- 1Department of Electrical Engineering, Indian Institute of Technology Indore, Indore, India
- 2The LNM Institute of Information Technology, Jaipur, India
- 3Department of Electronics Engineering, Indian Institute of Technology (IIT) BHU, Varanasi, India
Reflecting Intelligent Surfaces (RISs) are reshaping the landscape of wireless communications, particularly in the terahertz (THz) frequency bands, offering promising solutions to inherent challenges in the bands. THz communication boasts bandwidths exceeding 100 GHz, leading to data rates potentially in the terabits per second (Tbps) range, thereby making it an attractive proposition for wireless communications, imaging, and sensing. However, benefits come with challenges, including significant molecular absorption, scattering, diffraction, and hardware limitations. Moreover, as bandwidth in the THz range increases, so does the difficulty of signal processing at Nyquist rate. RIS emerges as a game-changer for 6G and beyond by providing programmable reflecting elements that can adaptively modify the phases and amplitudes of incident signals, enabling precision in directing THz waves and enhancing received signal strength. Such capabilities can significantly mitigate path loss and atmospheric absorption challenges. Furthermore, inherent pencil beamforming capabilities of RIS lead to optimized energy utilization. Major challenge in THz communications is the pressing needs for efficient algorithms for robust THz transceivers and optimizing RIS elements. This review describes the integration of RIS and near-field THz communications, highlighting their future potential and challenges for the next-generation wireless networks. In this article, a comprehensive understanding of the complexities and nuances of near-field propagation in 6G networks, especially as the technology shifts towards extremely large-scale antenna arrays (ELAA). Additionally, it will introduce the transformative potential of sub-Nyquist rate signal processing and artificial intelligence (AI) offering innovative solutions to address the inherent challenges of 6G communication, especially in channel estimation and beamforming strategies.
1 Introduction
Forthcoming sixth generation (6G) mobile networks are anticipated to enable a variety of new applications, ranging from holographic videos to digital replicas. To support these applications, significant research strides have been undertaken to pave the way for wireless technologies that exceed the fifth generation (5G) performance benchmarks Ali et al. (2020), Cui et al. (2023). For instance, innovations such as ultra-massive multiple-input multiple-output (UM-MIMO) and cell-free massive MIMO (CF-MIMO) are poised to achieve a tenfold increase in spectral efficiency for 6G, given their inherent spatial multiplexing and beamforming advantages Ali et al. (2020). Adding to this, the reconfigurable intelligent surface (RIS), which can dynamically adjust the wireless environment via hundreds or thousands of small elements, heralds novel approaches for augmenting capacity and coverage Cui et al. (2023). Furthermore, leveraging millimeter-wave (mmWave) and terahertz (THz) frequencies, UM-MIMO promises to unlock vast spectral reserves, potentially enabling peak data rates that are a hundredfold higher in 6G communications Nie et al. (2019), She et al. (2021).
A pivotal factor across these technologies, ranging from UM-MIMO to THz communications, is the necessity for a substantially larger number of antennas to maximize their potential. This implies that extremely large-scale antenna arrays (ELAA) will become indispensable for 6G’s potential technologies. This leap from 5G’s massive MIMO to 6G’s ELAA is not just about the sheer growth in the number of antennas; it translates to profound shifts in electromagnetic (EM) properties. The EM field is typically categorized into the far-field and near-field regions. This categorization depends on the Rayleigh distance or the Fraunhofer distance Hu et al. (2023), which is a function of the array aperture square and the carrier frequency. Beyond the Rayleigh distance lies the far-field region, characterized by planar wave EM field approximations. Within the Rayleigh distance, the propagation is primarily near-field, necessitating a spherical wave-based EM field model.
Scaling of antennas in the 5G’s massive MIMO meant Rayleigh distance to be of several meters wide, leading to a focus on far-field communication models. However, the increase in antenna counts and carrier frequencies anticipated for 6G signifies that the ELAA’s near-field domain will grow significantly. For instance, for a 2000-elements ELAA operating at 2.4 GHz as described in Cui et al. (2023) with 2 m × 3 m array dimension, its Rayleigh distance stands at approximately 150 m, surpassing a typical 5G cell’s radius, which emphasises the fact that the future 6G networks will need to shift towards near-field THz MIMO communications She et al. (2021). In the near field, the spherical wave propagation model takes precedence over its planar counterpart. The entry into the near-field domain brings forth unique challenges for ELAA setups, which must be pinpointed and tackled to truly harness 6G’s potential.
In this study, we conduct a comprehensive study of recent near-field communication methodologies poised for 6G integration. The highlights of this article include:
• In this review, we delve into the potential of THz communications within the realm of 6G, emphasizing its potential for ultra-high data rates and distinct propagation characteristics. We distinguish between the planar wavefronts of far-field communications, which direct signal energy angularly, and the spherical wavefronts of near-field communications that concentrate energy based on angle and distance. Furthermore, we discuss the Rayleigh distance, marking the transition from far-field to near-field, and extend its traditional definition from basic MIMO channels to RIS-enhanced communication structures.
• Subsequently, we will discuss the emerging field in the THz communication, distinguishing between solutions addressing inherent challenges and those leveraging its intrinsic benefits. Notably, techniques tailored for far-field contexts often underperform in near-field settings due to the transition from planar to spherical wavefront behaviors. This wavefront nonlinearity invalidates assumptions foundational to far-field methods, resulting in performance deficits in near-field scenarios Chen et al. (2023). The strategies in the first group are geared towards mitigating such discrepancies, with a particular emphasis on topics like near-field channel estimation and beamforming. However, the latter group unravels how the specific attributes of the near-field spherical wavefront can be channeled to bolster capacity metrics. A comprehensive discussion, encompassing the underlying principles, current advancements, merits, and demerits of these two streams of research, is presented.
• Moreover, we highlight several unresolved challenges and upcoming research areas in near-field RIS-assisted THz communications. One main point is fine-tuning the Rayleigh distance based on different communication measures. Many researchers believe that, signal processing techniques such as compressed sensing (CS), artificial intelligence (AI) or machine learning (ML) could play a key role in making near-field transmissions more efficient. The same is highlighted as a major technology advancement for the THz RIS-based communications.
1.1 The potential of THz communications
The dawn of the 21st century has witnessed an unprecedented surge in data communication demands due to the rise of internet of things (IoT) devices, autonomous vehicles, and augmented reality (AR), virtual reality (VR) and many more applications. To address the emerging data traffic demands, researchers have set their eyes on exploring higher frequency bands, primarily the THz spectrum Han et al. (2019), Akyildiz et al. (2020). The appeal of this spectrum mostly arises from its extensive range of frequencies, enabling data transmission speeds that surpass 100 Gbps Wu et al. (2023). In Jornet and Akyildiz (2011), authors underscore this capacity by presenting groundbreaking experimental results that highlight THz band’s capability to revolutionize next-generation communication networks. Their work explained that by leveraging the wide THz bands, it is plausible to achieve unparalleled data speeds, facilitating applications like real-time ultra-high-definition video streaming and instantaneous cloud computing. Beyond the merits of higher data rates, the THz spectrum’s unique properties offer novel applications outside traditional communication. For instance, THz’s unique propagation characteristics conducive to applications such as high-resolution imaging, biosensing, and medical diagnostics. These characteristics stem from the wave-matter interactions specific to this band, allowing for precision, which is unattainable in lower frequency domains. However, the massive potential of the THz spectrum does not come without its challenges. Significant molecular absorption, scattering, and diffraction losses pose inherent limitations to THz communications. As described in Han et al. (2015), these losses, especially the pronounced atmospheric absorption at certain frequency windows within the THz band, require innovative techniques for efficient channel utilization. Furthermore, the high frequencies associated with the THz band introduce challenges in signal processing, particularly concerning the Nyquist rate. Traditional Nyquist rate signal processing, which dictates that a signal must be sampled at least twice its highest frequency to be accurately reconstructed, becomes computationally intensive and resource-demanding in the THz domain. This necessitates the exploration of sub-Nyquist sampling techniques and advanced signal processing algorithms to ensure efficient, and accurate data transmission and reception in THz communications Sharma et al. (2021).
In the realm of MIMO communications, the distinction between near-field and far-field regions, as illustrated in Figure 1, is pivotal. The near-field is characterized by spherical wavefronts, governed by the Rayleigh distance, whereas far-fields are dominated by planar waves. When a user equipment (UE) is positioned beyond the Rayleigh distance from a base station (BS) equipped with a large antenna array, it resides in the far-field zone, experiencing planar EM waves. Conversely, within the Rayleigh distance, UE is in the near-field zone, encountering spherical EM waves, reminiscent of ripples in a pond, as depicted in Figure 1.
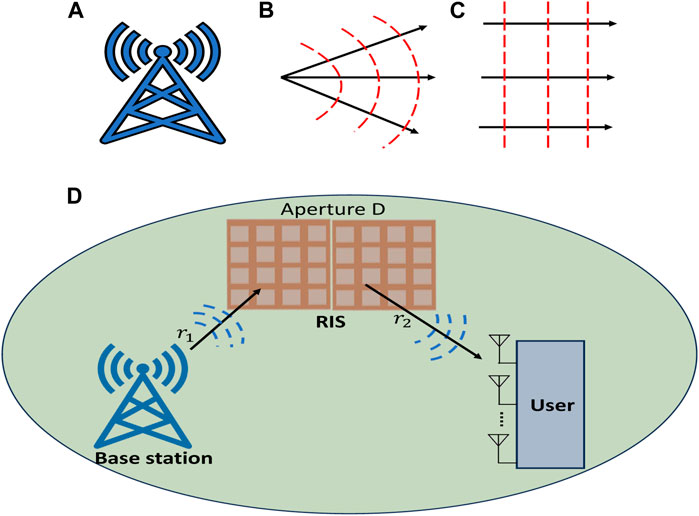
FIGURE 1. (A) Base station with ELAA. (B) Spherical wave front in the near-field scenario. (C) Planar wavefront in the far-field scenario. (D) RIS-assisted THz near-field MIMO system.
Planar waves result from the extension of center-focused waves over vast distances. In distant regions, the phase of these waves can be simplified to a planar form through Taylor expansion, facilitating beamsteering. This process directs energy based on the wave’s angle of arrival. However, the near-field’s wave phase demands intricate calculations, capturing both angle and distance data from the BS to the UE, a phenomenon termed as “beam focusing”. These distinct wave characteristics in the far-fields and near-fields present both challenges and opportunities for THz wireless systems, which will be further explored.
In Figure 1D the IRS-assisted THz near-field MIMO system is shown. The Rayleigh distance, defined as 2D2/λ where D is the antenna array size and λ is the wavelength, specifies the boundary between far-field and near-field regions. In far-fields, a simplified phase model is often employed, which can introduce phase discrepancies, especially at shorter distances. When the phase difference between all antennas of the BS and the UE reaches a threshold
A notable evolution is the adaptation of the Rayleigh distance for RIS-aided THz MIMO systems. In RIS systems, two links are considered: BS-RIS and RIS-UE. Phase differences are influenced by both these distances. If either distance is shorter than the Rayleigh distance, the RIS system operates in the near-field. With the integration of more antennas at elevated carrier frequencies, the near-field reach of large antenna arrays has expanded. For instance, a 0.36 m aperture antenna at 28 GHz offers a near-field range of approximately 25 m in SIMO/MISO configurations. In RIS scenarios, with a 50 m BS-RIS distance, any RIS-UE distance below 50 m categorizes it as near-field Asif Haider and Zhang (2023). These developments underscore the significance of near-field communications in the impending 6G era.
1.2 Challenges and recent developments: State of the art
1.2.1 THz channel estimation and codebooks
For an ELAA to operate with better accuracy, precise channel estimation is vital. Typically, there are fewer channel paths than the number of antennas, thus, efficient channel estimation methods aim to minimize the number of pilot signals sent. This minimization is achieved by simplifying the channel’s representation using codebooks Cui and Dai (2022). In far-field communications, these codebooks are designed around the idea of planar waves, which are linked to specific incident angles. Ideally, each communication path in the far-field can be described by a single codeword. This approach makes the channel representation straightforward since only a few paths exist. Techniques like beam training and CS can then be employed to gauge the far-field channels efficiently. However, these planar-wave-centric approaches are not applicable to near-field scenarios, which are based on spherical waves. A single path needs multiple codewords from the far-field codebook, making the channel representation complex Wei et al. (2022), which can lower the accuracy of channel estimation. Therefore, codebooks specifically designed for the near-field scenario are required.
Researchers have been exploring codebooks that use the concept of spherical wavefronts. One notable study in Wei et al. (2022); Cui and Dai (2022) is based on spherical grid codebook, which divides the whole communication space into grid segments, relating to a specific response from the near-field array. This structured approach captures both the angle and the distance data for each near-field path. The channels are then gauged using CS techniques, minimizing pilot overhead Chen et al. (2023). However, as user devices come closer, near-field communication becomes more pronounced, emphasizing the importance of distance based codebook. This observation indicates that the grid system has lower resolution for distant areas from the ELAA, whereas the codebook is denser for closely located users. Based on this fact, the study in Cui and Dai (2022) highlighted that angle divisions could be uniform, and distance divisions need variations. Essentially, closer the users are, the more detailed the grid should be. This approach allows for more accurate channel estimation across near- and far-field scenarios.
1.2.2 Signal processing challenges
In the domain of near-field THz MIMO communication, signal processing poses a set of unique challenges that are distinct from the conventional communication systems. Firstly, the THz band is characterized by significant molecular absorption and scattering effects, which can introduce non-trivial distortions to the transmitted signals. This necessitates sophisticated signal processing techniques to accurately estimate and compensate for these channel impairments. Secondly, the high-dimensional nature of MIMO systems, combined with the intricate multipath environment of near-field communications, complicates channel estimation. Traditional methods may not be well-suited to capture the channel’s sparsity Sarieddeen et al. (2020). Furthermore, the short wavelengths in the THz band demand precise beam alignment, making beamforming and beam tracking in the near-field scenarios particularly challenging. Additionally, the hardware imperfections and phase noise inherent to THz devices can further exacerbate signal processing complexities. In essence, while near-field THz MIMO communication holds the promise of ultra-high-speed data transmission, it also presents a myriad of signal processing challenges that necessitate innovative solutions for optimal system performance Tarboush et al. (2023).
1.3 Understanding the near-field beam split challenge
1.3.1 Beam split effect
Wireless systems operating in the THz wideband spectrum, especially those using ELAA, there is a phenomenon known as beam split, or sometimes referred to as beam squint or spatial-wideband effect Tan and Dai (2021); Wu et al. (2023). In THz beamforming, analog phase shifters are predominantly employed, offering consistent phase shifts across varied signal frequencies. However, these phase shifters are optimal primarily for narrowband signals, with wide frequency bands experiencing phase errors, resulting in beam splits. The implications of beam squint differ for far-field and near-field regions. In far-fields, different frequencies target distinct angles due to wavelength variations affecting the antenna’s radiation pattern. Conversely, in near-field scenarios, beams diverge in direction and strength, focusing at varying distances, which can misalign with the user’s location, affecting signal energy. While strategies exist to mitigate far-field beam splits, their efficacy diminishes in the near-field domain, posing challenges for THz ELAA communications.
1.3.2 Recent developments
There have been innovative attempts to tackle this near-field beam split challenge, as summarized in Table 1. One approach used a unique chirp sequence to adjust phase shifts, aiming to maintain consistent pencil beam gains across frequencies Myers and Heath (2021). The chirp signals do lessen the near-field beam split to a degree; however, the corresponding spectral efficiency is also reduced, especially for larger bandwidths. In another approach, known as the phase-delay focusing method, the ELAA is split into smaller sub-arrays with its respective RF chain. Frequency-dependent phase shifts are achieved by inserting a true-time-delay (TTD) line in the RF chain of the subarray, allowing the beams to focus precisely on the targeted user location Hao et al. (2023). Comparatively, though the first solution is simpler to execute, its performance leaves room for improvement. The second method can almost mitigate the near-field beam split problem; however, introducing TTD lines in RF chain can be complicated. Thankfully, emerging technology in graphene-based plasmonic waveguides offers promising and affordable ways to implement these TTD lines at higher frequencies, potentially bridging the gap between the theory and practical applications.
2 Exploring the benefits of near-field communications for 6G networks
The emergence of 6G networks is poised to leverage the transformative potential of near-field THz MIMO communications, offering a paradigm shift from traditional far-field methodologies. In far-field systems, the line-of-sight (LoS) channel is predominantly characterized by a rank-one matrix, thereby constraining the spatial degrees of freedom (DoFs). In contrast, the near-field environment, underpinned by the spherical propagation model, presents a LoS channel having a rank that can substantially augment the spatial DoFs. This enhancement is not merely a function of the antenna arrays, but also is influenced by the proximity between the BS and the UE Decarli and Dardari (2021). This transition to the near-field domain offers a richer multipath environment, enabling simultaneous transmission of multiple data streams, as opposed to the singular data stream capability inherent to the far-field region. Techniques such as distance-aware precoding and widely spaced multi-subarray precoding further optimizes this potential, dynamically adjusting to user proximity and enhancing spatial granularity. Furthermore, for multi-user scenarios, near-field communications provide opportunities for user differentiation. While far-field systems grapple with interference challenges for users at similar angles, the near-field allows the BS to discern users based on both angle and distance. This capability, often termed as location-division multiple access (LDMA), facilitates efficient multi-user access by targeting users based on their unique angle-distance profiles Zhang et al. (2021). In essence, the integration of near-field THz MIMO communication in 6G networks promises unprecedented capacity enhancements, higher data rates, and improved beamforming, heralding a new era in wireless communication systems.
3 Future research paths for near-field communications
3.1 Understanding near-field communication theory
Most communication theories use the Rayleigh distance, a measure based on phase differences, to define the range of near-fields. Recent efforts in Chen et al. (2023) are trying to refine this measure, for instance, the “effective Rayleigh distance” is defined in Cui and Dai (2021) that better describes certain losses in near-fields. Specifically, it aims to better capture the losses associated with beamforming gain and capacity in the near-field scenarios. However, there is more to explore, especially in ELAA/MIMO systems.
3.2 Signal processing directions
CS is another transformative signal processing technique that can be used in THz MIMO systems to address high-dimensional channel estimation challenges in near-field scenarios. CS exploits the sparsity of the near-field environment to reconstruct channel state information with fewer measurements than traditional methods. This application can lead to significant reductions in pilot overhead and enhance spectral efficiency. CS-based techniques can also cater to the unique propagation characteristics of the THz band, such as molecular absorption and scattering effects. This enables efficient channel estimation, beam alignment, and data detection in THz MIMO systems. Future research could focus on developing adaptive algorithms that dynamically adjust to varying sparsity levels in different near-field scenarios and integrating machine learning techniques with CS-based signal processing to further optimize the performance in near-field THz settings.
3.3 Exploring new transmission technologies
In near-fields, the complexity of managing extensive codebooks complicates tasks like channel estimation and beamforming. AI offers potential solutions by efficiently navigating near-field characteristics, though research in this domain remains emerging compared to far-field studies. RIS introduce unique spherical signal patterns in near-field-THz-communications, necessitating further exploration in beam focusing and channel estimation. Real-world systems will encompass both near and far-field components, with users at varied distances from the BS and differing frequencies introducing mixed conditions. Addressing these hybrid scenarios is crucial for advancing 6G networks. Furthermore, integrating CS-based signal processing can optimize the capacity of these networks Cui and Dai (2022).
Another important factor is the spatial non-stationarity. Unlike previous generations, in ELAA systems, different parts of the system might “see” different users or objects, which can introduce variations in signal quality across the system. Although some work has delved into the combined effects of non-stationarity and near-field behavior Petrov et al. (2023), more studies are needed, especially with state-of-the-art communication schemes.
4 Conclusion
In upcoming 6G networks, near-field THz communications with spherical wavefronts are gaining prominence as massive MIMO evolves to ELAA. Traditional methods, designed for long-distance flat wavefront, are inadequate. This review explores the benefits and challenges of near-field dynamics for 6G, highlighting the unique traits of spherical waves and their impact on the near-field range. We address challenges like channel estimation and beam management, presenting recent solutions. Emphasizing innovative strategies to boost network capacity, we also spotlight future research avenues, including refining Rayleigh distance, hybrid communications, and pencil beamforming by employing CS-signal processing techniques.
Author contributions
VS: Writing–original draft, Conceptualization, Methodology. NG: Conceptualization, Methodology, Supervision, Writing–review and editing. SS: Conceptualization, Methodology, Supervision, Writing–review and editing. VB: Methodology, Supervision, Writing–review and editing, Conceptualization, Funding acquisition, Project administration.
Funding
The author(s) declare financial support was received for the research, authorship, and/or publication of this article. TIH-IoT CHANAKYA Group Fellowship Pro-gram, 2022-2023” by the TIH Foundation for IoT and IoE, IIT Bombay.
Acknowledgments
The authors would like to thank “TIH-IoT CHANAKYA Group Fellowship Program, 2022-2023” by the TIH Foundation for IoT and IoE, IIT Bombay.
Conflict of interest
The authors declare that the research was conducted in the absence of any commercial or financial relationships that could be construed as a potential conflict of interest.
The author(s) declared that they were an editorial board member of Frontiers, at the time of submission. This had no impact on the peer review process and the final decision.
Publisher’s note
All claims expressed in this article are solely those of the authors and do not necessarily represent those of their affiliated organizations, or those of the publisher, the editors and the reviewers. Any product that may be evaluated in this article, or claim that may be made by its manufacturer, is not guaranteed or endorsed by the publisher.
References
Akyildiz, I. F., Kak, A., and Nie, S. (2020). 6G and beyond: the future of wireless communications systems. IEEE Access 8, 133995–134030. doi:10.1109/access.2020.3010896
Ali, S., Saad, W., Rajatheva, N., Chang, K., Steinbach, D., Sliwa, B., et al. (2020). 6G white paper on machine learning in wireless communication networks. Available at: https://arxiv.org/abs/2004.13875.
Asif Haider, M., and Zhang, Y. D. (2023). RIS-aided integrated sensing and communication: a mini-review. Front. Signal Process. 3. doi:10.3389/frsip.2023.1197240
Chen, Y., Li, R., Han, C., Sun, S., and Tao, M. (2023). Hybrid spherical- and planar-wave channel modeling and estimation for terahertz integrated UM-MIMO and IRS systems. IEEE Trans. Wirel. Commun. 22, 9746–9761. doi:10.1109/twc.2023.3273221
Cui, M., and Dai, L. (2021). Near-field wideband beamforming for extremely large antenna arrays. Available at: https://arxiv.org/abs/2109.10054.
Cui, M., and Dai, L. (2022). Channel estimation for extremely large-scale MIMO: far-field or near-field? IEEE Trans. Commun. 70, 2663–2677. doi:10.1109/tcomm.2022.3146400
Cui, M., Wu, Z., Lu, Y., Wei, X., and Dai, L. (2023). Near-field MIMO communications for 6G: fundamentals, challenges, potentials, and future directions. IEEE Commun. Mag. 61, 40–46. doi:10.1109/MCOM.004.2200136
Decarli, N., and Dardari, D. (2021). Communication modes with large intelligent surfaces in the near field. IEEE Access 9, 165648–165666. doi:10.1109/ACCESS.2021.3133707
Doeker, T., Samala, P. R., Negi, P. S., Rajwade, A., and Kürner, T. (2021). “Angle of arrival and angle of departure estimation using compressed sensing for terahertz communications,” in Proceedings of the 2021 15th European Conference on Antennas and Propagation (EuCAP), Dusseldorf, Germany, March 2021, 1–5.
Han, C., Akyildiz, I. F., and Gerstacker, W. H. (2015). “Timing acquisition for pulse-based wireless systems in the terahertz band,” in Proceedings of the Second Annual International Conference on Nanoscale Computing and Communication, New York, NY, USA, September 2015 (Association for Computing Machinery), 1–6. doi:10.1145/2800795.2800819
Han, C., Wu, Y., Chen, Z., and Wang, X. (2019). Terahertz communications (teracom): challenges and impact on 6g wireless systems. Available at: https://arxiv.org/abs/1912.06040.
Hao, W., You, X., Zhou, F., Chu, Z., Sun, G., and Xiao, P. (2023). The far-/near-field beam squint and solutions for THz intelligent reflecting surface communications. IEEE Trans. Veh. Techn. 72, 10107–10118. doi:10.1109/tvt.2023.3254153
Hu, S., Wang, H., and Ilter, M. C. (2023). Design of near-field beamforming for large intelligent surfaces. IEEE Trans. Wirel. Commun., 1. doi:10.1109/TWC.2023.3281885
Hu, Z., Chen, C., Jin, Y., Zhou, L., and Wei, Q. (2022). Hybrid-field channel estimation for extremely large-scale massive MIMO system. IEEE Commun. Lett. 27, 303–307. doi:10.1109/lcomm.2022.3219937
Jornet, J. M., and Akyildiz, I. F. (2011). Channel modeling and capacity analysis for electromagnetic wireless nanonetworks in the terahertz band. IEEE Trans. Wirel. Commun. 10, 3211–3221. doi:10.1109/TWC.2011.081011.100545
Luo, H., Gao, F., Yuan, W., and Zhang, S. (2023). Beam squint assisted user localization in near-field integrated sensing and communications systems. IEEE Tran. Wirel. Commu., 1. doi:10.1109/twc.2023.3319474
Myers, N. J., and Heath, R. W. (2021). InFocus: a spatial coding technique to mitigate misfocus in near-field LoS beamforming. IEEE Trans. Wirel. Commun. 21, 2193–2209. doi:10.1109/twc.2021.3110011
Nie, S., Jornet, J. M., and Akyildiz, I. F. (2019). “Intelligent environments based on ultra-massive MIMO platforms for wireless communication in millimeter wave and terahertz bands,” in Proceedings of the ICASSP, IEEE International Conference on Acoustics, Speech and Signal Processing, Brighton, UK, May 2019, 7849–7853.
Petrov, V., Bodet, D., and Singh, A. (2023). Mobile near-field terahertz communications for 6G and 7G networks: research challenges. Front. Commun. Netw. 4. doi:10.3389/frcmn.2023.1151324
Sarieddeen, H., Alouini, M.-S., and Al-Naffouri, T. Y. (2020). An overview of signal processing techniques for terahertz communications. Proc. IEEE 109, 1628–1665. doi:10.1109/jproc.2021.3100811
Schram, V., Moldovan, A., and Gerstacker, W. H. (2018). “Compressive sensing for indoor THz channel estimation,” in Proceedings of the 2018 52nd Asilomar Conference on Signals, Systems, and Computers, Pacific Grove, CA, USA, October 2018.
Sharma, V., Sharma, S., and Bhatia, V. (2021). “Compressive sensing-based low complexity terahertz receiver,” in Proceedings of the International Symposium on Advanced Networks and Telecommunication Systems, ANTS, Hyderabad, India, December 2021, 302–306.
She, C., Cheng, P., Li, A., and Li, Y. (2021). Grand challenges in signal processing for communications. Front. Signal Process. 1. doi:10.3389/frsip.2021.664331
Tan, J., and Dai, L. (2021). Wideband beam tracking in THz massive MIMO systems. IEEE J. Sel. Areas Commun. 39, 1693–1710. doi:10.1109/JSAC.2021.3071817
Tarboush, S., Ali, A., and Al-Naffouri, T. Y. (2023). “Compressive estimation of near field channels for ultra massive-MIMO wideband THz systems,” in Proceedings of the ICASSP 2023 - 2023 IEEE International Conference on Acoustics, Speech and Signal Processing (ICASSP), Rhodes Island, Greece, June 2023 (IEEE), 1–5.
Wei, X., Dai, L., Zhao, Y., Yu, G., and Duan, X. (2022). Codebook design and beam training for extremely large-scale RIS: far-field or near-field? China Commun. 19, 193–204. doi:10.23919/jcc.2022.06.015
Wu, Y., Song, G., Liu, H., Xiao, L., and Jiang, T. (2023). 3-D hybrid beamforming for terahertz broadband communication system with beam squint. IEEE Trans. Broadcast. 69, 264–275. doi:10.1109/TBC.2022.3205214
Zhang, H., Shlezinger, N., Guidi, F., Dardari, D., Imani, M. F., and Eldar, Y. C. (2021). “Beam focusing for multi-user MIMO communications with dynamic metasurface antennas,” in Proceedings of the ICASSP 2021 - 2021 IEEE International Conference on Acoustics, Speech and Signal Processing (ICASSP), Toronto, ON, Canada, June 2021 (Institute of Electrical and Electronics Engineers Inc), 4780–4784. doi:10.1109/ICASSP39728.2021.9413746
Keywords: RIS, beamforming, THz, 6G, ELLA, signal processing
Citation: Sharma V, Garg N, Sharma S and Bhatia V (2024) A mini-review of signal processing techniques for RIS-assisted near field THz communication. Front. Sig. Proc. 3:1297945. doi: 10.3389/frsip.2023.1297945
Received: 20 September 2023; Accepted: 22 December 2023;
Published: 08 January 2024.
Edited by:
Ramoni Adeogun, Aalborg University, DenmarkReviewed by:
Yang Yang, Zhongnan University of Economics and Law, ChinaAbstractCopyright © 2024 Sharma, Garg, Sharma and Bhatia. This is an open-access article distributed under the terms of the Creative Commons Attribution License (CC BY). The use, distribution or reproduction in other forums is permitted, provided the original author(s) and the copyright owner(s) are credited and that the original publication in this journal is cited, in accordance with accepted academic practice. No use, distribution or reproduction is permitted which does not comply with these terms.
*Correspondence: Vimal Bhatia, vbhatia@iiti.ac.in