- 1Department of Physiology, Ladoke Akintola University of Technology, Ogbomoso, Nigeria
- 2Reproductive Biology and Toxicology Research Laboratories, Oasis of Grace Hospital, Osogbo, Nigeria
- 3Department of Oral Biology and Biomedical Sciences, Faculty of Dentistry, MAHSA University, Jenjarom, Malaysia
- 4Brainwill Laboratories, Osogbo, Nigeria
- 5Department of Physiology, Faculty of Medicine, Biosciences and Nursing, MAHSA University, Jenjarom, Malaysia
- 6Redox Biology Group, Discipline of Pathology, Faculty of Medicine and Health, Charles Perkins Centre, The University of Sydney, Sydney, NSW, Australia
Viral infections have been a part of human existence to date, though viruses have posed a huge threat with various outbreaks lately. These threats are associated with reproductive health challenges, especially male infertility. The prime focus of this review is to highlight the mechanisms associated with viral infection-induced male infertility/subfertility and identify new treatment strategies with the aim to preserve male fertility. The reviewed data showed that viral infections stimulate inflammatory responses, resulting in the release of proinflammatory cytokines, which induces oxidative stress. This oxido-inflammatory cycle could continue in a vicious cycle and threaten male fertility. Existing data from human and experimental studies show that viral infection-induced oxido-inflammatory response results in testicular damage, atrophy of the seminiferous tubules and Sertoli cells, and reduced Leydig cell mass. This is accompanied by reduced circulatory testosterone, impaired spermatogenesis, reduced sperm motility, lipid peroxidation, DNA fragmentation and apoptosis of the sperm cells. Based on the available pieces of evidence, antioxidant therapy, in vivo and in vitro, may be beneficial and protects against the potential risk of male infertility from viral infection. It is, however recommended that more clinical studies be conducted to demonstrate the possible protective roles of antioxidants used as adjuvant therapy in viral infections, and in the in vitro treatment of semen samples for those utilizing semen washing and artificial reproductive techniques.
Introduction
Infectious diseases have been inflicting humans from time immemorial. This has worsened in the last four decades with various viral outbreaks. The most mention-worthy viruses include the Human Immunodeficiency Virus (HIV) that emerged in 1981, Severe Acute Respiratory Syndrome Coronavirus (SARS-CoV) that emerged in 2002, outbreaks of influenza A virus subtype H1N1 (A/H1N1) H1N1 strains (1918 flu pandemic, the 1977 Russian flu pandemic as well as the 2009 swine flu pandemic), Middle East Respiratory Syndrome Coronavirus (MERS-CoV) (caused epidemic in 2012), and Ebola virus (outbreak in 2013) and the Severe Acute Respiratory Syndrome Coronavirus-2 (SARS-CoV-2) causing Coronavirus disease-2019 (COVID 19) that emerged in 2019 and is still on-going (1–3). The emergence of infectious diseases has a direct influence on human health outcomes, ultimately impacting sustainable development (4). By the end of the twentieth century, an estimated 34.3 million individuals globally were infected with HIV/AIDS (5). Millions of children were orphaned due to the epidemics, which also undermined social life and corroded civic order and economic progress (6). The SARS-CoV pandemic had deadly consequences, affecting 8,098 persons, resulting in 774 fatalities by February 2003 (7). However, in many of the afflicted areas, the outbreak revealed some flaws in hospitals and community control mechanisms (8). The 2009 H1N1 influenza pandemic had a global impact, affecting 214 nations and resulting in 18,449 fatalities. With earlier influenza epidemics posing a persistent threat, scientific communities were adequately equipped in terms of frame of mind and infrastructure, allowing for faster and more thorough researches on the basic features of the disease, as well as implications for its regulations and learnings to deal with future epidemics (9). MERS-CoV, causing another coronavirus epidemic, has one of the highest case fatality rates among previous pandemics (43% in 147 individuals) (10). More recently, between 2013 and 2016, the Ebola virus posed a major public health threat in the afflicted nations, with significant social and economic consequences (11). As the new decade begins, the world is struggling to contain another pandemic-strength virus, the SARS-CoV-2 causing COVID 19. It is one of the most serious public health crises in human history. The virus was originally discovered in December 2019 and isolated from a group of pneumonia-stricken employees at the Wuhan seafood market in China (12–14). Shortly later, on March 11, 2020, the World Health Organization (WHO) proclaimed it a worldwide pandemic (15).
Viral infections lead to a multitude of health issues which mandates understanding the impact of these viruses on the vital physiological processes. Reports on the association of viral infection on female reproductive functions are profound, but the mechanism by which these viruses affect male fertility still awaits in-depth explanation. Infection is considered to be a contributing factor in male infertility. Certain infections, such as genital tract infections, have been proven to have a detrimental impact on sperm quality (16). Microbial infections and the subsequent inflammatory responses may disrupt the endogenous environment of the male reproductive system resulting in inflammation of the male reproductive organs. Reproductive tract infections-induced immunogenic dysregulations are responsible for about 15% of male infertility cases (17, 18). Activating the innate immune system, which typically disturbs the testis-specific restrictive immunological responses and leads to leukocyte infiltration and elevation of proinflammatory mediators, is a testicular strategy for fighting viral infections (19, 20). The inflammatory reactions may cause oxidative stress (OS), which is considered to be the central mechanism that elicits oxidative damage in the male reproductive tissues, affects every testicular cell and also lead to the disintegration of the physical barriers of the testes (17–23). These intricate processes of testicular responses to viral infections are still evolving. Thus, the present review aims to provide a comprehensive understanding of the mechanisms by which viral infections affect male reproduction and how intricately OS mediates the infection-induced male reproductive tissue disruptions.
Influenza Virus and Male Fertility
Direct viral invasion or secondary inflammatory pathways are two ways viral infections can influence both male and female reproductive processes. The effects of H1N1 on the quality of human sperm have been confirmed by a large body of evidence (24, 25). The flu can have long-lasting effects on sperm DNA integrity, and it can cause the transitory discharge of defective sperm. It has even been linked to an increased risk of infertility in some studies (24, 26).
Sergerie et al. (26) revealed that a febrile episode could negatively impact on sperm parameters and DNA integrity. Evenson et al. (24) investigated the characteristics of human sperm chromatin structure following a bout of influenza and a high fever and discovered that influenza might have latent effects on sperm chromatin structure, resulting in the transitory release of aberrant sperm. Several studies using animal models demonstrate that influenza can damage sperm quality and result in infertility (25). Devi et al. (27) showed in a mouse model that the human influenza virus can cause spermatozoa to develop chromosomal aberrations. While data suggests that a local inflammatory reaction is unlikely, the temporary sperm abnormalities seen are most likely the result of a combination of systemic fever and direct DNA damage, resulting in apoptosis and a sharp decline in fertility (28, 29).
As a result, solid evidence supporting the influence of influenza infection on sperm quality may be drawn. It is fascinating to consider whether influenza can cause future infertility. On this subject, data are scarce. Evenson et al. (24) propose that influenza has a latent effect on sperm, which could result in infertility. To sum up, more research on the link between influenza and infertility is still needed.
Zika Virus and Male Fertility
Studies have revealed that ZIKV thrives in the male genital tract. The virus is expressed in the semen of symptomatic Zika-infected men (30), and the ZIKV RNA has been reported to persist longer with a higher concentration in the semen than in the blood (31). Mansuy et al. (32) reported that the ZIKV RNA could be detected in the semen till the 188th day following infection. Also, it has been revealed that the clearance time is 25–83 days in the semen, while it is 5–15 days in the circulation (30, 33–35). Hence, it could be inferred that the male urogenital tract serves as a reservoir for this virus. The testes seem to be the organ of choice for ZIKV replication because they are capable of sustaining high viral load for a prolonged period (36–42). Although most of the ZIKV RNA in the semen does not have an infectious virus, implying that they loss their infectivity; the presence of the virus in the male urogenital tract remains a threat to male fertility as the length of days the virus will retain its infectivity in the male urogenital tract is yet unclear.
ZIKV can cause orchitis, epididymo-orchitis, and testicular atrophy, leading to infertility and hypogonadism in experimental animals (40, 43). Although the circulatory level of testosterone is unaltered during the acute phase infection (44), ZIKV decreases sperm count and increases abnormalities in sperm morphology up to 90 days post-infection (44). In a prospective follow-up study in Brazil, Avelino-Silva et al. (45) demonstrated that ZIKV infection led to a long-term detrimental effect on human male fertility evident by abnormal spermogram at 12 months post-infection. An earlier study by Govero et al. (43) in mouse-model showed that the initial ZIKV infection-induced damage was observed 2 weeks after infection, with germ cell loss and impairment of Sertoli cell function evident by reduced concentrations of circulatory inhibin B. With persistent infection, most germ cells were lost, and spermatogenesis was almost utterly lost on day 21. Govero et al. (43) also demonstrated significant expression of ZIKV in epididymal sperm cells seven days post-infection. This suggests that ZIKV may distort spermatogenesis and also cause direct damage to the spermatozoa and testis (46). The role of the Sertoli cells in maintaining immune privilege has been proposed to be beyond a simple barrier function but possibly dependent on secreted and surface immunosuppressive and immunoregulatory factors (47). During infection, these protective factors that maintain an anti-inflammatory immune environment in a physiological state may render the germ cells susceptible to microbial/viral attack.
Mumps Virus and Male Fertility
The hallmark of Mumps infection is bilateral swelling of the parotid gland (parotitis). Other than parotitis, inflammation of the testis (orchitis), usually unilateral, is the most common manifestation of Mumps, and affects 10–20% of adult men (48). This can negatively impact male fertility. Testicular swelling and tenderness, and scrotal inflammation are typical of Mumps orchitis. In many cases, inflammation of the epididymis (epididymitis), particularly the head region, is associated with orchitis, a condition usually termed epididymo-orchitis (49, 50). This implies that the infection does not only negatively impact testicular function, but also sperm function, when the viruses are released into the epididymis. The acute orchitis can resolve in 2 weeks but can significantly impair testicular function, including testicular atrophy reported in nearly half of the cases. Sperm count, motility and morphology have been shown to remain drastically affected even years after recovery, indicating that the virus impacts testicular and sperm functions (48).
Humans are the only natural reservoir of Mumps virus replication, and studies on viral pathogenesis are scarce. Differential response of testicular cells to viral replication is reported. For example, a mouse study showed that the virus can infect Leydig cells, Sertoli cells, germ cells and resident testicular macrophages (51). However, the viral replication was higher in Sertoli cells compared to Leydig cells and testicular macrophages, whereas germ cells were resistant to replication, indicating that testicular cells have a variable innate response to Mumps virus infection (51).
The acute inflammation in orchitis can lead to the release of inflammatory cytokines, which can impair organ function through oxidative stress-mediated pathways, resulting in disruption of testicular function. Increased levels of TNF-α were associated with Mumps and concomitant reduction in the testosterone synthesis by Leydig cells (52), possibly through inhibiting the functions of enzymes involved in testosterone synthesis. Further, pathological levels of TNF-α can disrupt the blood-testes-barrier (BTB) by impacting the levels of junctional complexes occludens and zonula occludens 1 as the primary target of TNF-α (53). The development of autoantibodies against GCs during orchitis is common and could result from disrupted BTB leading to infertility in young adults where spermatogenesis is active compared to children when it is inactive (54).
In summary, Mumps can have a drastic impact that may involve germ cell apoptosis, reduced sperm count, motility, morphology and testicular atrophy, all leading to male infertility. Therefore, vaccination is the solution to these infections. Further, due to limited data on pathogenesis studies of Mumps associated orchitis, experimental animal studies are critical.
HIV and Male Fertility
The virus has two subtypes; HIV-1 and HIV-2, which are primarily transmitted via sexual intercourse, vertical transmission, sharing of sharps and blood and blood products (55–57). HIV-1 is expressed in the semen of asymptomatic HIV-infected men as free HIV-1 RNA particles in the seminal plasma and as a cell-associated virus in non-spermatozoal cells like the lymphocytes and macrophages (58). Most HIV-1 RNA likely originates from the seminal vesicles and prostate since the concentration of HIV-1 RNA in the semen is not altered by vasectomy (59). Intermittent shedding of HIV-1 RNA is the commonest pattern of HIV-1 expressed in semen (60, 61). This is partly due to the varying composition of the ejaculate between men, and overtime in the same individual. The rise in HIV-1 RNA levels in semen independent of the circulatory levels of HIV-1 RNA levels following local inflammation also explains this phenomenon. Intermittent shedding may lead to discrepancies between circulatory and seminal plasma concentrations of HIV-1 RNA.
Although in rare cases, HIV-infected patients have been reported to have high semen viral load, usually, HIV load is lower in the seminal fluid than in the blood (62, 63). HIV has the propensity to integrate its DNA into the host genome. Studies have shown that HIV leads to chronic orchitis, resulting in hypergonadotropic hypogonadism with impaired steroidogenesis (64). It has also been revealed to cause oligozoospermia and teratozoospermia, usually secondary to HIV-induced hypogonadism and leukocytospermia (65). In addition, HIV triggers Sertoli-cell-only syndrome, germ cell damage and peritubular fibrosis. Studies have demonstrated that HIV reduces sperm motility and the percentage of spermatozoa with normal morphology (66, 67). Similarly, van Leeuwen et al. (68) reported a decrease in semen volume and sperm motility in multiple semen samples obtained before and after seroconversion for HIV-1 from a single donor. AIDS patients have been reported to have low circulatory testosterone and high levels of LH and FSH. The reduced testosterone level may be due to the fibrosis of the testicular interstitial tissue and reduced Leydig cell number (69). The HIV-associated rise in circulatory cytokines have also been reported to contribute to depressed testosterone synthesis (70).
Despite the effectiveness of highly active antiretroviral therapy (HAART) in the management of HIV/AIDS, it has also been implicated in infertility. Akhigbe et al. (71) demonstrated that HAART impaired male sexual acts via a testosterone-dependent mechanism and hyperprolactinemia. Administration of HAART has also been revealed to induce extensive atrophy of the seminiferous tubules and hypocellularity. Furthermore, HAART impairs spermatogenesis and steroidogenesis, resulting in reduced serum testosterone, semen volume and sperm motility (72, 73). In an attempt to evaluate the associated mechanism of HAART-induced male reproductive toxicity using a rat model, Akhigbe et al. (74) observed that HAART led to lipid peroxidation and inflammation of the testis. This was associated with reduced testicular and sperm DNA integrity and dysregulation of lactate transport and glutathione content.
Herpes Simplex Virus and Male Fertility
The transmission of Human Papilloma virus (HPV), for the most part, is by way of the sexual itinerary (75). The genital passage is infected by a relative amount of 30–40 varieties of HPV from the α-genus (76, 77). It is supposed that a greater part of men who engage in sexual activity tend to obtain genital HPV infection during their lifetime, although around 90% of these infections are not clinically significant, and a better part of the infection tend to resolve impulsively (78). HSV-1 and HSV-2 can be transmitted sexually. HSV, especially HSV-2, has been detected in the semen (79) and could likely be internalized into the sperm cells. HSV infects the penile mucosa and triggers a chronic infection (80). The virus replicates in the keratinocytes and then spreads to the nerve cells, where it rests in the latency phase. HSV returns to the epithelial surface upon reactivation, inducing cell death and vascular lesions (81). Although the seminiferous tubules seem to be spared due to the protection conferred by the blood-testicular barrier, HSV-2 infect almost all tissues/organs of the male genital tract (82, 83). The prevalence of HSV shedding in seminal fluid ranges between 0 and 100% in chronic infection (84–88).
Findings reporting the presence of HSV DNA in oligozoospermic samples suggest that HSV may cause infertility/subfertility. Although studies confirming HSV-induced infertility are rare, HSV has been associated with low sperm count and reduced sperm motility (89, 90). The virus's ability to infect almost all organs of the male reproductive tract could infer that it has the propensity to cause direct damage to the spermatozoa and alter the sperm quality (such as reducing sperm count, motility and sperm cells with normal morphology). During ART, HSV can be transmitted vertically through the sperm cells, thus increasing the risk of miscarriage and adverse effects to the fetus (91).
Human Papillomavirus and Male Fertility
The infection of seminal fluid is of great medical importance in the etiology of male infertility, which in most cases is not unconnected with defective semen quality (92, 93). Several studies have documented the transmission of HPV through sexual intercourse in males. It has been reported that about 16% of semen from men seeking fertility assessment and, or medical care had HPV in their semen and a 10% prevalence among the population as a whole (94).
In addition, HPV infection has been implicated as a worthy risk factor for infertility in men (95, 96). Though the functionality of the process that implicates HPV seminal infection with male infertility is shrouded in uncertainty (95), it has been proven that HPV infection considerably causes a reduction in essential sperm indices (96). A good indicator of sperm motility that has been generally accepted is sperm progressive motility which is perceived as a basic functional parameter needed for fertilization (97). A good number of researches has shown that HPV infection is implicated in male infertility with a reduction in the progressive motility of the sperm (98–106). On the contrary, Zheng et al. (107) established that there was no clear dissimilarity of sperm progressive motility proportion amidst infected and non-infected infertile males. The systematic review and meta-analysis explored the likely impingement of HPV infection in semen on the progressive motility of sperm cells in infertile males; however, the evidence is far from conclusive because of the sample sizes and existing confounding factors of the currently available studies.
Hepatitis Virus and Male Fertility
The prevalence of viral shedding in the seminal fluid is about 68% in chronic infections (108). Similarly, the prevalence of HCV in seminal fluid varies from 29 to 39% in the early-stage to 32–46% in chronic infections (109–113). Some studies documented the presence of HBV-DNA in the semen 120 days after it was no longer seen in the serum (114). Huang et al. (115) observed that HBV-infected patients have higher sperm chromosomal aberrations than the controls. Transfection of sperm cells with HBV has been revealed to induce apoptosis and decline fertilization capacity (116). Data on the impact of HCV on semen quality is conflicting. A study reported that HCV-infected semen does not alter semen quality, importing that HCV is likely not to exert a negative impact on sperm cells (117). Interestingly, other studies demonstrated that HCV reduced sperm count and motility and increased abnormal sperm morphology (118, 119). In addition, HCV has been reported to reduce circulatory testosterone and inhibin B (118–123). A recent study revealed that oxidative stress is implicated in the pathogenesis of HBV-induced sperm damage. HBV enhances reactive oxygen species (ROS) generation, resulting in oxidative damage of the spermatozoa and increased sperm DNA fragmentation and chromosome mutations (124).
Parvovirus and Male Fertility
The discovery of AAV vector DNA in the semen of patients enrolled in a gene therapy trial for hemophilia B based on the intramuscular delivery of AAV vectors carrying the factor IX gene suggested for the first time that semen infection could occur after delivery of engineered AAV vectors for gene therapy (125). This raised concerns on the danger of germline transduction and germline transmission risk associated with AAV vectors were investigated in different male laboratory animal models (126). Indeed, the seminal dissemination of the vector was greater with intravascular delivery than when it was injected intramuscularly. The sequences of the AAV vector were transiently detected, and the detection depended on the dose and time (127–129). With extensive follow-up and observation of numerous spermatogenic cycles, it was suggested that these vectors did not appear in recurrence in rabbit semen and thus bear low possibility of germline transmission for humans as well (127–129). It was also reported that in vasectomized rabbits, even in the absence of germ cells, the viral vector could contaminate the semen (128). This may indicate that the viral component may be transmitted via the seminal plasma alone, irrespective of the sperm concentration. Moreover, the viral vectors transduction directly into isolated murine spermatogonia or mature sperm could be done successfully (127), while transduction of the same in murine male germline stem cells was successful (130). Another study demonstrated that following either intraperitoneal AAV vector administration to mouse neonate or intravascular administration into mouse fetus, the viral components were persistently found in those animal gonads for over a year of follow-up. At the same time, viral components were absent in the isolated sperm DNA of these mice (131). Taken together, these findings suggest a low risk of accidental germline transmission of AAV, but precautions are needed when these viral vectors are employed for gene delivery (126). Nevertheless, in the semen of infertile men, the AAV DNA have been detected more frequent than in normal semen samples (20–40 per cent vs. 0–5 per cent, respectively) (132–134). Studies showed an association of AAV infection with oligoasthenozoospermia and oligospermia but found that the presence of AAV DNA in semen was not significantly related to semen quality, including the functional capacity of sperm (132–134).
The suggested significances of parvovirus infection in the male reproductive tract or semen include sexual transmission of infection; subfertility or infertility owing to dysfunctions of the testicular cells; viral genome incorporation into the germ cell genome and thereby transmission to female partners and infection of ova and subsequently of the embryo, which may cause miscarriage, embryonic abnormalities and also a risk of infection transmission with assisted reproduction (135). These data show that AAV infection of the male reproductive tract occurs rather often. The presence of AAV in ejaculates supports the theory that the virus is transmitted sexually, and the preference for viral DNA identification in aberrant semen samples from infertile men suggests that AAV may play a role in male infertility (135). Furthermore, as AAV DNA was found in testis biopsies in 26% of infertile (azoospermic) men (132), it is tempting to hypothesize that AAV might disrupt sperm cell development, resulting in infertile spermatozoa.
The mechanism associated with the entry of parvoviruses into the male reproductive tract, susceptibility of the testicular cells and the impact of the virus on reproductive endocrine regulations is an essential area subject to future in-depth research. In this aspect, knowledge of whether parvovirus infection affects male reproductive tissues via an OS-mediated pathway will help to understand the underlying pathogenesis better. The viral kinetics also needs to be elucidated as to know how the virus replicates in the male reproductive tract and whether the testis serves as a safe hide-out reservoir for the virus, as well as the testicular antiviral defense mechanisms against this virus.
Ebola Virus and Male Fertility
Semen samples collected from recovering survivors of the Ebola virus revealed its presence in the semen. In Sierra Leone, a study conducted on 93 survivors showed that semen specimens of all men (n = 9) were positive for virus 2–3 months after the disease onset although the virus was undetectable in circulating blood. Likewise, 26 of 40 semen samples and 11 of 43 were positive when tested after 4–6 and 7–9 months, respectively, post-disease onset (136). These findings show that even virus is undetected in peripheral blood smears, it stays in semen for months and can be a potential source of transmission to sexual partners. For example, a female acquired the Ebola virus through sexual transition from her partner whose semen tested positive for the virus even after 199 days after the disease onset (137). The Ebola virus has been detected in the infected man even >900 days post-infection, which triggers an alarm on its long-term sexual transmission (138). These results were echoed in another study on 277 survivors where 93% of men semen specimens were positive after 3 months, and the virus was still detectable in the semen of 0.06% of the cases after 2 years of the onset of infection (139).
The virus has been detected in the testicular interstitium, seminal vesicles, and prostate thus can have direct consequences not only on the testes but accessory sex glands (140). Like the Mumps virus, the Ebola virus was also shown to be associated more with Sertoli cells and BTS disruption, which has dire consequences on endocrine and spermatogenesis functions of the testes (141). Survivors of Ebola virus infection have reported complaints of erectile dysfunction (5–8%) and reduced sexual desire (10–12%), probably due to compromised endocrine function of the testes (142, 143). However, the causal relation of reproductive dysfunction to viral infections remains elusive. Nonetheless, significant production of a suite of inflammatory cytokines, including TNF-α, has been reported in response to Ebola infection. This points toward the role of inflammation-induced oxidative stress on cell and organ functions (144).
Taken together, the available literature indicates that the Ebola virus has a strong potential for sexual transmission for several months, and the virus localizes in Sertoli cells and other parts of the reproductive tract and can impact testicular function. The high mortality of this disease and scarce data on sperm parameters is a limitation and warrants further longitudinal studies on laboratory animals, including rodents and non-human primates.
Coronavirus and Male Fertility
Since the SARS-CoV epidemic in 2002, researchers have been reporting the possibility for coronaviruses to infect the human reproductive system (145, 146). Although studies are ongoing to understand the pathogenesis of this novel viral infection better, it has been established to be transmitted through inhalation of infected droplets and contact with infected persons, whether they are symptomatic or not (14). Coronavirus enters the human cells through endocytosis and translocation by binding to angiotensin-converting enzyme 2 (ACE 2), which primarily counterbalances ACE (147, 148). In the cell, it replicates, increases the pH of endosomes and lysosomes and activates p38 mitogen-activated protein kinases (MAPK) and extracellular-regulated protein kinases (ERK), which triggers a hyper-inflammatory response (149, 150).
When it comes to affecting reproductive functions, it has been hypothesized that SARS-CoV-2 may also activate MAPK/ERK signaling. Men have a larger expression of the ACE2 receptor than women do, which may explain why male reproductive functions appear to be farther vulnerable to the effects of SARS-CoV infection (146). It is known that sperm cells, Leydig cells, and Sertoli cells have significant levels of ACE2 receptor expression (150). However, according to certain investigations, the SARS-CoV virus and SARS-CoV-2 virus was not found in the semen samples of infected men (151, 152). The fact that SARS-CoV-2 virus particles were found in semen (153) raises questions about the validity of this claim. When regional or systemic inflammation occurs, the virus may succeed to get access to the germ cells owing to the compromised blood-testis barrier (153, 154). It is reported that serum luteinizing hormone (LH) in COVID-19 patients remain greater than in healthy men (150). On the contrary, these patients demonstrate significantly lower serum testosterone (150), and a reduced testosterone to LH and follicle-stimulating hormone (FSH) to LH ratio. These indicate that SARS-CoV-2 may have a direct impact upon testicular tissue rather than affecting the hypothalamus-pituitary-gonadal (HPG) axis (155), possibly via oxidative-sensitive MAPK/ERK-mediated hyper-inflammatory response.
Numerous other indirect impacts, for example, germ cell destruction and testicular dysfunction, resulting from a prolonged increase in body temperature consequent to the viral infection, have also been described. In some situations, hyperplasia of Leydig cells has been reported in patients who have been infected with the virus (153, 156). Some of the most noticeable symptoms of possible inflammatory responses resulting from SARS-CoV infection include disruption of functions of Leydig cell, thereby lower testosterone synthesis, and damage to the seminiferous epithelium causing disintegrated blood-testis barrier (146).
Studies have revealed that coronaviruses involved with previous epidemics are connected with orchitis, among which SARS-CoV is a prominent instance. This may result in the interruption of spermatogenesis and germ cell death, consequently reducing the quality of sperm produced (146). In testicular tissues, immunohistochemistry has verified the presence of IgG deposition; nevertheless, no viral genomic components were seen in either the testicular tissue or the seminal plasma. Inflammatory responses may have significant roles in the development of virus-mediated testicular injury and the generation of oxidative stress, as indicated by this finding (22). Furthermore, psychological stress is a powerful inducer of endogenous oxidative stress, and an abundance of evidence suggests that oxidative stress is associated with high-anxiety-related behavior, such as depression and post-traumatic-stress-disorder; however, the actual causal-effect association is yet to be known (157, 158). SARS-CoV-2, like SARS-CoV, is thought to employ a stress-evasion strategy that involves using amino acid sequences that mimic the adrenocorticotropic hormone (ACTH) of the host and, as a result, generate antibodies against these self ACTH molecules. This process subdues the stress response in the host, which is normally achieved by increasing cortisol levels, thereby reducing stress and inflammation in the organism (21, 159). As a result, uncontrolled inflammation continues to have a negative impact on the organs. The oxidative damage at the micro-level leads to membrane lipid peroxidation and sperm DNA fragmentation, both of which have a deleterious impact on testicular processes such as spermatogenesis and spermiogenesis. Male infertility is also associated with a decrease in sperm count and seminal volume, which can have a negative impact on reproductive outcomes and ultimately lead to infertility in males (160). Women infected with SARS-CoV are also more likely to have a disordered sexual function as a result of stress, which can have a poor influence on oocyte quality, menstrual cycle, and fertility (161).
Mechanism of Virus-Mediated Male Reproductive Disruption
Virus-mediated male reproductive dysfunction has been associated with oxido-inflammatory damage (Table 1; Figures 1, 2). Viruses trigger inflammatory responses, resulting in urethritis (inflammation of the urethra), prostatitis (inflammation of the prostate), epididymitis (inflammation of the epididymis), and orchitis (inflammation of the testis). These viruses activate the innate immune system, which causes leukocyte infiltration and upregulation of proinflammatory cytokines (19, 20). Although this inflammatory response mediates the innate ability to combat viral infections (19), it may also induce ROS generation and redox imbalance with consequent oxidative damage (12, 164). These expose the reproductive organs, especially the urethra, prostate and epididymis, to inflammatory and oxidative damage. The epididymal spermatozoa and sperm cells on transit during ejaculation are also exposed to the harmful effects of the cytokines and ROS, leading to reduced sperm count and motility and reduced sperm cells with normal morphology.
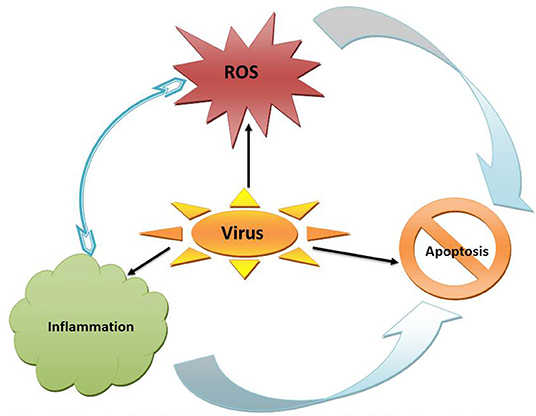
Figure 1. Enhanced generation of reactive oxygen species (ROS) could be a cause or consequence of inflammation; both of which can be triggered by viral infection and results in apoptosis.
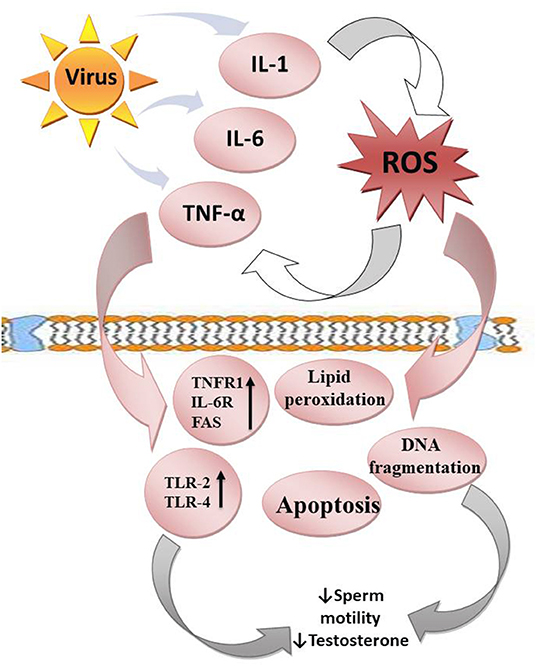
Figure 2. Viruses upregulate the release of proinflammatory cytokines (IL-1, IL-6, and TNF-α), which enhance ROS release. In response, ROS promote cytokine release. This leads to a vicious cycle. ROS attack the polyunsaturated fatty acids of the plasma membrane, resulting in lipid peroxidation. They also trigger DNA fragmentation and apoptosis of the testes and sperm cells. In addition, the cytokines upregulate TNFR1, IL-6R, FAS, TLR-2, and TLR-4, resulting in reduced sperm motility and steroidogenesis.
The impact of these cytokines (interleukin-1, IL-1, interleukin-6, IL-6, and tumor necrosis factor-α, TNF-α) on the testes depends on their environmental influences (20). At physiological levels, they may play homeostatic roles in the gap junctional communication of the blood-testis barrier (165) and enhance the integrity of the immune-privileged testis, thus maintain optimal spermatogenesis (166). However, when there is an upregulation of these proinflammatory cytokines, they mediate blood-testis barrier restructuring via deregulation of Cldn 11 in the Sertoli cells (167). Proinflammatory cytokines may also activate TNFR1, IL-6R, and FAS expressed in the germ cells, resulting in apoptosis of the germ cells (168). In addition, cytokines mediate male infertility by impairing sperm motility and repressing testosterone synthesis through the activation of Toll-like receptors-2 and 4 (TLR-2 and TLR-4) located on the human spermatozoa membrane (52, 169).
Furthermore, proinflammatory cytokines activate ROS-dependent oxidative stress, which could in turn further exaggerate inflammatory response, resulting in vicious cycle of oxido-inflammatory response (170). The high polyunsaturated fatty acids (PUFA) content of the plasma membranes of the testis and spermatozoa makes them readily prone to ROS-driven oxidation, resulting in lipid peroxidation (171), loss of testicular and sperm cell membrane integrity, and raised non-specific permeability to ions (172, 173). Following the destruction of the testicular and sperm cell membrane, the nuclear chromatin is exposed to oxidative damage, leading to base modification, DNA fragmentation, and testicular and sperm cell apoptosis (74, 174–176). Hence, viral infections could cause direct modification of semen quality, damage to testicular and sperm DNA integrity, and production of anti-sperm antibodies, which may alter steroidogenesis, sperm motility and sperm-oocyte binding (99, 120, 123, 177, 178), thus reducing fertilization capacity via an inflammation-driven oxidative stress-mediated pathway.
Fascinatingly, antiviral drugs, which have even been proven effective against viral infection, have been shown to impair male fertility. Ribavirin has been demonstrated to reversibly damage germ cells via impairment of cell growth through inhibition of inosine monophosphate dehydrogenase, which is necessary for DNA and RNA guanosine triphosphate biosynthesis (118, 179), with consequent apoptosis (121, 180). In addition, interferon α-2b, which is effective against mumps infection, has been reported to cause oligoasthenozoospermia (181, 182). Steroids have also been shown to suppress testosterone production (183). Efavirenz, lamivudine, stavudine, nevirapine, and tenofovir, which are effective against most viral infections, including HIV, have also been reported to impair testicular and sperm integrity and function. Antiviral drugs have been reported to induce atrophy of the seminiferous tubules (72, 184) and resultant distortion of spermatogenesis (74, 164). Studies have also revealed that antiviral drugs led to reduced testosterone levels consequent to reduced Leydig cell mass (72, 74). Antiviral therapy did not just distort steroidogenesis and spermatogenesis; it culminates in testicular and sperm cell DNA fragmentation (74, 185, 186), erectile dysfunction and reduced fertility indices (74, 164).
Management of Virus-Mediated Male Reproductive Disruption
In spite of the effectiveness of antiviral and antiretroviral drugs, and the improvement in the quality of life and life expectancy in people with viral infections, either acute or chronic, following antiviral therapy, the tendency of these drugs to impair male fertility remains a great concern. Assisted Reproductive Techniques (ART) using “sperm/semen washing” (SW) to reduce the seminal viral load provides a safety measure for serodiscordant men, especially those in their reproductive years and who wish to have children, with minimal risk of sexual and vertical transmission. Although Zamora et al. (187) reported a prevalence of 1.86% of retroviral positive semen after semen washing and recommended a post-wash retroviral screening on the semen before intracytoplasmic sperm injection (ICSI), semen washing and ICSI remains the current technique to reduce viral load. Also, it has been documented that currently, no semen processing techniques can eliminate seminal virus, especially ZIKV; the specific technique (intrauterine insemination, IUI, in vitro fertilization, IVF, or ICSI) used for medically assisted reproduction should be dependent on the cause of infertility (188).
Despite the available techniques in reducing viral load and preventing sexual and vertical transmission, two major challenges exist. First, achievement of sexual satisfaction. In addition, data on semen quality and sperm integrity in patients with viral infections after semen washing are scarce. Before semen washing, the viral infection might have induced sperm DNA fragmentation and apoptosis. Are these damages reversible even after sperm washing? Since semen washing and ART seems to be the current modality of achieving conception, especially in chronic viral infections, copulation may be a problem. However, safe the barrier method could be, scrotal transmission could pose another issue as in the case of HPV. Antioxidant therapy may be beneficial since oxidative stress has been implicated as a key player in viral infection-induced male infertility/subfertility. Although some studies have questioned the benefits of antioxidants in male infertility, inarguable pieces of evidence show that antioxidants alleviate oxidative stress-induced testicular and sperm damage. Vitamins (such as Vitamin C and E), carnitine, cysteine, and carotenoids have been shown to scavenge free radicals and act as energy sources (189–193). In addition to the free radical scavenging-ability of Coenzyme Q10 (CoQ10), it also serves as an intermediate in the mitochondrial transport (194), thus providing required energy. Folic acid preserves sperm DNA integrity (195), while selenium maintains redox balance and aids sperm motility (196). Zinc, on the other hand, maintains sperm chromatin stability (197). Furthermore, phytonutrients (such as flavonoids, polyphenols, and catechin compounds) scavenge free radicals and chelate metal ions, thus preserving sperm quality (198, 199). Arafa et al. (200) also demonstrated that in vivo antioxidant therapy (containing vitamins A, B6, B12, C, D3, E, K, thiamin, riboflavin, niacin, folate, biotin, pantothenic acid, iodine, zinc, selenium, copper, manganese, chromium, molybdenum, carnitine, arginine, CoQ10, cysteine, grapeseed extract, lycopene, and benfotiamine) for 3 months significantly improved conventional semen parameters, seminal oxidation-reduction potential and sperm DNA fragmentation. More so, Talevi et al. (201) showed that a combination of zinc, d-aspartate and CoQ10 in vitro treatment on human sperm enhanced sperm motility and reduced lipid peroxidation and sperm DNA fragmentation. The protective roles of antioxidant therapy on semen samples were also documented in a systemic review by Agarwal et al. (202). Hence, it is safe to state that using antioxidants as adjuvant therapy with appropriate antiviral/retroviral drugs may preserve testicular integrity and ensure optimal steroidogenesis and spermatogenesis, thus enhancing semen quality and sperm integrity and function. Also, treating semen samples with antioxidants before the appropriate medically assisted reproduction may enhance semen quality and sperm integrity and function.
Conclusion and Future Perspective
This study reviews the possible mechanisms associated with the viral threats to male fertility. It elucidates the role of inflammation-led oxidative stress in the pathogenesis of viral infection-induced male infertility. Though semen washing and ART is the available modality of achieving fertility and preventing vertical and horizontal transmission, especially in chronic cases of infection, the use of antioxidants as adjuvant therapy is proposed. However, it is recommended that more clinical studies should be conducted to demonstrate the protective roles of antioxidants used as adjuvant therapy in viral infections and in the in vitro treatment of semen samples for those utilizing semen washing and artificial reproductive techniques.
Author Contributions
RA and PS: conceptualization and design. RA, PS, and GA: methodology, resources, and supervision and validation. RA: project administration. RA, SD, PS, GA, MH, and AA: writing—original draft and writing—review and editing. All authors contributed to the article and approved the submitted version.
Conflict of Interest
The authors declare that the research was conducted in the absence of any commercial or financial relationships that could be construed as a potential conflict of interest.
Publisher's Note
All claims expressed in this article are solely those of the authors and do not necessarily represent those of their affiliated organizations, or those of the publisher, the editors and the reviewers. Any product that may be evaluated in this article, or claim that may be made by its manufacturer, is not guaranteed or endorsed by the publisher.
References
1. Bloom DE, Cadarette D. Infectious disease threats in the twenty-first century: strengthening the global response. Front Immunol. (2019) 10:549. doi: 10.3389/fimmu.2019.00549
2. Grubaugh ND, Ladner JT, Lemey P, Pybus OG, Rambaut A, Holmes EC, et al. Tracking virus outbreaks in the twenty-first century. Nat Microbiol. (2019) 4:10–19. doi: 10.1038/s41564-018-0296-2
3. Roychoudhury S, Das A, Sengupta P, Dutta S, Roychoudhury S, Choudhury AP, et al. Viral pandemics of the last four decades: pathophysiology, health impacts and perspectives. Int J Environ Res Public Health. (2020) 17:9411. doi: 10.3390/ijerph17249411
4. Di Marco M, Baker ML, Daszak P, De Barro P, Eskew EA, Godde CM, et al. Opinion: sustainable development must account for pandemic risk. Proc Natl Acad Sci. (2020) 117:3888–92. doi: 10.1073/pnas.2001655117
5. UNAIDS. Joint United Nations Programme on HIV/AIDS. Report on the global HIV/AIDS epidemic. UNAIDS (2020). Available online at: https://www.unaids.org/en/resources/fact-sheet (accessed January 19, 2022).
6. Gayle HD, Hill GL. Global impact of human immunodeficiency virus AIDS. Clin Microbiol Rev. (2001) 14:327–35. doi: 10.1128/CMR.14.2.327-335.2001
7. World Health Organization (WHO). Inadequate Plumbing Systems Likely Contributed to SARS Transmission. World Health Organization (2003). Available online at: https://www.who.int/news/item/26-09-2003-inadequate-plumbing-systems-likely-contributed-to-sars-transmission (accessed January 19, 2022).
8. Abdullah ASM, Tomlinson B, Thomas GN, Cockram CS. Impacts of SARS on health care systems and strategies for combating future outbreaks of emerging infectious diseases. In: Knobler S, Mahmoud AD, Lemon S, Mack A, Sivitz L, Oberholtzer K, editors. Learning from SARS: Preparing for the Next Disease Outbreak: Workshop Summary. Washington, DC: National Academies Press (2004). p. 83–90.
9. Cheng VC, To KK, Tse H, Hung IF, Yuen K-Y. Two years after pandemic influenza A/2009/H1N1: what have we learned? Clin Microbiol Rev. (2012) 25:223–63. doi: 10.1128/CMR.05012-11
10. World Health Organization (WHO). Middle East Respiratory Syndrome Coronavirus (MERS-CoV). World Health Organization (2013). Available online at: https://www.who.int/health-topics/middle-east-respiratory-syndrome-coronavirus-mers#tab=tab_1 (accessed January 19, 2022).
11. Calnan M, Gadsby EW, Kondé MK, Diallo A, Rossman JS. The response to and impact of the Ebola epidemic: towards an agenda for interdisciplinary research. Int J Health Policy Manag. (2018) 7:402. doi: 10.15171/ijhpm.2017.104
12. Akhigbe RE, Ajayi LO, Adelakun AA, Olorunnisola OS, Ajayi AF. Codeine-induced hepatic injury is via oxidao-inflammatory damage and caspase 3-mediated apoptosis. Mol Biol Rep. (2020) 47:9521–30. doi: 10.1007/s11033-020-05983-6
13. Guo Y-R, Cao Q-D, Hong Z-S, Tan Y-Y, Chen S-D, Jin H-J, et al. The origin, transmission and clinical therapies on coronavirus disease 2019 (COVID-19) outbreak–an update on the status. Military Med Res. (2020) 7:1–10. doi: 10.1186/s40779-020-00240-0
14. Ajayi AF, Akhigbe RE, Ram SK, et al. Management of COVID-19 among health care givers: an Afro-Asian perspective. Asian J Epidemiol. (2021) 14:11–21. doi: 10.3923/aje.2021.11.21
15. World Health Organization (WHO). Naming the Coronavirus Disease (COVID-19) and the Virus That Causes It. World Health Organization (2020). Available online at: https://www.who.int/emergencies/diseases/novel-coronavirus-2019/technical-guidance/naming-the-coronavirus-disease-(covid-2019)-and-the-virus-that-causes-it#:~:text=Human%20disease%20preparedness%20and%20response,virus%20on%2011%20February%202020 (accesssed January 19, 2022).
16. Pellati D, Mylonakis I, Bertoloni G, Fiore C, Andrisani A, Ambrosini G, et al. Genital tract infections and infertility. Eur J Obstet Gynecol Reprod Biol. (2008) 140:3–11. doi: 10.1016/j.ejogrb.2008.03.009
17. Sengupta P, Cho C. Pathophysiology of male infertility. In: Rizk B, Agarwal A, Sabanegh EJ, editors. Male Infertility in Reproductive Medicine: Diagnosis and Management. Baca Raton, FL: CRC Press (2019).
18. Sengupta P, Nwagha U, Dutta S, Krajewska-Kulak E, Izuka E. Evidence for decreasing sperm count in African population from 1965 to 2015. Afr Health Sci. (2017) 17:418–27. doi: 10.4314/ahs.v17i2.16
19. Jenabian M-A, Costiniuk CT, Mehraj V, Ghazawi FM, Fromentin R, Brousseau J, et al. Immune tolerance properties of the testicular tissue as a viral sanctuary site in ART-treated HIV-infected adults. Aids. (2016) 30:2777–86. doi: 10.1097/QAD.0000000000001282
20. Zhao S, Zhu W, Xue S, Han D. Testicular defense systems: immune privilege and innate immunity. Cell Mol Immunol. (2014) 11:428–37. doi: 10.1038/cmi.2014.38
21. Dutta S, Sengupta P. SARS-CoV-2 infection, oxidative stress and male reproductive hormones: can testicular-adrenal crosstalk be ruled-out? J Basic Clin Physiol Pharmacol. (2020) 31:20200205. doi: 10.1515/jbcpp-2020-0205
22. Dutta S, Sengupta P. SARS-CoV-2 and male infertility: possible multifaceted pathology. Reprod. Sci. (2021) 28:23–6. doi: 10.1007/s43032-020-00261-z
23. Sengupta P, Dutta S, Alahmar AT, D'souza UJA. Reproductive tract infection, inflammation and male infertility. Chem Biol Lett. (2020) 7:75–84.
24. Evenson DP, Jost LK, Corzett M, Balhorn R. Characteristics of human sperm chromatin structure following an episode of influenza and high fever: a case study. J Androl. (2000) 21:739–46.
25. Lugar DW, Ragland D, Stewart KR. Influenza outbreak causes reduction in semen quality of boars. J Swine Health Prod. (2017) 25:303–7.
26. Sergerie M, Mieusset R, Croute F, Daudin M, Bujan L. High risk of temporary alteration of semen parameters after recent acute febrile illness. Fertil Steril. (2007) 88:970.e1–7. doi: 10.1016/j.fertnstert.2006.12.045
27. Devi BY, Sharma G, Polasa H. Induction of chromosomal aberrations in mice spermatocytes by unpurified and purified human influenza viruses. Indian J Med Res. (1987) 86:506–10.
28. Le Tortorec A, Matusali G, Mahé D, Aubry F, Mazaud-Guittot S, Houzet L, et al. From ancient to emerging infections: the odyssey of viruses in the male genital tract. Physiol Rev. (2020) 100:1349–414. doi: 10.1152/physrev.00021.2019
29. Pilatz A, Kaiser R, Mankertz A, Schuppe H, Weidner W, Wagenlehner F. 139 Viral epididymitis and orchitis–myth or reality? Eur Urol Suppl. (2015) 14:e139. doi: 10.1016/S1569-9056(15)60141-5
30. Mead PS, Duggal NK, Hook SA, Delorey M, Fischer M, et al. Zika virus shedding in semen of symptomatic infected men. N Engl J Med. (2018) 378:1377–85. doi: 10.1056/NEJMoa1711038
31. Mansuy JM, Suberbielle E, Chapuy-Regaud S, Mengelle C, Bujan L, Marchou B, et al. Zika virus in semen and spermatozoa. Lancet Infect Dis. (2016) 16:1106–7. doi: 10.1016/S1473-3099(16)30336-X
32. Mansuy JM, Dutertre M, Mengelle C, Fourcade C, Marchou B, Delobel P, et al. Zika virus: high infectious viral load in semen, a new sexually transmitted pathogen? Lancet Infect Dis. (2016) 16:405. doi: 10.1016/S1473-3099(16)00138-9
33. Huits R, De Smet B, Arien KK, Van Esbroeck M, Bottieau E, et al. Zika virus in semen: a prospective cohort study of symptomatic travellers returning to Belgium. Bull World Health Organ;. (2017) 95:802–9. doi: 10.2471/BLT.17.181370
34. Barzon L, Percivalle E, Pacenti M, Rovida F, Zavattoni M, et al. Virus and antibody dynamics in travelers with acute Zika virus infection. Clin Infect Dis. (2018) 66:1173–80. doi: 10.1093/cid/cix967
35. Paz-Bailey G, Rosenberg ES, Sharp TM. Persistence of Zika virus in body fluids – final report reply. N Engl J Med;. (2019) 380:198–9. doi: 10.1056/NEJMc1814416
36. Chan JF, Zhang AJ, Chan CC, Yip CC, Mak WW, et al. Zika virus infection in dexamethasone-immunosuppressed mice demonstrating disseminated infection with multi-organ involvement including orchitis effectively treated by recombinant type I interferons. EBioMedicine. (2016) 14:112–22. doi: 10.1016/j.ebiom.2016.11.017
37. Osuna CE, Lim SY, Deleage C, Griffin BD, Stein D, et al. Zika viral dynamics and shedding in rhesus and cynomolgus macaques. Nat Med. (2016) 22:1448–55. doi: 10.1038/nm.4206
38. Hirsch AJ, Smith JL, Haese NN, Broeckel RM, Parkins CJ, Kreklywich C, et al. Zika Virus infection of rhesus macaques leads to viral persistence in multiple tissues PLoS Pathogens. (2017) 13:e1006219. doi: 10.1371/journal.ppat.1006219
39. Bagasra O, Addanki KC, Goodwin GR, Hughes BW, Pandey P, McLean E. Cellular targets and receptor of sexual transmission of Zika virus. Appl Immunohistochem Mol Morphol. (2017) 25:679–86. doi: 10.1097/PAI.0000000000000580
40. Clancy CS, Van Wettere AJ, Siddharthan V, Morrey JD, Julander JG. Comparative histopathologic lesions of the male reproductive tract during acute infection of Zika virus in AG129 and Ifnar-/- mice. Am J Pathol. (2018) 188:904–15. doi: 10.1016/j.ajpath.2017.12.019
41. Spencer JL, Lahon A, Tran LL, Arya RP, Kneubehl AR, Vogt MB et al. Replication of Zika virus in human prostate cells: a potential source of sexually transmitted virus. J Infect Dis. (2018) 217:538–47. doi: 10.1093/infdis/jix436
42. Matusali G, Houzet L, Satie AP, Mahe D, Aubry F, Couderc T, et al. Zika virus infects human testicular tissue and germ cells. J Clin Invest. (2018) 128:4697–710. doi: 10.1172/JCI121735
43. Govero J, Esakky P, Scheaffer SM, Fernandez E, Drury A, Platt DJ, et al. Zika virus infection damages the testes in mice. Nature. (2016) 540:438–42. doi: 10.1038/nature20556
44. Joguet G, Mansuy JM, Matusali G, Hamdi S, Walschaerts M, Pavili L, et al. Effect of Acute Zika virus infection on sperm and virus clearance in body fluids: a prospective observational study. Lancet Infect Dis. (2017) 17:1200–8. doi: 10.1016/S1473-3099(17)30444-9
45. Avelino-Silva VL, Alvarenga C, Abreu C, Tozetto-Mendoza TR, do Canto CL, Manuli ER, et al. Potential effect of Zika virus infection on human male fertility? Rev Inst Med Trop São Paulo. (2018) 60:e64. doi: 10.1590/s1678-9946201860064
46. Ma WQ, Li SH, Ma SQ, Jia LN, Zhang FC, Zhang Y, et al. Zika virus causes testis damage and leads to male infertility in mice. Cell. (2017) 167:1511–24. doi: 10.1016/j.cell.2016.11.016
47. Kaur G, Thompson LA, Dufour JM. Sertoli cells—immunological sentinels Of spermatogenesis. Semin Cell Dev Biol. (2014) 30:36–44. doi: 10.1016/j.semcdb.2014.02.011
48. Rubin S, Eckhaus M, Rennick LJ, Bamford CG, Duprex WP. Molecular biology, pathogenesis and pathology of mumps virus. J Pathol. (2015) 235:242–52. doi: 10.1002/path.4445
49. Wharton IP, Chaudhry AH, French ME. A case of mumps epididymitis. Lancet. (2006) 367:702. doi: 10.1016/S0140-6736(06)68274-3
50. Tarantino L, Giorgio A, de Stefano G, Farella N. Echo color Doppler findings in postpubertal mumps epididymo-orchitis. J Ultrasound Med. (2001) 20:1189–95 doi: 10.7863/jum.2001.20.11.1189
51. Wu H, Zhao X, Wang F, Jiang Q, Shi L, Gong M, et al. Mouse testicular cell type-specific antiviral response against mumps virus replication. Front Immunol. (2017) 8:117. doi: 10.3389/fimmu.2017.00117
52. Wu H, Shi L, Wang Q, Cheng L, Zhao X, Chen Q, et al. Mumps virus-Induced innate immune responses in mouse Sertoli and Leydig cells. Sci Rep. (2016) 6:19507. doi: 10.1038/srep19507
53. Siu MK, Lee WM, Cheng CY. The interplay of collagen IV. tumor necrosis factor-alpha, gelatinase B (matrix metalloprotease-9), and tissue inhibitor of metalloproteases-1 in the basal lamina regulates Sertoli cell-tight junction dynamics in the rat testis. Endocrinology. (2003) 144:371–87. doi: 10.1210/en.2002-220786
54. Wu H, Wang F, Tang D, Han D. Mumps orchitis: clinical aspects and mechanisms. Front Immunol. (2021) 12:582946. doi: 10.3389/fimmu.2021.582946
55. Akhigbe RE, Bamidele JO, Abodunrin OL. Seroprevalence of HIV infection in Kwara, Nigeria. Int J Virol. (2010) 6:158–63. doi: 10.3923/ijv.2010.158.163
56. Akhigbe RE, Bamidele JO. Prevalence and pattern of utilization of voluntary counseling and testing services and HIV infection in Ogbomoso, southwestern Nigeria. J Nat Sc Biol Med. (2013) 4:163–6. doi: 10.4103/0976-9668.107283
57. Deeks SG, Overbaugh J, Phillips A, Buchbinder S. HIV infection. Nat Rev Dis Primer. (2015) 1:15035. doi: 10.1038/nrdp.2015.35
58. Lowe SH, Sankatsing SU, Repping S, Veen FV, Reiss P, Lange JM, et al. Is the male genital tract really a sanctuary site for HIV? Arguments that it is not. AIDS. (2004) 18:1353–62. doi: 10.1097/01.aids.0000125979.64033.96
59. Krieger JN, Nirapathpongporn A, Chaiyaporn M, Peterson G, Nikolaev I, Akridge R, et al. Vasectomy and human immunodeficiency virus type 1 in semen. J Urol. (1998) 159:820–5. doi: 10.1016/S0022-5347(01)63742-X
60. Cohen MS, Hoffman IF, Royce RA, Kazembe P, Dyer JR, Daly CC, et al. Reduction of concentration of HIV-1 in semen after treatment of urethritis: implications for prevention of sexual transmission of HIV-1. IDSCAP Malawi Research Group. Lancet. (1997) 349:1868–73. doi: 10.1016/S0140-6736(97)02190-9
61. Ping LH, Cohen MS, Hoffman I, Vernazza P, Seillier-Moiseiwitsch F, Chakraborty H, et al.. Effects of genital tract inflammation on human immunodeficiency virus type 1 V3 populations in blood and semen. J Virol. (2000) 74:8946–52. doi: 10.1128/JVI.74.19.8946-8952.2000
62. Tachet A, Dulioust E, Salmon D, De Almeida M, Rivalland S, Finkielsztejn L, et al. Detection and quantification of HIV-1 in semen: identification of a subpopulation of men at high potential risk of viral sexual transmission. AIDS. (1999) 13:823–31. doi: 10.1097/00002030-199905070-00012
63. Pasquier C, Walschaerts M, Raymond S, Moinard N, Saune K, Daudin M, et al. Patterns of residual HIV-1 RNA shedding in the seminal plasma of patients on effective antiretroviral therapy. Basic Clin Androl. (2017) 27:17. doi: 10.1186/s12610-017-0063-x
64. Poretsky L, Can S, Zumoff B. Testicular dysfunction in human Immunodeficiency virus-infected men. Metabolism. (1995) 44:946–53. doi: 10.1016/0026-0495(95)90250-3
65. Kushnir VA, Lewis W. Human immunodeficiency virus/acquired Immunodeficiency syndrome and infertility: emerging problems in the era of highly active antiretrovirals. Fertil Steril. (2011) 96:546–53. doi: 10.1016/j.fertnstert.2011.05.094
66. Dulioust E, le Du A, Costagliola D, Guibert J, Kunstmann JM, Heard I, et al. Semen alterations in HIV-1 infected men. Hum Reprod. (2002) 17:2112–8. doi: 10.1093/humrep/17.8.2112
67. Nicopoullos JD, Almeida PA, Ramsay JW, Gilling-Smith C. The effect of human immunodeficiency virus on sperm parameters and the outcome of Intrauterine insemination following sperm washing. Hum Reprod. (2004) 19:2289–97. doi: 10.1093/humrep/deh426
68. van Leeuwen E, Cornelissen M, de Vries JW, Lowe SH, Jurriaans S, Repping S, et al. Semen parameters of a semen donor before and after infection with human immunodeficiency virus type 1: case report. Hum Reprod. (2004) 19:2845–8. doi: 10.1093/humrep/deh,510
69. Anderson JA, Ping LH, Dibben O, Jabara CB, Arney L, Kincer L, et al. HIV-1 populations in semen arise through multiple mechanisms. PLoS Path. (2010) 6:e1001053. doi: 10.1371/journal.ppat.1001053
70. Anderson D, Politch JA, Pudney J. HIV infection and immune defense of the penis. Am J Reprod Immunol. (2011) 65:220–9. doi: 10.1111/j.1600-0897.2010.00941.x
71. Akhigbe RE, Hamed MA, Odetayo AF. HAART and anti-Koch's impair Sexual competence, sperm quality and offspring quality when used singly and incombination in male Wistar rats. Andrologia. (2021) 53:13951. doi: 10.1111/and.13951
72. Awodele O, Popoola TD, Idowu O, Bashua BM, Awolola NA, Okunowo WO. Investigations into the risk of reproductive toxicity following exposure to highly active anti-retroviral drugs in rodents. Tokai J Exp Clin Med. (2018) 43:54–63.
73. Ajayi AF, Akhigbe RE. The physiology of male reproduction: Impact of drugs and their abuse on male fertility. Andrologia. (2020) 52:13672. doi: 10.1111/and.13672
74. Akhigbe RE, Hamed MA, Aremu AO. HAART exacerbates testicular damage and impaired spermatogenesis in anti-Koch-treated rats via dysregulation of lactate transport and glutathione content. Reprod Toxicol. (2021) 103:96–107. doi: 10.1016/j.reprotox.2021.06.007
75. Hernandez BY, Wilkens LR, Zhu X, Thompson P, McDuffie K, Shvetsov YB, et al. Transmission of human papillomavirus in heterosexual couples. Emerg Infect Dis. (2008) 14:888–94. doi: 10.3201/eid1406.0706162
76. Stanley MA, Pett MR, Coleman N. HPV: from infection to cancer. Biochem Soc Trans;. (2007) 35:1456–60. doi: 10.1042/BST0351456
77. IARC. Biological agents, volume 100B. A review of human carcinogens. IARC Monogr Eval Carcinog Risks Hum. (2012) 100:1–441.
78. Saraiya M, Unger ER, Thompson TD, Lynch CF, Hernandez BY, Lyu CW, et al. US assessment of HPV types in cancers: implications for current and 9-valent HPV vaccines. J Natl Cancer Inst. (2015) 107:djv086. doi: 10.1093/jnci/djv086
79. Wald A, Matson P, Ryncarz A, Corey L. Detection of herpes simplex virus DNA in semen of men with genital HSV-2 infection. Sexually Transm Dis. (1999) 26:1–3. doi: 10.1097/00007435-199901000-00001
81. Taylor TJ, Brockman MA, McNamee EE, Knipe DM. Herpes simplex virus. Front Biosci. (2002) 7:d752–64. doi: 10.2741/A809
82. Dejucq N, Jegou B. Viruses in the mammalian male genital tract and their effects on the reproductive system. Microbiol Mol Biol Rev. (2001) 65:208–31. doi: 10.1128/MMBR.65.2.208-231.2001
83. Zea-Mazo JW, Negrette-Mejia YA, Cardona-Maya W. Virus of sexual transmission: semen and virus relationship. Actas Urol Esp. (2010) 34:845–53. doi: 10.1016/S2173-5786(10)70212-2
84. Lisco A, Munawwar A, Introini A, Vanpouille C, Saba E, Fenh X, et al. Semen of HIV-1-infected individuals: local shedding of herpesviruses and reprogrammed cytokinenetwork. J Infect Dis. (2012) 205:97–105. doi: 10.1093/infdis/jir700
85. Gianella S, Smith DM, Vargas MV, Little SJ, Richman DD, Daar ES, et al. Shedding of HIV and human herpes viruses in the semen of effectively treated HIV-1-infected men who have sex with men. Clin Infect Dis. (2013) 57:441–7. doi: 10.1093/cid/cit252
86. Gianella S, Morris SR, Anderson C, Spina CA, Vargas MV, Young JA, et al. Herpes Viruses and HIV-1 drug resistance mutations influence the virologic and immunologic milieu of the male genital tract. AIDS. (2013) 27:39–47. doi: 10.1097/QAD.0b013e3283573305
87. Osborne BJ, Marsh AK, Huibner S, Shahabi K, Liu C, Contente T, et al. Clinical and mucosal immune correlates of HIV-1 semen levels in antiretroviral-naive men. Open Forum Infect Dis. (2017) 4:ofx033. doi: 10.1093/ofid/ofx033
88. Morris SR, Zhao M, Smith DM, Vargas MV, Little SJ, Gianella S. Longitudinal viral dynamics in semen during early HIV infection. Clin Infect Dis. (2017) 64:428–34. doi: 10.1093/cid/ciw784
89. Bezold G, Politch JA, Kiviat NB, Kuypers JM, Wolff H, Anderson DJ. Prevalence of sexually transmissible pathogens in semen from asymptomatic male infertility patients with and without leukocytospermia. Fertil Steril. (2007) 87:1087–97. doi: 10.1016/j.fertnstert.2006.08.109
90. Gimenes F, Souza RP, Bento JC, Teixeira JJ, Maria-Engler SS, Bonini MG, et al. Male infertility: a public health issue caused by sexually transmitted pathogens. Nat Rev Urol. (2014) 11:672–87. doi: 10.1038/nrurol.2014.285
91. Pallier C, Tebourbi L, Chopineau-Proust S, Schoevaert D, Nordmann P, Testart J, et al. Herpesvirus, cytomegalovirus, human sperm and assisted fertilization. Hum Reprod. (2002) 17:1281–1287 doi: 10.1093/humrep/17.5.1281
92. Rusz A, Pilatz A, Wagenlehner F, Linn T, Diemer T, Schuppe HC, et al. Influence of urogenital infections and inflammation of semen quality and male fertility. World J Urol. (2012) 30:23–30. doi: 10.1007/s00345-011-0726-8
93. Ochsendorf FR. Sexually transmitted infections: impact on male infertility. Andrologia. (2008) 40:72–5. doi: 10.1111/j.1439-0272.2007.00825.x
94. Laprise C, Trottier H, Monnier P, Coutlee F, Mayrand MH. Prevalence of Human papillomaviruses in semen: a systemic review and meta-analysis. Hum Repro. (2014) 29:640–51. doi: 10.1093/humrep/det453
95. Schuppe HC, Pilatz A, Hossain H, Diemer T, Wagenlehner F, Weidner W. Urogenital infection as a risk factor for male infertility. Dtsch Arztebl Int. (2017) 114:338–49. doi: 10.3238/arztebl.2017.0339
96. Xiong YQ, Chen YX, Cheng MJ, He WQ, Chen Q. The risk of human papillomavirus infection for male fertility abnormality: a meta-analysis. Asian J Androl. (2018) 20:493–7. doi: 10.4103/aja.aja_77_17
97. Cao X, Wei R, Zhang X, Zhou J, Lou J, Cui Y. Impact of human papillomavirus infection in semen on sperm progressive motility in infertile men: a systematic review and meta-analysis. Reprod Biol Endocrinol. (2020) 18:38. doi: 10.1186/s12958-020-00604-0
98. Gorolla A, Pizzol D, Bertoldo A, De Toni L, Barzon L. Association, prevalence, and clearance of human papillomavirus and antisperm antibodies in infected semen samples from infertile patients. Fertil Steril. (2013) 99:125–31. doi: 10.1016/j.fertnstert.2012.09.006
99. Foresta C, Patassini C, Bertoldo A, Menegazzo M, Francavilla F, et al. Mechanism of human papillomavirus binding to human spermatozoa and fertilizing ability of infected spermatozoa. PLoS ONE. (2011) 6:e15036. doi: 10.1371/journal.pone.0015036
100. Yang Y, Jia CW, Ma YM, Zhou LY, Wang SY. Correlation between HPV sperm infection and male infertility. Asian J Androl. (2013) 15:529–32. doi: 10.1038/aja.2013.36
101. Moghimi M, Zabihi-Mahmoodabadi S, Kheirkhah-Vakilabad A, Kargar Z. Significant correlation between high-risk HPV DNA in semen and impairment of sperm quality infertile men. Int J Fertil Steril. (2019) 12:306–9. doi: 10.22074/ijfs.2019.5421
102. Gorolla A, Engl B, Pizzol D, Ghezzi M, Bertoldo A, Bottacin A, et al. Spontaneous fertility and in vitro fertilization outcome: new evidence of human papillomavirus sperm infection. Fertil Steril. (2016) 105:65–72. doi: 10.1016/j.fertnstert.2015.09.018
103. Foresta C, Garolla A, Parisi S, Ghezzi M, Bertoldo A, Di Nisio A, et al. HPV prophylactic vaccination in males improves the clearance of semen infection. EBioMedicine (2015) 2:1487–93. doi: 10.1016/j.ebiom.2015.09.005
104. Foresta C, Bertoldo A, Garolla A, Pizzol D, Mason S, Lenzi A, et al. Human papillomavirus proteins are found in peripheral blood and semen Cd20+ and Cd56+ cells during HPV-16 semen infection. BMC Infect Dis. (2013) 13:593. doi: 10.1186/1471-2334-13-593
105. Yang Y, Li Y, Liang Y, Lan Y. Effect of male HPV infection on semen parameter. Chin J Birth Health Heredity. (2016) 24:113–4.
106. Yang X, Zhang Y, Wang X. Retrospective analysis of association between human papilloma virus infection and antisperm antibodies in male infertile patients in Xinjiang area. Int J Lab Med. (2015) 36:1910–2.
107. Zheng H, Wang L, Chen X. The effect of human papillomavirus on sperm motility and sperm chromatin integrity in semen. Chin J Clin (Electronic Edition). (2014) 8:70–4.
108. Fei QJ, Yang XD, Ni WH, Pan CS, Huang XF. Can hepatitis B virus DNA in semen be predicted by serum levels of hepatitis B virus DNA. HBeAg, and HBsAg in chronically infected men from infertile couples? Andrology. (2015) 3:506–11. doi: 10.1111/andr.12021
109. Leruez-Ville M, Kunstmann JM, De Almeida M, Rouzioux C, Chaix ML. Detection of hepatitis C virus in the semen of infected men. Lancet. (2000) 356:42–3. doi: 10.1016/S0140-6736(00)02435-1
110. Pasquier C, Bujan L, Daudin M, Righi L, Berges L, Thauvin L, et al. Intermittent detection of hepatitis C virus (HCV) in semen from men with human immunodeficiency virus type 1 (HIV-1) HCV. J Med Virol. (2003) 69:344–9. doi: 10.1002/jmv.10295
111. Briat A, Dulioust E, Galimand J, Fontaine H, Chaix ML, Letur-Könirsch H, et al. Hepatitis C virus in the semen of men coinfected with HIV-1: prevalence and origin. AIDS. (2005) 19:1827–35. doi: 10.1097/01.aids.0000189847.98569.2d
112. Bradshaw D, Lamoury F, Catlett B, Applegate TL, McAllister J, Dore GJ, et al. A comparison of seminal hepatitis C virus (HCV) RNA levels during recent and chronic HCV infection in HIV- infected and HIV-uninfected individuals. J Infect Dis. (2015) 211:736–43. doi: 10.1093/infdis/jiu550
113. Turner SS, Gianella S, Yip MJ, van Seggelen WO, Gillies RD, Foster AL, et al. Shedding of hepatitis C virus in semen of human immunodeficiency virus-infected men. Open Forum Infect Dis. (2016) 3:ofw057. doi: 10.1093/ofid/ofw057
114. Huysman A, Patel M, Dieterich DT. Hepatitis B: the immaculate infection. Gastroenterol Hepatol (N Y). (2007) 3:724–6.
115. Huang JM, Huang TH, Qiu HY, Fang XW, Zhuang TG, Qiu JW. Studies on the integration of hepatitis B virus DNA sequence in human sperm chromosomes. Asian J Androl. (2002) 4:209–12. doi: 10.3748/wjg.v9.i4.736
116. Huang JH, Zhong Y, Fang XW, Xie QD, Kang XJ, Wu R, et al. Hepatitis B virus S protein enhances sperm apoptosis and reduces sperm fertilizing capacity in vitro. PloS ONE. (2013) 8:e68688. doi: 10.1371/journal.pone.0068688
117. Bourlet T, Lornage J, Maertens A, Garret AS, Saoudin H, Tardy JC, et al. Prospectiveevaluation of the threat related to the use of seminal fractions from hepatitis C virus-infected men in assisted reproductive techniques. Hum Reprod. (2009) 24:530–5. doi: 10.1093/humrep/den414
118. Durazzo M, Premoli A, Di Bisceglie C, Bertagna A, Faga E, Biroli G, et al. Alterations of seminal and hormonal parameters: an extrahepatic manifestation of HCV infection? World J Gastroenterol. (2006) 12:3073–6. doi: 10.3748/wjg.v12.i19.3073
119. Lorusso F, Palmisano M, Chironna M, Vacca M, Masciandaro P, Bassi E, et al. Impact of chronic viral diseases on semen parameters. Andrologia. (2010) 42:121–6. doi: 10.1111/j.1439-0272.2009.00970.x
120. Moretti E, Federico MG, Giannerini V, Collodel G. Sperm ultrastructure meiotic segregation in a group of patients with chronic hepatitis B C. Andrologia. (2008) 40:286–91. doi: 10.1111/j.1439-0272.2008.00855.x
121. Hofer H, Donnerer J, Sator K, Staufer K, Scherzer TM, Dejaco C, et al. Seminal fluid ribavirin level and functional semen parameters in patients with chronic hepatitis C on antiviral combination therapy. J Hepatol. (2010) 52:812–6. doi: 10.1016/j.jhep.2009.12.039
122. Hofny ER, Ali ME, Taha EA, Nafeh HM, Sayed DS, Abdel-Azeem HG, et al. Semen and hormonal parameters in men with chronic hepatitis C infection. Fertil Steril. (2011) 95:2557–9. doi: 10.1016/j.fertnstert.2011.05.014
123. La Vignera S, Condorelli RA, Vicari E, D'Agata R, Calogero AE. Sperm DNA damage in patients with chronic viral, C, and hepatitis. Eur J Inter Med. (2012) 23:E19–24. doi: 10.1016/j.ejim.2011.08.011
124. Kang XJ, Xie QD, Zhou XL, Li FZ, Huang JH, Liu D, et al. Effects of hepatitis B virus S protein exposure on sperm membrane integrity and functions. PLoS ONE. (2012) 7:e33471. doi: 10.1371/journal.pone.0033471
125. Manno CS, Pierce GF, Arruda VR, Glader B, Ragni M, Rasko JJ, et al. Successful transduction of liver in hemophilia by AAV-Factor IX and limitations imposed by the host immune response. Nat Med. (2006) 12:342–7. doi: 10.1038/nm1358
126. Barzon L, Boscaro M, Palù G. Endocrine aspects of cancer gene therapy. Endocrine Rev. (2004) 25:1–44. doi: 10.1210/er.2002-0035
127. Arruda VR, Fields PA, Milner R, Wainwright L, De Miguel MP, Donovan PJ, et al. Lack of germline transmission of vector sequences following systemic administration of recombinant AAV-2 vector in males. Mol Ther. (2001) 4:586–92. doi: 10.1006/mthe.2001.0491
128. Favaro P, Downey HD, Zhou JS, Wright JF, Hauck B, Mingozzi F, et al. Host and vector-dependent effects on the risk of germline transmission of AAV vectors. Mol Ther. (2009) 17:1022–30. doi: 10.1038/mt.2009.56
129. Schuettrumpf J, Liu J-H, Couto LB, Addya K, Leonard DG, Zhen Z, et al. Inadvertent germline transmission of AAV2 vector: findings in a rabbit model correlate with those in a human clinical trial. Mol Ther. (2006) 13:1064–73. doi: 10.1016/j.ymthe.2006.03.002
130. Honaramooz A, Megee S, Zeng W, Destrempes MM, Overton SA, Luo J, et al. Adeno-associated virus (AAV)-mediated transduction of male germ line stem cells results in transgene transmission after germ cell transplantation. FASEB J. (2008) 22:374–82. doi: 10.1096/fj.07-8935com
131. Jakob M, Mühle C, Park J, Weiß S, Waddington S, Schneider H. No evidence for germ-line transmission following prenatal and early postnatal AAV-mediated gene delivery. J Gene Med. (2005) 7:630–7. doi: 10.1002/jgm.718
132. Erles K, Rohde V, Thaele M, Roth S, Edler L, Schlehofer JR. DNA of adeno-associated virus (AAV) in testicular tissue and in abnormal semen samples. Human Reprod. (2001) 16:2333–7. doi: 10.1093/humrep/16.11.2333
133. Rohde V, Erles K, Sattler HP, Derouet H, Wullich B, Schlehofer JR. Detection of adeno-associated virus in human semen: does viral infection play a role in the pathogenesis of male infertility? Fertil Steril. (1999) 72:814–6. doi: 10.1016/S0015-0282(99)00363-5
134. Schlehofer J, Boeke C, Reuland M, Eggert-Kruse W. Presence of DNA of adeno-associated virus in subfertile couples, but no association with fertility factors. Human Reprod. (2012) 27:770–8. doi: 10.1093/humrep/der427
135. Schlehofer JR. Adeno-associated virus (AAV) in semen and testis: a role in infertility? An overview. Andrologie. (2004) 14:317–9. doi: 10.1007/BF03034919
136. Deen GF, Broutet N, Xu W, Knust B, Sesay FR, McDonald SLR, et al. Ebola RNA persistence in semen of ebola virus disease survivors - final report. N Engl J Med. (2017) 377:1428–37. doi: 10.1056/NEJMoa1511410
137. Mate SE, Kugelman JR, Nyenswah TG, Ladner JT, Wiley MR, Cordier-Lassalle T. Molecular evidence of sexual transmission of ebola virus. N Engl J Med. (2015) 373:2448–54. doi: 10.1056/NEJMoa1509773
138. Fischer WA, Brown J, Wohl DA, Loftis AJ, Tozay S, Reeves E, et al. Ebola virus Ribonucleic acid detection in semen more than two years after resolution of acute ebola virus infection open forum. Infect Dis. (2017) 4:ofx155. doi: 10.1093/ofid/ofx155
140. Perry DL, Huzella LM, Bernbaum JG. Ebola virus Localization in theMacaque reproductive tract during acute Ebola virus disease. Am J Pathol. (2018) 188:550–8. doi: 10.1016/j.ajpath.2017.11.004
141. Coffin KM, Liu J, Warren TK. Persistent Marburg virus infection in the testes of nonhuman primate survivors. Cell Host Microbe. (2018) 24:405–16.e3. doi: 10.1016/j.chom.2018.08.003
142. Guetiya W, Samin A, Mafopa NG. Mobile health clinic for the medical management of clinical sequelae experienced by survivors of the 2013-2016 Ebola virus disease outbreak in Sierra Leone, West Africa. Eur J Clin Microbiol Infect Dis. (2017) 36:2193–200. doi: 10.1007/s10096-017-3045-1
143. de St Maurice A, Ervin E, Orone R, Choi M, Dokubo EK, Rollin PE, et al. Care of ebola survivors and factors associated with clinical sequelae-Monrovia, Liberia. Open Forum Infect Dis. (2018) 5:ofy239. doi: 10.1093/ofid/ofy239
144. Baize S, Leroy EM, Georges AJ, Georges-Courbot MC, Capron M, Bedjabaga I, et al. Inflammatory responses in Ebola virus-infected patients. Clin Exp Immunol. (2002) 128:163–8. doi: 10.1046/j.1365-2249.2002.01800.x
145. Sengupta P, Leisegang K, Agarwal A. The impact of COVID-19 on the male reproductive tract and fertility: a systematic review. Arab J Urol. (2021) 19:423–36. doi: 10.1080/2090598X.2021.1955554
146. Xu J, Qi L, Chi X, Yang J, Wei X, Gong E, et al. Orchitis: a complication of severe acute respiratory syndrome (SARS). Biol Reprod. (2006) 74:410–6. doi: 10.1095/biolreprod.105.044776
147. Zhou D, Dai S, Tong Q. COVID-19: a recommendation to examine the effect ofhydroxychloroquine in preventing infection and progression. J Antimicrob Chemother. (2020) 75:1667–70. doi: 10.1093/jac/dkaa114
148. Zhou P, Yang XL, Wang XG, Hu B, Zhang L, Zhang W, et al. A pneumonia outbreak associated with a new coronavirus of probable bat origin. Nature. (2020) 579:270–3. doi: 10.1038/s41586-020-2012-7
149. Aitken RJ. COVID-19 and human spermatozoa—Potential risks for infertility and sexual transmission? Andrology. (2020) 2020:12859. doi: 10.1111/andr.12859
150. Akhigbe RE, Hamed MA. Possible links between, COVID-,19 and male fertility. Asian Pac J Reprod. (2020) 9:211–4. doi: 10.4103/2305-0500.294662
151. Holtmann N, Edimiris P, Andree M, Doehmen C, Baston-Buest D, Adams O, et al. Assessment of SARS-CoV-2 in human semen-a cohort study. Fertil Steril. (2020) 114:233–8. doi: 10.1016/j.fertnstert.2020.05.028
152. Paoli D, Pallotti F, Colangelo S, Basilico F, Mazzuti L, Turriziani O, et al. Study of SARS-CoV-2 in semen and urine samples of a volunteer with positive naso-pharyngeal swab. J Endocrinol Invest. (2020) 43:1819–22. doi: 10.1007/s40618-020-01261-1
153. Li D, Jin M, Bao P, Zhao W, Zhang S. Clinical characteristics and results of semen tests among men with coronavirus disease 2019. JAMA Netw Open. (2020) 3:e208292. doi: 10.1001/jamanetworkopen.2020.8292
154. Bhattacharya K, Mukhopadhyay LD, Goswami R, Dutta S, Sengupta P, Irez T, et al. SARS-CoV-2 infection and human semen: possible modes of contamination and transmission. Middle East Fertility Soc J. (2021) 26:1–7. doi: 10.1186/s43043-021-00063-6
155. Ma L, Xie W, Li D, Shi L, Mao Y, Xiong Y, et al. Effect of SARS-CoV-2 infection upon male gonadal function: a single center-based study. MedRxi [Preprint]. (2020). doi: 10.1101/2020.03.21.2003726
156. Moghimi N, Farsani BE, Ghadipasha M, Mahmoudiasl GR, Piryaei A, Aliaghaei A, et al. COVID-19 disrupts spermatogenesis through the oxidative stress pathway following induction of apoptosis. Apoptosis. (2021) 26:415–30.
157. Blendon RJ, Benson JM, DesRoches CM, Raleigh E, Taylor-Clark K. The public's response to severe acute respiratory syndrome in Toronto and the United States. Clin Infect Dis. (2004) 38:925–31. doi: 10.1086/382355
158. Wheatland R. Molecular mimicry of ACTH in SARS - implications for corticosteroid treatment and prophylaxis. Med Hypotheses. (2004) 63:855–62. doi: 10.1016/j.mehy.2004.04.009
159. Bouayed J, Rammal H, Soulimani R. Oxidative stress and anxiety: relationship and cellular pathways. Oxid Med Cell Longev. (2009) 2:63–7. doi: 10.4161/oxim.2.2.7944
160. Sengupta P, Dutta S. Does SARS-CoV-2 infection cause sperm DNA fragmentation? Possible link with oxidative stress. Eur J Contracept Reprod Health Care. (2020) 25:405–6. doi: 10.1080/13625187.2020.1787376
161. Nillni YI, Wesselink AK, Hatch EE, Mikkelsen EM, Gradus JL, Rothman KJ, et al. Mental health, psychotropic medication use, and menstrual cycle characteristics. Clin Epidemiol. (2018) 10:1073–82. doi: 10.2147/CLEP.S152131
162. De Paepe ME, Waxman M. Testicular atrophy in AIDS: a study of 57 autopsy cases. Hum Pathol. (1989) 20:210–4.
163. Ashrafi GH, Salman NA. Pathogenesis of human papillomavirus-immunological responses to HPV infection. In: Rajkumar R, editor. Human Papillomavirus-Research in a Global Perspective. (2016) 13:243–53.
164. Akhigbe RE, Ajayi LO, Ajayi AF. Codeine exerts cardiorenal injury via upregulation of adenine deaminase/Xanthine oxidase and caspase 3 signaling. Life Sci. (2021) 273:118717. doi: 10.1016/j.lfs.2020.118717
165. Lui WY, Cheng CY. Regulation of cell junction dynamics by cytokines in the testis –A molecular and biochemical perspective. Cytokine Growth Factor Rev. (2007) 18:299–311. doi: 10.1016/j.cytogfr.2007.04.009
166. Syriou V, Papanikolaou D, Kozyraki A, Goulis DG. Cytokines and male infertility. Eur Cytokine Netw. (2018) 29:73–82. doi: 10.1684/ecn.2018.0412
167. Oh YS, Jo NH, Park JK. GyeMC.Changes in inflammatory cytokines accompany deregulation of Claudin-11, resulting in inter-Sertoli tight junctions in varicocele rat testes. J Urol. (2016) 196:1303–12. doi: 10.1016/j.juro.2016.05.004
168. Jacobo P, Guazzone VA, Theas MS, Lustig L. Testicular autoimmunity. Autoimmun Rev. (2011) 10:201–4. doi: 10.1016/j.autrev.2010.09.026
169. Fujita Y, Mihara T, Okazaki T, Shitanaka M, Kushino R, Ikeda C, et al. Toll-like receptors (TLR) 2 and 4 on human sperm recognize bacterial endotoxins and mediate apoptosis. Hum Reprod. (2011) 26:2799–806. doi: 10.1093/humrep/der234
170. Akhigbe R, Ajayi A. Testicular toxicity following chronic codeine administration is via oxidative DNA damage and up-regulation of NO/TNF-α and caspase 3 activities. PLoS ONE. (2020) 15:e0224052. doi: 10.1371/journal.pone.0224052
171. Makker K, Agarwal A, Sharma R. Oxidative stress and male infertility. Indian J Med Res. (2009) 129:357–67.
172. Agarwal A, Virk G, Ong C, du Plessis SS. Effect of oxidative stress on male reproduction. World J Mens Health. (2014) 32:1–17. doi: 10.5534/wjmh.2014.32.1.1
173. Ajayi AF, Akhigbe RE. In vivo exposure to codeine induces reproductive toxicity: role of HER2 and p53/Bcl-2 signaling pathway. Heliyon. (2020) 6:e05589. doi: 10.1016/j.heliyon.2020.e05589
174. Zribi N, Chakroun NF, Elleuch H, Abdallah FB, Ben Hamida AS, Gargouri J, et al. Sperm DNA fragmentation and oxidation are independent of malondialdheyde. Repro Biol Endocrinol. (2011) 9:47. doi: 10.1186/1477-7827-9-47
175. Aitken RJ, Baker MA. Causes and consequences of apoptosis in spermatozoa; contributions to infertility and impacts on development. Int J Dev Biol. (2013) 57:265–72. doi: 10.1387/ijdb.130146ja
176. Ajayi AF, Akhigbe RE. Codeine-induced sperm DNA damage is mediated Predominantly by oxidative stress rather than apoptosis. Redox Report. (2020) 25:33–40 doi: 10.1080/13510002.2020.1752003
177. Souho T, Benlemlih M, Bennani B. Human papillomavirus infection and fertility alteration: a systematic review. PLoS ONE. (2015) 10:e0126936. doi: 10.1371/journal.pone.0126936
178. Liu WH, Han RQ, Wu H, Han DS. Viral threat to male fertility. Andrologia. (2018) 50:e13140. doi: 10.1111/and.13140
179. Pecou S, Moinard N, Walschaerts M, Pasquier C, Daudin M, Bujan L. Ribavirin and pegylated interferon treatment for hepatitis C was associated not only with semen alterations but also with sperm deoxyribonucleic acid fragmentation in humans. Fertility and Sterility. (2009) 91:933.e17–22. doi: 10.1016/j.fertnstert.2008.07.1755
180. VIDAL Hoptimal,. VIDAL, l'information de reference sur les produits de sante. Consulte le 5 juillet (2016). Available online at: http://www.vidalfrance.com/solutions/solutions-professionnels/vidal-hoptimal/ (accessed December 21, 2021).
181. Erpenbach KH. Systemic treatment with interferon-alpha 2B: an effective method to prevent sterility after bilateral mumps orchitis. J Urol. (1991) 146:54–6. doi: 10.1016/S0022-5347(17)37713-3
182. Ku JH, Kim YH, Jeon YS, Lee NK. The preventive effect of systemic treatment with interferon-alpha2B for infertility from mumps orchitis. BJU Int. (1999) 84:839–42. doi: 10.1046/j.1464-410x.1999.00273.x
183. Lane TM, Hines J. The management of mumps orchitis. BJU Int. (2006) 97:1–2. doi: 10.1111/j.1464-410X.2006.05869.x
184. Azu OO, Naidu ECS, Naidu JS, Masia T, Nzemande NF, Chuturgoon A, et al. Testicular histomorphologic and stereological alterations following short-term treatment with highly active antiretroviral drugs (HAART) in an experimental animal model. Andrology. (2014) 2:772–9. doi: 10.1111/j.2047-2927.2014.00233.x
185. Bujan L, Sergerie M, Moinard N, Martinet S, Porte L, Massip P, et al. Decreased semen volume and spermatozoa motility in HIV-1-infected patients under antiretroviral treatment. J Androl. (2007) 28:444–52. doi: 10.2164/jandrol.106.001529
186. Savasi V, Oneta M, Laoreti A, Parisi F, Parrilla B, Duca P, et al. Effects of antiretroviral therapy on sperm DNA integrity of HIV-1-infected men. Am J Men Health. (2018) 12:1824–31. doi: 10.1177/1557988318794282
187. Zamora MJ, Obradors A, Woodward B, Vernaeve V, Vassena R. Semen residual viral load and reproductive outcomes in HIV-infected men undergoing ICSI after extended semen preparation. Reprod Biomed Online. (2016) 32:584–90. doi: 10.1016/j.rbmo.2016.02.014
188. ESHRE European European Society of Human Reproduction Embryology. Medically Assisted Reproduction in Patients with a Viral Infection/Disease. ESHRE (2021). Available online at: https://www.eshre.eu/-/media/sitecore-files/Guidelines/Viral-diseases/ESHRE-viral-infectiondisease-guideline_vs2.pdf (accessed January 19, 2022).
189. Hsieh YY, Sun YL, Chang CC, Lee YS, Tsai HD, Lin CS. Superoxide dismutase activities of spermatozoa and seminal plasma are not correlated with male infertility. J Clin Lab Anal. (2002) 16:127–31. doi: 10.1002/jcla.10029
190. Johnson EJ. The role of carotenoids in human health. Nutr Clin Care. (2002) 5:56–65. doi: 10.1046/j.1523-5408.2002.00004.x
191. Akmal M, Qadri JQ, Al-Waili NS, Thangal S, Haq A, Saloom KY. Improvement in human semen quality after oral supplementation of vitamin C. J Med Food. (2006) 9:440–42. doi: 10.1089/jmf.2006.9.440
192. Ciftci H, Verit A, Savas M, Yeni E, Erel O. Effects of N-acetylcysteine on semen parameters and oxidative/antioxidant status. Urology. (2009) 74:73–6. doi: 10.1016/j.urology.2009.02.034
193. Showell MG, Mackenzie-Proctor R, Brown J, Yazdani A, Stankiewicz MT, Hart RJ.Antioxidants for male subfertility. Cochrane Database Syst Rev. (2014) 1:CD007411. doi: 10.1002/14651858.CD007411.pub3
194. Nadjarzadeh A, Shidfar F, Amirjannati N, Vafa MR, Motevalian SA, Gohari MR, et al. Effect of Coenzyme Q10 supplementation on antioxidant enzymes activity and oxidative stress of seminal plasma: a double-blind randomized clinical trial. Andrologia. (2014) 46:177–83. doi: 10.1111/and.12062
195. Wong WY, Merkus HM, Thomas CM, Menkveld R, Zielhuis GA, Steegers-Theunissen RP. Effects of folic acid and zinc sulfate on male factor subfertility: a double-blind, randomized, placebo-controlled trial. Fertility Sterility. (2002) 77:491–8. doi: 10.1016/S0015-0282(01)03229-0
196. Ahsan U, Kamran Z, Raza I, Ahmad S, Babar W, Riaz MH, et al. Role of selenium in male reproduction–a review. Anim Reprod Sci. (2014) 146:55–62. doi: 10.1016/j.anireprosci.2014.01.009
197. Zhao J, Dong X, Hu X, Long Z, Wang L, Liu Q, et al. Zinc levels in seminal plasma and their correlation with male infertility: a systematic review and meta-analysis. Sci Rep. (2016) 6:22386. doi: 10.1038/srep22386
198. Harborne JB, Williams CA. Advances in flavonoid research since 1992. Phytochemistry. (2000) 55:481–504. doi: 10.1016/S0031-9422(00)00235-1
199. Heim KE, Tagliaferro AR, Bobilya DJ. Flavonoid antioxidants: chemistry, metabolism and structure-activity relationships. J Nutr Biochem. (2002) 13:572–84. doi: 10.1016/S0955-2863(02)00208-5
200. Arafa M, Agarwal A, Majzoub A, Selvam M, Baskaran S, Henkel R, et al. Efficacy of antioxidant supplementation on conventional and advanced sperm function tests in patients with idiopathic male infertility. Antioxidants. (2020) 9:219. doi: 10.3390/antiox9030219
201. Talevi R, Barbato V, Fiorentino I, Braun S, Longobardi S, Gualtieri R. Protective effects of in vitro treatment with zinc, d-aspartate and coenzyme q10 on human sperm motility, lipid peroxidation and DNA fragmentation. Reprod Biol Endocrinol. (2013) 11:81. doi: 10.1186/1477-7827-11-81
Keywords: antiviral, apoptosis, infertility, inflammation, oxidative stress, oxidative stress, retroviral, virus
Citation: Akhigbe RE, Dutta S, Hamed MA, Ajayi AF, Sengupta P and Ahmad G (2022) Viral Infections and Male Infertility: A Comprehensive Review of the Role of Oxidative Stress. Front. Reprod. Health 4:782915. doi: 10.3389/frph.2022.782915
Received: 25 September 2021; Accepted: 11 January 2022;
Published: 03 February 2022.
Edited by:
Peter Natesan Pushparaj, King Abdulaziz University, Saudi ArabiaReviewed by:
Giulia Collodel, University of Siena, ItalyMaciej Kurpisz, Department of Reproductive Biology and Stem Cells, Institute of Human Genetics, Polish Academy of Sciences (PAN), Poland
Gauthaman Kalamegam, Saveetha Dental College and Hospitals, India
Copyright © 2022 Akhigbe, Dutta, Hamed, Ajayi, Sengupta and Ahmad. This is an open-access article distributed under the terms of the Creative Commons Attribution License (CC BY). The use, distribution or reproduction in other forums is permitted, provided the original author(s) and the copyright owner(s) are credited and that the original publication in this journal is cited, in accordance with accepted academic practice. No use, distribution or reproduction is permitted which does not comply with these terms.
*Correspondence: Pallav Sengupta, cGFsbGF2X2N1JiN4MDAwNDA7eWFob28uY29t; Gulfam Ahmad, Z3VsZmFtLmFobWFkJiN4MDAwNDA7c3lkbmV5LmVkdS5hdQ==
†ORCID: Roland E. Akhigbe orcid.org/0000-0002-3874-5927
Sulagna Dutta orcid.org/0000-0002-7893-5282
M. A. Hamed orcid.org/0000-0003-3378-6794
Ayodeji F. Ajayi orcid.org/0000-0002-0992-1653
Pallav Sengupta orcid.org/0000-0002-1928-5048
Gulfam Ahmad orcid.org/0000-0003-3856-3207