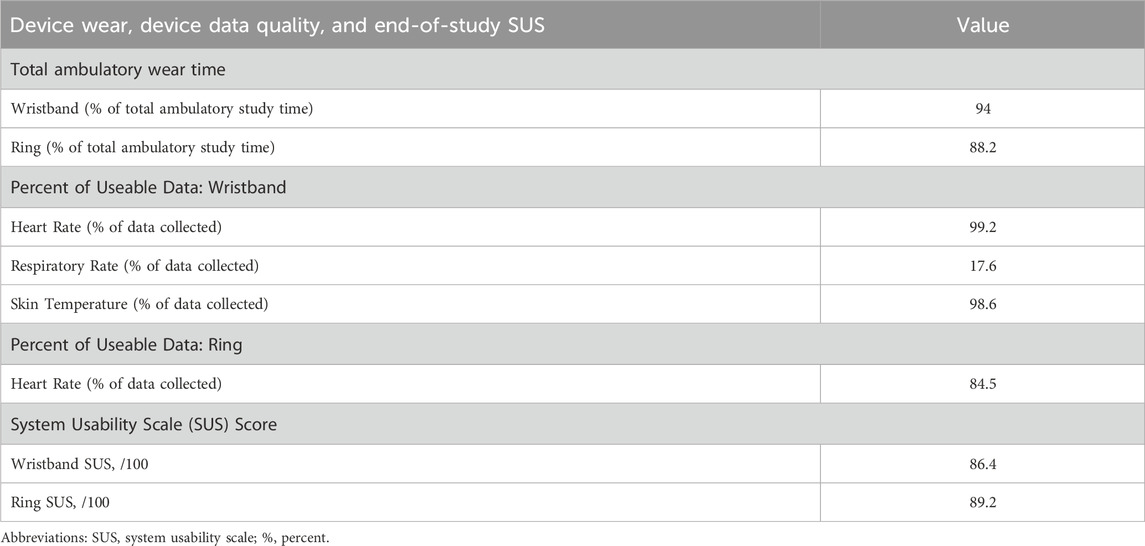
Table 3. Total ambulatory wear time, percent of useable data obtained, and system usability scale (SUS) scores for the wearable biometric wristband and ring.
The vital sign parameters from the wristband that were eligible for percent of useable data analysis were heart rate, respiratory rate, and skin temperature, since all were retrievable in 1-min increments continuously (day and night) over the entire 21-day study period. A high percentage of useable data for wristband-obtained heart rate and skin temperature, which were 99.2% and 98.6% of all data, respectively, was observed. Conversely, only 17.6% of all wristband-obtained respiratory rate data was useable. The only vital sign from the ring that was eligible for percent of useable data analysis was heart rate since it was the only parameter available in 5-min increments, near-continuously (day and night), over the 21-day study period. A high percentage of useable data, 84.5%, was observed for ring-obtained heart rate.
3.3 Secondary outcomes
The cross-sectional correlation plots and Bland-Altman plots of the measures of agreement between heart rate as measured by the Day Hospital nurse and heart rate as measured by the wristband and by the ring on study Day 1 are presented in Figure 1 and Figure 2, respectively. Spearman’s correlation coefficient r for the association between wristband-obtained and Day Hospital nurse-obtained heart rate was 0.56 (p = 0.11). Spearman’s correlation coefficient r for the association between ring-obtained and Day Hospital nurse-obtained heart rate was 0.81 (p = 0.0086). Wristband-obtained and ring-obtained heart rate demonstrated excellent cross-sectional agreement overall (mean difference of −0.98 ± 10.7 and −2.56 ± 8.73 bpm, respectively; Figure 1B, Figure 2B), with no statistically significant difference observed (p = 0.79 and p = 0.40, respectively) when compared with nurse-obtained heart rate.
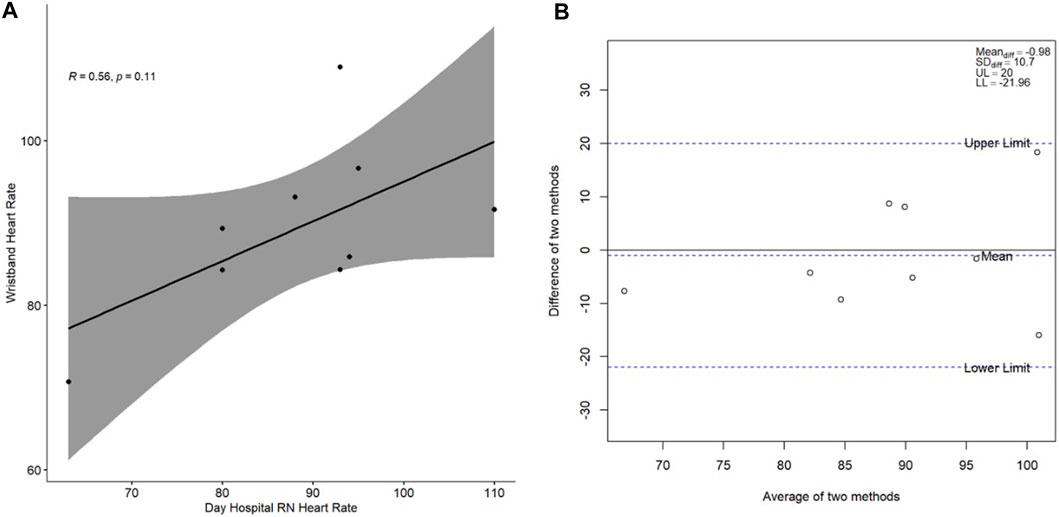
Figure 1. (A) Spearman correlation of wristband-obtained heart rate in relation to Day Hospital nurse-obtained heart rate; (B) Bland-Altman plot of the difference between the two heart rate measurement methods (y-axis) in relation to the average of the two heart rate measurement methods (x-axis). Heart rate is presented in beats per minute. Abbreviations: RN, nurse; R, Spearman correlation coefficient; Meandiff, mean of difference; SDdiff, standard deviation of difference; UL, upper limit; LL, lower limit.
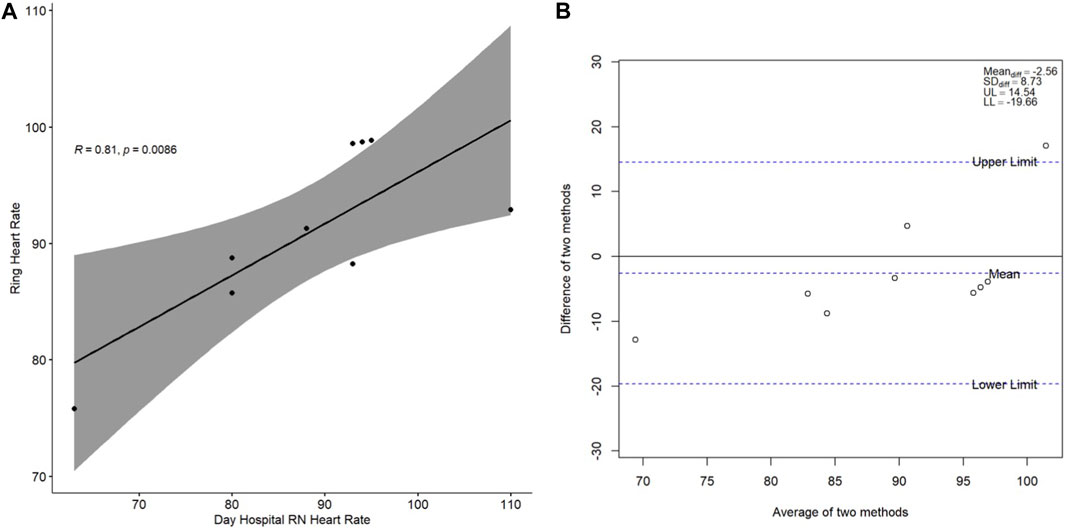
Figure 2. (A) Spearman correlation of ring-obtained heart rate in relation to Day Hospital nurseobtained heart rate; (B) Bland-Altman plot of the difference between the two heart rate measurement methods (y-axis) in relation to the average of the two heart rate measurement methods (x-axis). Heart rate is presented in beats per minute. Abbreviations: RN, nurse; R, Spearman correlation coefficient; Meandiff, mean of difference; SDdiff, standard deviation of difference; UL, upper limit; LL, lower limit.
The cross-sectional correlation plot and Bland-Altman plot of the measures of agreement between respiratory rate as measured by the Day Hospital nurse and respiratory rate as measured by the wristband alone on study Day 1 are presented in Figure 3. Overall, when compared with the cross-sectional heart rate results, the correlation for respiratory rate was weak (r = 0.15, p = 0.74) with modest cross-sectional agreement overall (mean difference of −3.05 ± 4.04 breaths/min; Figure 3B) and a difference observed when compared with nurse-obtained respiratory rate which did not reach statistical significance (p = 0.09).
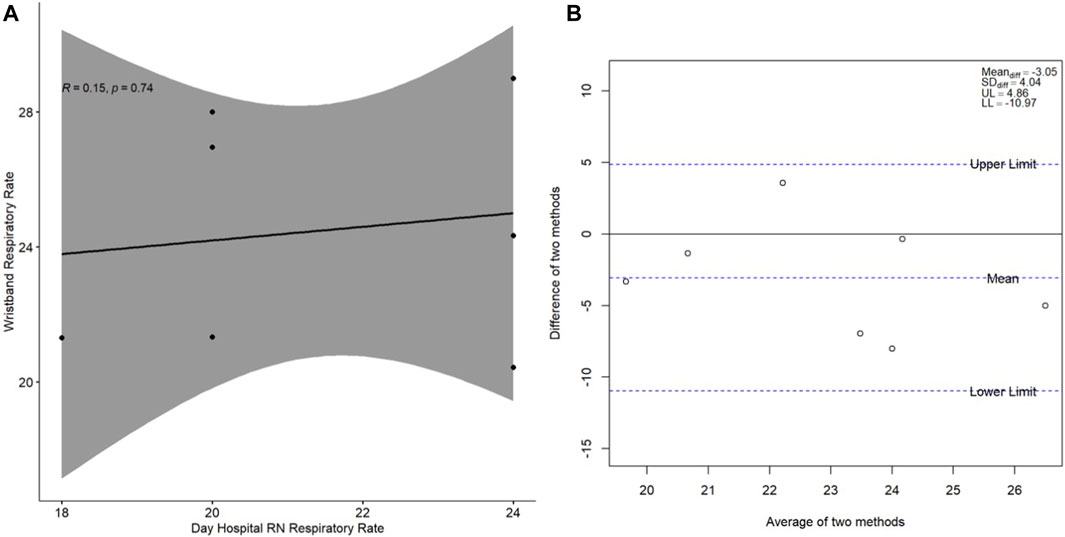
Figure 3. (A) Spearman correlation of wristband-obtained respiratory rate in relation to Day Hospital nurse-obtained respiratory rate; (B) Bland-Altman plot of the difference between the two respiratory rate measurement methods (y-axis) in relation to the average of the two respiratory rate measurement methods (x-axis). Respiratory rate is presented in breaths per minute. Abbreviations: RN, nurse; R, Spearman correlation coefficient; Meandiff, mean of difference; SDdiff, standard deviation of difference; UL, upper limit; LL, lower limit.
The longitudinal correlation plots between wristband-derived and ring-derived heart rate and respiratory rate are presented in Figure 4 and Figure 5, respectively. Strong, statistically significant inter-device correlations were observed for heart rate (r = 0.86, p < 0.001) and for respiratory rate correlation (r = 0.65, p < 0.001).
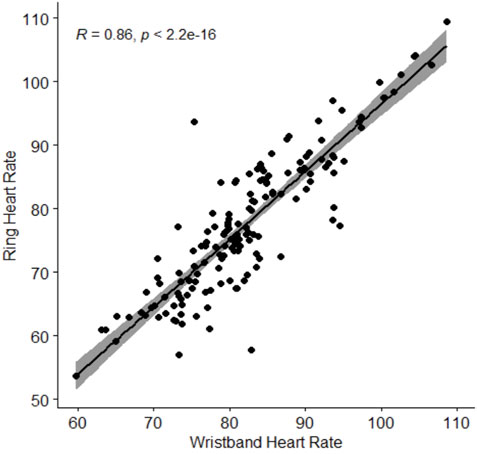
Figure 4. Spearman correlation of ring-obtained heart rate in relation to wristband-obtained heart rate. Heart rate is presented in beats per minute. Abbreviations: R, Spearman correlation coefficient.
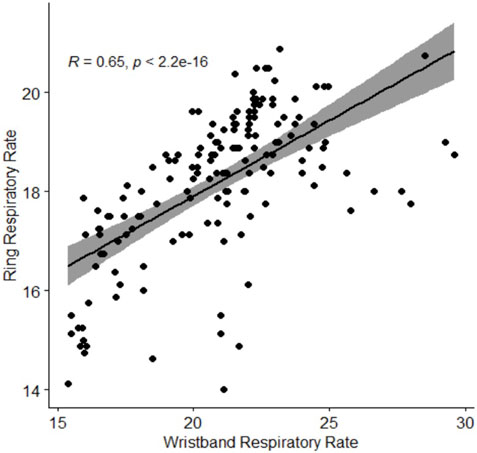
Figure 5. Spearman correlation of ring-obtained respiratory rate in relation to wristbandobtained respiratory rate. Respiratory rate is presented in breaths per minute. Abbreviations: R, Spearman correlation coefficient.
Lastly, end-of-study usability scores were excellent for both devices (Table 2). SUS scores for the wristband and ring were 86.4/100 and 89.2/100, respectively.
4 Discussion
The focus of this prospective, predefined feasibility study was to determine the ability of patients with severe COPD to successfully collect and transmit outpatient physiological data obtained from two wearable biometric devices, while experiencing a current acute exacerbation, in a remote and autonomous manner from their home environment, for 21 consecutive days. Total ambulatory wear time was observed to be very high for both the wristband and the ring, indicative of excellent wearables adherence. The percentage of useable vital sign data was high for wearable device-obtained heart rate (wristband and ring) and for skin temperature (wristband), however, was notably low for wristband-obtained respiratory rate. In secondary analyses on cardiorespiratory parameters, while device-obtained heart rate correlation was generally strong with nurse-obtained heart rate and was even stronger in magnitude with inter-device (wristband-ring) comparisons, cross-sectional respiratory rate correlation was notably weak with nurse-obtained respiratory rate, however, was strong in magnitude in inter-device comparisons. Lastly, user experience scores were excellent for both the wristband and the ring as rated by the COPD participants in this study.
4.1 Feasibility of wearable technology use in the study population
Total ambulatory wear time, a co-primary outcome in the present study, was excellent for the two biometric wearable devices over a multi-week (21 consecutive days) follow-up period. This is novel and notable for a number of reasons. Firstly, COPD is a complex disease and generally manifests in older adults, and study participants in the present study were indeed older adults. Limited knowledge, experience, and comfort level with technology has been associated with older age as well as with lower educational level and lower socioeconomic status (Cimperman et al., 2013; Western et al., 2021; Jiang et al., 2023). These factors, all of which have been consistently reported in the COPD patient population (Townend et al., 2017; Williams et al., 2024), can represent important barriers to participation and inclusion in e-health, telehealth, and technology-based innovations to care. Despite these important challenges, participants in the present study demonstrated excellent autonomy and a clear capacity to independently operate wearable technology and to record and upload physiologic data in a remote manner after only 1 single training session which occurred at the time of initial recruitment. Secondly, the time labeled in the present study as “non-wear” time actually encompassed necessary activities such as device re-charging in order for the equipment to remain functional over the multi-week study protocol, and therefore the total device wear time reported herein is actually likely an underrepresentation of feasibility and adherence in these COPD participants. Thirdly, a mandatory inclusion criterion of the present study was that all participants were experiencing an acute exacerbation at the time of recruitment. Thus, not only did all included participants have “severe” or “very severe” COPD, but moreover these participants also demonstrated the capacity to interact with and successfully operate these technologies while being in an acutely unwell condition. These study features strongly support the feasibility for subsequent remote patient monitoring healthcare interventions in patients with COPD given that included participants were older adults, had more severe forms of COPD, were in an acutely unwell condition, and were still able to collect and transmit data for several weeks at a time.
4.2 Heart rate: Percent of useable data and correlations
The percentage of useable data, a co-primary outcome in the present study, was observed to be very high for heart rate for both of the two biometric wearable devices. Regarding cross-sectional and longitudinal correlations (secondary outcomes), a stronger correlation was observed from the ring than from the wristband with the nurse-obtained heart rate in cross-sectional analyses. This outcome was unexpected considering the fact that the wristband collected heart rate data every 1 min while the ring collected heart rate only every 5 min, and furthermore given the wristband’s research-oriented design compared to the ring’s commercial consumer-facing focus. The anatomic location of wear, while potentially less cumbersome on the wrist than on the finger (as evidenced by the modestly better total wear time of the wristband compared with the ring) may be one relevant contributor overall monitoring quality; the fact that rings were fitted precisely to each individual participant based on measured ring size may also be contributory. Notably, however, the strongest heart rate correlation was observed between the wristband-derived and ring-derived (i.e., inter-device) measurements. This latter finding calls into question whether the current clinical comparator, a single set of clinical vital signs, should actually even serve as the reference “gold-standard” and moreover points to the possibility that current standard-of-care methods (single, infrequent vital signs checks) can stand to be improved. Remote longitudinal patient chronic disease management with the use of a wearables-based near-continuous sampling method appears to not only be possible but moreover may emerge as a preferred approach as these technologies continue to evolve and become increasingly incorporated into traditional models of healthcare delivery.
4.3 Respiratory rate: Percent of useable data and correlations
The percentage of useable data was observed to be low for respiratory rate as obtained from the wearable wristband in the present study. Furthermore, cross-sectional correlation between wristband-obtained respiratory rate and the current healthcare gold standard of respiratory rate obtained from one conventional set of clinical vital signs was found to be poor. Respiratory rate, often referred to as “the forgotten vital sign” (Parkes, 2011; Loughlin et al., 2018), is a highly clinically relevant and important vital sign which can indicate pathophysiological conditions such as respiratory or metabolic acidosis, hypoxia, and hypercapnia (Flenady et al., 2017). Respiratory rate has been shown to be an early indicator of clinical deterioration, and derangements in this vital sign appears to be particularly important in the early detection and timely diagnosis of new ECOPDs (Yañez et al., 2012; Borel et al., 2015). In real-world frontline clinical practice, however, respiratory rate has been shown to be nearly universally under-measured and/or sub-optimally measured given that busy healthcare workers must balance their very limited time on a variety of critical patient-related tasks for a large number of patients (Flenady et al., 2017; Palmer et al., 2023). A study which investigated vital sign monitoring practices in 441 patient interactions across two inpatient wards, notably one of which was a respiratory ward, demonstrated that while oxygen saturation and body temperature were taken 95% and 87% of the time, respectively, across the 229 instances where vital signs were performed, respiratory rate was only measured 22% of the time (Cardona-Morrell et al., 2016). Secondly, even though infrequent sets of point-of-care vital signs (typically spaced 8 h apart as an inpatient, and typically spaced three or more months apart as an outpatient) taken in unnatural/stressful environments for the patient such as in an ambulatory clinic, Day Hospital or Emergency Department, remains the current clinical standard of care, clinical practice guidelines increasingly highlight the clear importance of near-continuous ambulatory monitoring in less stressful/artificial settings to achieve a more accurate diagnosis. For example, the latest Hypertension Canada Comprehensible Guidelines favour blood pressure monitoring using a validated device worn by the patient for a 24-h ambulatory period, where the measurements are taken at 20- to 30-min intervals, rather than a single set taken in the clinic, as the preferred out-of-office method for the diagnosis of hypertension (Rabi et al., 2020). Notably, in the present study a pattern of highly repeated and predictable respiratory rate estimations were observed across the nine study participants during chart review (recorded either as exactly “20 breaths/minute” or as exactly “24 breaths/minute” in 78% of all participants) compared with the unique values observed to be obtained from the wearable wristband (ranging from 15 breaths/min to 29 breaths/min across the nine study participants). Furthermore, while wristband-obtained respiratory rate demonstrated poor correlation with the clinical set of vital signs, strong correlation was observed between wristband-obtained and ring-obtained (inter-device) respiratory rate akin to what was observed with heart rate. It is therefore possible that increasingly sophisticated wearable biometric devices may become the preferred method to monitor respiratory rate and other important vital signs remotely in the patient’s unperturbed condition (i.e., in their “natural” home environment) in a manner that is more accurate and that eases the limited time constraints of busy healthcare workers. Further innovations in hardware and software to definitively overcome large numbers of observations lost to artefact and non-high quality, as was observed with the low percentage of useable respiratory rate data from the wristband in the present study (roughly 1/6 of all minute-by-minute recordings over the 21-day observation period) would enhance and facilitate eventual adoption into real-world clinical practice.
4.4 COPD user experience with wearable technologies
The individual user experience with wearable biometric technology, a secondary outcome in the present study, was reported to be excellent for both the wristband and the ring. A series of qualitative and quantitative studies (Wu et al., 2018; Wu et al., 2019; Wu et al., 2021) have demonstrated that the COPD patient population is keen to engage with and participate in ambulatory monitoring interventions and that they view these advancements as generally positive. The concept of near-continuous monitoring, and of alerts which may inform the patient or the treating team, was reported to be reassuring. Recent work has also demonstrated the encouraging feasibility of wearables-based remote patient monitoring in the COPD patient population and the user experience with these technologies, even around the time of exacerbations. Hawthorne et al. observed that patients with COPD were able to use a biometric wearable vest following hospitalization for an ECOPD, however notably study participants reported feelings of restriction and breathlessness from the chosen study vest (Hawthorne et al., 2022). Even more discomfort and sensations of being physically constrained was reported with other wearable shirts and straps tested in a nested patient and public involvement exercise within and preceding that same study. Furthermore, overnight vital sign measurement was not possible, nor was any measurement of oxygen saturation, with that study vest. This outlines the importance of the selection of a device or combination of devices that prioritizes not only collecting the physiologic parameter(s) of highest clinical relevance with acceptably high quality, but moreover of also selecting device(s) based on patient comfort and with a positive, favourable user experience as was observed in the present study.
4.5 Study limitations
There are a number of important limitations that need to be considered when interpreting the results of the present study. Firstly, while the combination of wearable devices did cumulatively allow for the ambulatory detection of the three vital signs as included in the new ECOPD classification criteria (respiratory rate, heart rate, and oxygen saturation), non-redundancy/non-overlap of the parameters measured between wristband and ring (i.e., the wristband did not measure oxygen saturation) and the time delay in the first useable ring nightly measurements (i.e., study Day 2) precluded inter-device longitudinal comparisons as well as comparison with baseline clinical vital signs of oxygen saturation in the present study. Secondly, although the two wearable devices selected were capable of cumulatively measuring a broad range of parameters in a complementary manner (when non-overlapping) and provided concordant data (when overlapping), the distinct and highly heterogeneous methods of each device regarding the frequency of sampling, the length of time over which data is averaged and made available in a parameter-specific way, the handling of artefacts and data quality issue thresholds, and so on, significantly limit the ability of clinical researchers in general to optimize and individualize the functionality of wearables-based clinical remote patient monitoring platforms in a manner that is best suited to the adult COPD patient population. Indeed, as has been described very effectively in this literature, rather than devices which are deliberately designed and produced for patients with COPD, active research in this field is limited by having to make use of what is commercially available and largely targeted towards average healthy adult consumers in order to then tailor platforms as best as possible toward the COPD target population (Hawthorne et al., 2022). Thirdly, since the focus of the present study was on feasibility rather than on patient engagement and self-management, the results of the biometric data obtained by the wearable devices were intentionally not shared with study participants, though this feature is possible to unlock with the devices and interfaces used. Future studies in which remote monitoring platforms are developed and refined should include feedback functionality for the participant which, combined with self-management education (which is a standard of excellent chronic disease management in COPD) (Bourbeau et al., 2004; Bourbeau and Palen, 2009; GOLD, 2024), would greatly enhance the clinical utility of these platforms. Indeed, semi-structured interviews with patients with COPD have indicated a strong preference towards being able to receive direct feedback from wearables-based platforms, such that they can feel empowered to better engage in and manage their own chronic condition in partnership with their treating team (Wu et al., 2019). Finally, this was a small feasibility study which was not informed by any sample size calculations regarding primary or secondary outcome analyses, which leaves open the possibility of an underpowering of any and/or all of the inferential statistical tests performed. However, this intentional lack of an a priori sample size estimation in the present prespecified study is well-supported by best practices in the design, conduct and purpose of pilot and feasibility studies (Lancaster, 2015; Eldridge et al., 2016).
4.6 Summary
In conclusion, the current landscape of remote patient monitoring has been defined by rapid evolution and incredible innovations in technology as well as by sizeable and persisting challenges to clinical implementation and real-world application (Subramanian et al., 2004; Gottlieb et al., 2014; Iranpak et al., 2021; Coutu et al., 2023). The present study provides important foundational evidence for the autonomous operation of non-invasive, comfortable wearable devices including excellent adherence to device wear and effective ambulatory data collection in a near-continuous manner and remote transmission, for several weeks at a time, by older patients with severe forms of COPD experiencing a current exacerbation. This autonomy and capacity was notably observed in the context of a very favourable reported user experience by COPD participants. The feasibility demonstrated in this complex COPD patient subpopulation is encouraging, and supports a broad applicability of the study findings to the highest-risk patients in real-world clinical practice. Important future areas of research include developing a better understanding of the detailed pathophysiology of exacerbations of COPD in the unperturbed “natural” outpatient environment, developing functional remote patient monitoring platforms which are capable of the early detection of new exacerbations in a prospective manner, and testing these platforms against the current standard of care in order to demonstrate the tangible clinical benefits of remote patient monitoring solutions and their timely incorporation into the healthcare system.
4.7 Take-home points
➢ COPD is a highly prevalent chronic disease and exacerbations of COPD are a leading cause of adult hospitalization, representing an important opportunity for the investigation and development of wearable technology-based remote patient monitoring solutions.
➢ Included participants, older adults with “severe” and “very severe” COPD experiencing a current exacerbation, demonstrated excellent autonomous device wear and data collection/transmission over several weeks from the home environment and reported a highly favourable user experience with wearable technologies.
➢ Closer correlations were observed in inter-device comparisons than in device versus “gold standard” comparisons, supporting a paradigm shift in chronic disease management away from infrequent in-person vital sign checks and towards near-continuous remote home monitoring strategies.
Data availability statement
The original contributions presented in the study are included in the article/Supplementary Material, further inquiries can be directed to the corresponding author.
Ethics statement
The studies involving humans were approved by Research Ethics Board (REB) of the McGill University Health Centre (MUHC). The studies were conducted in accordance with the local legislation and institutional requirements. The participants provided their written informed consent to participate in this study.
Author contributions
OI: Conceptualization, Methodology, Visualization, Writing–original draft, Writing–review and editing. F-AC: Writing–review and editing. DM: Writing–review and editing. BR: Conceptualization, Formal Analysis, Methodology, Supervision, Visualization, Writing–review and editing.
Funding
The author(s) declare financial support was received for the research, authorship, and/or publication of this article. This study was funded by the McGill University Health Centre (MUHC) Department of Medicine Contract Academic Staff (CAS) Research Award.
Acknowledgments
The authors thank Isabelle Drouin, COPD Nurse at the Montreal Chest Institute of the MUHC, for her many contributions toward this study.
Conflict of interest
BAR has received Honoraria from the Canadian Thoracic Society as a CTS—presenter and educational content creator, is an educational content creator CHEST and Respiplus non-profit, an educational webinar presenter for Alberta Kinesiology Association, an APPQ—presenter Association des Pneumologues de la Province du Québec, an CPD—presenter McGill University Continuing Professional Development and a educational session moderator for GSK, has a COPD Speakership for COVIS and AZ. BAR has also received Research funding as Principal Investigator from the McGill University Health Centre (MUHC) Department of Medicine Contract Academic Staff (CAS) Research Award, the Quebec Respiratory Health Network (QHRN), the Ministére de l’Éducation et le Ministère de l’Enseignement Supérieur Innovation and Partnership Program (McGill University and Thorasys, Inc.), the MUHC Foundation/MCI Respiratory Research Campaign Innovation Grant, and the McGill Initiative in Infection and Immunity (MI4) Investigator Award.
The remaining authors declare that the research was conducted in the absence of any commercial or financial relationships that could be construed as a potential conflict of interest.
Publisher’s note
All claims expressed in this article are solely those of the authors and do not necessarily represent those of their affiliated organizations, or those of the publisher, the editors and the reviewers. Any product that may be evaluated in this article, or claim that may be made by its manufacturer, is not guaranteed or endorsed by the publisher.
References
Aliverti, A. (2017). Wearable technology: role in respiratory health and disease. Breathe 13, e27–e36. doi:10.1183/20734735.008417
Al Rajeh, A. M., and Hurst, J. R. (2016). Monitoring of physiological parameters to predict exacerbations of chronic obstructive pulmonary disease (COPD): a systematic review. J. Clin. Med. 5, 108. doi:10.3390/jcm5120108
Amegadzie, J. E., Lee, T. Y., Sadatsafavi, M., Lynd, L. D., Sin, D. D., and Johnson, K. M. (2023). Trends in hospital admissions for chronic obstructive pulmonary disease over 16 years in Canada. CMAJ 195, e1172–e1179. doi:10.1503/cmaj.221051
Boers, E., Barrett, M., Su, J. G., Benjafield, A. V., Sinha, S., Kaye, L., et al. (2023). Global burden of chronic obstructive pulmonary disease through 2050. JAMA Netw. Open 6, e2346598. doi:10.1001/jamanetworkopen.2023.46598
Borel, J. C., Pelletier, J., Taleux, N., Briault, A., Arnol, N., Pison, C., et al. (2015). Parameters recorded by software of non-invasive ventilators predict COPD exacerbation: a proof-of-concept study. Thorax 70, 284–285. doi:10.1136/thoraxjnl-2014-206569
Bourbeau, J., Nault, D., and Dang-Tan, T. (2004). Self-management and behaviour modification in COPD. Patient Educ. Couns. 52, 271–277. doi:10.1016/s0738-3991(03)00102-2
Bourbeau, J., and Palen, J. van der (2009). Promoting effective self-management programmes to improve COPD. Eur. Respir. J. 33, 461–463. doi:10.1183/09031936.00001309
Cardona-Morrell, M., Prgomet, M., Lake, R., Nicholson, M., Harrison, R., Long, J., et al. (2016). Vital signs monitoring and nurse–patient interaction: a qualitative observational study of hospital practice. Int. J. Nurs. Stud. 56, 9–16. doi:10.1016/j.ijnurstu.2015.12.007
Celli, B. R., Fabbri, L. M., Aaron, S. D., Agusti, A., Brook, R., Criner, G. J., et al. (2021). An updated definition and severity classification of chronic obstructive pulmonary disease exacerbations: the Rome proposal. Am. J. Respir. Crit. Care Med. 204, 1251–1258. doi:10.1164/rccm.202108-1819pp
Cimperman, M., Brenčič, M. M., Trkman, P., and Stanonik, M. de L. (2013). Older adults’ perceptions of home telehealth services. Telemed. J. E Health 19, 786–790. doi:10.1089/tmj.2012.0272
Cohen, J. (1988). Statistical power analysis for the behavioral sciences. 2nd ed. Hillsdale, N.J: L. Erlbaum Associates, 567.
Coutu, F. A., Iorio, O. C., and Ross, B. A. (2023). Remote patient monitoring strategies and wearable technology in chronic obstructive pulmonary disease. Front. Med. 10, 1236598. doi:10.3389/fmed.2023.1236598
Dechman, G., Aceron, R., Beauchamp, M., Bhutani, M., Bourbeau, J., Brooks, D., et al. (2020). Delivering pulmonary rehabilitation during the COVID-19 pandemic: a Canadian Thoracic Society position statement. Can. J. Respir. Crit. Care Sleep. Med. 4, 232–235. doi:10.1080/24745332.2020.1828683
Eden, S. K., Li, C., and Shepherd, B. E. (2022). Nonparametric estimation of Spearman’s rank correlation with bivariate survival data. Biometrics 78, 421–434. doi:10.1111/biom.13453
Eldridge, S. M., Chan, C. L., Campbell, M. J., Bond, C. M., Hopewell, S., Thabane, L., et al. (2016). CONSORT 2010 statement: extension to randomised pilot and feasibility trials. BMJ 355, i5239. doi:10.1136/bmj.i5239
Flenady, T., Dwyer, T., and Applegarth, J. (2017). Accurate respiratory rates count: so should you. Australas. Emerg. Nurs. J. 20, 45–47. doi:10.1016/j.aenj.2016.12.003
Ford, E. S. (2015). Hospital discharges, readmissions, and ED visits for COPD or bronchiectasis among US adults. Chest 147, 989–998. doi:10.1378/chest.14-2146
GOLD (2024). Global initiative for chronic obstructive lung disease - GOLD. Available at: https://goldcopd.org/2024-gold-report/.
Gottlieb, M., Marsaa, K., Andreassen, H., Strømstad, G., and Godtfredsen, N. (2014). Feasibility of a telecare solution for patients admitted with COPD exacerbation: screening data from a pulmonary ward in a university hospital. Eur. Clin. Respir. J. 1, 24193. doi:10.3402/ecrj.v1.24193
Hawthorne, G., Greening, N., Esliger, D., Briggs-Price, S., Richardson, M., Chaplin, E., et al. (2022). Usability of wearable multiparameter technology to continuously monitor free-living vital signs in people living with chronic obstructive pulmonary disease: prospective observational study. JMIR Hum. Factors 9, e30091. doi:10.2196/30091
Iranpak, S., Shahbahrami, A., and Shakeri, H. (2021). Remote patient monitoring and classifying using the internet of things platform combined with cloud computing. J. Big Data 8, 120. doi:10.1186/s40537-021-00507-w
Jiang, Y., Gao, J., Sun, P., Nan, J., Zou, X., Sun, M., et al. (2023). Factors associated with the e-health literacy among older adults with chronic obstructive pulmonary disease: a cross-sectional study. Telemed. E-Health. doi:10.1089/tmj.2023.0394
Karun, K. M., and Puranik, A. (2021). BA.plot: an R function for Bland-Altman analysis. Clin. Epidemiol. Glob. Health 12, 100831. doi:10.1016/j.cegh.2021.100831
Keogh, A., Dorn, J. F., Walsh, L., Calvo, F., and Caulfield, B. (2020). Comparing the usability and acceptability of wearable sensors among older Irish adults in a real-world context: observational study. JMIR MHealth UHealth 8, e15704. doi:10.2196/15704
Lancaster, G. A. (2015). Pilot and feasibility studies come of age. Pilot Feasibility Stud. 1, 1. doi:10.1186/2055-5784-1-1
Laue, J., Melbye, H., and Risør, M. B. (2017). Self-treatment of acute exacerbations of chronic obstructive pulmonary disease requires more than symptom recognition – a qualitative study of COPD patients’ perspectives on self-treatment. BMC Fam. Pract. 18, 8. doi:10.1186/s12875-017-0582-8
Lewis, J. R., and Sauro, J. (2009). “The factor structure of the system usability scale,” in Human centered design. Editor M. Kurosu (Berlin, Heidelberg: Springer Berlin Heidelberg), 5619, 94–103.
Liang, J., Xian, D., Liu, X., Fu, J., Zhang, X., Tang, B., et al. (2018). Usability study of mainstream wearable fitness devices: feature analysis and system usability scale evaluation. JMIR MHealth UHealth 6, e11066. doi:10.2196/11066
Liaqat, D., Abdalla, M., Abed-Esfahani, P., Gabel, M., Son, T., Wu, R., et al. (2019). WearBreathing: real world respiratory rate monitoring using smartwatches. Proc. ACM Interact. Mob. Wearable Ubiquitous Technol. 3, 1–22. doi:10.1145/3328927
Loughlin, P. C., Sebat, F., and Kellett, J. G. (2018). Respiratory rate: the forgotten vital sign—make it count. Jt. Comm. J. Qual. Patient Saf. 44, 494–499. doi:10.1016/j.jcjq.2018.04.014
Navathe, A. S., Crowley, A., and Liao, J. M. (2022). Remote patient monitoring—will more data lead to more health? JAMA Intern Med. 182, 1007–1008. doi:10.1001/jamainternmed.2022.3040
Palmer, J. H., James, S., Wadsworth, D., Gordon, C. J., and Craft, J. (2023). How registered nurses are measuring respiratory rates in adult acute care health settings: an integrative review. J. Clin. Nurs. 32, 4515–4527. doi:10.1111/jocn.16522
Parkes, R. (2011). Rate of respiration: the forgotten vital sign. Emerg. Nurse J. RCN Accid. Emerg. Nurs. Assoc. 19, 12–17. doi:10.7748/en2011.05.19.2.12.c8504
The R project for statistical computing (2023). Available at: https://www.rproject.org/.
Rabi, D. M., McBrien, K. A., Sapir-Pichhadze, R., Nakhla, M., Ahmed, S. B., Dumanski, S. M., et al. (2020). Hypertension Canada’s 2020 comprehensive guidelines for the prevention, diagnosis, risk assessment, and treatment of hypertension in adults and children. Can. J. Cardiol. 36, 596–624. doi:10.1016/j.cjca.2020.02.086
Rodriguez-Roisin, R. (2000). Toward a consensus definition for COPD exacerbations. Chest 117, 398S–401S. doi:10.1378/chest.117.5_suppl_2.398s
Rubio, N., Parker, R. A., Drost, E. M., Pinnock, H., Weir, C. J., Hanley, J., et al. (2017). Home monitoring of breathing rate in people with chronic obstructive pulmonary disease: observational study of feasibility, acceptability, and change after exacerbation. Int. J. Chron. Obstruct Pulmon Dis. 12, 1221–1231. doi:10.2147/copd.s120706
Safiri, S., Carson-Chahhoud, K., Noori, M., Nejadghaderi, S. A., Sullman, M. J. M., Heris, J. A., et al. (2022). Burden of chronic obstructive pulmonary disease and its attributable risk factors in 204 countries and territories, 1990-2019: results from the Global Burden of Disease Study 2019. BMJ 378, e069679. doi:10.1136/bmj-2021-069679
Scioscia, G., Blanco, I., Arismendi, E., Burgos, F., Gistau, C., Barbaro, M. P. F., et al. (2017). Different dyspnoea perception in COPD patients with frequent and infrequent exacerbations. Thorax 72, 117–121. doi:10.1136/thoraxjnl-2016-208332
Seemungal, T. A., Donaldson, G. C., Paul, E. A., Bestall, J. C., Jeffries, D. J., and Wedzicha, J. A. (1998). Effect of exacerbation on quality of life in patients with chronic obstructive pulmonary disease. Am. J. Respir. Crit. Care Med. 157, 1418–1422. doi:10.1164/ajrccm.157.5.9709032
Soler-Cataluña, J. J., Martínez-García, M. A., Román Sánchez, P., Salcedo, E., Navarro, M., and Ochando, R. (2005). Severe acute exacerbations and mortality in patients with chronic obstructive pulmonary disease. Thorax 60, 925–931. doi:10.1136/thx.2005.040527
Soon, S., Svavarsdottir, H., Downey, C., and Jayne, D. G. (2020). Wearable devices for remote vital signs monitoring in the outpatient setting: an overview of the field. BMJ Innov. 6, 55–71. doi:10.1136/bmjinnov-2019-000354
Stanojevic, S., Beaucage, F., Comondore, V., Faughnan, M., Kovesi, T., McCoy, C., et al. (2022). Resumption of pulmonary function testing during the COVID-19 pandemic. Can. J. Respir. Crit. Care Sleep. Med. 6, 78–81. doi:10.1080/24745332.2021.2010478
Subramanian, U., Hopp, F., Lowery, J., Woodbridge, P., and Smith, D. (2004). Research in home-care telemedicine: challenges in patient recruitment. Telemed. J. E-Health Off. J. Am. Telemed. Assoc. 10, 155–161. doi:10.1089/tmj.2004.10.155
Townend, J., Minelli, C., Mortimer, K., Obaseki, D. O., Ghobain, M. A., Cherkaski, H., et al. (2017). The association between chronic airflow obstruction and poverty in 12 sites of the multinational BOLD study. Eur. Respir. J. 49, 1601880. doi:10.1183/13993003.01880-2016
Wedzicha, J. A., and Seemungal, T. A. (2007). COPD exacerbations: defining their cause and prevention. Lancet Lond Engl. 370, 786–796. doi:10.1016/s0140-6736(07)61382-8
Western, M. J., Armstrong, M. E. G., Islam, I., Morgan, K., Jones, U. F., and Kelson, M. J. (2021). The effectiveness of digital interventions for increasing physical activity in individuals of low socioeconomic status: a systematic review and meta-analysis. Int. J. Behav. Nutr. Phys. Act. 18, 148. doi:10.1186/s12966-021-01218-4
Williams, P. J., Buttery, S. C., Laverty, A. A., and Hopkinson, N. S. (2024). Lung disease and social justice – COPD as a manifestation of structural violence. Am. J. Respir. Crit. Care Med. doi:10.1164/rccm.202309-1650ci
Wu, F., Burt, J., Chowdhury, T., Fitzpatrick, R., Martin, G., Scheer, J. W. van der, et al. (2021). Specialty COPD care during COVID-19: patient and clinician perspectives on remote delivery. BMJ Open Respir. Res. 8, e000817. doi:10.1136/bmjresp-2020-000817
Wu, R., Liaqat, D., Lara, E. de, Son, T., Rudzicz, F., Alshaer, H., et al. (2018). Feasibility of using a smartwatch to intensively monitor patients with chronic obstructive pulmonary disease: prospective cohort study. JMIR MHealth UHealth 6, e10046. doi:10.2196/10046
Wu, R. C., Ginsburg, S., Son, T., and Gershon, A. S. (2019). Using wearables and self-management apps in patients with COPD: a qualitative study. ERJ Open Res. 5, 00036–02019. doi:10.1183/23120541.00036-2019
Keywords: chronic obstructive pulmonary disease, exacerbations of COPD, remote patient monitoring, vital signs, respiratory rate, heart rate, wearable devices, wearable technology
Citation: Iorio OC, Coutu F-A, Malaeb D and Ross BA (2024) Feasibility, functionality, and user experience with wearable technologies for acute exacerbation monitoring in patients with severe COPD. Front. Sig. Proc. 4:1362754. doi: 10.3389/frsip.2024.1362754
Received: 28 December 2023; Accepted: 19 February 2024;
Published: 19 March 2024.
Edited by:
Shaoxiong Sun, The University of Sheffield, United KingdomReviewed by:
Jianan Li, Worcester Polytechnic Institute, United StatesColin K. Drummond, Case Western Reserve University, United States
Copyright © 2024 Iorio, Coutu, Malaeb and Ross. This is an open-access article distributed under the terms of the Creative Commons Attribution License (CC BY). The use, distribution or reproduction in other forums is permitted, provided the original author(s) and the copyright owner(s) are credited and that the original publication in this journal is cited, in accordance with accepted academic practice. No use, distribution or reproduction is permitted which does not comply with these terms.
*Correspondence: Bryan A. Ross, YnJ5YW4ucm9zc0BtY2dpbGwuY2E=