- Novel Devices Laboratory, University of Cincinnati, Cincinnati, OH, United States
History is full of technological breakthroughs that arguably were first discovered because fundamental research was conducted without commercial influence. Fundamental research also plays an important role in producing the next generation of researchers. However, this article argues that research in general, and particularly biosensor research, is suffering from diminishing impact because of a lack of entrepreneurial mindset when defining research objectives. The story of the author’s own biosensor research pathway is presented, and it provides an exemplary case study of this larger problem. An entrepreneurial mindset enables a stronger foundation even at the stage of forming the research hypothesis: the need for the research is justified; the research is not duplicative and is positioned to create new knowledge; the research product will be more readily translatable by industry. Most graduates in sensor research will work in industry, and therefore those students exposed to an entrepreneurial mindset will also start their careers with a more complete set of the skills that industry is looking for.
Introduction
Academic researchers are mostly rewarded for publications, citations, and grant funding above other metrics. It is therefore expected that these top metrics strongly influence the formation of research objectives. Historically, this conventional model of research has served society well in providing new breakthroughs and in generating the graduate workforce needed by industry to drive commercial innovation. However, this article will argue that, at least sensor research, and more specifically biosensor research, has a significant need to realign with real world needs and commercial viability. Yes, some fundamental research must continue without the burden of having to generate commercial impact, but this article will provide evidence suggesting that too much biosensor research proceeds without alignment with real needs in improving human health. This perspective article will highlight the continued academic pursuit of sweat biosensors. This pursuit persists despite the now-available knowledge that these sensors will, in nearly all cases, be far less accurate than commercially proven and patient-accepted biosensors such as continuous glucose monitors. The argument draws on the author’s own journey in biosensors, which started in 2012 in sweat biosensing; after 8 years of research, it resulted in a period of disillusionment and deep reflection and then further resulted in an entrepreneurially informed pivot into a much more productive and commercially viable pathway. This perspective is written as a story narrative. It is the author’s hope that other sensor researchers can learn from the story and therefore increasingly avoid misplaced research effort. The story will follow the plot of the well-known and industry-respected Gartner Hype Cycle (Figure 1). It will reveal the power of adopting an entrepreneurial mindset before or during the formation of research hypothesis. At the end of this perspective, the author will also provide a simple method centered on an entrepreneurial mindset that can act as an effective guide for other researchers who want to more quickly maximize the odds that their research will find real-world impact.
The innovation: trigger-non-invasive biosensing through sweat
In 2012, the US Air Force came to the author and said, “Jason, we have 2,000 sensors on a plane and only 1 sensor on the pilot and the pilot is the performance bottleneck for the entire system, we want you to help us figure out how to monitor pilot performance in real time with biosensors.” Having no experience in biosensors, the author was initially reluctant but then enthusiastic due to the excitement of pivoting his technological expertise into new applications. In 2013, bolstered by early publications by Dermot Diamond (Schazmann et al., 2010) and Joseph Wang (Jia et al., 2013), the author and the US Air Force began a focused effort in sweat biosensing. This was the innovation trigger illustrated in Figure 1. Within just a few years, the plot of Figure 2 demonstrates that publications by the author and other groups quickly generated global interest in sweat biosensing. The “sky was the limit”, as the lofty goal of a continuous non-invasive molecular biosensor seemed as though it could finally be a reality.
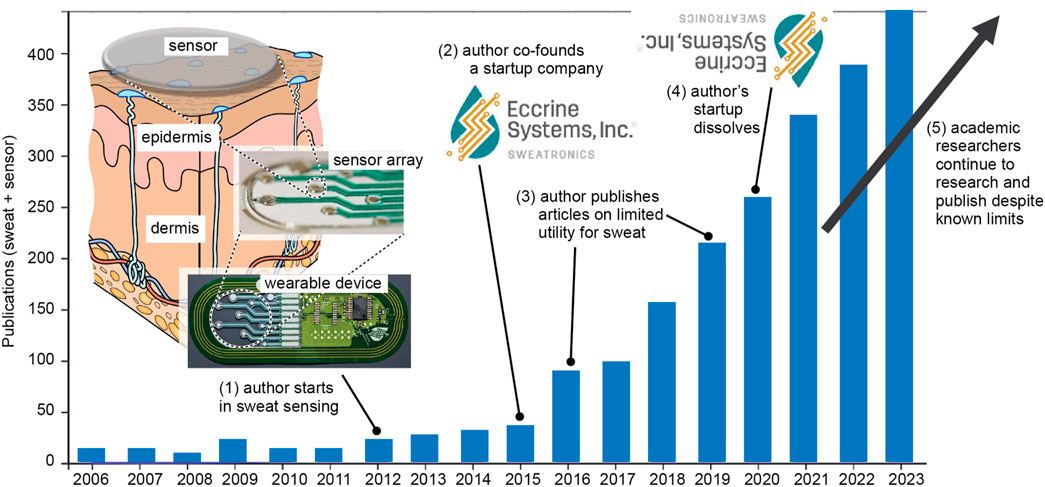
Figure 2. Publications with “sweat” + “sensor” vs. year (courtesy Web of Science) with annotations of key activities by the author as he entered and exited the field.
The peak of inflated expectations
The field of sweat biosensing rapidly gained momentum as the author and other researchers published clever-looking wearable devices (Heikenfeld, 2016) with continuous sweat molecule data, despite the fact that none of the publications were able to show any correlation with the blood levels of the molecules. The author published the first informative review on sweat gland physiology in 2015 (Sonner et al., 2015) and on sweat biosensing in 2016 (Heikenfeld, 2016) which concluded:
It is therefore the position of this author that the most important conclusions that can be made at this time are two-fold:
1) the opportunity provided in eccrine sweat is clearly compelling compared to other non-invasive biofluids, and has the potential to enable a true performance leap for wearable technology;
2) the many challenges for eccrine sweat are likely resolvable directly or indirectly through technological innovation. Importantly, circa 2016, the roadmap of requirements for addressing these challenges is now much more clear.”
These conclusions still hold true today; however, as will be seen in later sections of this perspective, early adoption of an entrepreneurial mindset would have resulted in a major revision of them. In the 2016 review (Heikenfeld, 2016), it was also stated and was already becoming clear that the most accurate diagnostic data in sweat were going to be limited mainly to small hydrophobic molecules (drugs, steroid hormones, etc.) or to sweat fluid loss for dehydration prevention. These conclusions were only reinforced over time with two Nature-series journals articles that included the author (Heikenfeld et al., 2019; Davis et al., 2024). Small hydrophobic molecules alone represented a moderate but significant potential commercial market. Therefore, in 2015, the author co-founded and helped grow a startup company, Eccrine Systems Inc. Eccrine Systems experienced rapid investor-driven growth such that even Bloomberg highlighted the company as a top global startup to watch (Huet, 2017).
At this point, the author’s academic research narrowed its focus to small hydrophobic analytes (Hauke et al., 2018) and sweat fluid loss (Zhao et al., 2019). However, in measuring molecule concentrations with blood correlation, the complexity of the devices was increasing to the point where commercial viability was arguably diminishing (Hauke et al., 2018). This point in time is where the deficiencies of an inward-looking academic biosensor research community become highly visible; despite the limited utility for sweat, the rapid growth of publications continued with a large focus on metabolites such as glucose and lactate. Sweat glucose and lactate were, and still remain, doubtful if not impossible for blood correlation with the approaches currently being demonstrated. For example, there is even recent research claiming that tight sweat glucose to blood correlation is possible (Sempionatto et al., 2021). In this and most sweat glucose papers, enzymatic sensors are utilized whose signal directly depends on analyte flux to the sensor. Therefore, the glucose signal will be proportional to sweat rate, and sweat rate is extremely variable across the population and within the individual. Even if a blood glucose correlation is possible, what is the end-user or healthcare system value proposition since it is well-known that metabolites are accurately measurable in interstitial fluid with a commercially proven device that is now even available direct to consumers (Heikenfeld et al., 2019; Friedel et al., 2023a)? It is also troubling that research foundations seeking to resolve tough chronic diseases such as irritable bowel syndrome have used important philanthropic funding to sponsor newer startups that promise revolutionary results with the inflammation proteins in sweat (Falci, 2024). The meaningful monitoring of proteins in sweat is difficult if not impossible based on current fundamental understanding of sweat physiology (Heikenfeld et al., 2019). Furthermore, if you are really trying to help chronic disease patients, why bother with sweat, knowing it is generally diagnostically inferior to biofluids such as interstitial fluid? In medicine, accuracy usually matters more than novelty or perceived user convenience.
The trough of disillusionment
Eccrine Systems eventually raised roughly $30M, grew to nearly 60 employees, and then dissolved shortly after the 2020 COVID pandemic caused a major decrease in venture capital investing. In retrospect, a quick dissolution of Eccrine Systems was likely beneficial to the author’s ongoing research because, even if the company had attracted additional investor funding, it is now clear that
- the technology required for continuous and blood-correlated sweat biosensing (Hauke et al., 2018) is likely far more complex than any wearable device that has ever been commercialized;
- it would have been a major uphill battle, as key decision makers such as doctors are highly suspicious of the diagnostic value of a new unproven biofluid such as sweat;
- the potential market value for sweat sensing devices is real but very small compared to successes such as glucose monitors or other wearable diagnostic devices.
Several other sweat-based startups either dissolved, pivoted away from sweat, or substantially reduced their focus to a more limited number of applications (Davis et al., 2024). Again, as shown in Figure 2, despite this trough of disillusionment from an industry perspective, academic research and publication in sweat biosensing continued to grow due to a lack of diligence by researchers or due to ignoring the now-known scientific facts.
The slope of enlightenment (the most important part of this story)
This next section follows a train of interviews and thought process over a 2-year time span that the author wishes he had utilized when he began working in biosensing in 2012.
In 2021, the author began an intensive effort to reposition (pivot) his biosensing research. Surely, there were applications worth pursuing beyond just glucose monitoring? Part of this 2021 pivot was enabled by some of the author’s earlier efforts in pursuit of sweat sensing. During his time with Eccrine Systems (2015–2020), the author, after starting as a chief technology officer, decided to revise his role to Principal Scientist. As Principal Scientist, the author invested his time and energy deep in the fundamentals of what technology breakthroughs were needed to make a compelling wearable sweat biosensor. Part of this journey led the author in 2015 to aptamer sensor pioneer Prof. Kevin Plaxco’s office at the University of California at Santa Barbara. This was an eye-opening visit that convinced the author that electrochemical aptamer sensors were essential for monitoring the most meaningful molecules in sweat (Arroyo-Currás et al., 2020). During this visit, the author was further influenced by a sign of good luck that he photographed (Figure 3) through the office window of Prof. Plaxco. Indeed, later in 2021, the author would extract durable value from this visit to Prof. Plaxco.
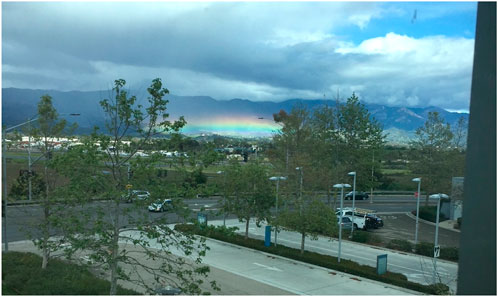
Figure 3. A sign of good fortune? Indeed, this photo during a 2015 visit to Prof. Kevin Plaxco ultimately would lead to the most fruitful outcome of the author’s efforts in sweat biosensing research.
In 2021, the author realized his focus was too technologically deep during his sweat biosensing years and that he could not “see the forest through the trees.” This was a mistake that the author vowed not to make again as he investigated a biosensing pivot in 2021. As part of this pivot, the author decided he needed to spend more time on the end-user and business aspects related to the future of biosensing. The first phase of the author’s discovery work was to leverage his position and network to interview the heads of research and development at major glucose monitor companies. The author began with a simple question that could be answered without a confidentiality agreement: “what are the most important questions you ask yourself when considering a new sensing modality beyond glucose?” The answers were very consistent: “we don’t care what a new sensor does unless it can last at least 3 days in a beaker, and it still a matter of debate on what molecules to pursue outside of diabetes management.”
So, which biosensor platform to pursue? In 2021, aptamer sensors were, and still are, along with enzymatic sensors the only class of sensors demonstrated in vivo for numerous analytes (Arroyo-Currás et al., 2020). However, the author was aware that aptamer sensors fell well short of the minimum 3-day goal stated by industry experts. In the author’s lab, aptamer sensors would provide accuracy for a mere 6–12 h. So, the author next went to several global academic experts in aptamer sensors with the simple question: “for how long can aptamer sensors operate accurately for.?” A common response was: “about 24 hours maybe.” At this point, a worthy research topic had been identified for the author, regardless of the end-application. Multi-week aptamer sensor longevity was clearly a worthy fundamental research goal because 1) as demonstrated with 2-week wearable glucose monitors, sensor longevity is critical for reducing costs and improving user ergonomics, and 2) the required aptamer sensor longevity had never been demonstrated and was an open fundamental question if it was even possible. Despite the significant challenge level of this goal, the author’s academic research team made 2-week aptamer sensor longevity their primary focus (see the next section for what was accomplished).
However, a sensor is only as good as the device to which it is connected and the biofluid in which it measures the molecule. After a rough experience with sweat biosensors, the author was resolved to avoid the two major risks associated with sweat biosensing: 1) complicated new device structures, and 2) a biofluid that was perceived as risky by doctors. The logical choice was clear: interstitial fluid a few millimeters beneath the skin surface—the same fluid accessed by continuous glucose monitors (Heikenfeld et al., 2019; Friedel et al., 2023a). The author’s team quickly and thoroughly investigated interstitial fluid fundamentals and hypothesized that most small and medium (ones to tens kDa) molecules could potentially have strong blood correlation (Friedel et al., 2023a). While such capability had not yet been demonstrated, an under-investigated hypothesis was a good fundamental scientific reason to pursue academic research in interstitial fluid.
However, a sensor must also reliably access its target biofluid. The author then began parallel investigative paths with microneedle sensing (currently a very hot topic in academic research) vs. a single indwelling needle sensor like that used in commercial glucose monitors. Despite some early success with microneedles, including the first ever human data with aptamer sensors (Friedel et al., 2023b), the author and his research team decided their main focus would be to mimic the commercial continuous glucose monitors which doctors trusted and which diabetes patients loved (Figure 4). The rationale for this focus was three-fold. First, the author’s team could not answer the question: “what significant problem do microneedles solve that the existing glucose monitor format could not already solve?” Second, deeper placement into the skin is likely more accurate, and in medical diagnostic applications accuracy is critical. Third, the current devices are absolute works of art and engineering, inexpensive to manufacture, and universally accepted, so why “reinvent the wheel”? Interestingly, despite these powerful arguments that the author’s team adopted in 2021, even today most academic molecular biosensor researchers worldwide remain predominantly focused on sweat or microneedles.
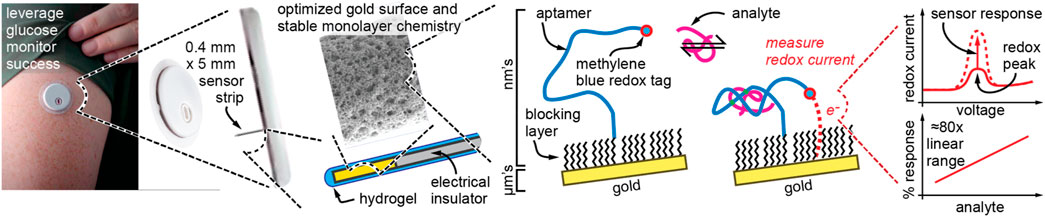
Figure 4. The author’s new strategy, to adapt proven commercial technology with a new sensing modality based on aptamers that allows biosensing beyond glucose. The device shown at left is Abbott’s Libre 3 glucose monitoring wearable and is just a representation of the author’s goal and not something that has yet been accomplished.
However, a biosensor is only valuable if it measures an analyte that people care about. In 2021, the author was very active in interviewing candidate CEOs to help launch a new startup company based on the above-described new research foci. The author spoke with a local venture firm who recommended he find a CEO with direct industry experience in diagnostic products. The venture firm further recommended he speak to Andrew Cothrel. Andrew was a serial entrepreneur with corporate experience at both Abbott and Roche diagnostics divisions, the perfect match to help guide the above research foci to the right applications. After 2 years of countless interviews with industry, doctors, and patients, the author and his CEO formed a strong hypothesis that molecular monitoring in cardiovascular diseases was the next big commercial market for wearable molecular monitors. The author and Cothrel further narrowed their initial focus on helping US hospitals reduce their $16B problem in heart failure readmissions. A new startup, Kilele Health, was formed (kilele means “peak” in Swahili), and the final puzzle pieces were in place for a potentially fruitful research path. Empowered by important new fundamental questions to answer and applications that matter, the author was able to quickly obtain over a dozen new research grants to support his research pivot in biosensors. Despite leveraging as much existing technology and proof points as possible, these fundamental research grants were appropriate because, as the author has experienced, biosensing research is always incredibly difficult and requires a large fundamental research effort just to reach preliminary in vivo results.
The plateau of productivity–will we get there?
This story is not finished. There are no aptamer sensors on the market, and even large companies have struggled to release compelling biosensing products beyond glucose. However, the approach taken above was empowered by an entrepreneurial mindset and achieved by seeking critical inputs outside the academic community. Furthermore, recalling the goal of aptamer sensors that last at least 7 days, the author’s team achieved a record 7-day operation in 2023 (Watkins et al., 2023) and recently shattered their own aptamer sensor longevity record with an unprecedented 60 days of continuous accurate sensor operation in whole serum at body temperature (Watkins et al., 2024). Furthermore, the author’s team is now demonstrating in vivo placement of these aptamer sensors on sensor electrodes that mimic the form factors and insertion methods used in continuous glucose monitors. The author now strongly feels that, at minimum, he has maximized his chances of being successful in advancing molecular monitoring beyond glucose. Only the future will tell how this story ends.
A reflection and summary
Turning back to Figure 2, it is clear that the thought process used to define academic research objectives is very different than the entrepreneurial mindset the author used to pivot his research into a productive new pathway. How many more research papers must be published in sweat glucose or lactate until the academic field realizes some of the same insights that the author realized? Beyond sweat biosensors, similar problems exist across biosensors in general. John Roger’s pioneering electronic tattoo work (Heikenfeld et al., 2018) may have utility for fragile neonate skin, but it has also inspired its own hype cycle and overextension of proposed utility by other researchers. Similar problems also exist for energy harvesting for biosensors. If you talk to wearable biosensor companies, small, rigid, and battery-powered devices are just fine. Again, what real-world problems exist that need solving?
The first line of this article states, “Academic researchers are mostly rewarded for publications, citations, and grant funding above other metrics.” If this system of rewards does not change, then it is likely that academic research will continue to struggle to align with the most fruitful research paths. There are some potentially simple solutions. Research does not happen without grant funding. Ultimately, having grant panels and reviews with even more industry experts and end stakeholders would drive a more entrepreneurial mindset into sensor research (e.g., for biosensors to include doctors, industry experts, and patients). The evaluation of proposed research projects is a worldwide problem involving a traditional process that can be difficult to change or improve. In the meantime, the author hopes that this article will inspire today’s sensor researchers to adopt an entrepreneurial mindset in defining their next research objectives. Why wait? Students will benefit too, given that most graduates in biosensor research will work in industry where entrepreneurial thinking is critical, even inside large companies.
It is not the purpose of this article to diminish the importance of academic or theoretic research but rather to emphasize the strong importance of stakeholder- and outcome-driven research in wearable medical sensors. This article ends with a step-by-step method to incorporate an entrepreneurial mindset into sensor research. Similar versions of this method are outlined near the end of the author’s other recent perspectives (Friedel et al., 2023a; Davis et al., 2024). This process, if done well, may take several months, if not years, of continuous effort to maximize its impact, but it will be well worth the effort. If your research lab has eight people, that equates to >10,000 h per year on research. Therefore spending tens or even hundreds of hours in an entrepreneurial mindset seems justifiable. To do this, here is a simple guide.
1) Start with finding the best problems to solve, not pushing your current technology. The author had created world-leading sweat sensing technology (Hauke et al., 2018) but abandoned it when it was time for his pivot. Instead, ask the industry what problems are holding back biosensor progress. Ask end-users (doctors and nurses, patients, and payers) what problems they have with existing diagnostic technology or what problems could benefit from technological assistance. This typically requires dozens of well-structured interviews, if not more, with stakeholders typically only found outside of academia. Interdisciplinary engagement is critical to building a strong foundation.
2) Now that you have found a problem worth solving, ensure that the problem is fundamentally solvable against the end requirements you learned during interviews. Solvability could be limited by the stability of sensor chemistries, or, for example, by factors out of your control such as physiology not being able to provide reliable access to a molecule (Heikenfeld et al., 2019; Friedel et al., 2023a). If conventional wisdom says it cannot be done, question it because sometimes the upside is so big it that is worth taking the risk to challenge conventional wisdom (e.g., the author’s team’s effort in aptamer sensor longevity).
3) Now that you have found problems worth solving and which are solvable, ask two more sequential questions:
a. Are there existing technologies or tools that can simply be readapted to help solve the problem, such that unnecessary research efforts are avoided? In vivo demonstration of a working biosensor is hard enough, so do not take on more challenges than you absolutely must.
b. If the answer to (a) above is “no,” then as you pursue your research iteratively, try to innovate by subtraction and reducing the complexity of your biosensor device. While complexity may make for an interesting paper or presentation to the research community (Hauke et al., 2018), end-users just want the problem solved, and the more complex the solution, the less likely it will be successful.
The above step-by-step method is, of course, highly abbreviated in explanation; for further consideration look at programs and methods such as the National Science Foundation’s I-Corps program in the United States. You can also turn to your local venture capital firms or startup incubators, who typically have some or all these resources internally. Thank you for reading this article. Hopefully you can learn and benefit from the mistakes made by the author as he made his first attempt at biosensor research.
Data availability statement
The raw data supporting the conclusion of this article will be made available by the author, without undue reservation.
Author contributions
JH: writing–original draft and writing–review and editing.
Funding
The author declares that no financial support was received for the research, authorship, and/or publication of this article.
Conflict of interest
JH is a co-founder of Kilele Health Inc. which is commercializing wearable molecular biosensors.
Publisher’s note
All claims expressed in this article are solely those of the authors and do not necessarily represent those of their affiliated organizations, or those of the publisher, the editors, and the reviewers. Any product that may be evaluated in this article, or claim that may be made by its manufacturer, is not guaranteed or endorsed by the publisher.
References
Arroyo-Currás, N., Dauphin-Ducharme, P., Scida, K., and Chávez, J. L. (2020). From the beaker to the body: translational challenges for electrochemical, aptamer-based sensors. Anal. Methods 12, 1288–1310. doi:10.1039/d0ay00026d
Davis, N., Heikenfeld, J., Milla, C., and Javey, A. (2024). The challenges and promise of sweat sensing. Nat. Biotechnol. Epub ahead of print. PMID: 38212492. doi:10.1038/s41587-023-02059-1
Falci (2024). No blood, some sweat, No tears: wearable biosensor represents future of noninvasive IBD monitoring, IBD visible. Available at: https://www.crohnscolitisfoundation.org/blog/no-blood-some-sweat-no-tears-wearable-biosensor-represents-future-of-noninvasive-ibd.
Friedel, M., Thompson, I. A. P., Kasting, G., Polsky, R., Cunningham, D., Soh, H. T., et al. (2023a). Opportunities and challenges in the diagnostic utility of dermal interstitial fluid. Nat. Biomed. Eng. 7, 1541–1555. doi:10.1038/s41551-022-00998-9
Friedel, M., Werbovetz, B., Drexelius, A., Watkins, Z., Bali, A., Plaxco, K. W., et al. (2023b). Continuous molecular monitoring of human dermal interstitial fluid with microneedle-enabled electrochemical aptamer sensors. Lab. Chip 23 (14), 3289–3299. PMID: 37395135. doi:10.1039/d3lc00210a
Hauke, A., Simmers, P., Ojha, Y., Cameron, B., Ballweg, R., Zhang, T., et al. (2018). Complete validation of a continuous and blood-correlated sweat biosensing device with integrated sweat stimulation. Lab a Chip 18, 3750–3759. doi:10.1039/C8LC01082J
Heikenfeld, J., Jajack, A., Feldman, B., Granger, S. W., Gaitonde, S., Begtrup, G., et al. (2019). Accessing analytes in biofluids for peripheral biochemical monitoring. Nat. Biotechnol. 37, 407–419. doi:10.1038/s41587-019-0040-3
Heikenfeld, J. C. (2016). Non-invasive analyte access and sensing through eccrine sweat: challenges and outlook circa 2016. Electroanalysis 28, 1242–1249. doi:10.1002/elan.201600018
Heikenfeld, J. C., Jajack, A., Rogers, J., Gutruf, P., Tian, L., Pan, T., et al. (2018). Wearable sensors: modalities, challenges, and prospects. Lab a chip 18 (2), 217–248. doi:10.1039/c7lc00914c
Huet, E. (2017) These are the 50 most promising startups you’ve never heard of. New York: Bloomberg. Available at: https://www.bloomberg.com/graphics/2017-fifty-best-startups/.
Jia, W., Bandodkar, A. J., Valdés-Ramírez, G., Windmiller, J. R., Yang, Z., Ramírez, J., et al. (2013). Electrochemical tattoo biosensors for real-time noninvasive lactate monitoring in human perspiration. Anal. Chem. 85 (14), 6553–6560. doi:10.1021/ac401573r
Schazmann, B., Morris, D., Slater, C., Beirne, S., Fay, C., Reuveny, R., et al. (2010). A wearable electrochemical sensor for the real-time measurement of sweat sodium concentration. Anal. Methods 2 (4), 342–348. doi:10.1039/B9AY00184K
Sempionatto, J. R., Moon, J. M., and Wang, J. (2021). Touch-based fingertip blood-free reliable glucose monitoring: personalized data processing for predicting blood glucose concentrations. ACS Sens. 6 (5), 1875–1883. Epub 2021 Apr 19. PMID: 33872007. doi:10.1021/acssensors.1c00139
Sonner, Z., Wilder, E., Heikenfeld, J., Kasting, G., Beyette, F., Swaile, D., et al. (2015). The microfluidics of the eccrine sweat gland, including biomarker partitioning, transport, and biosensing implications. Biomicrofluidics 9 (3), 031301. PMID: 26045728; PMCID: PMC4433483. doi:10.1063/1.4921039
Watkins, Z., Karajic, A., Young, T., White, R., and Heikenfeld, J. (2023). Week-long operation of electrochemical aptamer sensors: new insights into self-assembled monolayer degradation mechanisms and solutions for stability in serum at body temperature. ACS Sens. 8 (3), 1119–1131. Epub 2023 Mar 8. PMID: 36884003; PMCID: PMC10443649. doi:10.1021/acssensors.2c02403
Watkins, Z., Karajic, A., Young, T., White, R., and Heikenfeld, J. (2024) Advancements in electrochemical aptamer sensors for long-lasting and accurate in-vivo biosensing. New Orleans: Paper ID: 3990562 ACS Spring Meeting.
Keywords: sensors, biosensors, wearables, diagnostics, entrepreneurship, research
Citation: Heikenfeld J (2024) “Please learn from my mistakes”: the acute need for an entrepreneurial mindset in academic biosensor research. Front. Sens. 5:1408158. doi: 10.3389/fsens.2024.1408158
Received: 27 March 2024; Accepted: 16 May 2024;
Published: 24 June 2024.
Edited by:
Laís Canniatti Brazaca, São Paulo State University, BrazilReviewed by:
Marc Parrilla, University of Antwerp, BelgiumCopyright © 2024 Heikenfeld. This is an open-access article distributed under the terms of the Creative Commons Attribution License (CC BY). The use, distribution or reproduction in other forums is permitted, provided the original author(s) and the copyright owner(s) are credited and that the original publication in this journal is cited, in accordance with accepted academic practice. No use, distribution or reproduction is permitted which does not comply with these terms.
*Correspondence: Jason Heikenfeld, aGVpa2VuamNAdWNtYWlsLnVjLmVkdQ==