- 1Glickman Urological Institute, Cleveland Clinic Foundation, Cleveland, OH, United States
- 2Department of Cardiovascular and Metabolic Sciences, Cleveland Clinic Foundation, Cleveland, OH, United States
- 3Department of Urology, Tulane University School of Medicine, New Orleans, LA, United States
Research question: Male infertility accounts for almost half of all infertility cases worldwide, with idiopathic male infertility accounting for up to 30% of the cases. Sperm proteomics has revealed critical molecular pathway changes in men with infertility. However, the sperm mitochondrial proteome remains poorly understood. We attempted to answer the following question: Do patients with idiopathic primary male infertility exhibit a proteomic signature associated with mitochondrial dysfunction that could be used as a target for future mechanistic investigations?
Design: Patients with idiopathic primary infertility (20–40 years old) referred to the Cleveland Clinic between March 2012 and April 2014 were compared with fertile donor controls. Sperm proteins were analyzed using sodium dodecyl sulphate-polyacrylamide gel electrophoresis page (SDS-PAGE) and liquid chromatography-mass spectrometry (LC-MS), and differentially expressed proteins (DEPs) were identified based on significance test results and fold change thresholds. Protein expression was validated using western blotting.
Results: Proteomic analysis of pooled samples from fertile donors (n = 5) and patients with idiopathic primary infertility (n = 5) identified 1,134 proteins, including 344 DEPs. Mitochondrial dysfunction topped the ingenuity toxicity list. Analysis of expression levels of three mitochondrial proteins known to combat oxidative stress revealed that peroxiredoxin-5 (PRDX5) and superoxide dismutase 2 (SOD2), but not glutathione disulphide reductase, were significantly decreased in patient samples compared with those in fertile-donor samples.
Conclusions: This study revealed an association of downregulated expression of PRDX5 and SOD2 in sperm samples of patients with idiopathic primary male infertility. Our results support future mechanistic studies and development of advanced diagnostic methods to better identify men with mitochondria-related male infertility.
Introduction
Infertility is a widespread global health problem affecting an average of 17.5% of couples worldwide (1). Approximately 50% of these cases are attributed to male-factor infertility, either as the primary reason or as a significant contributing factor (2). The causes of male infertility vary and can be broadly classified as congenital, acquired, and idiopathic, each of which can negatively affect the crucial process of spermatogenesis (3). An idiopathic etiology accounts for 30% of all reported cases (4). Potential complex causes include factors such as defective sperm DNA integrity (5) abnormal sperm transcripts (6), and disrupted sperm protein expression, (7) all of which impair critical processes such as fertilization, the acrosome reaction, sperm-oocyte fusion, and embryo growth and development (8).
Spermatozoa, which are transcriptionally and translationally quiescent, depend entirely on existing proteins to perform essential biological functions (9). To date, research on the sperm proteome has identified and characterized different numbers of proteins (7, 10, 11) and the latest published number on sperm proteome is 7,000 gene products (12). These proteins play essential roles in energy production, apoptosis, oxidative stress response, cytoskeleton structure, flagellar movement, cell recognition, protein transport, and mitochondrial function (13, 14).
Mitochondria are the most important organelles in spermatozoa, providing cellular energy through ATP production to facilitate viability (15). Each spermatozoon contains between 50 and 75 mitochondria, which are located in the mid-piece and exhibit unique characteristics (16). Mitochondria are involved in several key functions, such as calcium homeostasis, generation of reactive oxygen species (ROS) (17–19), regulation of apoptotic pathways, and biosynthesis of steroid hormones (20). Defective mitochondrial function in spermatozoa has been linked to various pathologies and activities, including varicocele (21) and diabetes, leading to disorders in spermatogenesis due to the overproduction of ROS and inhibition of cell proliferation (22–24). Furthermore, mitochondrial damage has been linked to repetitive fertilization failure after intracytoplasmic sperm injection (ICSI) (25) and cigarette smoking in males (26). Studies have highlighted the importance of proper mitochondrial function for male fertility and reproductive health (15, 27, 28).
Some proteomics studies have analyzed the proteome of asthenozoospermia patients vs. normozoospermic individuals, with a predominant focus on mitochondrial proteins. Yet, there has been no clear clarification regarding whether the patients had idiopathic etiology or if the control group were fertile or infertile normozoospermic samples (29, 30). A recent study by Pacheco et al., analyzed the proteome of male infertility of unknown origin, including both idiopathic and unexplained male infertility samples (31). However, their control group included male partners from infertile couples who were normozoospermic with a female factor infertility. These individuals may not represent truly fertile normozoospermic males, as male factor infertility could also contribute to the infertility in these couples.
To date, there are no studies yet that have evaluated the proteome of idiopathic primary male infertility compared to proven fertile donors and using liquid chromatography-mass spectrometry (LC-MS) to evaluate any association between idiopathic primary male infertility and mitochondrial function. Consequently, no diagnostic tests based on the detection of mitochondrial proteins, are available to clinically diagnose mitochondrial dysfunction and idiopathic primary male infertility.
In this pilot study, we investigated whether patients with idiopathic primary male infertility exhibit a proteomic signature in the sperm that could potentially be used to identify mitochondrial dysfunction and treat the condition.
Materials and methods
Ethics statement
This study was conducted at the Andrology Centre, Cleveland Clinic, Cleveland, OH, USA. The proteomics data utilized were derived from our proteomic project on male infertility (IRB#11-451) in which we conducted multiple proteomic analyses on infertility patients with various etiologies, such as varicocele, primary infertility, and secondary infertility. In this study, we chose to focus on a group of idiopathic primary infertility patients. Our hypothesis evolved from preliminary findings in our broader proteomic analysis. For Western blot validation, we recruited a different set of participants following approval by the Cleveland Clinic Institutional Review Board (IRB#17-422). All individuals who participated in this study provided informed consent after signing a consent form.
We followed the recommendations for biomarker identification and qualification in clinical proteomics, the EQUATOR Network (32).
Sample and data collection
Patients (n = 5) with idiopathic primary infertility between the ages of 20–40 years old, who were referred to the Glickman Urological and Kidney Institute at Cleveland Clinic were included in this study. Idiopathic primary infertility was diagnosed under the following criteria: (1) Semen analysis showed at least one abnormality regarding concentration, motility, or normal sperm morphology, in line with the fifth edition of the World Health Organization (WHO) 2010 guidelines (33), (2) No specific cause for infertility or semen pathology was identified, (3) A normal fertility assessment was confirmed for the female partner. The control group (n = 5) consisted of fertile donor participants who were in good health, exhibited a normal body mass index, were non-smokers, refrained from alcohol consumption, displayed normozoospermia, with no high oxidative stress defined as ROS levels <93 RLU/sec (Relative Light Units per second), and had successfully fathered a child within the preceding two years. The exclusion criteria for both groups included smoking, female factor infertility, exposure to radiation or chemicals, fever in the previous 90 days, genetic defects (such as Y-chromosome microdeletions and karyotype abnormalities), leukocytospermia, infection or inflammation of the reproductive tract, sexually transmitted diseases, and azoospermia.
To ensure adequate protein concentration for subsequent proteomic analysis, a sample pooling strategy was adopted for both the patient and donor groups. A protein concentration-normalization procedure was implemented for each pooled sample to achieve diverse amalgamations of the samples within each group. This procedure ensured an equal contribution of protein from an equivalent number of spermatozoa per sample within the pool. This was achieved using an aliquot of 75 μl from samples exhibiting a protein concentration of 1.5 mg/ml, derived from a sperm concentration ranging between 80 and 100 million spermatozoa/ml.
For a comprehensive global proteomic analysis, each sample from both the control (fertile donor) and patient groups underwent triplicate runs and analysis. This approach ensured the robustness and reliability of the obtained results.
Semen analysis
Semen samples were collected at the Andrology Laboratory through masturbation, following a period of sexual abstinence lasting at least 2–7 days. The samples were allowed to liquefy completely for 30 min at 37°C, and semen analysis was conducted in accordance with the WHO (2010) (33) guidelines using a disposable Leja sperm counting chamber (Spectrum Technologies, Healdsburg, CA) to assess sperm count, motility, and morphology. After the routine semen analysis, samples were centrifuged at 13,000 g for 20 min. The seminal plasma was then removed, and the sperm samples were stored at −80°C.
Protein extraction and characterization
A detailed description of our protein-related research methods, including extraction, proteomics analysis, liquid chromatography-mass spectrometry (LC-MS) analysis, database searching, criteria for identification, and quantitative proteomics, is provided in Supplementary Material. In addition, a study flowchart is provided in Figure 1. The analysis was conducted in strict compliance with the Minimum Information about a Proteomics Experiment guidelines established by the Human Proteome Organization's Proteomics Standards Initiative (HUPO-PSI), a framework detailed in a 2013 publication by (34).
Bioinformatics analysis
We primarily utilized Ingenuity Pathway Analysis (IPA) from Ingenuity® Systems to perform functional pathway analysis of the differentially expressed proteins (DEPs). IPA facilitated the examination of the top canonical pathways, diseases, biofunctions, causal networks, and upstream regulators associated with the DEPs. In addition, we employed publicly available bioinformatics annotation tools and databases, including GO Term Finder (35), STRING database (36), UniProt for researching annotations of proteins, and the Database for Annotation, Visualization and Integrated Discovery (DAVID) (37). These resources enriched our analysis and allowed a comprehensive exploration of protein annotations and associated information.
Protein selection and validation using western blotting
To validate the results of our bioinformatics analysis, we employed western blot techniques using different sample sets from patients with idiopathic primary infertility and healthy donors. We successfully recruited four individuals for each group, adhering to the established inclusion and exclusion criteria previously used for proteomic analysis recruitment.
An aliquot of protein (20 µg) for each sample was resolved in a 4%–15% acrylamide gel using SDS-PAGE for 2 h at 90 V. Subsequently, the proteins were transferred onto polyvinylidene difluoride (PVDF) membranes and blocked for 90 min at room temperature of 20°C–25°C using a 5% non-fat milk solution in tris-buffered saline tween-20. For protein analysis, the membranes were incubated with the following specific primary antibodies overnight at 4°C: peroxiredoxin-5 (PRDX5; P30044, ab180587; Abcam, Cambridge, UK), superoxide dismutase 2 (SOD2; P04179, ab137037; Abcam), and glutathione disulphide reductase (GSR; P00390, ab128933; Abcam). Following incubation, the membranes were incubated with secondary Anti-Rabbit IgG VHH Single Domain (HRP; Abcam) at room temperature of 20°C–25°C for 1 h and then exposed to enhanced chemiluminescence (ECL) reagent (GE Healthcare, Marlborough, MA, USA) for 5 min. Chemiluminescence signals were detected using a Chemi-Doc™ MP Imaging System (Bio-Rad, Hercules, CA, USA). Glyceraldehyde 3-phosphate dehydrogenase (GAPDH) was used as a loading control, and the expression of each protein was normalized against GAPDH expression. We used HeLa Cells (ab157396; Abcam) as positive controls.
All the PVDF membranes used for protein identification were also subjected to total protein staining. The membranes were washed twice for 10 min in distilled water before incubating with total colloidal gold protein (Bio-Rad) for 2 h at room temperature with gentle shaking. After staining, the membranes were washed twice with distilled water for 10 min each, and densitometry images were captured in colorimetric mode using the Chemi-Doc™ MP Imaging System (Bio-Rad).
Statistical analysis
To assess the semen parameters, we used a combination of statistical tests. Analysis of variance (ANOVA) and two-sample t-tests were used to compare the quantitative measurements of protein expression between the infertile patients and fertile donors. For the statistical comparison of proteomic data, individual t-tests were performed to compare pairs of groups. Specifically, for each protein, we conducted a two-sample t-test to assess differences in expression between two groups (infertile patients vs. fertile controls). While no adjustments were made for multiple comparisons, this approach allowed for a focused examination of the proteins of interest in our study. The analysis was carried out using MedCalc Statistical Software (version 17.8; MedCalc Software, Ostend, Belgium), with statistical significance considered at p < 0.05.
To compare the results of the semen analysis we employed the Mann-Whitney test, with significance established at P < 0.05. Additionally, a two-tailed Student's t-test was used to compare the western blot intensity readings.
Results
Semen analysis
The parameters of the semen samples, including the concentration, motility and morphology are shown in Table 1. Fertile donors exhibited normal sperm parameters while idiopathic infertile patients presented with at least one abnormal sperm parameter. While all patients had high level of abnormal sperm morphology, no significant difference was observed in the sperm concentration, motility, and morphology between the two groups.
Proteomic profiling
Proteomic analysis of pooled seminal samples from the fertile donor cohort (n = 5) and patients with idiopathic primary infertility (n = 5) led to the identification of 1,134 proteins overall, with a subset of 141 proteins was present exclusively in the fertile donor group, nine proteins were present exclusively in patients with idiopathic primary infertility and 344 proteins were deferentially expressed (as described in the Supplementary Material A) with 235 proteins underexpressed and 109 proteins overexpressed. The 1,134 proteins fell into distinct abundance categories: 559 were categorized as very low abundance, 273 as low abundance, 224 as medium abundance, and 78 as high abundance (see description in Supplementary Material B).
Bioinformatics analysis
We utilized DAVID software for functional annotation analysis to elucidate the roles of the proteins exclusively expressed in fertile donors, proteins exclusively expressed in patients with idiopathic infertility and for DEPs in terms of biological processes, cellular components, and molecular function. The proteins that were unique to fertile donors were involved in electron transport, aerobic respiration, electron transport, ubiquitin system, cilium movement and protein folding. Proteins unique to patients with idiopathic infertility were involved in regulation od DNA replication and transcription, post translation modification, cell cycle G1/S phase and cell myosis (Table 2). Seventy-nine clusters were reported for functional annotation clustering, and 78 DEPs were involved in mitochondrial function, thirty four in mitochondrial matrix and 28 in mitochondrial inner membrane (Table 3). In addition, we harnessed the capabilities of IPA software to conduct a more comprehensive investigation into the associated canonical pathways, diseases, and biofunctions of the identified DEPs. Tables 4, 5 summarize the top disease and biofunction results. Mitochondrial dysfunction was at the top of the tox list with 8.8% overlap (15/171), (P = 1.78E–11). DEPs were involved in skeletal and muscular disorders, organismal injury and abnormalities, endocrine system disorder, neurological disease, and hereditary disorder. Most of the DEP's of them were involved in cell death and survival (n = 103), small molecule biochemistry (n = 51), nucleic acid metabolism (n = 29), translation modification (n = 18) and protein folding (n = 18). In addition, we also noticed that 32 DEP were involved in reproductive system development and function. Fifteen proteins from our DEPs were associated with the mitochondrial dysfunction pathways [-log (p-value) = 10.7 and ratio = 0.0877]: ACO2, ATP5F1A, ATP5F1B, ATP5PD, COX4I1, COX5B, GSR, NDUFS1, PARK7, PRDX5, SDHA, SOD2, TXNRD2, UQCRC2, and VDAC. We uploaded these 15 proteins to the STRING database for predicted protein-protein interaction networks and enrichment analysis, which revealed 73 interaction networks, as summarized in (Figure 2).
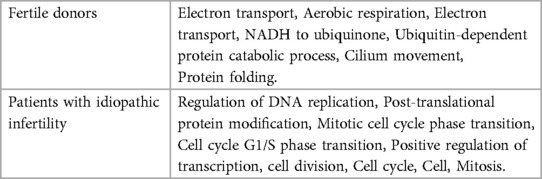
Table 2. Functional annotation clustering using DAVID bioinformatics resources for the unique proteins identified in fertile donors and patients with idiopathic infertility.
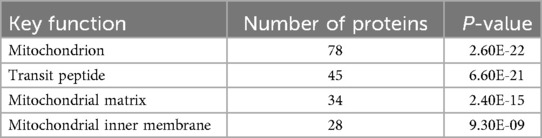
Table 3. Functional annotation clustering using DAVID bioinformatics resources for differentially expressed proteins.
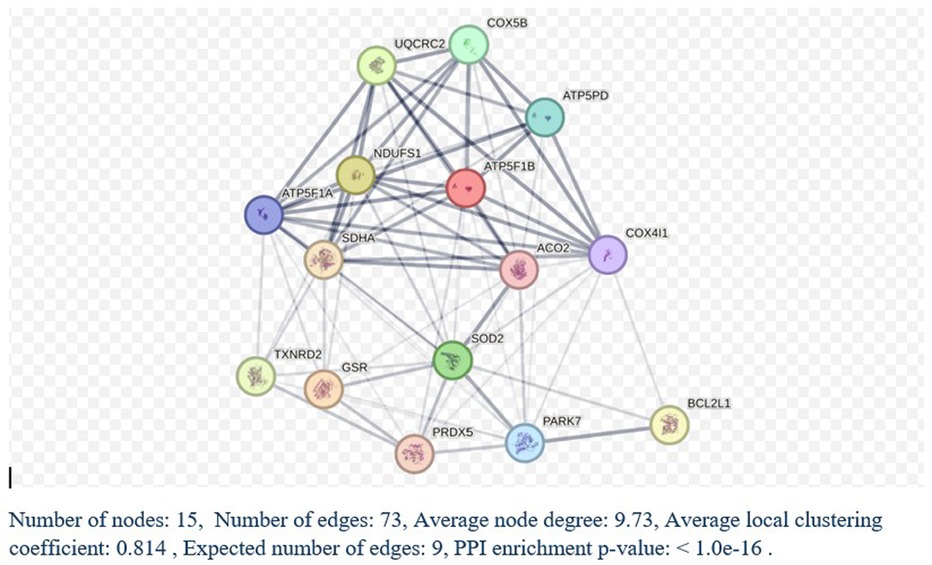
Figure 2. STRING analysis of protein-protein interactions. Network Status: number of nodes, 141; number of edges, 711; average node degree, 10.1; expected number of edges, 290; average local clustering coefficient, 0.433, and protein-protein interaction (PPI) enrichment P-value < 1.0E-16. a Count in network refers to the number of DEPs included in the STRING interaction network.
From the pool of identified proteins, we selected GSR, SOD2, and PRDX5 for subsequent western blot validation. The selection of these proteins was driven by several important factors relevant to our study. First, these proteins exhibited significant expression changes in our bioinformatic analysis of infertile patients (Table 6), making them highly pertinent to our research. Furthermore, they play essential roles in the male reproductive system and have well-documented involvement in mitochondrial function, which aligns with our central hypothesis of mitochondrial dysfunction in idiopathic infertility. Although these proteins were not the top DEPs, we prioritized them due to their strong association with mitochondrial processes, a key focus of our investigation. Additionally, the availability of reliable antibodies and positive controls allowed for robust validation via western blot. In our proteomics analysis, SOD2 and PRDX5 were underexpressed, while GSR was overexpressed, underscoring their potential significance in the pathophysiology of male infertility (Supplementary Material B).
Western blotting validation
Analysis of the western blot results (Figure 3) revealed that two proteins, SOD2 (3.8 fold; P = 0.0026), and PRDX5 (5.3 fold; P = 0.0006), were expressed in accordance with that observed in the proteomic analysis (Table 5). No significant difference was observed in the expression of GSR (P = 0.3); however, this result is inconclusive considering the low concentration observed for the normalizing protein GAPDH as well as the increased expression of this protein in one of the four patient samples (Patient 2).
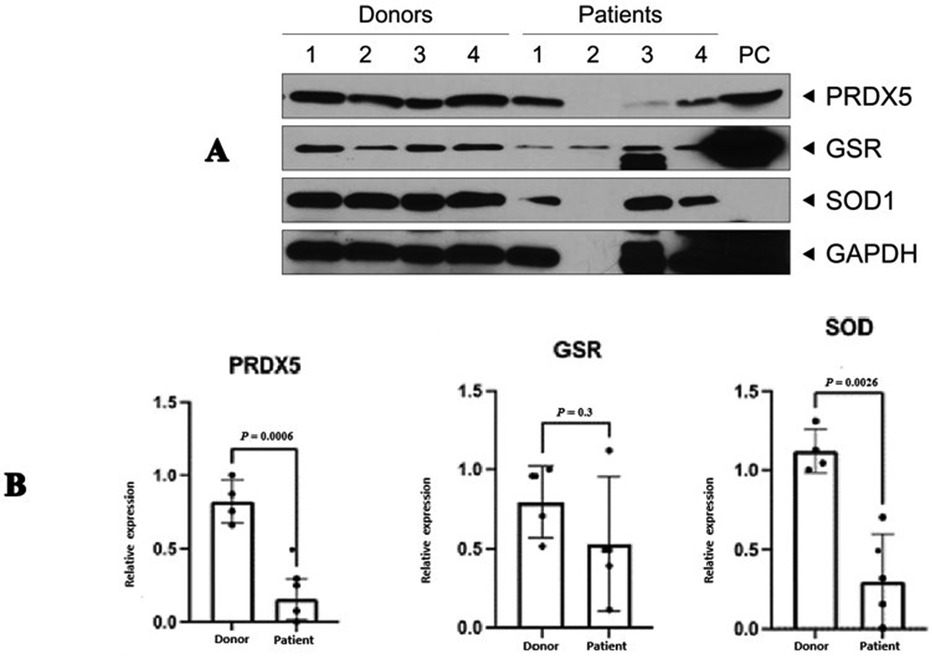
Figure 3. Western blot validation of three differentially expressed proteins in patients with idiopathic primary infertility (n = 4) and in healthy fertile donors (n = 4). (A) Representative image of WB, (B) Quantification of the WB results. Results are expressed as the mean fold change ± standard error of the mean and normalised to that of the control protein. PRDX5 and SOD were significantly decreased in patient sperm samples when compared with that of healthy donors. GAPDH. GSR, glutathione disulphide reductase; SOD, superoxide dismutase; PRDX5, peroxiredoxin-5; GAPDH, glyceraldehyde 3-phosphate dehydrogenase.
Discussion
Idiopathic male infertility is diagnosed when there is an abnormality in the semen analysis with no obvious etiology. In this study, we identified mitochondrial dysfunction as a key factor linked to idiopathic primary male infertility. Using global proteomics analysis, we found a variety of DEPs between healthy donors and infertile patients, with a notable subset related to mitochondrial function. Specifically, three mitochondrial proteins (GSR, PRDX5, and SOD2) exhibited expression patterns that have not been previously documented in the context of male infertility. PRDX5 and SOD2 were significantly underexpressed, while GSR was overexpressed in the proteomics analysis, and bioinformatics indicated a general downregulation of mitochondrial activity in the infertile group. These results offer a potential basis for new biomarkers and therapeutic targets for diagnosing and managing idiopathic male infertility. The changes in expression of PRDX5 and SOD2, in particular, highlight the importance of oxidative stress regulation in this condition.
In sperm cells, these proteins work together to control oxidative stress. SOD2 initially neutralizes superoxide radicals, resulting in the formation of hydrogen peroxide (38). PRDX5 subsequently converts this hydrogen peroxide into water, which helps prevent the buildup of ROS (39). At the same time, GSR regenerates glutathione, maintaining the cell's antioxidant capacity (40). Any disruptions in the expression or function of these enzymes can lead to an imbalance in oxidative stress, raising ROS levels and damaging sperm DNA, lipids, and proteins, all of which can negatively impact sperm quality and fertility (41).
PRDX5 is a mitochondrial protein encoded by PRDX5 in humans; it belongs to the six-member peroxiredoxin family of antioxidant enzymes. PRDX5 functions as a critical cytoprotective antioxidant enzyme that effectively mitigates the build-up of both endogenous and exogenous peroxides, including H2O2 (42). PRDX5 plays a crucial role in antioxidative and cytoprotective functions during oxidative stress. Reduced PRDX5 expression has been linked to an increased susceptibility to oxidative damage and peroxide-induced apoptosis (43) Previous proteomic studies have revealed decreased levels of PRDX5 in the spermatozoa of male patients with infertility (44). Abnormal PRDX5 activity affects various sperm-related factors, including motility, capacitation, mitochondrial membrane potential, and DNA integrity (45). These findings highlight the intricate roles of PRDX5 and its fellow peroxiredoxins in sperm function and overall male reproductive health. Our findings demonstrate for the first time, an association between PRDX5 expression and idiopathic primary male infertility.
SOD2 is encoded by SOD2 in humans; it belongs to the superoxide dismutase iron/manganese family. SOD2 catalyzes the conversion of toxic superoxide, a by-product of the mitochondrial electron transport chain, into H2O2 and O2 (46). As a result, SOD2 effectively removes mitochondrial ROS, thereby protecting mitochondria and cells against oxidative stress. A clear connection exists between SOD gene polymorphisms and male infertility, with impacts on in vitro fertilization (IVF) outcomes (47). Our study further underscores the significance of SOD in the context of idiopathic primary male infertility using proteomic analysis. Similar to our findings regarding PRDX5, this finding emphasizes the potential role of oxidative stress as a causative factor in idiopathic primary male infertility owing to low protein levels of PRDX5 and SOD2.
GSR is encoded by GSR in humans; it catalyzes the reduction of glutathione disulphide to glutathione, a crucial molecule for combating oxidative stress and maintaining a cellular reducing environment (48). GSR has previously been reported as a biomarker of male infertility, particularly in cases associated with oxidative stress and varicoceles (49). According to our proteomics analysis, GSR was overexpressed (NSAF ratio = 4.43, P = 0.00070). However, IPA revealed a negative change in GSR expression, and the WB analysis revealed no significant difference in the total GSR expression, although increased GSR expression was detected in one of the four patient samples. Multiple factors could explain these differences, such as data normalization, data processing errors, control group-related differences, statistical analysis methods, and biological variability (46, 50, 51). Our data check did not reveal any technical or analytical errors; thus, we assume that the aforementioned differences in GSR expression are due to biological variability. This assumption needs to be validated using larger sample sizes for both the proteomics study and the western blot-based validation.
To the best of our knowledge, this study represents the first report on an association between PRDX5, and SOD2 expression and idiopathic primary male infertility. This indicates that the mitochondria in sperm of patients with idiopathic primary male infertility likely exhibit oxidative stress owing to relatively low antioxidant enzyme activity expanding our understanding of this condition beyond sperm parameters such as concentration, motility, and morphology. This association of idiopathic male infertility and mitochondrial oxidative stress was also indicated through the study by Agarwal et al. (52) when the researchers evaluated the sperm proteome of idiopathic infertile male before and after antioxidant supplementation, revealing post-treatment activation of transcriptional factors associated with antioxidant defence system and free radical scavenging.
The major limitations of this pilot study include its small sample size used for protein validation through western blot. We were able to include only 4 samples in each group and it turns out to be insufficient to validate the proteomics and bioinformatics analysis. Furthermore, although damage to sperm resulting in infertility, including DNA damage, caused by mitochondrial stress remains a key hypothesis, the mechanistic basis for these changes remains unknown, especially because we did not exclude patients with high levels of oxidative stress or high DNA fragmentation. These findings need to be re-evaluated in patients with primary infertility with and without high oxidative stress or high DNA fragmentation. Future studies should evaluate the response of the mtDNA repair mechanisms, as it could offer deeper insights into the underlying pathology of sperm damage. This could include measuring key enzymes involved in mtDNA repair, such as those in the base excision repair pathway, and assessing their correlation with mitochondrial protein dysfunction.
Whether these findings offer additional diagnostic insights beyond DNA damage alone remains to be tested, but they do potentially support the rapid assessment of key protein content using ELISA rather than complex DNA fragmentation testing. This study offers mitochondrial pathology as the underlying etiology of idiopathic male infertility and suggests an association with the downregulated expression of mitochondrial PRDX5 and SOD2. These findings encourage further mechanistic research and development of improved diagnostic techniques based on mitochondrial proteins for identifying mitochondrial pathology causing male infertility.
Data availability statement
The original contributions presented in the study are included in the article/Supplementary Material, further inquiries can be directed to the corresponding author.
Ethics statement
The studies involving humans were approved by Cleveland Clinic Institutional Review Board (IRB#17-422). The studies were conducted in accordance with the local legislation and institutional requirements. The participants provided their written informed consent to participate in this study.
Author contributions
RSK: Conceptualization, Data curation, Formal Analysis, Funding acquisition, Investigation, Methodology, Project administration, Resources, Software, Supervision, Validation, Visualization, Writing – original draft, Writing – review & editing. SDM: Validation, Writing – review & editing. MKPS: Data curation, Methodology, Writing – review & editing. AWM: Supervision, Writing – review & editing. SCJ: Supervision, Writing – review & editing. SDL: Methodology, Supervision, Visualization, Writing – review & editing.
Funding
The author(s) declare financial support was received for the research, authorship, and/or publication of this article. This work was partially supported by AAUW International Fellowship Award [award numbers 012370].
Acknowledgements
The authors are thankful to Dr. Keshab Kumar Karni, Dr. Renata Finelli, Dr. Shinnosuke Kuroda for their help and support throughout the study.
Conflict of interest
The authors declare that the research was conducted in the absence of any commercial or financial relationships that could be construed as a potential conflict of interest.
Publisher's note
All claims expressed in this article are solely those of the authors and do not necessarily represent those of their affiliated organizations, or those of the publisher, the editors and the reviewers. Any product that may be evaluated in this article, or claim that may be made by its manufacturer, is not guaranteed or endorsed by the publisher.
Supplementary material
The Supplementary Material for this article can be found online at: https://www.frontiersin.org/articles/10.3389/frph.2024.1479568/full#supplementary-material
Abbreviations
DEP, differentially expressed protein; GAPDH, glyceraldehyde 3-phosphate dehydrogenase; GSR, glutathione disulphide reductase; ICSI, intracytoplasmic sperm injection; IPA, ingenuity pathway analysis; PRDX5, peroxiredoxin-5; SOD2, superoxide dismutase 2; WHO, World Health Organization; SDS-PAGE, sodium dodecyl sulphate-polyacrylamide gel electrophoresis page; LC-MS, liquid chromatography-mass spectrometry; IVF, in vitro fertilization; ROS, reactive oxygen species; RLU/sec, relative light units per second.
References
1. WHO. World Health Organization (WHO)—Infertility Prevalence Estimates 1990–2021. Geneva: World Health Organization (2023). p. 1–98. Available online at: https://www.who.int/publications/i/item/978920068315 (accessed June 02, 2024)
2. Agarwal A, Baskaran S, Parekh N, Cho CL, Henkel R, Vij S, et al. Male infertility. Lancet. (2021) 397(10271):319–33. doi: 10.1016/S0140-6736(20)32667-2
3. Punab M, Poolamets O, Paju P, Vihljajev V, Pomm K, Ladva R, et al. Causes of male infertility: a 9-year prospective monocentre study on 1,737 patients with reduced total sperm counts. Hum Reprod. (2017) 32(1):18. doi: 10.1093/HUMREP/DEW284
4. Duca Y, Calogero AE, Cannarella R, Condorelli RA, La Vignera S. Current and emerging medical therapeutic agents for idiopathic male infertility. Expert Opin Pharmacother. (2019) 20(1):55–67. doi: 10.1080/14656566.2018.1543405
5. Cannarella R, Condorelli RA, Duca Y, La Vignera S, Calogero AE. New insights into the genetics of spermatogenic failure: a review of the literature. Hum Genet. (2019) 138(2):125–40. doi: 10.1007/S00439-019-01974-1
6. García-Herrero S, Meseguer M, Martínez-Conejero JA, Remohí J, Pellicer A, Garrido N. The transcriptome of spermatozoa used in homologous intrauterine insemination varies considerably between samples that achieve pregnancy and those that do not. Fertil Steril. (2010) 94(4):1360–73. doi: 10.1016/J.FERTNSTERT.2009.07.1671
7. Castillo J, Jodar M, Oliva R. The contribution of human sperm proteins to the development and epigenome of the preimplantation embryo. Hum Reprod Update. (2018) 24(5):535–55. doi: 10.1093/HUMUPD/DMY017
8. Barroso G, Valdespin C, Vega E, Kershenovich R, Avila R, Avendaño C, et al. Developmental sperm contributions: fertilization and beyond. Fertil Steril. (2009) 92(3):835–48. doi: 10.1016/J.FERTNSTERT.2009.06.030
9. Rahman MS, Lee JS, Kwon WS, Pang MG. Sperm proteomics: road to male fertility and contraception. Int J Endocrinol. (2013) 2013:360986. doi: 10.1155/2013/360986
10. Baker MA, Reeves G, Hetherington L, Müller J, Baur I, Aitken RJ. Identification of gene products present in triton X-100 soluble and insoluble fractions of human spermatozoa lysates using LC-MS/MS analysis. Proteomics Clin Appl. (2007) 1(5):524–32. doi: 10.1002/PRCA.200601013
11. Wang G, Guo Y, Zhou T, Shi X, Yu J, Yang Y, et al. In-depth proteomic analysis of the human sperm reveals complex protein compositions. J Proteomics. (2013) 79:114–22. doi: 10.1016/J.JPROT.2012.12.008
12. Greither T, Dejung M, Behre HM, Butter F, Herlyn H. The human sperm proteome—toward a panel for male fertility testing. Andrology. (2023) 11(7):1418–36. doi: 10.1111/andr.13431
13. Martínez-Heredia J, Estanyol JM, Ballescà JL, Oliva R. Proteomic identification of human sperm proteins. Proteomics. (2006) 6(15):4356–69. doi: 10.1002/PMIC.200600094
14. Selvam MKP, Agarwal A, Pushparaj PN, Baskaran S, Bendou H. Sperm proteome analysis and identification of fertility-associated biomarkers in unexplained male infertility. Genes (Basel). (2019) 10(7):522. doi: 10.3390/GENES10070522
15. Boguenet M, Bouet P-E, Spiers A, Reynier P, May-Panloup P. Mitochondria: their role in spermatozoa and in male infertility. Hum Reprod Update. (2021) 27(4):697–719. doi: 10.1093/humupd/dmab001
16. Barbagallo F, La Vignera S, Cannarella R, Aversa A, Calogero AE, Condorelli RA. Evaluation of sperm mitochondrial function: a key organelle for sperm motility. J Clin Med. (2020) 9(2):363. doi: 10.3390/JCM9020363
17. Wright C, Milne S, Leeson H. Sperm DNA damage caused by oxidative stress: modifiable clinical, lifestyle and nutritional factors in male infertility. Reprod Biomed Online. (2014) 28(6):684–703. doi: 10.1016/j.rbmo.2014.02.004
18. Ayaz A, Agarwal A, Sharma R, Kothandaraman N, Cakar Z, Sikka S. Proteomic analysis of sperm proteins in infertile men with high levels of reactive oxygen species. Andrologia. (2018) 50(6):e13015. doi: 10.1111/and.13015
19. Aitken RJ, Baker MA. Oxidative stress, spermatozoa and leukocytic infiltration: relationships forged by the opposing forces of microbial invasion and the search for perfection. J Reprod Immunol. (2013) 100(1):11–9. doi: 10.1016/j.jri.2013.06.005
20. Amaral A, Lourenço B, Marques M, Ramalho-Santos J. Mitochondria functionality and sperm quality. Reproduction. (2013) 146(5):163–74. doi: 10.1530/REP-13-0178
21. Samanta L, Agarwal A, Swain N, Sharma R, Gopalan B, Esteves SC, et al. Proteomic signatures of sperm mitochondria in varicocele: clinical use as biomarkers of varicocele associated infertility. J Urol. (2018) 200(2):414–22. doi: 10.1016/j.juro.2018.03.009
22. An T, Wang YF, Liu JX, Pan YY, Liu YF, He ZC, et al. Comparative analysis of proteomes between diabetic and normal human sperm: insights into the effects of diabetes on male reproduction based on the regulation of mitochondria-related proteins. Mol Reprod Dev. (2018) 85(1):7–16. doi: 10.1002/mrd.22930
23. Huang R, Chen J, Guo B, Jiang C, Sun W. Diabetes-induced male infertility: potential mechanisms and treatment options. Mol Med. (2024) 30(1):11. doi: 10.1186/s10020-023-00771x
24. Condorelli RA, La Vignera S, Mongioì LM, Alamo A, Calogero AE. Diabetes Mellitus and infertility: different pathophysiological effects in type 1 and type 2 on sperm function. Front Endocrinol (Lausanne). (2018) 9:268. doi: 10.3389/fendo.2018.00268
25. Torra-Massana M, Jodar M, Barragán M, Soler-Ventura A, Delgado-Dueñas D, Rodríguez A, et al. Altered mitochondrial function in spermatozoa from patients with repetitive fertilization failure after ICSI revealed by proteomics. Andrology. (2021) 9(4):1192–204. doi: 10.1111/andr.12991
26. Antoniassi MP, Intasqui P, Camargo M, Zylbersztejn DS, Carvalho VM, Cardozo KH, et al. Analysis of the functional aspects and seminal plasma proteomic profile of sperm from smokers. BJU Int. (2016) 118(5):814–22. doi: 10.1111/BJU.13539
27. Durairajanayagam D, Singh D, Agarwal A, Henkel R. Causes and consequences of sperm mitochondrial dysfunction. Andrologia. (2021) 53(1):e13666. doi: 10.1111/AND.13666
28. Irigoyen P, Pintos-Polasky P, Rosa-Villagran L, Skowronek MF, Cassina A, Sapiro R. Mitochondrial metabolism determines the functional status of human sperm and correlates with semen parameters. Front Cell Dev Biol. (2022) 10:926684. doi: 10.3389/FCELL.2022.926684
29. Mirshahvaladi S, Topraggaleh TR, Bucak MN, Rahimizadeh P, Shahverdi A. Quantitative proteomics of sperm tail in asthenozoospermic patients: exploring the molecular pathways affecting sperm motility. Cell Tissue Res. (2023) 392(3):793–810. doi: 10.1007/S00441-023-03744-Y
30. Moscatelli N, Lunetti P, Braccia C, Armirotti A, Pisanello F, De Vittorio M, et al. Comparative proteomic analysis of proteins involved in bioenergetics pathways associated with human sperm motility. Int J Mol Sci. (2019) 20(12):3000. doi: 10.3390/IJMS20123000
31. Pacheco RI, Cristo MI, Anjo SI, Silva AF, Sousa MI, Tavares RS, et al. New insights on sperm function in male infertility of unknown origin: a multimodal approach. Biomolecules. (2023) 13(10):1462. doi: 10.3390/BIOM13101462
32. Mischak H, Allmaier G, Apweiler R, Attwood T, Baumann M, Benigni A, et al. Recommendations for biomarker identification and qualification in clinical proteomics. Sci Transl Med. (2010);2(46):46ps42. doi: 10.1126/scitranslmed.3001249
33. WHO laboratory manual for the examination and processing of human semen. Available online at: https://iris.who.int/handle/10665/44261 (accessed June 02, 2024)
34. Martínez-Bartolomé S, Deutsch EW, Binz PA, Jones AR, Eisenacher M, Mayer G, et al. Guidelines for reporting quantitative mass spectrometry based experiments in proteomics. J Proteomics. (2013) 95:84–8. doi: 10.1016/J.JPROT.2013.02.026
35. Skrzypek MS, Binkley J, Sherlock G. How to use the Candida genome database. Methods Mol Biol. (2022) 2542:55–69. doi: 10.1007/978-1-0716-2549-1_4
36. Szklarczyk D, Kirsch R, Koutrouli M, Nastou K, Mehryary F, Hachilif R, et al. The STRING database in 2023: protein-protein association networks and functional enrichment analyses for any sequenced genome of interest. Nucleic Acids Res. (2023) 51(D1):D638–46. doi: 10.1093/nar/gkac1000
37. Sherman BT, Hao M, Qiu J, Jiao X, Baseler MW, Lane HC, et al. DAVID: a web server for functional enrichment analysis and functional annotation of gene lists (2021 update). Nucleic Acids Res. (2022) 50(W1):W216–21. doi: 10.1093/nar/gkac194
38. O'Flaherty C, Scarlata E. Oxidative stress and reproductive function: the protection of mammalian spermatozoa against oxidative stress. Reproduction. (2022) 164(6):F67–78. doi: 10.1530/REP-22-0200
39. O'Flaherty C, de Souza AR. Hydrogen peroxide modifies human sperm peroxiredoxins in a dose-dependent manner. Biol Reprod. (2011) 84(2):238–47. doi: 10.1095/biolreprod.110.085712
40. Sharif FA, Ashour MJ, Abuwarda HN, Ismail S, Salem N, Suleiman R, et al. Antioxidant genes variants and their association with sperm DNA fragmentation. Biochem Genet. (2024). doi: 10.1007/s10528-023-10559-7
41. Hussain T, Kandeel M, Metwally E, Murtaza G, Kalhoro DH, Yin Y, et al. Unravelling the harmful effect of oxidative stress on male fertility: a mechanistic insight. Front Endocrinol (Lausanne). (2023) 14:1070692. doi: 10.3389/fendo.2023.1070692
42. Knoops B, Goemaere J, Van Der Eecken V, Declercq JP. Peroxiredoxin 5: structure, mechanism, and function of the mammalian atypical 2-cys peroxiredoxin. Antioxid Redox Signal. (2011) 15:817–29. doi: 10.1089/ARS.2010.3584
43. Yuan J, Murrell GAC, Trickett A, Landtmeters M, Knoops B, Wang MX. Overexpression of antioxidant enzyme peroxiredoxin 5 protects human tendon cells against apoptosis and loss of cellular function during oxidative stress. Biochim Biophys Acta. (2004) 1693(1):37–45. doi: 10.1016/J.BBAMCR.2004.04.006
44. O’Flaherty C. Peroxiredoxins: hidden players in the antioxidant defence of human spermatozoa. Basic Clin Androl. (2014) 24(1):4. doi: 10.1186/2051-4190-24-4
45. O’Flaherty C. Redox regulation of mammalian sperm capacitation. Asian J Androl. (2015) 17(4):583–90. doi: 10.4103/1008-682X.153303
46. Holley AK, Bakthavatchalu V, Velez-Roman JM, St. Clair DK. Manganese superoxide dismutase: guardian of the powerhouse. Int J Mol Sci. (2011) 12(10):7114. doi: 10.3390/IJMS12107114
47. Fallah F, Colagar AH, Saleh HA, Ranjbar M. Variation of the genes encoding antioxidant enzymes SOD2 (rs4880), GPX1 (rs1050450), and CAT (rs1001179) and susceptibility to male infertility: a genetic association study and in silico analysis. Environ Sci Pollut Res Int. (2023) 30(36):86412–24. doi: 10.1007/s11356-023-28474-0
48. Vyas B, Bhowmik R, Akhter M, Ahmad FJ. Identification, analysis of deleterious SNPs of the human GSR gene and their effects on the structure and functions of associated proteins and other diseases. Sci Rep. (2022) 12(1):5474. doi: 10.1038/S41598-022-09295-6
49. Mannervik B. The enzymes of glutathione metabolism: an overview. Biochem Soc Trans. (1987) 15(4):717–8. doi: 10.1042/BST0150717
50. Dubois E, Galindo AN, Dayon L, Cominetti O. Assessing normalization methods in mass spectrometry-based proteome profiling of clinical samples. Biosystems. (2022) 215–216:104661. doi: 10.1016/J.BIOSYSTEMS.2022.104661
51. Schork K, Podwojski K, Turewicz M, Stephan C, Eisenacher M. Important issues in planning a proteomics experiment: statistical considerations of quantitative proteomic data. Methods Mol Biol. (2021) 2228:1–20. doi: 10.1007/978-1-0716-1024-4_1/FIGURES/3
Keywords: sperm proteome, spermatozoa protein, idiopathic male infertility, mitochondrion, reactive oxygen species, bioinformatics
Citation: Sawaid Kaiyal R, Mukherjee SD, Panner Selvam MK, Miller AW, Vij SC and Lundy SD (2024) Mitochondrial dysfunction signatures in idiopathic primary male infertility: a validated proteomics-based diagnostic approach. Front. Reprod. Health 6:1479568. doi: 10.3389/frph.2024.1479568
Received: 12 August 2024; Accepted: 18 November 2024;
Published: 12 December 2024.
Edited by:
Roland Eghoghosoa Akhigbe, Ladoke Akintola University of Technology, NigeriaReviewed by:
Yimin Shu, University of Kansas Medical Center, United StatesPrecious Oyedokun, Ladoke Akintola University of Technology, Nigeria
Copyright: © 2024 Sawaid Kaiyal, Mukherjee, Panner Selvam, Miller, Vij and Lundy. This is an open-access article distributed under the terms of the Creative Commons Attribution License (CC BY). The use, distribution or reproduction in other forums is permitted, provided the original author(s) and the copyright owner(s) are credited and that the original publication in this journal is cited, in accordance with accepted academic practice. No use, distribution or reproduction is permitted which does not comply with these terms.
*Correspondence: Raneen Sawaid Kaiyal, cmFuZWVuLnNhd2FpZEBnbWFpbC5jb20=
†ORCID:
Raneen Sawaid Kaiyal
orcid.org/0000-0002-3111-3978
Manesh Kumar Panner Selvam
orcid.org/0000-0002-9120-2278