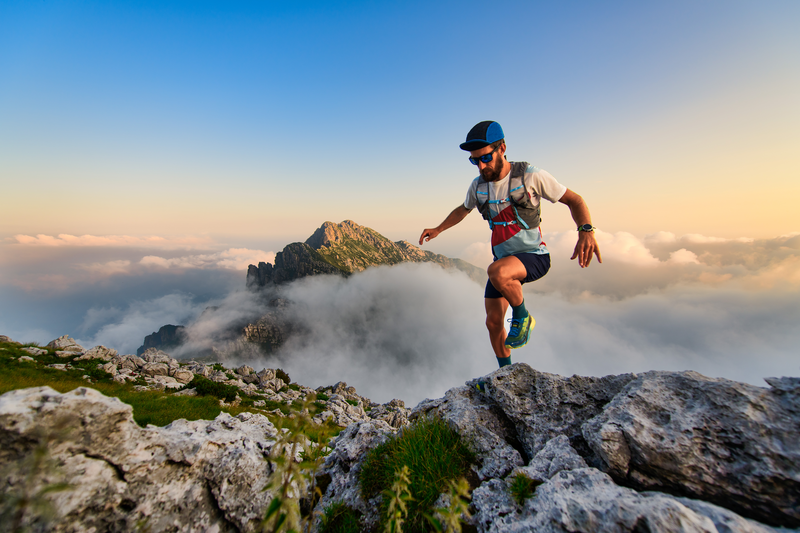
94% of researchers rate our articles as excellent or good
Learn more about the work of our research integrity team to safeguard the quality of each article we publish.
Find out more
ORIGINAL RESEARCH article
Front. Remote Sens.
Sec. Acoustic Remote Sensing
Volume 6 - 2025 | doi: 10.3389/frsen.2025.1482244
This article is part of the Research Topic Detection and Characterization of Unidentified Underwater Biological Sounds, Their Spatiotemporal Patterns and Possible Sources. View all 9 articles
The final, formatted version of the article will be published soon.
You have multiple emails registered with Frontiers:
Please enter your email address:
If you already have an account, please login
You don't have a Frontiers account ? You can register here
Through sharing characteristics of chorus activity, especially in regions that are particularly data deficient, we can aim at a broader, global understanding of fish chorusing and consequently important spatiotemporal changes in habitat use by schooling fish. Here, we identify seasonal changes in fish chorusing activity using passive acoustic monitoring, in the Central Red Sea mesophotic and adjacent shallow coral reef zones. For this study, recorders were placed in the mesophotic coral reef zone (70-80 m), and adjacent shallow reef sites (10 m), over two weeks during summer and winter seasons. A total of eleven choruses were identified and catalogued according to timing, location and acoustic characteristics of frequency and sound pressure levels.The presence of choruses in both deep and shallow reef sites is indicative of critical habitat for fish foraging, courtship, spawning, and/or migratory activity. All but two choruses were found to originate at or near the mesophotic sites. Four choruses unique to summer and winter respectively were most prevalent in soundscapes. Temperature and oxygen levels, measured to document conditions under which the choruses were present, showed little change across the mesophotic zone even between seasons, while daily fluctuation occurred in the adjacent shallow sites in both seasons.
Keywords: fish, chorus, acoustic, Monitoring, soundscape, Mesophotic, coral, reef
Received: 17 Aug 2024; Accepted: 03 Mar 2025.
Copyright: © 2025 Havlik, Parry, Steckbauer, Watts, Marchese, Benzoni and Duarte. This is an open-access article distributed under the terms of the Creative Commons Attribution License (CC BY). The use, distribution or reproduction in other forums is permitted, provided the original author(s) or licensor are credited and that the original publication in this journal is cited, in accordance with accepted academic practice. No use, distribution or reproduction is permitted which does not comply with these terms.
* Correspondence:
Michelle-Nicole Havlik, King Abdullah University of Science and Technology, Thuwal, Saudi Arabia
Disclaimer: All claims expressed in this article are solely those of the authors and do not necessarily represent those of their affiliated organizations, or those of the publisher, the editors and the reviewers. Any product that may be evaluated in this article or claim that may be made by its manufacturer is not guaranteed or endorsed by the publisher.
Research integrity at Frontiers
Learn more about the work of our research integrity team to safeguard the quality of each article we publish.