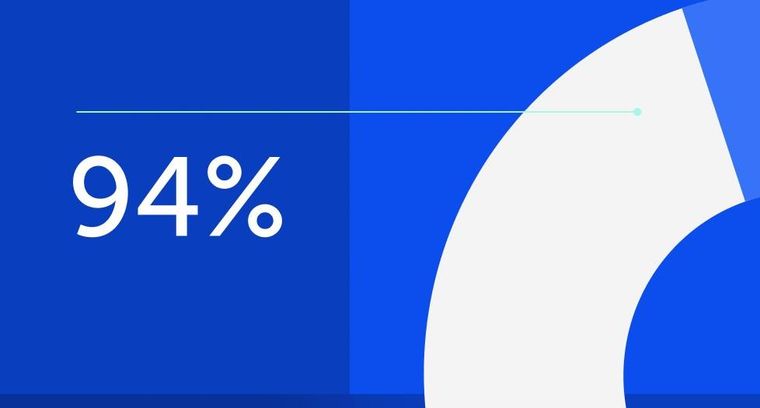
94% of researchers rate our articles as excellent or good
Learn more about the work of our research integrity team to safeguard the quality of each article we publish.
Find out more
BRIEF RESEARCH REPORT article
Front. Robot. AI, 09 July 2020
Sec. Virtual Environments
Volume 7 - 2020 | https://doi.org/10.3389/frobt.2020.00081
This article is part of the Research TopicVirtual and Robotic Embodiment – From Neuroscience to Virtual Reality and RoboticsView all 8 articles
In our study, we tested a combination of virtual reality (VR) and robotics in the original adjuvant method of post-stroke lower limb walk restoration in acute phase using a simulation with visual and tactile biofeedback based on VR immersion and physical impact to the soles of patients. The duration of adjuvant therapy was 10 daily sessions of 15 min each. The study showed the following significant rehabilitation progress in Control (N = 27) vs. Experimental (N = 35) groups, respectively: 1.56 ± 0.29 (mean ± SD) and 2.51 ± 0.31 points by Rivermead Mobility Index (p = 0.0286); 2.15 ± 0.84 and 6.29 ± 1.20 points by Fugl-Meyer Assessment Lower Extremities scale (p = 0.0127); and 6.19 ± 1.36 and 13.49 ± 2.26 points by Berg Balance scale (p = 0.0163). P-values were obtained by the Mann–Whitney U test. The simple and intuitive mechanism of rehabilitation, including through the use of sensory and semantic components, allows the therapy of a patient with diaschisis and afferent and motor aphasia. Safety of use allows one to apply the proposed method of therapy at the earliest stage of a stroke. We consider the main finding of this study that the application of rehabilitation with implicit interaction with VR environment produced by the robotics action has measurable significant influence on the restoration of the affected motor function of the lower limbs compared with standard rehabilitation therapy.
The problem of rehabilitation of acute and subacute cerebrovascular disorders does not lose its relevance at all stages of the disease. The use of modern understanding of neuroplasticity expands rehabilitation opportunities, making them available at different periods of stroke and other neurological disease (Khan et al., 2016; Alia et al., 2017). A comprehensive rehabilitation approach requires rehabilitation from the earliest time of the disease in order to achieve a meaningful recovery of lost functions in the future. From the point of view of motor rehabilitation in early stroke, one of the most important tasks is a patient's verticalization and restoration of overall mobility (Winstein et al., 2016; Powers et al., 2019).
The use of virtual reality (VR) in adjuvant methods of rehabilitation has received much attention recently (Cervera et al., 2018; Maier et al., 2019). Using VR as a tool for repeatedly and naturalistically demonstrating scenes of interaction with real-world objects can influence intercortical interactions in the form of their activation or inhibition in both motor and premotor areas (Léonard and Tremblay, 2006; Laver et al., 2017). There are quite powerful cortico-cortical connections between the occipital, frontal, and parietal lobes, which are used together in the processing of visual, motor, and proprioceptive information (Dum, 2005; Borra and Luppino, 2017). There is evidence that a significant number of neurons in the motor, premotor, and parietal regions are modulated by visual information (Caldara et al., 2004; Ertelt et al., 2007), and virtual avatar movements reveal common parieto-frontal links (Adamovich et al., 2009; Mellet et al., 2009).
Thus, our study is based on previous findings that visual stimulation (in terms of VR) and physical impact to affected limbs contribute to increasing the efficiency of motor rehabilitation of patients with acute cerebrovascular accident.
The purpose of the study was to test the effect of a new method of supplementary motor rehabilitation including VR + robotics therapy on the restoration of the affected walking function in the acute and early recovery periods of ischemic stroke with supratentorial localization.
The study continued from November 2018 to October 2019 and included rehabilitation of stroke patients using the ReviVR walk simulator (Figures 1A,B). The ReviVR walk simulator is a proprietary product developed in Samara State Medical University and is protected by Russian and international patents (priority date 29.12.2016; RU2655200C1, WO2018124940A1). The simulator provides immersion in a life-like VR environment and walking imitation with visual and tactile biofeedback based on physical impact—alternate pressing to the soles, synchronized with the “steps” of the avatar in the VR environment.
Figure 1. ReviVR rehabilitation walk simulator: (A) Equipment overview. (B) Patient in a chair with pneumatic orthoses on the feet. (C) Patient's avatar and virtual environment overview, third-person view. (D) View for a patient in VR-headset, first-person view.
The patient was wearing a VR headset and two orthoses equipped with four-chamber pneumatic cuffs each fixed on both feet. The chambers in the cuffs were inflated sequentially when a virtual avatar was making a “step.” Such sequential inflation of the four chambers imitated the contact of the sole with the surface during a real walk. The operation of the pressure valves in the chambers (pumping and venting) was synchronized to provide pace of the “walking” to 26 taps per minute on each sole (equivalent to 0.43 “step” per second or one “step” every 2.3 s). The maximal pressure of the chambers on the soles was 0.5 kg/cm2 of the plantar surface of the foot. Stimulation of the soles with pneumatic cuffs occurred for both a paralyzed and a healthy limb. The patients saw the virtual environment and their avatar from the “first-person view” in a walking position. By turning a head, the patient could observe the movement of the limbs of the virtual avatar (Figures 1C,D).
Below are the criteria for the inclusion of a patient in the study (in the presence of all of the following):
1. Age 18–80 years with the first-occurred acute ischemic cerebral circulation disorder in the carotid pool.
2. An acute period of cerebrovascular accident: no more than 5 days from the date of stroke.
3. One confirmed focus of ischemic stroke of supratentorial localization according to computerized tomography (CT).
4. Motor disorders in the lower extremities in the form of a central paresis three or less points of a six-point Medical Research Council scale for Muscle Strength (MRC, 1981).
5. Ability and willingness of the patient to comply with the protocol of study requirements.
6. Signed written informed consent.
Below are the exclusion criteria (in the presence of at least one of the following):
1. Explicit cognitive impairment: 10 or less points according to the Montreal Cognitive Assessment scale (MoCA, 1996).
2. Neurological diseases that cause a decrease in muscle strength or an increase in muscle tone in the lower extremities due to any pathology.
3. Clinically significant limitation of the amplitude of passive movements in the lower extremities.
4. Lack of lower limb due to amputation.
5. Any medical condition, including a mental illness or epilepsy, that might affect the interpretation of the results of the study, the conduct of the study, or patient safety.
6. The abuse of alcohol or narcotics within 12 months preceding the moment of inclusion in the study.
7. Treatment with botulinum toxin type A or B in the previous 6 months prior to inclusion in the study.
8. Surgery in the previous 6 months prior to inclusion in the study; for example, abdominal, back, leg, or knee surgery.
9. The severity of the patient's condition according to neurological or somatic status, which does not allow full rehabilitation intervention.
10. Blindness in one or both eyes, or explicit visual impairment more than 20/30 according to Snellen Eye Chart.
The study was performed in the neurology department of Samara Regional Clinical Hospital named after VD Seredavin (53 patients) and in the Research Center of Cerebrovascular Pathology and Stroke, Ministry of Health of the Russian Federation, Moscow (9 patients). The study was approved by the local ethics committee of the Samara Regional Clinical Hospital named after VD Seredavin (protocol #146, 14.03.2018).
All patients included in the study underwent standard rehabilitation. According to their functional state, in addition to medication, they could receive physiotherapy and neuromuscular electrical stimulation (NMES). The choice of methods and the scope of standard therapy by attending clinicians were based on the set of rehabilitation tasks and the functional state of the patient.
Sixty-two patients (M/F, left or right primary hemisphere ischemic stroke) were randomized to the Control (N = 27) and Experimental (N = 35) groups. Patients' clinical data at the moment of inclusion in the study are presented in Table 1.
A patient in the Experimental group, initially lying in a bed and then, after 2–3 days, sitting in a chair, received 10 rehabilitation sessions with ReviVR, 15 min each. Thus, the total adjuvant therapy duration was 2.5 h for each patient. Adverse events were monitored throughout the study and were not recorded.
Patients in the Control group were also able to receive rehabilitation with the ReviVR simulator after completion of their participation in the study.
The study completion visit was carried out at the day of discharge of the patient from the hospital, usually on the 21st day. This visit included an assessment on the study scales by an independent neurologist, who was blinded for the patient's rehabilitation group. Rehabilitation performance was evaluated using NIHSS, RMI, FMA-LE, and BBS scales.
Statistical analysis was performed using the STATISTICA data analysis software system, version 12 (StatSoft Inc., 2014, www.statsoft.com).
It should be noted that patients in both the Control and Experimental groups showed positive rehabilitation dynamics that was observed when assessing the motor function of the lower extremities when performing isolated motor tasks by an affected extremity and during synergistic movements of both lower extremities.
To assess the effectiveness of rehabilitation in the compared groups, we evaluated the progress points (the difference in scores after and before rehabilitation). The progress points were checked for normality of the distribution with the Shapiro–Wilk test. All data showed a non-normal distribution (p < 0.02). Assessment of progress in groups was carried out using the two-tailed Mann–Whitney U test.
The following are the significant rehabilitation progress points in Control and Experimental groups, respectively: −1.26 ± 0.62 and −2.83 ± 0.32 points by the NIHSS scale (p = 0.0003); 1.56 ± 0.29 and 2.51 ± 0.31 points by the Rivermead Mobility Index (p = 0.0286); 2.15 ± 0.84 and 6.29 ± 1.20 points by the Fugl–Meyer Assessment Lower Extremities scale (p = 0.0127); and 6.19 ± 1.36 and 13.49 ± 2.26 points by the Berg Balance scale (p = 0.0163). A higher decrease is better for the NIHSS scale; a higher increase is better for the RMI, FMA-LE, and BBS scales. Summary information on rehabilitation is presented in Table 2 and Figure 2.
Figure 2. The rehabilitation progresses. Comparison of the Control (N = 27) and Experimental (N = 35) groups by the study scales. The difference in scores after and before rehabilitation. P-value obtained by two-tailed Mann–Whitney U test. (A) NIHSS progress. (B) RMI progress. (C) FMA-LE progress. (D) BBS progress.
We compared our research with the most similar studies performed in the last 6 years for VR- and robotics-based lower limb rehabilitation (Lee et al., 2014; Xiang et al., 2014; Givon et al., 2015; Ko et al., 2015; Song and Park, 2015; Gibbons et al., 2016; Lo et al., 2017; Darbois et al., 2018; Clark et al., 2019). These studies used the same clinical scales to measure the rehabilitation progress of lower limb function and/or were performed in the acute period of ischemic stroke. Authors also noted an increase in the effectiveness of rehabilitation when using adjuvant therapy.
Evaluating the results of similar studies and our own results, we believe that this influence is due to the impact on motor and premotor areas of neuroplasticity caused by visual, sensory, and cognitive evoked intercortical interactions. The patient's involvement in the virtual environment and the use of the game-like component during the rehabilitation of the lower extremities greatly improve the motor function. Our results are consistent with the findings that patients assimilated the virtual lower limbs as if they were their own legs (Shokur et al., 2016), and we assume that, in our case, there was a similar mechanism of identification (agency) that had a positive effect on neuroplasticity and motor recovery.
The main distinctions of our study are as follows:
A. We used the complex activation of neuroplasticity by immersing patients in synchronous visual and sensory passive interaction with virtual environments.
B. In our study, we used a relatively simple device to mimic the proprioception sense during the rehabilitation and achieved the progress comparable to the results of interventions with sophisticated robotic equipment that moves the whole lower limbs or the whole body to imitate independent walk.
C. The concept of rehabilitation and the design of equipment allow rehabilitation of the following patients:
− in acute stroke (all 35 patients in the Experimental group);
− bedridden at the beginning of the study (25 of 35 patients);
− with severe paresis, diaschisis, or persisting low muscle tone of the lower limb (27 of 35 patients);
− with afferent and motor aphasia (17 of 35 patients);
− with restrictions for verticalization due to cardiac arrhythmia, which can cause a cardioembolic stroke and the risk of thromboembolic complications (20 of 35 patients).
Our study shows that an adjuvant post-stroke VR + robotics therapy of the lower extremities in acute phase using interaction via realistic proprioceptive and implicit tactile impacts significantly improves the performance of standard rehabilitation.
We suggest that the use of explicit interaction within walking synergy may show better clinical effects of rehabilitation. We will clarify this hypothesis in our further work.
All datasets generated for this study are included in the article/Supplementary Material.
The studies involving human participants were reviewed and approved by Local Ethics Committee of the Samara Regional Clinical Hospital named after VD Seredavin (protocol #146, 14.03.2018). The patients/participants provided their written informed consent to participate in this study.
AK, AZ, and GI were responsible for the design and the conception of the study. AZ, EK, and YB were responsible for the neurophysiology aspects, organization of patients' enrollment and evaluation, and gathering of the experimental data. AZ and VB contributed to the statistical analysis, manuscript writing, and revision. All authors contributed to the article and approved the submitted version.
This study was implemented with the financial support of the Ministry of Science and Higher Education of the Russian Federation (grant RFMEFI60418X0208).
AZ, AK, and EK are employees of Samara State Medical University, which developed the ReviVR simulator used in the study. VB was employed by company IT Universe Ltd.
The remaining authors declare that the research was conducted in the absence of any commercial or financial relationships that could be construed as a potential conflict of interest.
We thank Eugene Tunik (Bouvé College of Health Sciences, Northeastern University, Boston), Alexei Ossadtchi (NRU HSE, Moscow), Ilia Borishchev (IT Universe Ltd, Toronto), and Sergey Agapov (IT Universe Ltd, Samara) for invaluable advisory and Dmitry Krasnorylov (Samara Regional Clinical Hospital named after VD Seredavin) for collecting biomedical data.
The Supplementary Material for this article can be found online at: https://www.frontiersin.org/articles/10.3389/frobt.2020.00081/full#supplementary-material
Adamovich, S. V., Fluet, G. G., Tunik, E., and Merians, A. S. (2009). Sensorimotor training in virtual reality: a review. Neurorehabilitation 25, 29–44. doi: 10.3233/nre-2009-0497
Alia, C., Spalletti, C., Lai, S., Panarese, A., Lamola, G., Bertolucci, F., et al. (2017). Neuroplastic changes following brain ischemia and their contribution to stroke recovery: novel approaches in neurorehabilitation. Front. Cell. Neurosci. 11:76. doi: 10.3389/fncel.2017.00076
Borra, E., and Luppino, G. (2017). Functional anatomy of the macaque temporo-parieto-frontal connectivity. Cortex 97, 306–326. doi: 10.1016/j.cortex.2016.12.007
Caldara, R., Deiber, M.-P., Andrey, C., Michel, C. M., Thut, G., and Hauert, C.-A. (2004). Actual and mental motor preparation and execution: a spatiotemporal ERP study. Exp. Brain Res. 159, 389–399. doi: 10.1007/s00221-004-2101-0
Cervera, M. A., Soekadar, S. R., Ushiba, J., Millán, J. D. R., Liu, M., Birbaumer, N., et al. (2018). Brain-computer interfaces for post-stroke motor rehabilitation: a meta-analysis. Ann. Clin. Transl. Neurol. 5, 651–663. doi: 10.1002/acn3.544
Clark, W. E., Sivan, M. J., and O'Connor, R., undefined (2019). Evaluating the use of robotic and virtual reality rehabilitation technologies to improve function in stroke survivors: a narrative review. J. Rehabil. Assist. Technol. Eng. 6:205566831986355. doi: 10.1177/2055668319863557
Darbois, N., Guillaud, A., and Pinsault, N. (2018). Do robotics and virtual reality add real progress to mirror therapy rehabilitation? A scoping review. Rehabil. Res. Pract. 2018, 1–15. doi: 10.1155/2018/6412318
Dum, R. P. (2005). Frontal lobe inputs to the digit representations of the motor areas on the lateral surface of the hemisphere. J. Neurosci. 25, 1375–1386. doi: 10.1523/jneurosci.3902-04.2005
Ertelt, D., Small, S., Solodkin, A., Dettmers, C., Mcnamara, A., Binkofski, F., et al. (2007). Action observation has a positive impact on rehabilitation of motor deficits after stroke. Neuroimage 36, T164–T173. doi: 10.1016/j.neuroimage.2007.03.043
Gibbons, E. M., Thomson, A. N., Noronha, M. D., and Joseph, S. (2016). Are virtual reality technologies effective in improving lower limb outcomes for patients following stroke–a systematic review with meta-analysis. Top. Stroke Rehabil. 23, 440–457. doi: 10.1080/10749357.2016.1183349
Givon, N., Zeilig, G., Weingarden, H., and Rand, D. (2015). Video-games used in a group setting is feasible and effective to improve indicators of physical activity in individuals with chronic stroke: a randomized controlled trial. Clin. Rehabil. 30, 383–392. doi: 10.1177/0269215515584382
Khan, F., Amatya, B., Galea, M. P., Gonzenbach, R., and Kesselring, J. (2016). Neurorehabilitation: applied neuroplasticity. J. Neurol. 264, 603–615. doi: 10.1007/s00415-016-8307-9
Ko, Y., Ha, H., Bae, Y.-H., and Lee, W. (2015). Effect of space balance 3D training using visual feedback on balance and mobility in acute stroke patients. J. Phys. Ther. Sci. 27, 1593–1596. doi: 10.1589/jpts.27.1593
Laver, K. E., Lange, B., George, S., Deutsch, J. E., Saposnik, G., and Crotty, M. (2017). Virtual reality for stroke rehabilitation. Cochrane Database Syst. Rev. 11:CD008349. doi: 10.1002/14651858.cd008349.pub4
Lee, C.-H., Kim, Y., and Lee, B.-H. (2014). Augmented reality-based postural control training improves gait function in patients with stroke: randomized controlled trial. Hong Kong Physiother. J. 32, 51–57. doi: 10.1016/j.hkpj.2014.04.002
Léonard, G., and Tremblay, F. (2006). Corticomotor facilitation associated with observation, imagery and imitation of hand actions: a comparative study in young and old adults. Exp. Brain Res. 177, 167–175. doi: 10.1007/s00221-006-0657-6
Lo, K., Stephenson, M., and Lockwood, C. (2017). Effectiveness of robotic assisted rehabilitation for mobility and functional ability in adult stroke patients. JBI Database Syst. Rev. Implement. Rep. 15, 3049–3091. doi: 10.11124/jbisrir-2017-003456
Maier, M., Ballester, B. R., Duff, A., Oller, E. D., and Verschure, P. F. M. J. (2019). Effect of specific over nonspecific vr-based rehabilitation on poststroke motor recovery: a systematic meta-analysis. Neurorehabil. Neural Repair 33, 112–129. doi: 10.1177/1545968318820169
Mellet, E., Laou, L., Petit, L., Zago, L., Mazoyer, B., and Tzourio-Mazoyer, N. (2009). Impact of the virtual reality on the neural representation of an environment. Hum. Brain Mapp. 31, 1065–1075. doi: 10.1002/hbm.20917
Powers, W. J., Rabinstein, A. A., Ackerson, T., Adeoye, O. M., Bambakidis, N. C., Becker, K., et al. (2019). Guidelines for the early management of patients with acute ischemic stroke: 2019 update to the 2018 guidelines for the early management of acute ischemic stroke: a guideline for healthcare professionals from the American Heart Association/American Stroke Association. Stroke 50, e344–e418. doi: 10.1161/str.0000000000000211
Shokur, S., Gallo, S., Moioli, R. C., Donati, A. R. C., Morya, E., Bleuler, H., et al. (2016). Assimilation of virtual legs and perception of floor texture by complete paraplegic patients receiving artificial tactile feedback. Sci. Rep. 6:32293. doi: 10.1038/srep32293
Song, G. B., and Park, E. C. (2015). Effect of virtual reality games on stroke patients' balance, gait, depression, and interpersonal relationships. J. Phys. Ther. Sci. 27, 2057–2060. doi: 10.1589/jpts.27.2057
Winstein, C. J., Stein, J., Arena, R., Bates, B., Cherney, L. R., Cramer, S. C., et al. (2016). Guidelines for adult stroke rehabilitation and recovery. Stroke 47, e98–e169. doi: 10.1161/str.0000000000000098
Xiang, X., Yu-rong, M., Jiang-li, Z., Le, L., Guang-qing, X., and Dong-feng, H. (2014). Virtual reality-enhanced body weight-supported treadmill training improved lower limb motor function in patients with cerebral infarction. Chin. J. Tissue Eng. Res. 18:1143. doi: 10.3969/j.issn.2095-4344.2014.07.026
Keywords: stroke, rehabilitation, virtual reality, tactile feedback, biofeedback
Citation: Zakharov AV, Bulanov VA, Khivintseva EV, Kolsanov AV, Bushkova YV and Ivanova GE (2020) Stroke Affected Lower Limbs Rehabilitation Combining Virtual Reality With Tactile Feedback. Front. Robot. AI 7:81. doi: 10.3389/frobt.2020.00081
Received: 12 November 2019; Accepted: 19 May 2020;
Published: 09 July 2020.
Edited by:
Doron Friedman, Interdisciplinary Center Herzliya, IsraelReviewed by:
Solaiman Shokur, École Polytechnique Fédérale de Lausanne, SwitzerlandCopyright © 2020 Zakharov, Bulanov, Khivintseva, Kolsanov, Bushkova and Ivanova. This is an open-access article distributed under the terms of the Creative Commons Attribution License (CC BY). The use, distribution or reproduction in other forums is permitted, provided the original author(s) and the copyright owner(s) are credited and that the original publication in this journal is cited, in accordance with accepted academic practice. No use, distribution or reproduction is permitted which does not comply with these terms.
*Correspondence: Vladimir A. Bulanov, dmJAaXQtdW5pdmVyc2UucnU=
Disclaimer: All claims expressed in this article are solely those of the authors and do not necessarily represent those of their affiliated organizations, or those of the publisher, the editors and the reviewers. Any product that may be evaluated in this article or claim that may be made by its manufacturer is not guaranteed or endorsed by the publisher.
Research integrity at Frontiers
Learn more about the work of our research integrity team to safeguard the quality of each article we publish.