- Department of Biological Sciences, Simon Fraser University, Burnaby, BC, Canada
Polycystic ovary syndrome (PCOS) exhibits high prevalence and heritability despite causing negative impacts on fertility and fecundity. Previous hypotheses have postulated that some PCOS-associated traits, especially above-average levels of testosterone, were associated with benefits in ancestral environments. As such, PCOS would represent, in part, a maladaptive extreme of adaptations related to relatively high testosterone. To evaluate this hypothesis, we conducted a series of systematic literature reviews on the associations of testosterone levels, and prenatal testosterone metrics, with measures of strength, robustness, muscularity, and athleticism in females. We also systematically reviewed the literature on associations of testosterone with dominance in females and reviewed archaeological evidence concerning female strength and muscularity and its correlates. The main findings were fivefold: (1) elevated testosterone levels were generally associated with higher strength, muscularity and athleticism in females; (2) females with PCOS showed notable evidence of increased strength, muscularity, and athleticism compared to controls; (3) females with higher testosterone levels exhibited clear evidence of high dominance, (4) despite evidence that higher testosterone is linked with higher bone mineral density in healthy females, PCOS was not clearly associated with this phenotype; and (5) archaeological evidence from osteology, and data from some current small-scale societies, indicated that females often exhibit substantial levels of muscularity. Overall, the hypothesis that relatively high levels of testosterone are associated with benefits to females in some contexts was largely supported. These results provide evidence for the “maladaptive extremes of adaptation” model, with implications for treatment of females with PCOS and for future research.
Introduction
Some female-limited diseases with negative impacts on fertility and fecundity are present at high frequencies within contemporary populations. For example, polycystic ovary syndrome (PCOS) and endometriosis each demonstrate a prevalence of about 5%–15% (1–3) and a heritability of 50%–70% (4–6), while notably reducing lifetime fitness (7, 8). How and why have risks for such diseases evolved, and how are they maintained?
Hypotheses for the maintenance of common, deleterious diseases can be addressed by several evolutionary factors including mismatches, trade-offs, and extremes of adaptations (9–11). In each case, maladaptive aspects of the disease reflect adaptations, and their constituent trade-offs, that have become dysregulated in particular ways. For example, risks and symptoms of endometriosis show evidence of involving evolutionary trade-offs and extremes of adaptations related to female life histories and sexual selection (10–13). Low testosterone levels, earlier menarche, and earlier menopause are all correlates of endometriosis that are consistent with a fast life history, with increased investment in earlier reproduction (13). For any given reproductive disorder, the challenge is to determine how the risks and symptoms are related to adaptations and trade-offs, in the contexts of current and ancestral environments.
In this paper, we evaluate the primary evolutionary hypothesis described thus far for the maintenance of PCOS, one of the most common disorders of female reproduction. We describe the symptoms and diagnostic criteria for PCOS, and then briefly explain the main hypothesis described thus far for explaining the evolutionary basis of risk for PCOS: that non-clinical PCOS-associated traits, especially relatively high levels of testosterone and associated dominance, strength, robustness, and muscularity, are contextually advantageous especially in the context of higher survival (9, 11, 14–18). Some evidence relevant to this hypothesis has been described previously, but data salient to testing the hypothesis has yet to be evaluated comprehensively. We do so here, using a series of systematic reviews that target key predictions of the hypothesis.
Symptoms and diagnosis of PCOS
PCOS is characterized by some combination of three main criteria: (i) hyperandrogenism (high androgen production by the ovaries and the adrenal glands); (ii) anovulation or oligo-ovulation (absent or infrequent ovulation); and (iii) polycystic ovaries, which contain multiple small follicles, with curtailed development, that resemble cysts (9, 19). Hyperandrogenism in females with PCOS is also associated with the development of hirsutism, alopecia, and other health-related issues including insulin resistance, abdominal obesity, and pancreatic β-cell dysfunction [e.g., (20–24)]. High levels of insulin, found in about 65%–70% of women with PCOS, can increase the production of ovarian testosterone (23, 25). However, the presence of insulin resistance is not a diagnostic criterion for PCOS, and the relationship between this phenotype, PCOS, and high testosterone remains unclear [e.g., (26)]. The expression of high testosterone levels, low ovulation frequencies, and multifollicular ovaries grades continuously from clinical levels in females with PCOS to low levels, or absence of the traits, in healthy females (27).
High prenatal testosterone levels represent an important risk factor for PCOS, as indicated by experimental animal models (28–30) and by data linking PCOS risk with relatively long anogenital distances (AGD) and low second-to-fourth digit ratios (2D:4D) in humans (31–33). PCOS risk is also transgenerational, such that daughters of women with PCOS are about five times more likely than controls to develop the syndrome, in part as a consequence of exposure, in utero, to the high androgen levels of their mother (34). The links of prenatal testosterone levels with the symptoms and causes of PCOS indicate that a significant contributing factor to this disease is high prenatal testosterone levels, that program the developing hypothalamic-pituitary-gonadal (HPG) axis (35).
Evolutionary causes of risk for PCOS
The main hypothesis proposed to explain the evolutionary basis for the risk and symptoms of PCOS, and its high frequencies in current populations, postulates that PCOS-associated traits, especially relatively-high testosterone, may have provided survival advantages to females in ancestral environments through several main effects: (1) increased strength, muscularity and dominance; (2) higher bone mineral density, increasing robustness and reducing fracture risk, especially in the context of heavy labor; and (3) higher body mass index through relatively-enhanced visceral fat storage, and higher propensity to develop insulin resistance, which may provide advantages under reduced-food conditions and when subject to infection (9, 11, 16–18, 36). In modern environments, increased food intake and reduced physical activity would result in maladaptation due to the effects of chronic obesity in increasing insulin resistance and testosterone levels, which interfere with ovulation and thus reduce fertility (15, 37, 38). As such, the evolutionary causes of PCOS risk include maladaptive extremes of testosterone levels, due predominantly to mismatches of current with ancestral environments, and dysregulation of trade-offs between investment in survival and reproduction.
PCOS and the putative benefits of high testosterone in females
The main hypothesis addressed here for the maintenance and symptoms of PCOS is based primarily on the benefits of non-clinical PCOS-associated traits, most of which derive from relatively high levels of testosterone and its effects. By this hypothesis, females with relatively high testosterone exhibit advantages, especially in terms of strength, athleticism, dominance, and robustness, that in some social and ecological circumstances, can outweigh its evolutionary drawbacks in the context of reduced or delayed fertility. The hypothesis presented here does not view PCOS itself to be adaptive. Instead, it considers PCOS as a maladaptive extreme of processes and traits that can be adaptive in some circumstances.
In this article, we focus specifically on the hypothesis of benefits to relatively high testosterone in females that are related to strength, muscularity, robustness, and dominance. The sets of tests performed can be conceptualized into three sub-hypotheses. By the first hypothesis, healthy females with higher testosterone exhibit greater physical strength and athleticism. This hypothesis predicts that: (1) higher prenatal testosterone is associated with increased strength, muscularity, dominance, athleticism, and BMD in females, (2) elevated testosterone levels are associated with higher strength, muscularity, athleticism, BMD, and social and physical dominance in females, and (3) current testosterone levels in women with PCOS should more closely align with testosterone levels in female athletes than controls.
By the second hypothesis, females with PCOS should exhibit greater physical strength and athleticism. This hypothesis predicts that: (1) females with PCOS show evidence of increased strength, muscularity, athleticism, and bone mineral density, (2) lean females with PCOS, an important subgroup that may be especially relevant to ancestral conditions and less affected by negative metabolic conditions, also exhibit higher strength, muscularity, and athleticism compared to controls, and (3) females with PCOS show higher levels of social and physical dominance than controls.
By the third hypothesis, females from ancestral environments and modern-small scale societies are expected to show evidence of greater strength and muscularity than females in modern Westernized societies. This hypothesis predicts that: (1) female specimens seen in the archaeological record exhibit evidence of increased bone strength and muscularity, where variation in these factors overlaps with relevant traits among males, and (2) females in some ancestral environments and modern small-scale societies exhibit evidence of substantial strength, muscularity, and athleticism, in the context of heavy labour or other activities associated with their local socioecology. These hypotheses were evaluated with a series of systematic reviews.
Methods
A series of systematic literature reviews was conducted to test the predictions of each hypothesis. Details regarding the review methodology, including the search terms, databases searched, inclusion criteria and exclusion criteria, are provided in Table 1. Additional methods, such as calculations of percent differences, are provided in the footers of relevant tables. Given that the reviews are systematic, they are unbiased regarding study inclusion. Throughout the exposition, the term “female” refers to biological sex, in the context of XX chromosome complement.
Web of Science (WOS), PubMed, and Google Scholar databases were searched for each relevant table. Google Scholar, a more broadly inclusive search engine, was searched using the specific terms listed in Table 1, for any date, and with sorting by relevance. For this database, the first 200 citations were included in the initial screening for each relevant table. The reference lists of relevant articles from each search were also searched for additional relevant citations. In each table that analysed serum or salivary testosterone concentrations, these concentrations were converted to nmol/litre (nmol/L) to standardize across studies.
Results
Markers of prenatal testosterone and athleticism, strength, muscularity, dominance, and bone mineral density in healthy females
High prenatal testosterone has been strongly associated with PCOS in animal models (39, 40), and prenatal testosterone levels can be indexed by measuring AGD or, with considerably less accuracy and repeatability, 2D:4D (41–43). By the hypothesis addressed here, lower 2D:4D and higher AGD (and thus higher inferred prenatal testosterone) should be associated with higher strength, athleticism, muscularity, bone mineral density, and dominance in females.
In the data reviewed here, lower 2D:4D ratios were significantly associated with measures of higher athleticism, speed, strength, or endurance across 24 of 36 studies overall (Supplementary Table S3). However, studies regarding correlations between measures of athleticism, strength, muscularity, dominance, or BMD and AGD were not found in the literature search. There was limited data regarding associations between markers of prenatal testosterone and dominance. However, Manning and Fink (44) reported a significant negative association between right 2D:4D and dominance scores in females, where dominance scores were measured via questions from the International Personality Pool (IPIP). Digit ratios were significantly and positively associated with measures of BMD (indicating an association of BMD with lower prenatal testosterone) in two of three studies (Supplementary Table S9).
Serum or salivary testosterone and athleticism and its correlates in healthy females
By the main hypothesis addressed here, athleticism and its correlates should be associated with higher adult testosterone, among healthy females without diagnoses of PCOS. Higher testosterone levels have been significantly and positively associated with measures of athletic performance across 20 of the 34 studies in Table 2. The various measures of athletic performance in Table 2 included strength, sprint speed, stamina, lean muscle mass, training intensity and motivation, muscle fatigue, and competitiveness, in the contexts of a wide variety of sports and athletic training.
For studies that quantified serum testosterone levels, four of nine showed significantly higher testosterone levels in athletes compared to controls (Table 3). Across the studies that quantified salivary testosterone levels, all four showed significantly higher mean testosterone levels in athletes than in controls (Table 3).
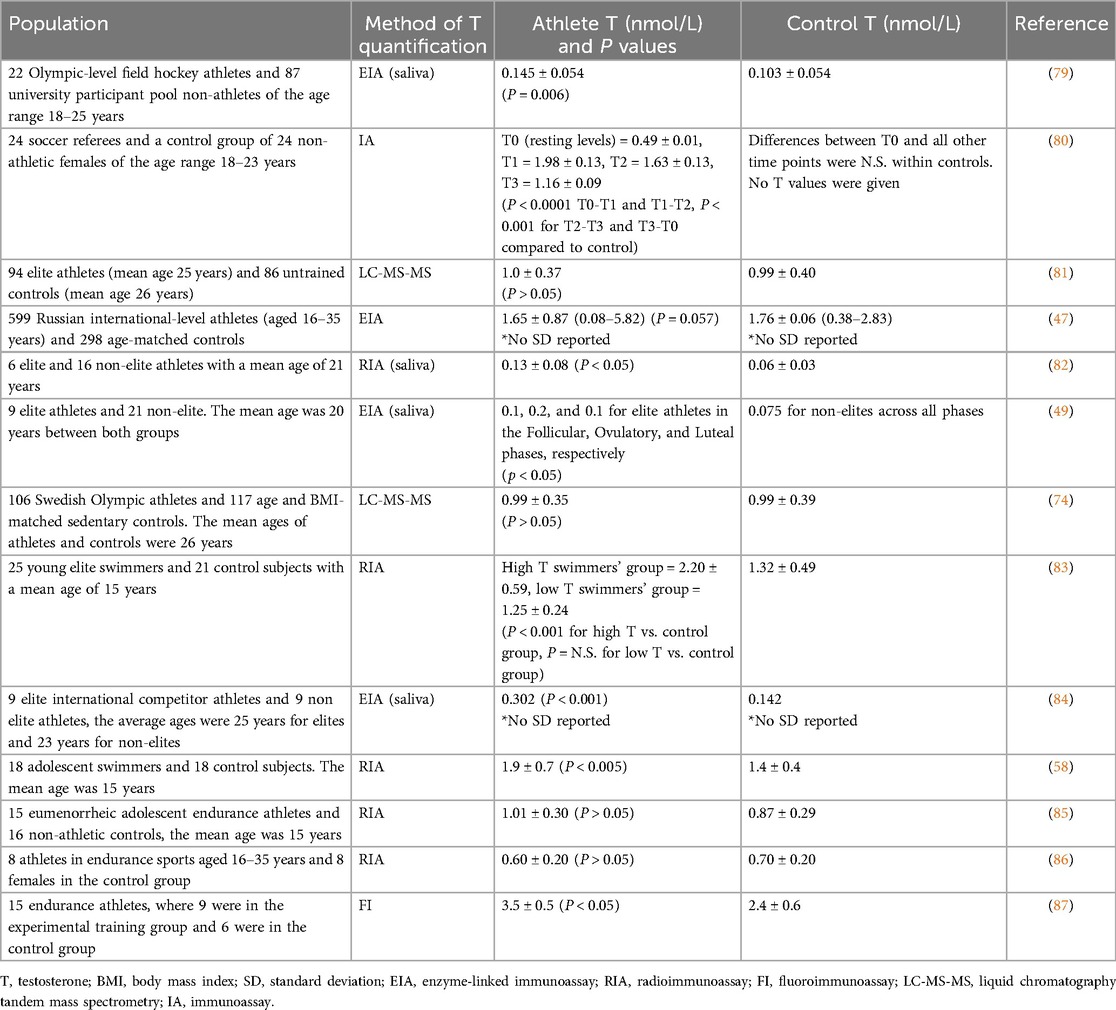
Table 3. Comparison of serum and salivary testosterone levels between healthy female athletes and female controls/non-athletes.
Across the eight studies that quantified serum testosterone levels and included control groups, mean serum testosterone levels were approximately 18% higher in the athlete group compared to controls. Across the four studies that analyzed salivary testosterone levels and included control groups, mean salivary testosterone levels were about 90% higher in the athlete group compared to healthy controls (Table 4 and Supplementary Table S2). The studies reviewed here did not compare levels of testosterone from different sources (mainly serum vs. saliva). High salivary testosterone levels compared to serum can be influenced by several factors, including binding proteins in serum (e.g., SHBG and albumin), salivary gland function, measurement differences in sensitivity or specificity, and physiological variations such as stress, circadian rhythms, or individual metabolic differences (88).
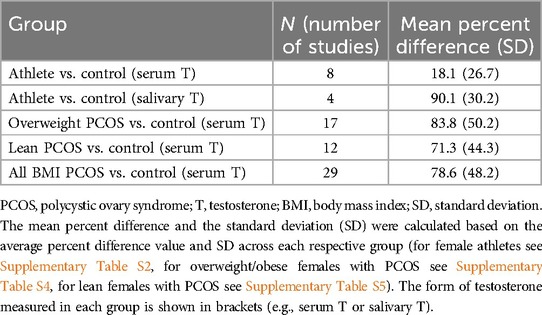
Table 4. Comparisons of serum and salivary testosterone percent difference between controls and women athletes, women with overweight PCOS, and women with lean PCOS.
Serum and salivary testosterone levels in relation to measures of BMD in pre-menopausal and post-menopausal healthy females
By the hypothesis addressed here, higher serum and salivary testosterone levels should be associated with increased BMD in healthy females. Serum testosterone was significantly positively associated with BMD in 12 of 30 studies (Supplementary Table S8). There were no significant associations between testosterone and BMD in 14 studies, and in 2 studies there were negative correlations. There was also a mix of positive and null results in 2 of 25 studies: for example, Nunes et al. (89) found that serum testosterone levels were significantly positively associated with BMD scores for the hip, but not for the lumbar spine, in postmenopausal females. This diversity of results was apparently not related to pre- vs. post-menopausal status, as similar patterns of positive and null results were found in each group (Supplementary Table S8). Salivary testosterone was significantly positively associated with BMD in the single study that analyzed this metric (90). These findings suggest that testosterone and BMD are positively correlated, but with notable heterogeneity across studies, perhaps due in part to the high diversity of the populations and skeletal regions subject to analysis.
Testosterone levels, muscle mass, physical strength, endurance, and BMD in females with PCOS
The results presented above that modest (15%–20%) increases in serum testosterone are linked with enhanced athleticism in healthy females raises the question of whether females with PCOS, who show notably larger increases in testosterone (91–93), benefit in terms of strength, muscularity, and athleticism.
By the hypothesis addressed here, females with PCOS are predicted to exhibit increased muscle mass, physical strength, and endurance compared to healthy matched controls, predominantly as a function of their increased levels of testosterone. This hypothesis was largely supported by available data from the literature (Table 5). Overall, females with PCOS exhibited increased muscle mass (seven of eleven studies), physical strength (six of eight studies), sporting performance (one of one), and endurance (one of three studies) when compared to healthy controls. All the other studies showed no significant differences between groups.
In contrast to these results for various measures of strength and muscularity, the differences in BMD between females with and without PCOS varied considerably. Across 21 studies, six showed significantly higher BMD in females with PCOS compared to controls, six showed significantly lower measures of BMD in females with PCOS, and 10 showed no significant differences between the two groups (Supplementary Table S7). These findings indicate that PCOS cannot be considered to involve higher BMD, for reasons that remain unclear but may involve insulin resistance (113, 114) or other factors.
Comparison of mean total serum testosterone levels across female athletes, females with PCOS, and controls
Are the high testosterone levels in females with PCOS comparable to the levels found in female athletes? Although direct comparison of testosterone levels between female athletes and females with PCOS cannot be conducted using data from the literature (due to methodological differences in testosterone quantification methods across studies), the percent differences in serum testosterone levels between females with PCOS or athletes, and each respective control group can usefully be compared. Using this approach, females with PCOS had an average of about 79% higher serum testosterone levels compared to controls across a sample of studies (mean = 78.6, SD = 48.2, N = 29), whereas female athletes showed a smaller difference (about 18%) in serum testosterone levels compared to their respective controls (mean = 18.1, SD = 26.7, N = 8) (Table 4).
Given these simple mean differences, are the distributions of mean total serum testosterone levels comparable, and overlapping, between female athletes, controls, and females with PCOS? Mean serum testosterone levels, calculated from the data in Supplementary Table S2, Supplementary Table S4, and Supplementary Table S5 were 1.61 nmol/L (N = 8, SD = 0.94, range = 0.6–3.5) in the athletes, 1.29 nmol/L (N = 37, SD = 0.48, range = 0.5–2.6) in the controls, and 2.23 nmol/L (N = 29, SD = 0.72, range = 1–3.7) in females with PCOS. The data showed an approximately normal distribution in the PCOS group, as well as considerable overlap between all three groups (Figure 1). Athletes and controls did not show normal distributions, likely due to the lower end of the distribution not falling below the normal range of testosterone in healthy females (range = 0.52–1.7 nmol/L (115). The athlete group exhibited an especially broad overlap with the PCOS group. This overlap was notably different from that of the overlap with the control group, especially in the region where mean serum testosterone levels exceeded 2.5 nmol/L. It is important to note that the data presented here is from Western and modern populations, and that testosterone levels are probably lower in females from small-scale societies (116).
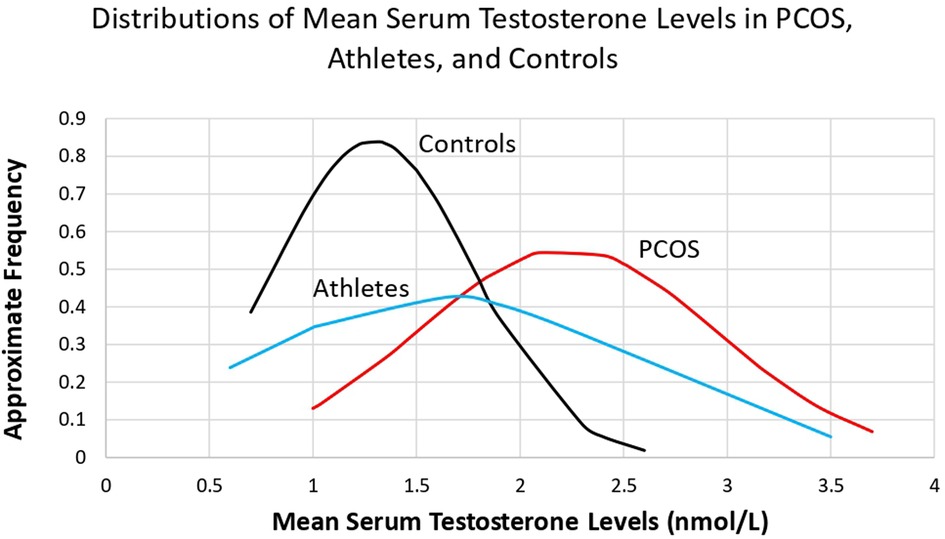
Figure 1. Comparison of the distributions of mean serum testosterone levels in women with PCOS, athletes, and controls, calculated from the data in Supplementary Table S2, Supplementary Table S4, and Supplementary Table S5.
Strength, muscularity, and athleticism in lean females with PCOS
PCOS is commonly associated with high BMI and insulin resistance (117–119), which appears to be due, in part, to the modern obesogenic environment characterized by low physical activity and high-energy food intake (9). So-called “lean” females with PCOS exhibit BMIs near or below 25 kg/m2, and as such, these females may provide a relatively more-suitable model (compared to high-BMI females) of PCOS-related traits as found in traditional or ancestral populations.
By the hypothesis addressed here, lean females with PCOS are predicted to exhibit increased strength, muscularity, and athleticism compared to healthy matched controls, due at least in part to higher testosterone. The elevations of testosterone levels in lean females with PCOS (mean = 71.3, SD = 44.3, N = 12) are similar to those of overweight females with PCOS (mean = 83.8, SD = 50.2, N = 17; Table 4). Only one study (not included in the previous section or in Table 5) has reported muscular strength in lean females with PCOS compared to controls (96); these authors found that females with PCOS exhibited increased average power in the lower limbs, and that this measure of performance was positively correlated with levels of bioavailable testosterone. Peak muscle force output was also higher in females with elevated BMI, in both controls and females with PCOS.
Despite the paucity of data on lean females with PCOS, there is considerable evidence showing a high prevalence of PCOS-related traits in female athletes, who are typically lean. Thus, PCOS was found to be the main cause of menstrual disorders among Olympic female athletes, where the incidence of polycystic ovaries in athletes who were not using hormonal contraceptives was high (37%) (120) compared with the estimated incidence of approximately 20% in the general population (121). Dadgostar et al. (122) reported that PCOS was the most common cause of menstrual irregularities among 788 Iranian female athletes, where 9% of individuals had amenorrhea or oligomenorrhea. Coste et al. (58) found that 11 out of 18 female swimmers had serum testosterone levels >0.5 ng/ml and were thus classified as hyperandrogenic, and 45% of these hyperandrogenic swimmers met the Rotterdam criteria for PCOS (two or three of the three symptoms hyperandrogenism, oligo- or anovulation, and polycystic ovaries). Moreover, Rickenlund et al. (123) found that one third of female endurance athletes with menstrual irregularities showed hyperandrogenism. These findings indicate that there is a high prevalence of PCOS, and PCOS-related traits, in female athletes.
Interpretation of these data is predicated on distinguishing between two distinct causes of reduced ovulation rates in lean female athletes (124–126). First, amenorrhea in athletes with low BMI may be caused by energy deficiency, specifically low-fat reserves, which cause hypothalamic inhibition through reduction of GnRH secretion (127, 128). Second, oligomenorrhea in female athletes has been found to be associated with hyperandrogenism, as in females with PCOS (86, 123, 129). These two groups exhibit distinct hormonal profiles, with the main distinguishing trait being 24-hr secretion of testosterone (86). The generally elevated testosterone levels of female athletes, documented above, suggests that they fall predominantly into the latter group.
Social and physical dominance among females with higher testosterone
In nonhuman female primates, social and physical dominance are consistently associated with higher testosterone levels (130–135). By the hypothesis addressed here, both females with higher testosterone levels (without PCOS), and females with PCOS, are predicted to show high social and physical dominance compared to healthy matched controls.
Across 15 studies measuring testosterone levels in relation to social and physical dominance in females, measures of dominance were significantly and positively correlated with testosterone levels in 10 studies (Table 6). Across these studies, dominance was typically measured by acts of aggression, intimidation, forcefulness, frequency of smiling, or gaze duration. Human gaze is an important indicator of dominant and submissive behaviors, where longer durations of face-gazing is indicative of higher dominance (49, 138, 143). Significant negative relationships have also been reported between right 2D:4D digit ratio and dominance scores (44), as well as between 2D:4D and dominance ratings (150), which suggests that higher prenatal testosterone is associated with dominance. There are apparently no studies in which measures of social or physical dominance in females with PCOS are compared to controls.
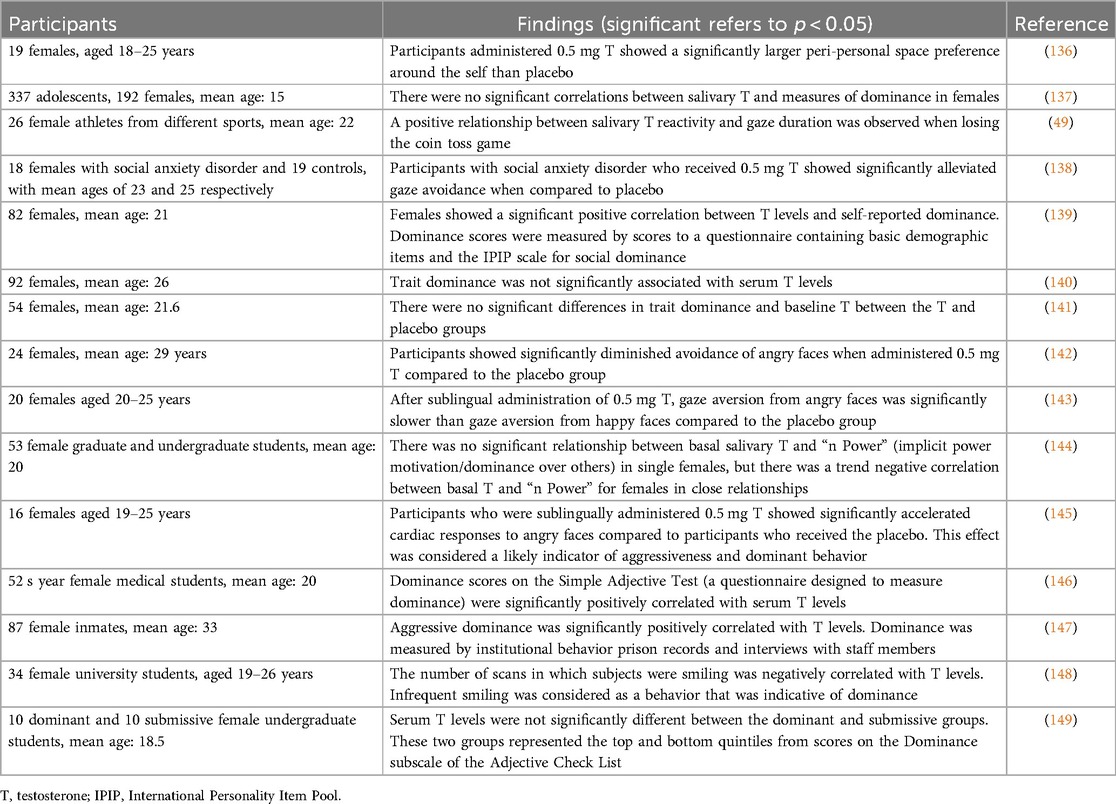
Table 6. Serum or salivary testosterone levels in relation to measures of behavioral dominance in females.
Females in ancestral environments showed evidence of substantial strength and muscularity
A systematic review of archaeological evidence related to muscularity in females demonstrated five main lines of evidence suggesting that females in some ancestral populations exhibited high strength and muscularity, in the contexts of their local ecologies (Supplementary Table S1).
First, cross sectional geometry (CSG) analysis of humerus bones showed that Muisca females from the Tibanica archaeological site (1,000–1,400 AD) in Colombia had highly robust and strong upper arms, and for six of eight CSG measures they exhibited significantly larger CSG values than their male peers (151). Macintosh et al. (152) also reported that Neolithic, Bronze Age, and Iron Age females had notably strong upper limbs with CSG properties closely matching the values observed in modern semi-elite female rowers. Robust upper bodies and strengthened humeri were also documented in Pottery Mound (700–500 YBP) females from New Mexico, USA (153). Kralick and Zemel (154) reported that young female skeletons from the agricultural period (1,150–1,550 AD) from the Georgia coast had significantly lower CSG values for nearly every measurement when compared to pre-agricultural (2,200 BC–AD 1,150) young adults from the same site. By contrast, Marchi et al. (155) reported that the CSG properties of Later Upper Paleolithic and Neolithic (6,000–5,500 BP) indicated patterns of decreased robusticity in females.
Second, BMD analyses conducted in Norway showed that young medieval females (1,050–1,536 CE) had the highest mean BMD of all time periods that were studied (750 CE–present), including modern females (156). Similarly, Holck (157) reported that females from the medieval period had significantly higher BMDs when compared to modern females, but there were no significant differences in BMD when comparing Prehistoric (5,000 BC–800 AD) or Viking Age (800–1,050 AD) females to modern females. Moreover, Spinek et al. (158) found that Neolithic females showed significantly higher BMD values compared to early medieval, medieval, and modern females.
Third, most (about 60%–70%) of studies that quantified musculoskeletal stress marker (MSM) scores (that indicate muscularity) have reported that males exhibited higher MSM scores than females, on average. However, several studies showed opposite sex-related patterns, where females exhibited higher MSM scores than males. For example, MSM scores from skeletons from both Natufian and Neolithic sites in the Levant (8,000–6,000 BC) were higher in females than in males, particularly in muscles attached to the ulna or radius (159). Similarly, using a sample of 185 skeletons from Pecos Pueblo (1,200–1,838 AD) in New Mexico, Chapman (160) reported that females had higher MSM scores in the pectoralis minor, a muscle likely utilized in grinding maize. Within a sample of 136 Early Thule Eskimo skeletons from northwest Hudson Bay (radiocarbon dated to 1,205 AD), Hawkey and Merbs (161) found that females had higher MSM scores for the trapezius and pectoralis minor, muscles likely utilized in rowing umiaks. Moreover, Hershkovitz and Gopher (162) reported that MSM scores in Neolithic females were significantly higher than in the preceding Natufian populations, which suggests that Neolithic females took over a greater proportion of physical activities compared to Natufian females.
Fourth, anthropological evidence suggests that females in some ancestral environments engaged in strenuous physical activities and heavy agricultural labor. For example, Miller et al. (151) proposed that stronger humeri in skeletal samples of Muisca females from the Tibanica site in Colombia (1,000–1,400 AD) was due to regular involvement in vigorous physical activities, such as grinding maize. Similarly, Cassidy (163) reported that in archaic-period (3,000–1,000 BC) Indian Knoll populations, females were exposed to an increased workload with the adoption of agriculture. Moreover, Bridges (164) reported that maize agriculture was more physically demanding than hunting and gathering and affected females more than males. Finally, Weber and Bettinger (165) reported that Late Neolithic to Early Bronze Age (5,800–4,000 BP) males and females showed non-significant differences in femur robusticity and a relatively equitable distribution of labor. It is important to note that the anthropological studies selected in this review describing physical activities and labor do not necessarily discuss “strength”, but more so describe “use”. As such, studies that measure BMD, CSG, or MSM provide clearer evidence regarding strength and muscularity in females from ancestral environments.
Fifth, a recent study provided evidence that the majority (about 80%) of females, from 63 different foraging societies across the globe, have been documented to participate in hunting over the last 100 years (166). The 63 societies described included 19 from North America, six from South America, 12 from Africa, 15 from Australia, five from Asia and six from the Oceanic region. Anderson et al. (166) also reported that over 70% of hunting done by females was described as intentional, indicating that females are skilled in the practice and exhibited distinct hunting strategies and training regimes compared to their male counterparts [e.g., (167–170)]. This study provides evidence that females in foraging societies across the world participate in hunting during recent time periods, thus providing another line of evidence for the proposed benefits of increased testosterone within these societies, at least to the degree that increased strength and athleticism enhance hunting abilities.
Taken together, this evidence suggests that high levels of strength and muscularity were not uncommon among females in some ancestral environments, thus representing socioecological contexts for benefits to relatively high testosterone.
Discussion
In this study we have reviewed diverse lines of evidence to evaluate the hypothesis that the risks, symptoms, and correlates of PCOS in current populations represent, in part, extreme and maladaptive manifestations of adaptive traits, especially phenotypes associated with relatively high testosterone levels, that appear to confer benefits to females in terms of muscularity, athleticism, dominance, and robustness. Overall, this hypothesis, as set forth in various ways by Azziz et al. (16), Casarini et al. (171), Ünlütürk et al. (18), Fessler et al. (17), Charifson and Trumble (9), Dumesic et al. (36), and Parker et al. (11), was supported by multiple convergent lines of evidence. This evidence includes: (1) the links of markers of prenatal testosterone with both PCOS and athleticism, (2) the positive associations of serum testosterone with strength in healthy females, (3) the higher strength and muscularity found in females with PCOS, (4) the links of testosterone with higher dominance in females of humans and other mammals, and (5) the data showing archeological evidence of notable strength and muscularity in females from a diversity of small-scale populations. This work thus supports the hypothesis that higher testosterone and muscularity may have provided benefits to females inhabiting ancestral environments, as well as mediating risk of PCOS in extant populations, in conjunction with the novel environments of higher availability of food and reduced levels of exercise, and other environmental contributors, including endocrine disrupting chemicals, circadian disruption, stress, and lack of social support (9) (Figure 2B).
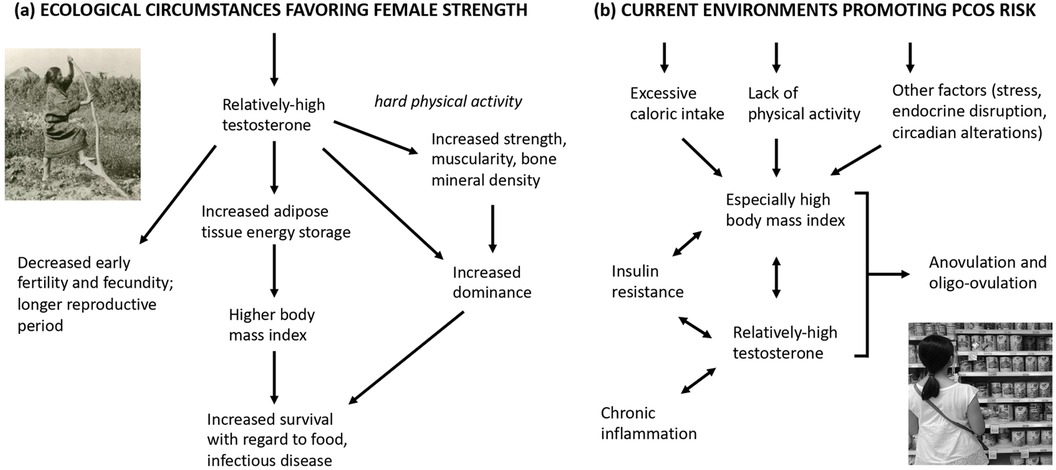
Figure 2. Hypothesized ecological circumstances favoring (a) high testosterone in females, compared to (b) current environments promoting PCOS risk. The single-headed arrow represents hypothesized effects, while the double-headed arrow represents positive associations between traits.
The benefits of higher testosterone in females, which include enhanced physical strength and athletic abilities due to anabolic effects on muscle and bone growth, and intensified competitive behavior (74, 78, 172), show evidence of applying to both lean and high-BMI females with PCOS. This finding is important because most females in ancestral populations would not have exhibited high BMI, at least not in most circumstances (173). As such, lean females with PCOS or PCOS-related traits may be relatively similar, metabolically and physiologically, to females in ancestral environments. Overall, females with PCOS (and lean females with PCOS) exhibit testosterone levels nearly twice as high, on average, compared to healthy matched controls, but with substantial overlap between the distributions, as shown in Figure 1. As such, many healthy females, and most female athletes, exhibit serum testosterone values in the range of females with PCOS; these females may exhibit subclinical PCOS traits (such as reduced ovulation rates, as described below), but also gain benefits from the advantages described above. This hypothesis could be evaluated more directly by analyzing levels of serum testosterone and muscular strength, in relation to ovulation rates and fecundability, among healthy females without PCOS.
An alternative hypothesis for the positive associations of testosterone with measures of strength and athleticism would be that exercise and strength training themselves lead to increased testosterone. Several lines of evidence contradict this hypothesis. First, exercise and strength training in females does lead to relative increases in testosterone, but the increases are transient and apparently do not lead to effects longer than several hours (174–176). Second, several studies document the strong heritability of testosterone in females, with estimates ranging from 26 to 70 percent (177–179). These findings support the idea that some females have higher testosterone levels due to genetically based factors, and not solely because of environmental factors (e.g., exercise, training, etc.). Finally, the hypothesis cannot explain the observed correlations of prenatal testosterone with strength and athleticism described above. Despite the limitations of 2D4D, this marker still provides evidence for a causal direction of the effects of prenatal testosterone on strength and athleticism in females. Overall, there is thus no clear evidence that exercise and strength training themselves lead to increases in testosterone in females.
Evidence regarding the effects of prenatal and postnatal testosterone, as well as PCOS, on BMD in females displayed notable heterogeneity across studies. A substantial proportion (about half) of the studies reported positive correlations between testosterone and BMD in healthy females; however, there were also two studies that reported negative correlations. Study results varied even more among females with PCOS compared to controls, with comparable numbers of positive, negative, and null results, such that PCOS itself cannot be considered to involve higher BMD. The differences in patterns of results between healthy females, and females with PCOS, may be attributable to factors unique to PCOS, such as insulin resistance or effects of obesity [e.g., (180)].
Systematic review also provided evidence that testosterone levels are higher in females that show higher levels of social and physical dominance, which is consistent with evidence from non-human primates and other social mammals (130, 132–135). However, dominance has yet to be studied in females with PCOS, and the links of social or physical dominance with components of fitness in human populations remain largely unexplored.
In addition to conferring potential fitness-related benefits, high testosterone in females, and in women with PCOS, also involves clear costs to reproduction. Most importantly, PCOS is a major cause of reduced fertility, as approximately 70% of females with PCOS exhibit anovulation or oligo-ovulation (181, 182). Similarly, females who have not been diagnosed with PCOS, but exhibit PCOS-associated traits, have also been shown to incur reproductive costs. Specifically, high testosterone levels and high BMI have both been linked to reduced rates of ovulation [e.g., (183–185)]. These findings are suggestive of a trade-off between investment in maintenance and survival (which is associated with higher testosterone, higher BMI, higher BMD, some degree of insulin resistance, and associated fat storage more in visceral than gluteofemoral deposits), compared to investment in higher fertility and faster reproduction (which is associated with lower testosterone) (13). Such trade-offs have yet to be investigated in females but are consistent with a model whereby PCOS and endometriosis represent diametric disorders that reflect, in part, slow vs. fast life histories (12, 13).
In a life history context, the higher BMIs of females with PCOS may also represent differential investment in visceral “survival fat” that enhances survivorship during food shortages and when subject to infectious disease risks (186–188); a slower life history is also suggested by the later ages of menarche found among average-weight females with PCOS (189–191), and the later age of menopause found among females with this condition (192–194). Among healthy females, later age of menarche has been associated with a suite of traits, including reduced mortality rates, and a longer lifespan (195–198). Such life history considerations, the hypothesized links between testosterone-related phenotypes in females, and the dysregulation of PCOS-associated traits due to modern environments, are depicted in Figure 2.
While reduced ovulation rates represent a clear correlate of high testosterone and PCOS, such reductions in fertility are reversible and contingent on physiological condition. Thus, weight loss in non-ovulating, high-BMI females commonly results in the restoration of normal ovulation (199–201). For example, Pasquali et al. (199) found that a mean weight loss of about 10 kg in 20 obese amenorrhoeic hyperandrogenic females led to the resumption of menstrual cycles in 14 (70%) of them. Similarly, Clark et al. (201) reported that after a mean weight loss of 6.5 kg, 90% of previously anovulatory obese females were ovulating spontaneously by the fifth month of the weight loss program. These findings indicate that the reproductive costs of PCOS, in terms of reduced ovulation rates, may be considerably reduced in average-weight females compared to those who are overweight. Thus, females with PCOS in ancestral populations, who were probably lean under most conditions, may have incurred relatively few reproductive costs from relatively high testosterone in terms of ovulation rates, compared to females in modern populations.
Limitations and implications
The main limitation of this study is that although the reviews conducted are systematic, and thus unbiased regarding the hypotheses addressed, they are not exhaustive, nor have the findings been subject to meta-analyses. As such, further studies would be needed for more detailed, and functionally in-depth, analyses for any specific hypothesis of the many addressed here.
The findings reported here have several implications for future research and for treatment of PCOS in the context of its fitness-related costs and benefits. First, this work has identified a number of data gaps, including lacks of information on: (1) social and physical dominance in females with PCOS, (2) muscularity and BMD in lean females with PCOS, (3) whether female strength and muscularity are associated with markers of prenatal testosterone (especially anogenital distance); (4) levels of testosterone in females from traditional populations who engage in heavy labor, and (5) the means whereby dominant, high-testosterone females of non-human mammalian species apparently avoid the physiological, PCOS-related costs of high testosterone.
Second, if risk for PCOS evolved in the context of ecological situations favoring high levels of labor by females, and relatively high testosterone and muscularity, then treatments for PCOS may usefully focus on weight-bearing and high-intensity resistance training exercise. Such exercise, which has demonstrated positive effects on females with PCOS in the few studies conducted to date (202, 203), in part through effects in reducing insulin resistance (204), may represent the behavioral-ecological condition most relevant to the selective pressures that led to PCOS-related traits. As such, strength and resistance training should tend to simulate ancestral conditions for females with PCOS-related traits, and, in theory, may promote more-regular ovulation, insulin sensitivity, and higher fertility more effectively than other forms of exercise or treatment.
Conclusions
This overview of systematic reviews provides evidence that the risks of PCOS in current populations may, in part, reflect extreme and maladaptive manifestations of adaptive traits. These traits, particularly those linked with higher testosterone levels, appear to offer benefits to females in terms of muscularity, athletic performance, strength, and dominance. The findings also provide support for PCOS evidence-based guidelines for physical activity, whereby treatments for PCOS could usefully focus on weight loss, strength training, and resistance training exercises.
Author contributions
AB: Conceptualization, Data curation, Formal Analysis, Investigation, Methodology, Visualization, Writing – original draft, Writing – review & editing. BC: Conceptualization, Formal Analysis, Funding acquisition, Methodology, Project administration, Resources, Supervision, Validation, Visualization, Writing – original draft, Writing – review & editing.
Funding
The author(s) declare financial support was received for the research, authorship, and/or publication of this article. This project was funded by NSERC Discovery Grant 2019-04208.
Conflict of interest
The authors declare that the research was conducted in the absence of any commercial or financial relationships that could be construed as a potential conflict of interest.
Publisher's note
All claims expressed in this article are solely those of the authors and do not necessarily represent those of their affiliated organizations, or those of the publisher, the editors and the reviewers. Any product that may be evaluated in this article, or claim that may be made by its manufacturer, is not guaranteed or endorsed by the publisher.
Supplementary material
The Supplementary Material for this article can be found online at: https://www.frontiersin.org/articles/10.3389/frph.2024.1475132/full#supplementary-material
References
1. McCartney CR, Marshall JC. Polycystic ovary syndrome. N Engl J Med. (2016) 375:54–64. doi: 10.1056/NEJMcp1514916
2. March W, Moore V, Willson K, Phillips DIW, Norman RJ, Davies MJ, et al. The prevalence of polycystic ovary syndrome in a community sample assessed under contrasting diagnostic criteria. Hum Reprod. (2010) 25:544–51. doi: 10.1093/humrep/dep399
3. Vercellini P, Viganò P, Somigliana E, Fedele L. Endometriosis: pathogenesis and treatment. Nat Rev Endocrinol. (2014) 10:261–75. doi: 10.1038/nrendo.2013.255
4. Mykhalchenko K, Lizneva D, Trofimova T, Walker W, Suturina L, Diamond MP, et al. Genetics of polycystic ovary syndrome. Expert Rev Mol Diagn. (2017) 17:723–33. doi: 10.1080/14737159.2017.1340833
5. Vink JM, Sadrzadeh S, Lambalk CB, Boomsma DI. Heritability of polycystic ovary syndrome in a Dutch twin-family study. J Clin Endocrinol Metab. (2006) 91:2100–04. doi: 10.1210/jc.2005-1494
6. Rosenfield R. Identifying children at risk for polycystic ovary syndrome. J Clin Endocrinol Metab. (2007) 92:787–96. doi: 10.1210/jc.2006-2012
7. Witchel S, Oberfield S, Peña A. Polycystic ovary syndrome: pathophysiology, presentation, and treatment with emphasis on adolescent girls. J Endocr Soc. (2019) 3:1545–73. doi: 10.1210/js.2019-00078
8. Parasar P, Ozcan P, Terry K. Endometriosis: epidemiology, diagnosis, and clinical management. Curr Obstet Gynecol Rep. (2017) 6:34–41. doi: 10.1007/s13669-017-0187-1
9. Charifson M, Trumble B. Evolutionary origins of polycystic ovary syndrome: an environmental mismatch disorder. Evol Med Public Health. (2019) 2019:50–63. doi: 10.1093/emph/eoz011
10. Dinsdale N, Crespi B. Endometriosis and polycystic ovary syndrome are diametric disorders. Evol Appl. (2021) 14:1693–715. doi: 10.1111/eva.13244
11. Parker J, O’Brien C, Hawrelak J, Gersh FL. Polycystic ovary syndrome: an evolutionary adaptation to lifestyle and the environment. Int J Environ Res Public Health. (2022) 19:1336. doi: 10.3390/ijerph19031336
12. Crespi B, Dinsdale NL. The sexual selection of endometriosis. Evol Behav Sci. (2023) 17:123–70. doi: 10.1037/ebs0000275
13. Dinsdale N, Nepomnashcy P, Crespi B. The evolutionary biology of endometriosis. Evol Med Public Health. (2021) 9:174–91. doi: 10.1093/emph/eoab008
14. Good C, Tulchinsky M, Mauger D, Demers LM, Legro RS. Bone mineral density and body composition in lean women with polycystic ovary syndrome. Fertil Steril. (1999) 72:21–5. doi: 10.1016/S0015-0282(99)00203-4
15. Corbett S, McMichael A, Prentice A. Type 2 diabetes, cardiovascular disease, and the evolutionary paradox of the polycystic ovary syndrome: a fertility first hypothesis. Am J Hum Biol. (2009) 21:587–98. doi: 10.1002/ajhb.20937
16. Azziz R, Dumesic D, Goodarzi M. Polycystic ovary syndrome: an ancient disorder? Fertil Steril. (2011) 95:1544–8. doi: 10.1016/j.fertnstert.2010.09.032
17. Fessler D, Natterson-Horowitz B, Azziz R. Evolutionary determinants of polycystic ovary syndrome: part 2. Fertil Steril. (2016) 106:42–7. doi: 10.1016/j.fertnstert.2016.05.016
18. Ünlütürk U, Sezgin E, Yildiz B. Evolutionary determinants of polycystic ovary syndrome: part 1. Fertil Steril. (2016) 106:33–41. doi: 10.1016/j.fertnstert.2016.05.010
19. Mohammad M, Seghinsara A. Polycystic ovary syndrome (PCOS), diagnostic criteria, and AMH. Asian Pac J Cancer Prev. (2017) 18:17–21. doi: 10.22034/APJCP.2017.18.1.17
20. Qin JZ, Pang LH, Li MJ, Fan XJ, Huang RD, Chen HY. Obstetric complications in women with polycystic ovary syndrome: a systematic review and meta-analysis. Reprod Biol Endocrinol. (2013) 11:56. doi: 10.1186/1477-7827-11-56
21. Khomami MB, Tehrani FR, Hashemi S, Farahmand M, Azizi F. Of PCOS symptoms, hirsutism has the most significant impact on the quality of life of Iranian women. PLoS One. (2015) 10:e0123608. doi: 10.1371/journal.pone.0123608
22. Mathur R, Alexander CJ, Yano J, Trivax V, Azziz R. Use of metformin in polycystic ovary syndrome. Am J Obstet Gynecol. (2008) 199:596–609. doi: 10.1016/j.ajog.2008.09.010
23. Marshall J, Dunaif A. All women with PCOS should be treated for insulin resistance. Fertil Steril. (2012) 97:18–22. doi: 10.1016/j.fertnstert.2011.11.036
24. Day F, Karaderi T, Jones M, Meun C, He C, Drong A, et al. Large-scale genome-wide meta-analysis of polycystic ovary syndrome suggests shared genetic architecture for different diagnosis criteria. PLoS Genet. (2018) 14:e1007813. doi: 10.1371/journal.pgen.1007813
25. Ding H, Zhang J, Zhang F, Zhang S, Chen X, Liang W, et al. Resistance to the insulin and elevated level of androgen: a major cause of polycystic ovary syndrome. Front Endocrinol (Lausanne). (2021) 12:741764. doi: 10.3389/fendo.2021.741764
26. Sanchez-Garrido MA, Tena-Sempere M. Metabolic dysfunction in polycystic ovary syndrome: pathogenic role of androgen excess and potential therapeutic strategies. Mol Metab. (2020) 35:100937. doi: 10.1016/j.molmet.2020.01.001
27. Toosy S, Sodi R, Pappachan J. Lean polycystic ovary syndrome (PCOS): an evidence-based practical approach. J Diabetes Metab Disord. (2018) 17:277–85. doi: 10.1007/s40200-018-0371-5
28. Abbott D, Barnett D, Levine J, Padmanabhan V, Dumesic DA, Jacoris S, et al. Endocrine antecedents of polycystic ovary syndrome in fetal and infant prenatally androgenized female rhesus monkeys. Biol Reprod. (2008) 79:154–63. doi: 10.1095/biolreprod.108.067702
29. Wu X, Li Z, Wu C, Liu YM, Lin H, Wang SH, et al. Endocrine traits of polycystic ovary syndrome in prenatally androgenized female sprague-dawley rats. Endocr J. (2010) 57:201–9. doi: 10.1507/endocrj.K09E-205
30. Mimouni NE, Giacobini P. Polycystic ovary syndrome mouse model by prenatal exposure to high anti-Müllerian hormone. STAR Protoc. (2021) 2:100684. doi: 10.1016/j.xpro.2021.100684
31. Barrett E, Hoeger K, Sathyanarayana S, Abbott DH, Redmon JB, Nguyen RNH, et al. Anogenital distance in newborn daughters of women with polycystic ovary syndrome indicates fetal testosterone exposure. J Dev Orig Health Dis. (2018) 9:307–14. doi: 10.1017/S2040174417001118
32. Sanchez-Ferrer ML, Mendiola J, Hernandez-Penalver AI, Biyang-Corbalán S, Barnosi-Carmona A, Sánchez-Prieto MT, et al. Presence of polycystic ovary syndrome is associated with longer anogenital distance in adult Mediterranean women. Hum Reprod. (2017) 32:2315–23. doi: 10.1093/humrep/dex274
33. Wu Y, Zhong G, Chen S, Zheng C, Liao D, Xie M. Polycystic ovary syndrome is associated with anogenital distance, a marker of prenatal androgen exposure. Hum Reprod. (2017) 32:937–43. doi: 10.1093/humrep/dex042
34. Risal S, Pei Y, Lu H, Manti M, Fornes R, Pui HP, et al. Prenatal androgen exposure and transgenerational susceptibility to polycystic ovary syndrome. Nat Med. (2019) 25:1894–904. doi: 10.1038/s41591-019-0666-1
35. Dumesic D, Abbott D, Padmanabhan V. Polycystic ovary syndrome and its developmental origins. Rev Endocr Metab Disord. (2007) 8:127–41. doi: 10.1007/s11154-007-9046-0
36. Dumesic DA, Padmanabhan V, Chazenbalk GD, Abbott DH. Polycystic ovary syndrome as a plausible evolutionary outcome of metabolic adaptation. Reprod Biol Endocrinol. (2023) 20:12. doi: 10.1186/s12958-021-00878-y
37. Pollard TM, Unwin N, Fischbacher C, Chamley JK. Differences in body composition and cardiovascular and type 2 diabetes risk factors between migrant and British-born British Pakistani women. Am J Hum. (2008) 20:545–9. doi: 10.1002/ajhb.20773
38. Merkin SS, Phy JL, Sites CK, Yang D. Environmental determinants of polycystic ovary syndrome. Fertil Steril. (2016) 1:16–24. doi: 10.1016/j.fertnstert.2016.05.011
39. Abbott AD, Colman RJ, Tiefenthaler R, Dumesic DA, Abbott DH. Early-to-mid gestation fetal testosterone increases right hand 2D:4D finger length ratio in polycystic ovary syndrome-like monkeys. PLoS One. (2012) 7:e42372. doi: 10.1371/journal.pone.0042372
40. Abbott D, Dumesic D, Levine J. Hyperandrogenic origins of polycystic ovary syndrome — implications for pathophysiology and therapy. Expert Rev Endocrinol Metab. (2019) 14:131–43. doi: 10.1080/17446651.2019.1576522
41. Thankamony A, Pasterski V, Ong KK, Acerini CL, Hughes IA. Anogenital distance as a marker of androgen exposure in humans. Andrology. (2016) 4:616–25. doi: 10.1111/andr.12156
42. Zheng Z, Cohn M. Developmental basis of sexually dimorphic digit ratios. Proc Natl Acad Sci U S A. (2011) 108:16289–94. doi: 10.1073/pnas.1108312108
43. Manning J. Resolving the role of prenatal sex steroids in the development of digit ratio. Proc Natl Acad Sci U S A. (2011) 108:16143–4. doi: 10.1073/pnas.1113312108
44. Manning J, Fink B. Digit ratio (2D:4D), dominance, reproductive success, asymmetry, and sociosexuality in the BBC internet study. Am J Hum Biol. (2008) 20:451–61. doi: 10.1002/ajhb.20767
45. Collado-Boira E, Baliño P, Boldo-Roda A, Martínez-Navarro I, Hernando B, Recacha-Ponce P, et al. Influence of female sex hormones on ultra-running performance and post-race recovery: role of testosterone. Int J Environ Res Public Health. (2021) 18:10403. doi: 10.3390/ijerph181910403
46. Zhou W, Zeng G, Lyu C, Kou F, Zhang S, Wei H. The effect of strength-endurance training on serum and urine metabolic profiles of female adolescent volleyball athletes. Physiol Int. (2021) 108:285–302. doi: 10.1556/2060.2021.00150
47. Ahmetov II, Stepanova AA, Biktagirova EM, Semenova E, Shchuplova IS, Bets LV, et al. Is testosterone responsible for athletic success in female athletes? J Sports Med Phys Fitness. (2020) 60:1377–82. doi: 10.23736/S0022-4707.20.10171-3
48. Hirschberg AL, Elings Knutsson J, Helge T, Godhe M, Ekblom M, Bermon S, et al. Effects of moderately increased testosterone concentration on physical performance in young women: a double blind, randomised, placebo controlled study. Brit J Sports Med. (2020) 54:599–604. doi: 10.1136/bjsports-2018-100525
49. Crewther BT, Cook CJ. A longitudinal analysis of salivary testosterone concentrations and competitiveness in elite and non-elite women athletes. Physiol Behav. (2018) 188:157–61. doi: 10.1016/j.physbeh.2018.02.012
50. Arazi H, Khanmohammadi A, Asadi A, Haff GG. The effect of resistance training set configuration on strength, power, and hormonal adaptation in female volleyball players. Appl Physiol Nutr Metab. (2018) 43:154–64. doi: 10.1139/apnm-2017-0327
51. Crewther B, Obminski Z, Cook C. The effect of steroid hormones on the physical performance of boys and girls during an Olympic weightlifting competition. Pediatr Exerc Sci. (2016) 28:580–7. doi: 10.1123/pes.2016-0070
52. Łagowska K, Kapczuk K. Testosterone concentrations in female athletes and ballet dancers with menstrual disorders. Eur J Sport Sci. (2016) 16:490–7. doi: 10.1080/17461391.2015.1034786
53. Nunes JA, Moreira A, Crewther BT, Nosaka K, Viveiros L, Aoki MS. Monitoring training load, recovery-stress state, immune-endocrine responses, and physical performance in elite female basketball players during a periodized training program. J Strength Cond Res. (2014) 28:2973–80. doi: 10.1519/JSC.0000000000000499
54. Huang G, Basaria S, Travison T, Ho MH, Davda M, Mazer NA, et al. Testosterone dose-response relationships in hysterectomized women with or without oophorectomy: effects on sexual function, body composition, muscle performance and physical function in a randomized trial. Menopause. (2014) 21:612–23. doi: 10.1097/GME.0000000000000093
55. Cook CJ, Beaven CM. Salivary testosterone is related to self-selected training load in elite female athletes. Physiol Behav. (2013) 116–117:8–12. doi: 10.1016/j.physbeh.2013.03.013
56. Caruso JF, Lutz BM, Davidson ME, Wilson K, Crane CC, Craig CE, et al. Salivary hormonal values from high-speed resistive exercise workouts. J Strength Cond Res. (2012) 26:625–32. doi: 10.1519/JSC.0b013e31822c7267
57. Le Panse B, Labsy Z, Baillot A, Vibarel-Rebot N, Parage G, Albrings D, et al. Changes in steroid hormones during an international powerlifting competition. Steroids. (2012) 77:1339–44. doi: 10.1016/j.steroids.2012.07.015
58. Coste O, Paris F, Galtier F, Letois F, Maïmoun L, Sultan C. Polycystic ovary-like syndrome in adolescent competitive swimmers. Fertil Steril. (2011) 96:1037–42. doi: 10.1016/j.fertnstert.2011.07.006
59. Nunes JA, Crewther BT, Ugrinowitsch C, Tricoli V, Viveiros L, de Rose Jr D, et al. Salivary hormone and immune responses to three resistance exercise schemes in elite female athletes. J Strength Cond Res. (2011) 25:2322–7. doi: 10.1519/JSC.0b013e3181ecd033
60. Nunes JA, Crewther BT, Viveiros L, de Rose Jr D, Aoki MS. Effects of resistance training periodization on performance and salivary immune-endocrine responses of elite female basketball players. J Sports Med Phys Fitness. (2011) 51:676–82.22212272
61. Pilz-Burstein R, Ashkenazi Y, Yaakobovitz Y, Cohen Y, Zigel L, Nemet D, et al. Hormonal response to taekwondo fighting simulation in elite adolescent athletes. Eur J Appl Physiol. (2010) 110:1283–90. doi: 10.1007/s00421-010-1612-6
62. Eliakim A, Portal S, Zadik Z, Rabinowitz J, Adler-Portal D, Cooper DM, et al. The effect of a volleyball practice on anabolic hormones and inflammatory markers in elite male and female adolescent players. J Strength Cond Res. (2009) 23:1553–9. doi: 10.1519/JSC.0b013e3181aa1bcb
63. Cardinale M, Stone MH. Is testosterone influencing explosive performance? J Strength Cond Res. (2006) 20:103–7. doi: 10.1519/R-16864.1
64. Kraemer WJ, Hakkinen K, Triplett-Mcbride NT, Fry AC, Koziris LP, Ratamess NA, et al. Physiological changes with periodized resistance training in women tennis players. Med Sci Sports Exerc. (2003) 35:157–68. doi: 10.1097/00005768-200301000-00024
65. Bosco C, Colli R, Bonomi R, Viru A. Monitoring strength training: neuromuscular and hormonal profile. Med Sci Sports Exerc. (2000) 32:202–8. doi: 10.1097/00005768-200001000-00030
66. Häkkinen K, Pakarinen A, Hannonen P, Häkkinen A, Airaksinen O, Valkeinen H, et al. Effects of strength training on muscle strength, cross-sectional area, maximal electromyographic activity, and serum hormones in premenopausal women with fibromyalgia. J Rheumatol. (2002) 29(6):1287–95.
67. Kobayashi T, Seki S, Hwang I. Effects of resistance training on bone mineral density and resting serum hormones in female collegiate distance runners: a randomized controlled pilot trial. J Sports Med Phys Fitness. (2023) 63(6):765–72. doi: 10.23736/S0022-4707.23.14571-3
68. Moghadasi M, Siavashpour S. The effect of 12 weeks of resistance training on hormones of bone formation in young sedentary women. Eur J Appl Physiol. (2013) 113(1):25–32. doi: 10.1007/s00421-012-2410-0
69. Kraemer WJ, Fleck SJ, Dziados JE, Harman EA, Marchitelli LJ, Gordon SE, et al. Changes in hormonal concentrations after different heavy-resistance exercise protocols in women. J Appl Physiol. (1993) 75(2):594–604. doi: 10.1152/jappl.1993.75.2.594
70. Marx JO, Ratamess NA, Nindl BC, Gotshalk LA, Volek JS, Dohi K, et al. Low-volume circuit versus high-volume periodized resistance training in women. Med Sci Sports Exerc. (2001) 33(4):635–43. doi: 10.1097/00005768-200104000-00019
71. Sallinen J, Pakarinen A, Fogelholm M, Sillanpää E, Alen M, Volek JS, et al. Serum basal hormone concentrations and muscle mass in aging women: effects of strength training and diet. Int J Sport Nutr Exerc Metab. (2006) 16(3):316–31. doi: 10.1123/ijsnem.16.3.316
72. Yoo EJ, Jun TW, Hawkins SA. The effects of a walking exercise program on fall-related fitness, bone metabolism, and fall-related psychological factors in elderly women. Res Sports Med. (2010) 18(4):236–50. doi: 10.1080/15438627.2010.510098
73. Bezuglov E, Ahmetov II, Lazarev A, Mskhalaya G, Talibov O, Ustinov V, et al. The relationship of testosterone levels with sprint performance in young professional track and field athletes. Physiol Behav. (2023) 1(271):114344. doi: 10.1016/j.physbeh.2023.114344
74. Eklund E, Berglund B, Labrie F, Carlström K, Ekström L, Hirschberg AL. Serum androgen profile and physical performance in women Olympic athletes. Br J Sports Med. (2017) 51(17):1–9. doi: 10.1136/bjsports-2017-097582
75. Bermon S, Garnier PY, Hirschberg AL, Robinson N, Giraud S, Nicoli R, et al. Serum androgen levels in elite female athletes. J Clin Endocrinol Metab. (2014) 99(11):4328–35. doi: 10.1210/jc.2014-1391
76. Crewther BT, Obmiński Z, Orysiak J, Al-Dujaili EAS. The utility of salivary testosterone and cortisol concentration measures for assessing the stress responses of junior athletes during a sporting competition. J Clin Lab Anal. (2018) 32(1):e22197. doi: 10.1002/jcla.22197
77. Copeland JL, Consitt LA, Tremblay MS. Hormonal responses to endurance and resistance exercise in females aged 19–69 years. J Gerontol Ser A. (2002) 57(4):B158–65. doi: 10.1093/gerona/57.4.B158
78. Bermon S, Garnier PY. Serum androgen levels and their relation to performance in track and field: mass spectrometry results from 2127 observations in male and female elite athletes. Br J Sports Med. (2017) 51:1309–14. doi: 10.1136/bjsports-2017-097792
79. Casto KV, Arthur LC, Edwards DA, Hamilton D. Testosterone, athletic context, oral contraceptive use, and competitive persistence in women. Adapt Hum Behav Physiol. (2022) 8:52–78. doi: 10.1007/s40750-021-00180-6
80. Muscella A, My G, Okba S, Zangla D, Bianco A, Marsigliante S. Effects of training on plasmatic cortisol and testosterone in football female referees. Physiol Rep. (2022) 10:e15291. doi: 10.14814/phy2.15291
81. Eklund E, Andersson A, Ekström L, Hirschberg AL. Urinary steroid profile in elite female athletes in relation to serum androgens and in comparison with untrained controls. Front Physiol. (2021) 12:702305. doi: 10.3389/fphys.2021.702305
82. Cook CJ, Kilduff LP, Crewther BT. Basal and stress-induced salivary testosterone variation across the menstrual cycle and linkage to motivation and muscle power. Scand J Med Sci Sports. (2018) 28:1345–53. doi: 10.1111/sms.13041
83. Maïmoun L, Coste O, Philibert P, Briot K, Mura T, Galtier F, et al. Testosterone secretion in elite adolescent swimmers does not modify bone mass acquisition: a 1-year follow-up study. Fertil Steril. (2013) 99:270–8. doi: 10.1016/j.fertnstert.2012.08.020
84. Cook CJ, Crewther BT, Smith AA. Comparison of baseline free testosterone and cortisol concentrations between elite and non-elite female athletes. Am J Hum Biol. (2012) 24:856–8. doi: 10.1002/ajhb.22302
85. Russell M, Stark J, Nayak S, Miller KK, Herzog DB, Klibanski A, et al. Peptide YY in adolescent athletes with amenorrhea, eumenorrheic athletes and non-athletic controls. Bone. (2009) 45:104–9. doi: 10.1016/j.bone.2009.03.668
86. Rickenlund A, Thorén M, Carlström K, Schoultz BO, Hirschberg AL. Diurnal profiles of testosterone and pituitary hormones suggest different mechanisms for menstrual disturbances in endurance athletes. J Clin Endocrinol Metab. (2004) 89:702–7. doi: 10.1210/jc.2003-030306
87. Uusitalo AL, Uusitalo AJ, Rusko HK. Exhaustive endurance training for 6–9 weeks did not induce changes in intrinsic heart rate and cardiac autonomic modulation in female athletes. Int J Sports Med. (1998) 19:532–40. doi: 10.1055/s-2007-971956
88. Granger DA, Shirtcliff EA, Booth A, Kivlighan K, Schwartz EB. The “trouble” with salivary testosterone. Psychoneuroendocrinology. (2004) 29(10):1229–40. doi: 10.1016/j.psyneuen.2004.02.005
89. Nunes E, Gallardo E, Morgado-Nunes S, Fonseca-Moutinho J. Steroid hormone levels and bone mineral density in women over 65 years of age. Sci Rep. (2023) 13:4925. doi: 10.1038/s41598-023-32100-x
90. Orozco P, Navarro MA, Nolla JM. Salivary testosterone is associated with higher lumbar bone mass in premenopausal healthy women with normal levels of serum testosterone. Eur J Epidemiol. (2000) 16:907–12. doi: 10.1023/A:1011064606060
91. Kogure GS, Silva RC, Miranda-Furtado CL, Ribeiro VB, Pedroso DCC, Melo AS, et al. Hyperandrogenism enhances muscle strength after progressive resistance training, independent of body composition, in women with polycystic ovary syndrome. J Strength Cond Res. (2018) 32:2642–51. doi: 10.1519/JSC.0000000000002714
92. Konieczna A, Rachoń D, Owczarek K, Kubica P, Kowalewska A, Kudlak B, et al. Serum bisphenol A concentrations correlate with serum testosterone levels in women with polycystic ovary syndrome. Reprod Toxicol. (2018) 82:32–7. doi: 10.1016/j.reprotox.2018.09.006
93. Deng Y, Zhang Y, Li S, Zhou W, Ye L, Wang L, et al. Steroid hormone profiling in obese and nonobese women with polycystic ovary syndrome. Sci Rep. (2017) 7:14156. doi: 10.1038/s41598-017-14534-2
94. Baioccato V, Quinto G, Rovai S, Conte F, Dassie F, Neunhäeuserer D, et al. Do androgenic pattern, insulin state and growth hormone affect cardiorespiratory fitness and strength in young women with PCOS? Biomedicines. (2022) 10:2176. doi: 10.3390/biomedicines10092176
95. Kogure GS, Ribeiro VB, Gennaro FGO, Ferriani RA, Miranda-Furtado CL, Dos Reis R. Physical performance regarding handgrip strength in women with polycystic ovary syndrome. Rev Bras Ginecol Obstet. (2020) 42:811–19. doi: 10.1055/s-0040-1718953
96. Guzelce EC, Eyupoglu D, Torgutalp S, Aktoz F, Portakal O, Demirel H, et al. Is muscle mechanical function altered in polycystic ovary syndrome? Arch Gynecol Obstet. (2019) 300:771–6. doi: 10.1007/s00404-019-05229-2
97. Vieira de Melo MH, Albuquerque Barbosa Cabral Micussi MT, Dias de Medeiros R, Cobucci RN, de Oliveira Maranhão TM, Gonçalves AK. Pelvic floor muscle thickness in women with polycystic ovary syndrome. Clin Exp Obstet Gynecol. (2018) 45:813–16. doi: 10.12891/ceog4113.2018
98. Micussi MT, Freitas RP, Varella L, Soares EM, Lemos TM, Maranhão TC. Relationship between pelvic floor muscle and hormone levels in polycystic ovary syndrome. Neurourol Urodyn. (2016) 35:780–5. doi: 10.1002/nau.22817
99. Vassimon FI, Ferreira CH, Martins WP, Ferriani RA, de Andrade Batista RL, Bo K. Surface electromyography and ultrasound evaluation of pelvic floor muscles in hyperandrogenic women. Int Urogynecol J. (2016) 27:587–91. doi: 10.1007/s00192-015-2865-8
100. Kogure GS, Miranda-Furtado CL, Silva RC, Melo AS, Ferriani RA, Silva De Sá MF. Resistance exercise impacts lean muscle mass in women with polycystic ovary syndrome. Med Sci Sports Exerc. (2016) 48:589–98. doi: 10.1249/MSS.0000000000000822
101. Kogure GS, Silva RC, Picchi Ramos FK, Miranda-Furtado CL, da Silva Lara LA, Ferriani RA, et al. Women with polycystic ovary syndrome have greater muscle strength irrespective of body composition. Gynecol Endocrinol. (2015) 31:237–42. doi: 10.3109/09513590.2014.982083
102. Antônio FI, Bø K, Ferriani RA, Silva de Sá MF, de Sá Rosa e Silva ACJ, Ferreira CHJ. Pelvic floor muscle strength and urinary incontinence in hyperandrogenic women with polycystic ovary syndrome. Int Urogynecol J. (2013) 24:1709–14. doi: 10.1007/s00192-013-2095-x
103. Mario FM, do Amarante F, Toscani MK, Spritzer PM. Lean muscle mass in classic or ovulatory PCOS: association with central obesity and insulin resistance. Exp Clin Endocrinol Diabetes. (2012) 120:511–16. doi: 10.1055/s-0032-1309006
104. Schmidt J, Dahlgren E, Brännström M, Landin-Wilhelsen K. Body composition, bone mineral density and fractures in late postmenopausal women with polycystic ovary syndrome — a long-term follow-up study. Clin Endocrinol (Oxf). (2012) 77:207–14. doi: 10.1111/j.1365-2265.2012.04378.x
105. Carmina E, Guastella E, Ra L, Rini GB, Lobo RA. Correlates of increased lean muscle mass in women with polycystic ovary syndrome. Eur J Endocrinol. (2009) 161:583–9. doi: 10.1530/EJE-09-0398
106. Thomson RL, Buckley JD, Moran LJ, Noakes M, Clifton PM, Norman RJ, et al. The effect of weight loss on anti-müllerian hormone levels in overweight and obese women with polycystic ovary syndrome and reproductive impairment. Hum Reprod. (2009) 24:1976–81. doi: 10.1093/humrep/dep101
107. Soyupek F, Guney M, Eris S, Cerci S, Yildiz A, Mungan T. Evaluation of hand functions in women with polycystic ovary syndrome. Gynecol Endocrinol. (2008) 24:571–5. doi: 10.1080/09513590802288218
108. Kirchengast S, Huber J. Body composition characteristics and body fat distribution in lean women with polycystic ovary syndrome. Hum Reprod. (2001) 16:1255–60. doi: 10.1093/humrep/16.6.1255
109. Fighera TM, Dos Santos BR, Spritzer PM. Lean mass and associated factors in women with PCOS with different phenotypes. PLoS One. (2023) 18(10):e0292623. doi: 10.1371/journal.pone.0292623
110. Çelik Eroğlu B, Karaçoban L, Idılman IS, Korkusuz F, Yildiz BO. Evaluation of muscle and bone composition and function in aging women with polycystic ovary syndrome. Maturitas. (2024) 185:107982. doi: 10.1016/j.maturitas.2024.107982
111. Ahmad B, Zahra SM. The impact of polycystic ovary syndrome on the sporting performance of female players. J Ther Rehabil Sci. (2020) 1(2):26–30. doi: 10.54393/tt.v1i2.22
112. Hansen SL, Bojsen-Møller KN, Lundsgaard AM, Hendrich FL, Nilas L, Sjøberg KA, et al. Mechanisms underlying absent training-induced improvement in insulin action in lean, hyperandrogenic women with polycystic ovary syndrome. Diabetes. (2020) 69(11):2267–80. doi: 10.2337/db20-0062
113. Napoli N, Conte C, Pedone C, Strotmeyer ES, Barbour KE, Black DM, et al. Effect of insulin resistance on BMD and fracture risk in older adults. J Clin Endocrinol Metab. (2019) 104:3303–10. doi: 10.1210/jc.2018-02539
114. Shanbhogue V, Finkelstein J, Bouxsein M, Yu EW. Association between insulin resistance and bone structure in nondiabetic postmenopausal women. J Clin Endocrinol Metab. (2016) 101:3114–22. doi: 10.1210/jc.2016-1726
115. Braunstein G, Reitz R, Buch A, Schnell D, Caulfield MP. Testosterone reference ranges in normally cycling healthy premenopausal women. J Sex Med. (2011) 8:2924–34. doi: 10.1111/j.1743-6109.2011.02380.x
116. Trumble BC, Pontzer H, Stieglitz J, Cummings DK, Wood B, Thompson ME, et al. Energetic costs of testosterone in two subsistence populations. Am J Hum Biol. (2023) 35:e23949. doi: 10.1002/ajhb.23949
117. Kakoly N, Khomami M, Joham A, Cooray SD, Misso ML, Norman RJ, et al. Ethnicity, obesity and the prevalence of impaired glucose tolerance and type 2 diabetes in PCOS: a systematic review and meta-regression. Hum Reprod Update. (2018) 24:455–67. doi: 10.1093/humupd/dmy007
118. Dumesic D, Abbott D, Sanchita S, Chazenbalk GD. Endocrine-metabolic dysfunction in polycystic ovary syndrome: an evolutionary perspective. Curr Opin Endocr Metab Res. (2020) 12:41–8. doi: 10.1016/j.coemr.2020.02.013
119. Azziz R, Marin C, Hoq L, Badamgarav E, Song P. Health care-related economic burden of the polycystic ovary syndrome during the reproductive life span. J Clin Endocrinol Metab. (2005) 90:4650–8. doi: 10.1210/jc.2005-0628
120. Hagmar M, Berglund B, Brismar K, Hirschberg AL. Hyperandrogenism may explain reproductive dysfunction in Olympic athletes. Med Sci Sports Exerc. (2009) 41:1241–8. doi: 10.1249/MSS.0b013e318195a21a
121. Lowe P, Kovacs G, Howlett D. Incidence of polycystic ovaries and polycystic ovary syndrome amongst women in Melbourne, Australia. Aust N Z J Obstet Gynaecol. (2005) 45:17–9. doi: 10.1111/j.1479-828X.2005.00334.x
122. Dadgostar H, Razi M, Aleyasin A. The relation between athletic sports and prevalence of amenorrhea and oligomenorrhea in Iranian female athletes. Sports Med Arthrosc Rehabil Ther Technol. (2009) 1:16. doi: 10.1186/1758-2555-1-16
123. Rickenlund A, Carlström K, Ekblom B, Brismar TB, von Schoultz B, Hirschberg AL. Hyperandrogenicity is an alternative mechanism underlying oligomenorrhea or amenorrhea in female athletes and may improve physical performance. Fertil Steril. (2003) 79:947–55. doi: 10.1016/S0015-0282(02)04850-1
124. Koltun K, Williams N, Scheid J, De Souza MJ. Discriminating hypothalamic oligomenorrhea/amenorrhea from hyperandrogenic oligomenorrhea/amenorrhea in exercising women. Appl Physiol Nutr Metab. (2020) 45:707–14. doi: 10.1139/apnm-2019-0640
125. Sum M, Warren M. Hypothalamic amenorrhea in young women with underlying polycystic ovary syndrome. Fertil Steril. (2009) 92:2106–8. doi: 10.1016/j.fertnstert.2009.05.063
126. Wang J, Lobo R. The complex relationship between hypothalamic amenorrhea and polycystic ovary syndrome. J Clin Endocrinol Metab. (2008) 93:1394–7. doi: 10.1210/jc.2007-1716
127. Loucks A, Mortola J, Girton L, Yen SS. Alterations in the hypothalamic-pituitary-ovarian and the hypothalamic-pituitary-adrenal axes in athletic women. J Clin Endocrinol Metab. (1989) 68:402–11. doi: 10.1210/jcem-68-2-402
128. Loucks A, Verdun M, Heath E. Low energy availability, not stress of exercise, alters LH pulsatility in exercising women. J Appl Physiol. (1998) 84:37–46. doi: 10.1152/jappl.1998.84.1.37
129. Awdishu S, Williams N, Laredo S, De Souza MJ. Oligomenorrhoea in exercising women: a polycystic ovarian syndrome phenotype or distinct entity? Sports Med. (2009) 39:1055–69. doi: 10.2165/11317910-000000000-00000
130. Joslyn W. Androgen-induced social dominance in infant female rhesus monkeys. J Child Psychol Psychiat. (1973) 14:137–45. doi: 10.1111/j.1469-7610.1973.tb01181.x
131. Batty K, Herbert J, Keverne E, Vellucci SV. Differences in blood levels of androgens in female talapoin monkeys related to their social status. Neuroendocrinology. (1986) 44:347–54. doi: 10.1159/000124667
132. Palanza P, Parmigiani S, vom Saal FS. Urine marking and maternal aggression of wild female mice in relation to anogenital distance at birth. Physiol Behav. (1995) 58:827–35. doi: 10.1016/0031-9384(95)00107-T
133. Beehner JC, Phillips-Conroy JE, Whitten PL. Female testosterone, dominance rank, and aggression in an Ethiopian population of hybrid baboons. Am J Primatol. (2005) 67:101–19. doi: 10.1002/ajp.20172
134. Petty J, Drea C. Female rule in lemurs is ancestral and hormonally mediated. Sci Rep. (2015) 5:9631. doi: 10.1038/srep09631
135. Davies C, Smyth K, Greene L, Walsh DA, Mitchell J, Clutton-Brock T, et al. Exceptional endocrine profiles characterise the meerkat: sex, status, and reproductive patterns. Sci Rep. (2016) 6:35492. doi: 10.1038/srep35492
136. Masson C, van der Westhuizen D, Noel JP, Prevost AM, van Honk J, Fotopoulou A, et al. Testosterone administration in women increases the size of their peripersonal space. Exp Brain Res. (2021) 239:1639–49. doi: 10.1007/s00221-021-06080-1
137. Shields AN, Brandes CM, Reardon KW, España RA, Tackett JL. Do testosterone and cortisol jointly relate to adolescent dominance? A pre-registered multi-method interrogation of the dual-hormone hypothesis. Adapt Hum Behav Physiol. (2021) 7:183–208. doi: 10.1007/s40750-021-00167-3
138. Enter D, Terburg D, Harrewijn A, Spinhoven P, Roelofs K. Single dose testosterone administration alleviates gaze avoidance in women with social anxiety disorder. Psychoneuroendocrinology. (2016) 63:26–33. doi: 10.1016/j.psyneuen.2015.09.008
139. Cobey KD, Nicholls M, Leongómez JD, Roberts CS. Self-reported dominance in women: associations with hormonal contraceptive use, relationship status, and testosterone. Adapt Hum Behav Physiol. (2015) 1:449–59. doi: 10.1007/s40750-015-0022-8
140. Ziomkiewicz A, Wichary S, Gomula A, Pawlowski B. Trait anxiety moderations the association between estradiol and dominance in women. Physiol Behav. (2015) 143:97–103. doi: 10.1016/j.physbeh.2015.02.045
141. Mehta PH, van Son V, Welker KM, Prasad S, Sanfey AG, Smidts A, et al. Exogenous testosterone in women enhances and inhibits competitive decision-making depending on victory-defeat experience and trait dominance. Psychoneuroendocrinology. (2015) 60:224–36. doi: 10.1016/j.psyneuen.2015.07.004
142. Enter D, Spinhoven P, Roelofs K. Alleviating social avoidance: effects of single dose testosterone administration on approach-avoidance action. Horm Behav. (2014) 65:351–4. doi: 10.1016/j.yhbeh.2014.02.001
143. Terburg D, Aarts H, van Honk J. Testosterone affects gaze aversion from angry faces outside of conscious awareness. Psychol Sci. (2012) 23:459–63. doi: 10.1177/0956797611433336
144. Stanton SJ, Schultheiss OC. Basal and dynamic relationships between implicit power motivation and estradiol in women. Horm Behav. (2007) 52:571–80. doi: 10.1016/j.yhbeh.2007.07.002
145. van Honk J, Tuiten A, Hermans E, Putman P, Koppeschaar H, Thijssen J, et al. A single administration of testosterone induces cardiac accelerative responses to angry faces in healthy young women. Behav Neurosci. (2001) 115:238–42. doi: 10.1037/0735-7044.115.1.238
146. Grant VJ, France JT. Dominance and testosterone in women. Biol Psychol. (2001) 58:41–7. doi: 10.1016/S0301-0511(01)00100-4
147. Dabbs J, Hargrove M. Age, testosterone, and behavior among female prison inmates. Psychosomatic Med. (1997) 59:477–80. doi: 10.1097/00006842-199709000-00003
148. Cashdan E. Hormones, sex, and status in women. Horm Behav. (1995) 29:354–66. doi: 10.1006/hbeh.1995.1025
149. Rejeski WJ, Parker PE, Gagne M, Koritnik DR. Cardiovascular and testosterone responses to contested dominance in women. Health Psychol. (1990) 9:35–47. doi: 10.1037/0278-6133.9.1.35
150. Madison G, Aasa U, Wallert J, Woodley MA. Feminist activist women are masculinized in terms of digit-ratio and social dominance: a possible explanation for the feminist paradox. Front Psychol. (2014) 5:1011. doi: 10.3389/fpsyg.2014.01011
151. Miller M, Agarwal S, Aristizabal L, Langebaek C. The daily grind: sex- and age-related activity patterns inferred from cross-sectional geometry of long bones in a pre-Columbian muisca population from tibanica, Colombia. Am J Phys Anthropol. (2018) 167:311–26. doi: 10.1002/ajpa.23629
152. Macintosh A, Pinhasi R, Stock J. Prehistoric women’s manual labor exceeded that of athletes through the first 5500 years of farming in central Europe. Sci Adv. (2017) 3:eaao3893. doi: 10.1126/sciadv.aao3893
153. Ogilvie M, Hilton E. Cross-sectional geometry in the humeri of foragers and farmers from the prehispanic American southwest: exploring patterns in the sexual division of labor. Am J Phys Anthropol. (2011) 144:11–21. doi: 10.1002/ajpa.21362
154. Kralick A, Zemel B. Evolutionary perspectives on the developing skeleton and implications for lifelong health. Front Endocrinol. (2020) 11:99. doi: 10.3389/fendo.2020.00099
155. Marchi D, Sparacello VS, Holt BM, Formicola V. Biomechanical approach to the reconstruction of activity patterns in neolithic western Liguria, Italy. Am J Phys Anthropol. (2006) 131:447–55. doi: 10.1002/ajpa.20449
156. Brødholt ET, Günther CC, Gautvik KM, Sjøvold T, Holck P. Bone mineral density through history: dual-energy x-ray absorptiometry in archaeological populations of Norway. J Archaeol Sci. (2021) 36:102792. doi: 10.1016/j.jasrep.2021.102792
157. Holck P. Bone mineral densities in the prehistoric, Viking-age, and medieval populations of Norway. Int J Osteoarchaeol. (2007) 17:199–206. doi: 10.1002/oa.870
158. Spinek AE, Lorkiewicz W, Mietlinska J, Sewerynek E, Klys A, Caramelli D, et al. Evaluation of chronological changes in bone fractures and age-related bone loss: a test case from Poland. J Archaeol Sci. (2016) 72:117–27. doi: 10.1016/j.jas.2016.06.007
159. Eshed V, Gopher A, Galili E, Hershkovitz I. Musculoskeletal stress markers in natufian hunter-gatherers and neolithic farmers in the levant: the upper limb. Am J Phys Anthropol. (2004) 123:303–15. doi: 10.1002/ajpa.10312
160. Chapman N. Evidence for spanish influence on activity induced musculoskeletal stress markers at Pecos pueblo. Int J Osteoarchaeol. (1997) 7:497–506. doi: 10.1002/(SICI)1099-1212(199709/10)7:5%3C497::AID-OA394%3E3.0.CO;2-H
161. Hawkey D, Merbs C. Activity-induced musculoskeletal stress markers (MSM) and subsistence strategy changes among ancient Hudson Bay eskimos. Int J Osteoarchaeol. (1995) 5:324–38. doi: 10.1002/oa.1390050403
162. Hershkovitz I, Gopher A. Demographic, biological and cultural aspects of the neolithic revolution: a view from the southern levant. In: Bocquet-Appel J-P, Bar-Yosef O, editors. The Neolithic Demographic Transition and its Consequences. Dordrecht: Springer (2008). p. 441–79. doi: 10.1007/978-1-4020-8539-0_17
163. Cassidy CM. Skeletal evidence for prehistoric subsistence adaptation in the central Ohio river valley. In: Cohen MH, editor. Paleopathology at the Origins of Agriculture. Tallahassee, FL: FDAHRM (1984). p. 307–38.
164. Bridges P. Changes in activities with the shift to agriculture in the southeastern United States. Curr Anthropol. (1989) 30:385–94. doi: 10.1086/203756
165. Weber AW, Bettinger R. Middle holocene hunter-gatherers of cis-baikal, siberia: an overview for the new century. J Anthropol Archaeol. (2010) 29:491–506. doi: 10.1016/j.jaa.2010.08.002
166. Anderson A, Chilczuk S, Nelson K, Ruther R, Wall-Scheffler C. The myth of man the hunter: women’s contribution to the hunt across ethnographic contexts. PLoS One. (2023) 18:e0287101. doi: 10.1371/journal.pone.0287101
167. Estioko-Griffin AA. Women as hunters: the case of an eastern cagayan agta group. In: Griffin PB, Estioko-Griffin AA, editors. The Agta of Northeastern Luzon: Recent Studies. Cebu City: University of San Carlos (1985). p. 18–32.
168. Goodman MJ, Griffin PB, Estioko-Griffin AA, Grove JS. The compatibility of hunting and mothering among the agta hunter-gatherers of the Philippines. Sex Roles. (1985) 12:1199–209. doi: 10.1007/BF00287829
169. Noss AJ, Hewlett BS. The contexts of female hunting in Central Africa. Am Anthropol. (2001) 103:1024–40. doi: 10.1525/aa.2001.103.4.1024
170. Khorasani DG, Lee SH. Women in human evolution redux. In: Willermet C, Lee SH, editors. Evaluating Evidence in Biological Anthropology. Cambridge: Cambridge University Press (2020). p. 11–34.
171. Casarini L, Simoni M, Brigante G. Is polycystic ovary syndrome a sexual conflict? A review. Reprod Biomed Online. (2016) 32:350–61. doi: 10.1016/j.rbmo.2016.01.011
172. Hirschberg A. Female hyperandrogenism and elite sport. Endocr Connect. (2020) 9:R81–92. doi: 10.1530/EC-19-0537
173. Pontzer H, Wood BM, Raichlen DA. Hunter-gatherers as models in public health. Obesity Rev. (2018) 19:24–35. doi: 10.1111/obr.12785
174. Hackney AC, Prado RCR, Dolan E. Androgenic steroid hormones and endurance exercise in athletic women. Endocrines. (2024) 5:252–60. doi: 10.3390/endocrines5030018
175. Monje C, Rada I, Castro-Sepulveda M, Peñailillo L, Deldicque L, Zbinden-Foncea H. Effects of a high intensity interval session on mucosal immune function and salivary hormones in male and female endurance athletes. J Sports Sci Med. (2020) 19:436–43.32390738
176. Nindl BC, Kraemer WJ, Gotshalk LA, Marx JO, Volek JS, Bush FA, et al. Testosterone responses after resistance exercise in women: influence of regional fat distribution. Int J Sport Nutr Exerc Metab. (2001) 11:451–65. doi: 10.1123/ijsnem.11.4.451
177. Coviello AD, Zhuang WV, Lunetta KL, Bhasin S, Ulloor J, Zhang A, et al. Circulating testosterone and SHBG concentrations are heritable in women: the Framingham heart study. J Clin Endocrinol Metab. (2011) 96:E1491–5. doi: 10.1210/jc.2011-0050
178. Harris JA, Vernon PA, Boomsma DI. The heritability of testosterone: a study of Dutch adolescent twins and their parents. Behav Genet. (1998) 28:165–71. doi: 10.1023/A:1021466929053
179. Hong Y, Gagnon J, Rice T, Perusse L, Leon AS, Skinner JS, et al. Familial resemblance for free androgens and androgen glucuronides in sedentary black and white individuals: the HERITAGE family study. Health, risk factors, exercise training and genetics. J Endocrinol. (2001) 170:485–92. doi: 10.1677/joe.0.1700485
180. Shieh A, Greendale GA, Cauley JA, Srikanthan P, Karlamangla AS. Longitudinal associations of insulin resistance with change in bone mineral density in midlife women. JCI Insight. (2022) 7:e162085. doi: 10.1172/jci.insight.162085
181. Hamilton-Fairley D, Taylor A. Anovulation. Br Med J. (2003) 327:546–9. doi: 10.1136/bmj.327.7414.546
182. Hull MG. Epidemiology of infertility and polycystic ovarian disease: endocrinological and demographic studies. Gynecol Endocrinol. (1987) 1:235–45. doi: 10.3109/09513598709023610
183. Green B, Weiss N, Daling J. Risk of ovulatory infertility in relation to body weight. Fertil Steril. (1988) 50:721–6. doi: 10.1016/S0015-0282(16)60305-9
184. Grodstein F, Goldman M, Cramer D. Body mass index and ovulatory infertility. Epidemiology. (1994) 5:247–50. doi: 10.1097/00001648-199403000-00016
185. Rich-Edwards J, Goldman MB, Willett W, Hunter DJ, Stampfer MJ, Colditz GA, et al. Adolescent body mass index and infertility caused by ovulatory disorder. Am J Obstet Gynecol. (1994) 171:171–7. doi: 10.1016/0002-9378(94)90465-0
186. Prentice AM. Starvation in humans: evolutionary background and contemporary implications. Mech Ageing Dev. (2005) 126:976–81. doi: 10.1016/j.mad.2005.03.018
187. Roth J, Sahota N, Patel P, Mehdi SF, Wiese M, Mahboob HB, et al. Obesity paradox, obesity orthodox, and the metabolic syndrome: an approach to unity. Mol Med. (2016) 22:873–85. doi: 10.2119/molmed.2016.00211
188. Speakman JR. The evolution of body fatness: trading off disease and predation risk. J Exp Biol. (2018) 221:jeb167254. doi: 10.1242/jeb.167254
189. Carroll J, Saxena R, Welt CK. Environmental and genetic factors influence age at menarche in women with polycystic ovary syndrome. J Pediatric Endocrinol Metab. (2012) 25:459–66. doi: 10.1515/jpem-2012-0047
190. Sadrzadeh S, Klip W, Broekmans F, Schats R, Willemsen WNP, Burger CW, et al. Birth weight and age at menarche in patients with polycystic ovary syndrome or diminished ovarian reserve, in a retrospective cohort. Hum Reprod. (2003) 18:2225–30. doi: 10.1093/humrep/deg409
191. Welt CK, Carmina E. Lifecycle of polycystic ovary syndrome (PCOS): from in utero to menopause. J Clin Endocrinol Metab. (2013) 98:4629–38. doi: 10.1210/jc.2013-2375
192. Forslund M, Landin-Wilhelmsen K, Schmidt J, Brännström M, Trimpou P, Dahlgren E. Higher menopausal age but no differences in parity in women with polycystic ovary syndrome compared with controls. Acta Obstet Gynecol Scand. (2019) 98:320–6. doi: 10.1111/aogs.13489
193. Minooee S, Tehrani FR, Rahmati M, Mansournia MA, Azizi F. Prediction of age at menopause in women with polycystic ovary syndrome. Climacteric. (2018) 21:29–34. doi: 10.1080/13697137.2017.1392501
194. Tehrani FR, Solaymani-Dodaran M, Hedayati M, Azizi F. Is polycystic ovary syndrome an exception for reproductive aging? Hum Reprod. (2010) 25:1775–81. doi: 10.1093/humrep/deq088
195. Sear R. Height and reproductive success: is bigger always better? In: Frey U, Stoermer C, Willfuehr K, editors. Homo Novus: A Human Without Illusions. Springer (2010). p. 127–43. ISBN: 9783642121418.
196. Shadyab A, Macera C, Shaffer R, Jain S, Gallo LC, Gass MLS, et al. Ages at menarche and menopause and reproductive lifespan as predictors of exceptional longevity in women: the women’s health initiative. Menopause. (2017) 24:35–44. doi: 10.1097/GME.0000000000000710
197. Zhang X, Liu L, Song F, Song Y, Dai H. Ages at menarche and menopause, and mortality among postmenopausal women. Maturitas. (2019) 130:50–6. doi: 10.1016/j.maturitas.2019.10.009
198. Tamakoshi K, Yatsuya H, Tamakoshi A. Early age at menarche associated with increased all-cause mortality. Eur J Epidemiol. (2011) 26:771–8. doi: 10.1007/s10654-011-9623-0
199. Pasquali R, Antenucci D, Casimirri F, Venturoli S, Paradisi R, Fabbri R, et al. Clinical and hormonal characteristics of obese amenorrheic hyperandrogenic women before and after weight loss. J Clin Endocrinol Metab. (1989) 68:173–9. doi: 10.1210/jcem-68-1-173
200. Kiddy D, Hamilton-Fairly D, Bush A, Short F, Anyaoku V, Reed MJ, et al. Improvement in endocrine and ovarian function during dietary treatment of obese women with polycystic ovary syndrome. Clin Endocrinol. (1992) 36:105–11. doi: 10.1111/j.1365-2265.1992.tb02909.x
201. Clark AM, Thornley B, Tomlinson L, Galletley C, Norman RJ. Weight loss in obese infertile women results in improvement in reproductive outcome for all forms of fertility treatment. Hum Reprod. (1998) 13:1502–5. doi: 10.1093/humrep/13.6.1502
202. Wright P, Corbett C, Pinto B, Dawson RM, Wirth MD. The impact of exercise perceptions and depressive symptoms of polycystic ovary syndrome-specific health-related quality of life. Womens Health (Lond). (2021) 17:17455065211065865. doi: 10.1177/17455065211065865
203. Kite C, Parkes E, Taylor S, Davies RW, Lagojda L, Brown JE, et al. Time to load up—resistance training can improve the health of women with polycystic ovary syndrome (PCOS): a scoping review. Med Sci (Basel). (2022) 10:53. doi: 10.3390/medsci10040053
Keywords: polycystic ovary syndrome, evolution, testosterone, strength, muscularity, dominance
Citation: Bushell A and Crespi BJ (2024) The evolutionary basis of elevated testosterone in women with polycystic ovary syndrome: an overview of systematic reviews of the evidence. Front. Reprod. Health 6:1475132. doi: 10.3389/frph.2024.1475132
Received: 2 August 2024; Accepted: 12 September 2024;
Published: 30 September 2024.
Edited by:
Laura Buggio, IRCCS Ca 'Granda Foundation Maggiore Policlinico Hospital, ItalyReviewed by:
Jim Parker, University of Wollongong, AustraliaPaola Vigano', IRCCS Ca 'Granda Foundation Maggiore Policlinico Hospital, Italy
Copyright: © 2024 Bushell and Crespi. This is an open-access article distributed under the terms of the Creative Commons Attribution License (CC BY). The use, distribution or reproduction in other forums is permitted, provided the original author(s) and the copyright owner(s) are credited and that the original publication in this journal is cited, in accordance with accepted academic practice. No use, distribution or reproduction is permitted which does not comply with these terms.
*Correspondence: Aiden Bushell, YWlkZW5fYnVzaGVsbEBzZnUuY2E=