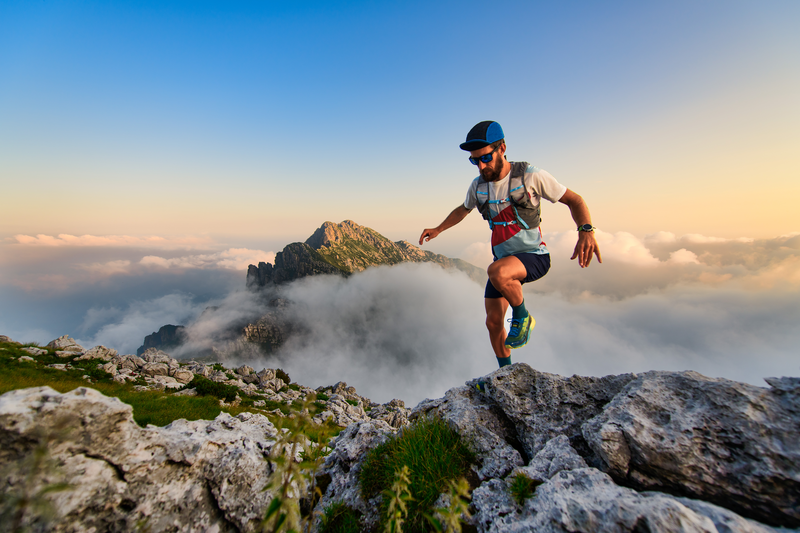
94% of researchers rate our articles as excellent or good
Learn more about the work of our research integrity team to safeguard the quality of each article we publish.
Find out more
MINI REVIEW article
Front. Sens. , 09 November 2023
Sec. Physical Sensors
Volume 4 - 2023 | https://doi.org/10.3389/fsens.2023.1279158
People with urinary incontinence (UI) often face a significant social stigma feeling ashamed of their condition and worrying about others discovering it. In order to improve the quality of life of those with incontinence, recent technological advancements enabled the development of non-invasive devices for detecting urinary leakage (UL). However, no comprehensive study has been conducted to state the most suitable types of sensors and the fundamental features necessary to design such devices, while also pointing gaps for future research. To address this, we conducted a mini review using four electronic databases limiting our search to English-written papers published in peer-reviewed journals. We retrieved articles that met the chosen inclusion criteria and classified them based on sensor type used, its location, the detection technique employed, and whether it was an e-Textile design and a reusable product or not. Across the studies, UL was detected using different approaches leading to heterogeneous results. Electrodes commonly used as sensing elements, along with textile as substrate material, and an indicator of UL based on resistance value, appeared to be widely exploited. However, the outcomes were not correlated with any specific type of UI. Consequently, we hypothesize that any non-invasive device could potentially be used for different types of UI. Nevertheless, further studies need to be conducted to confirm this statement. The designed literature mapping provides readers with an overview of the recent non-invasive wearable technologies in UL detection and offers a roadmap for future innovations.
Urinary incontinence (UI), defined as an involuntary loss of urine, affects around 420 million adults worldwide (Milsom and Gyhagen, 2019). Studies conducted in multiple countries reported a prevalence of UI ranging from 5% to 70%, with the highest prevalence in women (Milsom et al., 2013). Among the people with UI, 72% were women and 28% were men. Depending on the diagnostic, UI is commonly categorized as 1) stress incontinence (involuntary leakage occurs on physical exertion); 2) urge incontinence (sudden need to void associated with loss of urine); 3) mixed incontinence (combination of both stress and urgency incontinence); 4) overflow incontinence (incomplete bladder emptying) and 5) functional incontinence (caused by mental or physical disability) (The Canadian Continence Foundation, 2018). Consequences of incontinence are significant since it greatly affects the quality of life (QoL) of the affected people (depression, social isolation, etc.), and also the QoL of the caregivers in terms of responsibilities and emotional toll. In addition, there are direct and indirect financial expenses for the patients (diapers costs, absenteeism, loss productivity), and for the insurances (healthcare costs) (The Canadian Continence Foundation, 2014).
To reduce the urinary symptoms, various approaches have been developed. The first line management of UI is non-surgical and includes conventional methods such as lifestyle modifications, pelvic floor muscle training and bladder training (Dumoulin, 2016; Lamin et al., 2016). However, for many patients, access to these programs remains a barrier since the costs are not covered by healthcare plans (The Canadian Continence Foundation, 2014). Besides lifestyle changes (such as weight loss) and physiotherapy, pharmacotherapy is also used as conservative treatment of UI. Several drugs (mirabegron, duloxetine, etc.) have been approved to treat certain types of UI (Gandi and Sacco, 2021). They result in many side effects (dry mouth, constipation, potential cognitive impairment, etc.) (Vaughan and Markland, 2020), making the reduction of urinary symptoms more difficult. Multiple surgical methods such as colposuspension, anterior colporrhaphy, needle suspension and sling procedures are also available as alternatives solutions (Akdemir et al., 2020). However, not only is there a disagreement about the precise technique by which the incontinence can be addressed, but also, they are associated with a high complication and complex procedure (Aoki et al., 2017). While the effects of these methods on the UI remain unchanged over time, pads, diapers, urine collection devices or other minimally invasive techniques, such as laser therapy, urethral bulking agents, etc. (Alsulihem and Corcos, 2021; Anand et al., 2021), are also explored to improve QoL of people with UI. Despite their potential benefits, many affected people are still embarrassed to talk about their own condition. Consequently, there is a need to tailor another solution to remove the barriers to treatment.
Rapid urinary leakage detection (ULD) using a comfortable non-invasive system could improve the QoL of people with UI. Recent advances in technology have made it possible by opening a promising avenue. To our best knowledge, no comprehensive research study has highlighted the non-invasive technologies available in literature for ULD, to better guide caregivers and researchers in their decision-making. Jung et al. (Jung et al., 2015) proposed an overview of traditional products and automatic urine systems where no finding was reported regarding non-invasive wearable devices. Another comparative review (Porter et al., 2022) summarized the technology related to microwaves and their applications for UI as well some recommendations. However, the development and analysis of non-invasive devices were not the study’s focus. Very few commercial systems such as Opro 9 (Opro9, 2022), Smardii’s (Smardii, 2019), Therapee (Therapee, 2022) exist and are well known for real-time urine detection. These devices are mostly designed for children, and assuredly are very expensive, complex and need training for users. Documentary search in literature allowed us to remark that they are not widely accepted and require a license to use. Moreover, their validity is not clearly established since they are not well documented. Therefore, such devices are not covered in this paper.
Considering the medical importance of the UI and its related societal aspects, our objective was to present a mini review of original published papers on ULD utilizing non-invasive technologies. In this context, non-invasive refers to procedures where data are measured without inserting a device into the body. To achieve this, we conducted a systematic review to highlight the necessary features for optimal ULD focusing on sensor technology.
This mini review has followed the Preferred Reporting Items for Systematic Reviews and Meta-Analyses (PRISMA) guidelines. Four electronic databases (IEEE Xplore, Scopus, Web of Science and PubMed) were searched for studies reporting information on urinary incontinence, non-invasive technologies, detection and sensors. Each database was limited to articles published between 2007 and May 2023. References retrieved from the four databases were exported into the Covidence systematic review software. After removing duplicate papers from the search results, two independent reviewers screened the titles and abstracts to identify potentially eligible articles. In cases where the reviewers had differing opinions, a third reviewer was consulted to make the final decision regarding the selection. All authors independently reviewed the full-text articles of the pre-selected references to determine if they met the following selection criteria.
• 1) used non-invasive devices with sensors; 2) on adults aged 18 years or older; and 3) published in English.
The extracted data are: full article reference, participants’ characteristics (if available), features in the experimental protocol and study design. Additionally, the minimal urine volume detected, and the types of UI were recorded. Finally, the reported accuracy of the results was also documented.
Our electronic databases search yielded 640 articles, of which 361 duplicates were removed. Among the 279 remaining records, 250 were excluded during the title and abstract screening. A full text review was conducted for the remaining 29 references resulting into 12 articles that met the inclusion criteria. The search history and selection process are presented in Supplementary Material. Most of the included articles were published in the past 10 years. Figure 1 presents the most recent non-invasive wearable devices used for ULD. In Table 1, we provide a summary of the survey results of 12 non-invasive systems. To characterize these systems, we mainly used 5 classes: sensor type, sensor location, detection technique, e-Textile design, and reusable.
FIGURE 1. The different types of sensors embedded in non-invasive devices for urinary leakage detection (A–B) Conductivity sensor, image adapted respectively from (Wang et al., 2009), with permission from (copyright 2009, IEE) and (Tanaka et al., 2021) licensed CC-BY- 4.0 (C and D) Combination of gas, humidity and temperature sensor, adapted respectively from (Ortiz Pérez et al., 2017) licensed CC-BY- 4.0 and (Esfahani et al., 2020) with permission from (copyright 2020, IEEE) (E) light sensor, adapted from (Ishida et al., 2012) licensed CC-BY- 4.0 (F) Biosensor, adapted from (Su et al., 2022) with permission from (copyright 2022, Elsevier) (G) Humidity sensor, adapted from (Tekcin et al., 2022) licensed CC-BY- 4.0.
During the study of related works, we extracted six different types of sensors (Figure 1). The predominant type among them is the conductivity sensor. A conductivity sensor consists of electrodes used to measure electrical resistance or voltage. Most of the included studies (n = 6) reported a conductivity sensor as a single sensor for ULD (Table 1). Wang et al. (Wang et al., 2009) combined this type of sensor with a 3D-accelerometer and inclinometer. Their study demonstrated that a combination of sensors can accurately detect 0.5 mL of urine leakage during physical activities. As observed in the literature, the gas sensor needed to be combined with a humidity and a temperature sensor (Ortiz Pérez et al., 2017; Esfahani et al., 2020). However, the humidity sensor could also be used independently (Tekcin et al., 2022) (Figure 1). On the other hand, sensing elements such as light sensors and biosensors are less exploited in the existing literature (Table 1).
In this review, we restricted the sensor location to two options: 1) the sensor is located on a casing and attached to the underwear, diaper, bed mat or belt; or 2) the sensor is directly printed on a diaper. Among the experimental designs in literature (Table 1), diapers were the first case reported. The substances (biofluids or gases) that can be used to test the functionality of a system intended to detect UL are also highlighted in Table 1. Different concentrations of solution have been used but there is no homogeneity between them. However, the literature has proved that a non-invasive device could be able to detect 0.5 mL as the amount of UL (Wang et al., 2009). Unlike other studies, Esfahani et al. (2020) and Ortiz Pérez et al. (2017) demonstrated that their system could detect gases like Methyl mercaptan, which could be found in urine. For optimal results, Esfahani et al. (2020) showed that the data collected can be flowed to a neural network to identify synthetic urine from other odours with 100% accuracy.
Voltage, current and resistance are the measurement methods of ULD reported. Despite differences in methodologies across studies, all of them involve directly electrical signals. In the presence of urine, the literature has consistently shown a decrease in the resistance values when the changes in electrical impedance are measured (Table 1). For example, Tekcin et al. (Tekcin et al., 2022) and Fischer et al. (Fischer et al., 2019) respectively reported high resistance values (in MΩ) at dry state (Table 1). By adding 20 mL of biofluid, the resistance value measured by the sensor dropped to 20 kΩ (Fischer et al., 2019). Voltage and current variations are also used as indicators to differentiate between wet and dry state; however, no study has reported clear outcomes regarding the detection limit value and its corresponding range. In most studies, measurements are then sent to an external device like a smartphone, where parameters such as humidity and temperature (Esfahani et al., 2020), and the presence of urine, along with other raw sensor data are displayed.
To enhance reliability, flexibility and discretion, some studies have investigated textile-based support. According to the European committee of standardization (ISO/TR 16298, 2011), the integration of electronics into a textile can be identified by four different levels: a) Level 1 (the electronics are removable without damaging the product); b) Level 2 (attached, i.e., it is not removable without destroying the product); c) Level 3 (mixed, i.e., combination of textile integrated and attached components); d) Level 4 (full textile solution: textile finishing) (Simegnaw et al., 2021). Among the 8 studies dealing with e-textile and classified according to the different levels (Table 1), only 2 studies proposed washable products (reusable) while other systems are disposable (not reusable). In certain cases, the sensor was directly printed on a textile (Fischer et al., 2019) and its position can vary from covering the full length of the diaper (Sakamoto et al., 2018) to being located to a small part of the diaper (Wang et al., 2009).
The performance and the different limitations of the sensors are discussed in the following subsections in order to guide the next innovations.
From the literature, the conductivity sensor such as used in Tanaka et al. (2014); Tanaka et al. (2021) and Sakamoto et al. (2018) is the most commonly used sensor for ULD (Coulombe et al., 2023), probably because of its versatility and availability in different types. However, devices using this type of sensor were unable to distinguish urine from sweat, resulting in false messages convey to the patient (Fernandes et al., 2011). This issue can arise from physical effort, hot weather conditions or women experiencing menopause. To overcome this situation, Wang et al. (2009) proposed a system incorporating an accelerometer and inclinometer. Their system has the capability to detect 0.5 mL of urine as well as the patient’s activities (walking in treadmill, jumping, etc.). However, no research in the literature has assessed the impact of sequential activities (rising from a chair and sitting down, lying down and standing up, etc.) on sensor’s results. Thus, further research is required to fully address false triggering challenges. To ensure that the sensor will not easily damaged (loss of conductivity due to usage, washing of the electrodes made of conductive yarns, etc.) Fischer et al. (2019) introduced a reference electrode to enhance longevity and allow flexibility in adjusting the threshold value for detecting incontinence. All systems exploiting conductivity sensors have an e-textile level of 2, except the study of Nag et al. (2017).
This type of sensor has either 0 or very low energy consumption until urine is present. Indeed, it is important to note that conductivity is influenced by factors such as temperature and humidity. Changes in either of these factors can alter conductivity measurements and decrease the performance of urinary leakage systems.
Gas sensors demonstrated valuable results in detecting specific gas molecules found in urine with very high sensitivity. However, the devices involving gas sensors seem to require the use of humidity and temperature sensors. According to Esfahani et al. (2020) and Ortiz Pérez et al. (2017), ammonia, acetone, ethyl acetate, etc., can be detected with a concentration as low as 1 ppm. However, several limitations should be noted. Firstly, one drawback from the survey works is that they have been tested in controlled lab conditions where gas flow is regulated. In real-life situations, we believe that factors such as body movement, wind, proximity to areas where dogs or other individuals have urinated, etc., could significantly affect the accuracy of their findings. Secondly, the gas sensors have a continuous active energy consumption, which means they constantly consume energy during data collection. The two gas detectors utilized in the literature (Ortiz Pérez et al., 2017; Esfahani et al., 2020) are rather large for discreet utilization in ULD system. It should be noted that neither of these gas detector devices are e-textile-based (Table 1). Indeed, they are separate devices that need to be added to the patient, typically in the belt region or other applicable areas (Figure 1).
Tekcin et al. (2022) have experimented with a single humidity sensor used in different ways contrary to those reported in (Ortiz Pérez et al., 2017; Esfahani et al., 2020). Indeed, the authors demonstrated promising results by achieving a level 4 of e-textile: flexible, washable and durable fully integrated sensor in fabric. This humidity sensor has high sensitivity allowing it to detect 0.1 mL of saltwater. However, it was unable to distinguish urine from sweat.
Biosensor was capable of detecting concentrations as low as 10−4 mol/L of glucose or uric acid found in urine (Su et al., 2022). This sensor does not consume energy when urine is absent. It distinguishes itself from others by generating its own electric signal through a chemical reaction upon contact with urine. As an e-textile of level 2, with low energy requirement, and high sensitivity towards specific molecules present in urine, the biosensors have promising applications for urine incontinence detection systems (comfortable, convenient and efficient), as well as large health monitoring applications.
The light sensor developed by Ishida et al. (2012) stands out as the only photosensor among the examined articles. They demonstrated the capability to successfully detect urine by measuring the change in voltage when a liquid leaks into the patient’s underwear. This can be promising for patients with occasional urinary incontinence who prefer not to wear a diaper. However, further research is required for the development of a smaller and more discreet system that can be integrated into clothing. As seen in Figure 1, this system can be uncomfortable to wear due to its heaviness. Although this device is not an e-textile, exploring the possibility of incorporating it into clothing is a viable option worth considering.
All non-Textile systems except Nag et al. (2017) have exploited commercial sensors such as HumidIcon 6,100 series (Ortiz Pérez et al., 2017), BME280 (Esfahani et al., 2020), CCS811 (Esfahani et al., 2020), SFH4110 (Ishida et al., 2012). Based on the technical specifications (Honeywell HIH6100, 2023; ams-OSRAM USA INC, 2007; ams Datasheet, 2016; Bosch Sensortec, 2018), these sensors cannot be easily damaged except in case of improper operations during embedding procedure. Moreover, in a product intended to be used for UL, we believe that sensors cannot be easily damaged when they are enclosed in a small casing (Ortiz Pérez et al., 2017; Esfahani et al., 2020).
The e-Textile systems are manufactured products in which electronic components are embroidered in a textile. Although, Fernandes et al. (Fernandes et al., 2011), emphasized the development of a robust circuit, no research work has yet diagnosed damaged sensors and their robustness by establishing a clear testing procedure. Nevertheless, we cannot deny that embedded sensors in a textile for UL detection remain a new area research. The process requires several tests before confirming its robustness, including its durability under different usages.
One of the most challenging aspects of a UL system is to develop a solution that is both comfortable and discreet, allowing patients to wear it with ease. Esfahani et al. (2020) concluded that although their system seemed to be effective, it would be too large for a person to wear comfortably. Studies attempting to design a small and portable device, suitable for comfortable use, failed to propose a device with a rapid response time. In study conducted by Fernandes et al. (2011), it was noted that when trying to fabricate a more discreet system, the detection accuracy decreases, leading to potential false alarms.
In this paper, we aimed to provide an overview of the current state of the art and guide the future developments by reviewing the recent advances in non-invasive technologies for UI detection. To date, no study has proposed a device considering the type of UI. We therefore hypothesize that any non-invasive device could potentially be used for any type of incontinence. Although this paper presents significant features for device design, it also has some limitations. Firstly, three of the included articles are from the same group of researchers, under the same project. This can introduce statistical biases in our results, as any shared methods among these studies may influence the overall findings. Secondly, the research was solely conducted using data from four databases, potentially excluding other essential articles. To achieve a more comprehensive review, additional databases could be explored in future works allowing a broader understanding of the advancements of UI devices. The next innovations for UI detection should also evaluate the physical activities of the user. Machine learning and other models can be integrated in order to face the complications of human activity in the real world.
MB: Conceptualization, Data curation, Methodology, Writing–original draft, Writing–review and editing. IH: Conceptualization, Data curation, Methodology, Writing–original draft, Writing–review and editing. AT: Data curation, Formal Analysis, Investigation, Writing–review and editing. JA: Data curation, Formal Analysis, Investigation, Writing–review and editing. YO: Investigation, Writing–review and editing, Formal Analysis. LE: Supervision, Validation, Writing–review and editing, Funding acquisition. BC: Investigation, Writing–review and editing, Supervision, Validation, Funding Acquisition. NM: Conceptualization, Supervision, Validation, Writing–review and editing, Funding Acquisition.
The author(s) declare financial support was received for the research, authorship, and/or publication of this article. This research was supported by the Programme Audace—Fonds de recherche du Québec—FRQ and the Canada Research Chair on Biomedical Data Mining (950-231214).
The authors declare that the research was conducted in the absence of any commercial or financial relationships that could be construed as a potential conflict of interest.
All claims expressed in this article are solely those of the authors and do not necessarily represent those of their affiliated organizations, or those of the publisher, the editors and the reviewers. Any product that may be evaluated in this article, or claim that may be made by its manufacturer, is not guaranteed or endorsed by the publisher.
The Supplementary Material for this article can be found online at: https://www.frontiersin.org/articles/10.3389/fsens.2023.1279158/full#supplementary-material
Akdemir, Y., Dincer, F., Buyukuysal, C., Ozmen, U., Harma, M., and Harma, M. I. (2020). Comparison of outcomes of Burch colposuspension and transobturator tape and single incision needleless procedures (DynaMesh®-SIS minor) for the surgical treatment of female stress urinary incontinence patients who underwent combined pelvic reconstructive surgery or hysterectomy. Int. Urology Nephrol. 52 (9), 1665–1673. doi:10.1007/s11255-020-02549-0
Alsulihem, A., and Corcos, J. (2021). The use of vaginal lasers in the treatment of urinary incontinence and overactive bladder, systematic review. Int. Urogynecol J. 32 (3), 553–572. doi:10.1007/s00192-020-04548-2
ams Datasheet (2016). CCS811 ultra-low power digital gas sensor for monitoring indoor air quality. Available at: https://cdn.sparkfun.com/assets/learn_tutorials/1/4/3/CCS811_Datasheet-DS000459.pdf.
ams-OSRAM USA INC (2007). SFH4110 GaAs infrared emitter. Available at: https://mm.digikey.com/Volume0/opasdata/d220001/medias/docus/896/SFH_4110_Ver1.0.pdf.
Anand, A., Khan, S. M., and Khan, A. A. (2021). Stress urinary incontinence in females. Diagnosis and treatment modalities - past, present and the future. J. Clin. Urology 0, 205141582110445. doi:10.1177/20514158211044583
Aoki, Y., Brown, H. W., Brubaker, L., Cornu, J. N., Daly, J. O., and Cartwright, R. (2017). Urinary incontinence in women. Nat. Rev. Dis. Prim. 3, 17042. doi:10.1038/nrdp.2017.42
Bosch Sensortec (2018). BME280 Combined humidity and pressure sensor. Available: https://www.mouser.com/datasheet/2/783/BST-BME280-DS002-1509607.pdf.
Coulombe, A., Guérineau, J., and Mezghani, N. (2023). Smart apparel design for urinary incontinence detection. IOP Conf. Ser. Mater. Sci. Eng. 1266, 012007. IOP Publishing. doi:10.1088/1757-899X/1266/1/012007
Dumoulin, C. (2016). L'incontinence urinaire: La prévenir, la traiter. Les Éditions du CIUSSS du Centre-Sud-de-l'Île-de-Montréal.
Esfahani, S., Shanta, M., Specht, J. P., Xing, Y., Cole, M., and Gardner, J. W. (2020). “Wearable IoT electronic nose for urinary incontinence detection,” in 2020 IEEE SENSORS, Rotterdam, Netherlands, 25-28 October 2020, 1–4.
Fernandes, B., Gaydecki, P., Jowitt, F., and van den Heuvel, E. (2011). Urinary incontinence: a vibration alert system for detecting pad overflow. Assist. Technol. 23 (4), 218–224. doi:10.1080/10400435.2011.614675
Fischer, M., Renzler, M., and Ussmueller, T. (2019). Development of a smart bed insert for detection of incontinence and occupation in elder care. IEEE Access 7, 118498–118508. doi:10.1109/ACCESS.2019.2931041
Gandi, C., and Sacco, E. (2021). Pharmacological management of urinary incontinence: current and emerging treatment. Clin. Pharmacol. Adv. Appl. 13, 209–223. doi:10.2147/cpaa.s289323
Honeywell HIH6100 (2023). Humidity and temperature sensor Datasheet. Available: https://prod-edam.honeywell.com/content/dam/honeywell-edam/sps/siot/es-mx/products/sensors/humidity-with-temperature-sensors/honeywell-humidicon-hih6100-series/documents/sps-siot-humidicon-hih6100-series-product-sheet-009059-6-en-ciid-142165.pdf.
Ishida, K., Habata, T., Takeuchi, A., Tsuruta, H., Hirose, M., and Ikeda, N. (2012). “Study of light transmission through the underwear for development of a urinary incontinence sensor,” in International Conference on Biomedical Electronics and Devices (BIODEVICES), 311–314.
ISO/TR 16298 (2011). Textiles and textile products — smart (Intelligent) textiles— definitions, categorisation, applications and standardization needs.
Jung, Y. G., Koh, E., and Park, S. (2015). Advances in urine and feces disposal systems for the treatment of incontinence. Inf. Jpn. 18 (4), 1463–1468.
Lamin, E., Parrillo, L. M., Newman, D. K., and Smith, A. L. (2016). Pelvic floor muscle training: underutilization in the USA. Curr. Urol. Rep. 17 (2), 10. doi:10.1007/s11934-015-0572-0
Milsom, I., Altman, D., Cartwright, R., Lapitan, M., Nelson, R., Sillén, U., et al. (2013). “Epidemiology of urinary incontinence (UI) and other lower urinary tract symptoms (LUTS), pelvic organ prolapse (POP) and anal incontinence (AI),” in Incontinence: 5th International Consultation on Incontinence, Paris, February 2012, 15–107. ICUD-EAU.
Milsom, I., and Gyhagen, M. (2019). The prevalence of urinary incontinence. Climacteric 22 (3), 217–222. doi:10.1080/13697137.2018.1543263
Nag, A., Mukhopadhyay, S., and Kosel, J. (2017). “Urinary incontinence monitoring system using laser-induced graphene sensors,” in 2017 IEEE SENSORS, Glasgow, UK, 29 October 2017 - 01 November 2017, 1–3.
Opro9 (2022). Opro9. Available: https://www.opro9.com/fr/opro9-fr/(Accessed November 22, 2022).
Ortiz Pérez, A., Kallfaß-de Frenes, V., Filbert, A., Kneer, J., Bierer, B., Held, P., et al. (2017). Odor-Sensing system to support social participation of people suffering from incontinence. Sensors Switz. 17 (1), 58. doi:10.3390/s17010058
Porter, E., Raterink, A., and Farshkaran, A. (2022). Microwave-based detection of the bladder state as a support tool for urinary incontinence [bioelectromagnetics]. IEEE Antennas Propag. Mag. 64 (1), 112–122. doi:10.1109/MAP.2021.3129687
Sakamoto, H., Tanaka, A., Suematsu, R., Nakajima, Y., and Douseki, T. (2018). “Self-Powered wireless urinary-incontinence sensor system detecting urine amount and diaper change timing in under 10 minutes,” in 2018 12th International Symposium on Medical Information and Communication Technology (ISMICT), Sydney, NSW, Australia, 26-28 March 2018, 1–4.
Simegnaw, A. A., Malengier, B., Rotich, G., Tadesse, M. G., and Van Langenhove, L. (2021). Review on the integration of microelectronics for E-textile. Mater. (Basel) 14 (17), 5113. doi:10.3390/ma14175113
Smardii (2019). Smardii. Available: https://www.smardii.com/.
Su, H., Sun, F. Y., Lu, Z. P., Zhang, J. R., Zhang, W. Y., and Liu, J. (2022). A wearable sensing system based on smartphone and diaper to detect urine in-situ for patients with urinary incontinence. Sensors Actuators B-Chemical 357, 131459. doi:10.1016/j.snb.2022.131459
Tanaka, A., Utsunomiya, F., and Douseki, T. (2014). “Wearable self-powered diaper-shaped urinary-incontinence sensor suppressing response-time variation with 0.3-V start-up converter,” in Sensors, 684–687.
Tanaka, A., Imoto, S., and Douseki, T. (2021). “Urine-activated battery using conductive yarn electrodes with folded backstitch structure for self-powered urinary incontinence sensor,” in CEUR workshop proceedings, 126–131.
Tekcin, M., Sayar, E., Yalcin, M. K., and Bahadir, S. K. (2022). Wearable and flexible humidity sensor integrated to disposable diapers for wetness monitoring and urinary incontinence. Electronics 11 (7), 1025. doi:10.3390/electronics11071025
The Canadian Continence Foundation (2014). Incontinence: the Canadian perspective. Cameron Institute.
Therapee (2022). The world's #1 bedwetting solution. Available: https://www.bedwettingtherapy.com/(Accessed November 22, 2022).
Vaughan, C. P., and Markland, A. D. (2020). Urinary incontinence in women. Ann. Intern. Med. 172 (3), ITC17–ITC32. doi:10.7326/aitc202002040
Keywords: urinary incontinence, urinary leakage, sensor, detection, non-invasive
Citation: Ben Arous M, Haddar I, Truong A, Ayena JC, Ouakrim Y, El Kamel L, Chikhaoui B and Mezghani N (2023) Non-invasive wearable devices for urinary incontinence detection—a mini review. Front. Sens. 4:1279158. doi: 10.3389/fsens.2023.1279158
Received: 17 August 2023; Accepted: 13 October 2023;
Published: 09 November 2023.
Edited by:
Wen-Sheng Zhao, Hangzhou Dianzi University, ChinaReviewed by:
Tao Wang, Hefei University of Technology, ChinaCopyright © 2023 Ben Arous, Haddar, Truong, Ayena, Ouakrim, El Kamel, Chikhaoui and Mezghani. This is an open-access article distributed under the terms of the Creative Commons Attribution License (CC BY). The use, distribution or reproduction in other forums is permitted, provided the original author(s) and the copyright owner(s) are credited and that the original publication in this journal is cited, in accordance with accepted academic practice. No use, distribution or reproduction is permitted which does not comply with these terms.
*Correspondence: Myriam Ben Arous, bXlyaWFtLmJlbi5hcm91c0B1bW9udHJlYWwuY2E=
Disclaimer: All claims expressed in this article are solely those of the authors and do not necessarily represent those of their affiliated organizations, or those of the publisher, the editors and the reviewers. Any product that may be evaluated in this article or claim that may be made by its manufacturer is not guaranteed or endorsed by the publisher.
Research integrity at Frontiers
Learn more about the work of our research integrity team to safeguard the quality of each article we publish.