- 1Center for Interacting Urban Networks (CITIES), New York University, Abu Dhabi, United Arab Emirates
- 2Marine Biology Laboratory, New York University, Abu Dhabi, United Arab Emirates
- 3Mubadala ACCESS Center, New York University, Abu Dhabi, United Arab Emirates
Since the 1970s oil boom, nations surrounding the Arabian Gulf have witnessed rapid coastal urbanization, which accelerated in the early 2000s with the emergence of large-scale coastal ‘mega-projects’ designed to accommodate growing populations, attract international investments, and promote tourism. This development surge has had profound environmental impacts, including significant habitat modification, land use and land cover (LULC) change, and increased environmental pressure. Remote sensing (RS) technologies have become indispensable tools for monitoring these changes, offering cost-effective and non-intrusive methods to map and assess coastal zones. However, RS applications across the Arabian Gulf have been spatially limited, often focusing narrowly on specific cities or habitats while neglecting the broader geographical and coastal dimensions of urbanization. This study addresses this gap by conducting a systematic review of peer-reviewed RS literature from 1971 to 2022, covering the coastal regions of the eight nations bordering the Arabian Gulf. A total of 186 publications were categorized into three focal areas: 1) coastal urbanization and LULC, 2) coastal and marine habitats, and 3) environmental pressures and state changes. The results reveal a significant increase in RS studies in recent years, with around two-thirds of the publications (64.3%) appearing between 2016 and 2022. Studies predominantly focused on environmental pressures and state changes (35%), followed by habitat modification (27%), and coastal urbanization (20%). Geographically, RS research primarily concentrated on the coasts of the southern Gulf (UAE and Qatar) and western Gulf (Bahrain and Saudi Arabia), where major urban centers are located, while the northern Gulf (Kuwait and Iraq) and Iranian coast have been less studied. The systematic review highlights the need for integrated RS and GIS-based monitoring systems that combine different sources of RS data and in situ measurements to evaluate the Gulf as a unified system. Expanding spatial coverage, enhancing temporal analysis, and fostering regional collaboration are necessary to improve the understanding and management of coastal urbanization and environmental changes in the Arabian Gulf. This approach will more effectively inform decision-makers, and support more sustainable coastal management and long-term environmental resilience in the region.
1 Introduction
The accelerated modification of landscapes and seascapes at global scales has produced a variety of environmental impacts on coastal ecosystems, despite these being some of the most biodiverse and economically valuable ecosystems on Earth, where nearly half of the human population and two-thirds of the world’s megacities are located (Burt, 2014; Burt and Bartholomew, 2019). The magnitude of this matter has been notably pronounced in the Arabian Gulf (also known as the Persian Gulf and hereafter referred to as the Gulf) - a water body with geopolitical significance in the energy and trade sectors, but also underappreciated importance for the marine ecosystems that line its shores. These include saltmarshes, mudflats, sabkhas (salt flats) and mangrove forests in the intertidal zone, as well as algal beds, seagrass meadows and coral reefs in nearshore habitats (Burt, 2014; van Lavieren et al., 2011; Vaughan et al., 2019). As a subtropical sea lying between 24°N and 30°N latitude and 48°E and 57°E longitude, the Gulf operates as a biogeographic extension of the Western Indian Ocean (Burt et al., 2011). Its shallow depths (mean: 30 m) and geographic isolation from the Indian Ocean position it as one of the most extreme seas on Earth, with waters characterized by hyper-salinity, highly variable and severe temperatures (12°C–36°C annually), high turbidity and intermittent hypoxia (Burt and Paparella, 2023). While each of these natural stressors affect the physiology of marine organisms, they are also overlain by anthropogenic pressures arising from the growth of coastal populations (Friis and Burt, 2020).
The eight states surrounding the Gulf, consisting of Oman, the United Arab Emirates (UAE), Qatar, Bahrain, the Kingdom of Saudi Arabia (KSA), Kuwait, the Republic of Iraq, and the Islamic Republic of Iran, have witnessed rapid urban development over the past five decades. This development can be distinctly observed by the regional quadrupling of the population from 48 million in 1971 to 189 million in 2021 (Figure 1) (Asghar Pilehvar, 2021; Burt and Bartholomew, 2019). While the ancient settlements of Dilmun in Bahrain (3,000 BC to 400 BC), Um Alnar in Abu Dhabi (2,800 BC), and Failaka in Kuwait (2,300 BC), among others, show that urbanization is not a novel phenomenon in this region, scholars suggest that it is the nature and rate of such urban development have drastically increased since the discovery of oil and gas and through the ensuing migration, economic initiatives, and social welfare policies enacted (El-Arifi, 1986; Ramadan, 2015). Presently, the Gulf has assumed a key geo-economic role as a major maritime conduit by carrying approximately 21 million barrels of oil daily to the world (U.S. Department of Energy, 2019). And, despite country-level variations in urban rates and increased levels of prosperity for coastal communities, it has become increasingly clear that rapid urbanization has exerted substantial pressure on coastal ecosystems.
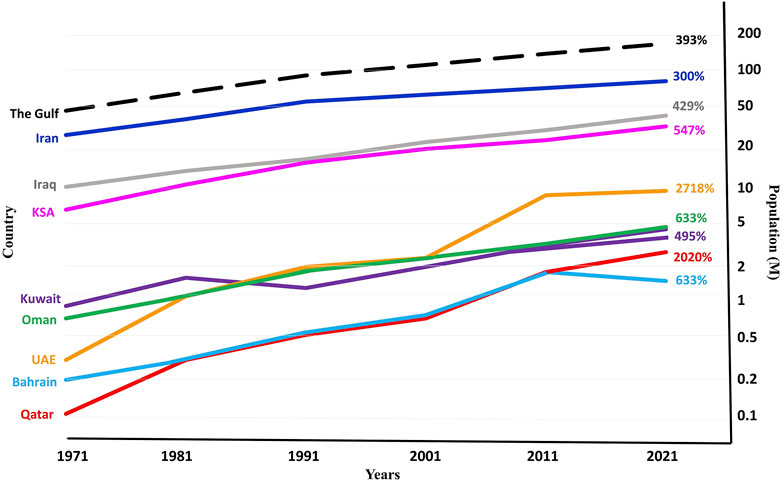
Figure 1. The population growth in the eight Gulf countries from 1971 to 2021 in million (M) (Source: World Bank (World Total Population)(12)). The growth percentage over the 50-year period is indicated at the end of each line.
The prominent features of urban development, including the iconic coastal mega-developments characterized by quay walls, piers, breakwaters, and accompanying infrastructure along coastal cities, as well as the exurban industrial networks supporting these burgeoning urban populations, resulted in significant coastal footprints. These have been exacerbated in marine systems by dredging of shipping channels, infilling and reclamation for ports expansion, construction of defence structures around power and desalination facilities, and the installation of hundreds of wellheads and thousands of kilometers of subsea piping that supports regional oil and gas industries (Bugnot et al., 2021). The need to accommodate a growing population, attract international investments, and promote tourism also spurred the development of massive coastal ‘mega-projects’ across the Gulf. Some notable mega-projects include the ‘Palm Islands’ and ‘World Islands’ in the UAE, the ‘Pearl’ in Qatar, the ‘Durrat Al Bahrain’ in Bahrain, and Sabah Al Ahmed City in Kuwait (Burt, 2014; van Lavieren et al., 2011).
With this pace of development often outstripping regulatory and management efforts in this region, there has been a growing need to assess and evaluate future urban growth and its environmental consequences (Burt and Bartholomew, 2019; Burt et al., 2017). Geospatial technologies, particularly remote sensing (RS) and geographic information systems (GIS) are essential tools for understanding the spatiotemporal patterns of regional coastal development and urbanization. By employing tools that extend across decadal time-frames and national borders, these tools can reveal insights into development patterns, environmental impacts, and the underlying forces driving them, surpassing the limitations of single-site or ‘snapshot’ perspectives often encountered in the past. RS, particularly, proves invaluable for documenting changes in land use and land cover (LULC) and monitoring the spatiotemporal trajectories of various habitat types (Alqurashi & Kumar, 2016; Dahy et al., 2021; Dahy et al., 2022; Issa et al., 2023; Issa et al., 2021). Features such as a synoptic view of RS data, repetitive data acquisition, digital formats optimized for computer processing, and cost-effective and accessible archival RS data offer great potential for the detection and mapping of long-term LULC change (Lu et al., 2004; Ramachandra et al., 2012).
RS approaches have been applied across the globe to analyze trends in coastal urbanization through the use of satellite imagery, aerial photos, or drone-based imagery (Mahrad et al., 2020) to document the urban expansion of recently built infrastructure (Wong et al., 2019), reclaimed land and/or dredged waterways (Chen et al., 2017; Duan et al., 2016; Wu et al., 2022; Zhang et al., 2017). These techniques have also been used to inform coastal and marine environmental management by retroactively tracking temporal changes in ecosystems in response to coastal development, such as changes in coral (Bennett et al., 2020; Purkis, 2018; Xu and Zhao, 2014), seagrass beds (Bakirman et al., 2016; Elso et al., 2017; Traganos et al., 2018) and mangrove forests (Wang et al., 2019; Yancho et al., 2020). RS can also be applied to address environmental impacts, including the degradation of coastal and marine habitats (e.g., direct removal or damage of critical habitats such as seagrass, corals, and beaches) (Cunning et al., 2019; Hill et al., 2014), changes in hydrodynamics (Mancini et al., 2012), reduced water quality (e.g., turbidity and contamination) (Naimaee et al., 2024), the overexploitation of marine resources (e.g., destructive fishing methods) (Klemas, 2013), and the introduction of non-native aquatic species (Mannino et al., 2021; Theriault et al., 2006). In the Gulf region, RS-based research has increasingly been utilized to collect primary data. However, these studies have typically concentrated on urbanization alone or focused on specific cities (Yaqoub and Kolan, 2006; Daoudi and Niang, 2021), countries (Asghar Pilehvar, 2021; Uddin et al., 2010), or habitats (Rondon et al., 2023; Khan and Kumar, 2009). The broader coastal and environmental dimensions of these studies have often ambiguous or insufficient. No study, to date, has comprehensively and systematically reviewed RS literature on coastal urbanization and development across the eight nation-states bordering the Gulf. Consequently, there is limited information on the various remote sensed data-types and techniques used to understand LULC change, marine habitat impacts and environmental pressures. To address this knowledge gap, this study conducted a systematic review to collate all published peer-reviewed literature related to RS techniques and approaches applied to coastal zones across the eight countries surrounding the Gulf, spanning from the earliest publication in 1971 through to 2022. The objectives of this study serve to (a) illustrate the temporal and spatial distribution of the reviewed RS-based articles in the Gulf (Section 3.1), (b) highlight RS data and methods used for mapping and detecting LULC changes, emphasizing the “coastal urban” class and coastal/marine habitats (Section 3.2; Section 3.3), (c) situate the findings of RS-based studies within the regional environmental context, focusing on environmental pressures and state changes in the Gulf (Section 3.4), and (d) identify opportunities for future RS-based studies and present recommendations that can contribute to sustainable coastal development and management moving forward (Section 5).
2 Materials and methods
As one of the most robust methods for the selection, consolidation, and evaluation of emergent evidence, systematic reviews rely on transparent and reproducible processes to identify areas where evidence may be lacking, contradictory, or entangled, to inform evidence-based decision making and to illustrate the historical development of knowledge, with many more directed advantages (Aromataris and Pearson, 2014). This review pursued a broad search strategy for all RS publications examining coastal areas and zones in the Gulf, which was then distilled down through the categorization and sub-categorization of the included studies to understand trends, not simply across time and space, but also with three critical topics: coastal urbanization, habitats, and environmental pressures. This approach serves to strike a balance between search strategy sensitivity and precision as well as to prevent the omission of relevant articles.
2.1 Sources and search strategies
We developed and computed a search strategy within three databases: ScienceDirect, Scopus, and Web of Science. The Boolean search string, as depicted below, employed the truncation function (*) to account for variation in word endings in the terms “remote sens*” (e.g., remote sensing and remote sensors) and “coast*” (e.g., coastline and coastal erosion) as well as listed the eight bordering countries and urban centers in the region (Supplementary File 1 for search strings per database).
Search String Line 1: “remote sens*” and “coast*”
AND.
Search String Line 2: “Arabian Gulf” OR “Persian Gulf” OR “Oman” OR “UAE” OR “United Arab Emirates” OR “Qatar” OR “Bahrain” OR “Saudi Arabia” OR “Kuwait” OR “Iraq” OR “Iran” OR “Musandam” OR “Ras Al*Khaimah” OR “Umm Al*Quwain” OR “Ajman” OR “Sharjah” OR “Dubai” OR “Abu Dhabi” OR “Doha” OR “Manama” OR “Dammam” OR “Al*Khobar” OR “Dhahran” OR “Qatif” OR “Ras Tanura” OR “Khafji” OR “Kuwait” OR “Basrah” OR “Khor Al*Zubair” OR “Bandar Abbas” OR “Bushehr” OR “Qeshm Island” OR “Kish Island”
In complement with these three databases, the reference lists of included papers were also reviewed and screened for eligibility.
2.2 Study selection
As per the inclusion and exclusion criteria (Table 1), the review included English and/or Arabic language peer-reviewed journal articles that relied on remote sensing approaches in coastal zones of urban centers and nations around the Gulf that were published prior to 31 December 2022. Reviews, book chapters, policy reports, editorial material, and conference papers were excluded to confine the scope to high-quality and peer-reviewed original research (Meline, 2006).
2.3 Data screening and extraction
In line with best practice, we followed the Preferred Reporting Items for Systematic Reviews and Meta-Analyses (PRISMA) guidelines by tracking all journal articles identified, included, and excluded in a PRISMA flow diagram (Figure 2) (Page et al., 2021). The initial search yielded 651 hits: ScienceDirect (n = 370), Scopus (n = 218), Web of Science (n = 186), and Other (n = 47). Using the software COVIDENCE, 190 duplicates were removed, after which two independent reviewers (MA and AA) screened the papers for eligibility using, first, the title and abstract review and, secondly, the full text (Babineau, 2014). A total of 186 articles were included in the review, whereby we then extracted information on the study country, year, marine province, RS sensors used, study objective, GIS integration with fieldwork, classification, and change detection techniques, accuracy assessment methods, coastal habitat types (algal mats, corals, mangroves, and multiples), and main findings.
2.4 Data analysis
In accordance with the information extracted from the full-text screening, included studies were coded into four broad categories to cluster how remote sensing was deployed, whether it relates to primarily: 1) coastal urbanization (e.g., LULC), 2) coastal and marine habitats (e.g., coral reefs, seagrass, mangroves), 3) environmental pressures and state changes (e.g., oil spills, sea surface temperature) and 4) other. Under each category, relevant sub-categories were defined based on the specific coastal region (southern, western, northern, or Iranian coasts) or topic covered. The four mentioned coasts exhibit environmental divergence, representing unique subsections from an environmental perspective. The categories and subcategories, along with the extracted information, serve as the basis for the generation of figures and tables.
It is worth mentioning that the authors did not delve deeply into the ‘other’ category, which includes articles unrelated to the main focus areas but were included due to their relevance to RS coastal applications. This category covers diverse topics such as air quality (Beegum et al., 2018; Hamidi et al., 2014; Karami et al., 2021; Tariq et al., 2022), land surface temperature (Jaber, 2020; Lazzarini et al., 2013; Nassar et al., 2016; Radhi et al., 2013), geomorphological changes (Al-Hinai et al., 1987; Al-Hurban, 2014; Al-Rashidi and Al-Hurban, 2019; Al Hurban et al., 2008; Heidarzadeh et al., 2020; Miliaresis, 2013; Mirakhorlou et al., 2017), soil erosion and salinity (Abdelfattah et al., 2009; Alanazi and Ghrefat, 2013), meteorological factors (Nodej and Rezazadeh, 2018; Sultana and Nasrollahi, 2018; Zanchetta and Zecchetto, 2021), geometry and mapping bathometry (Albanai, 2021; Liu et al., 2019; Moeinkhah et al., 2019), and fish catches (Al-Abdulrazzak and Pauly, 2014).
3 Results
3.1 An overview of remote sensing literature on Gulf coasts
The first published study employing RS techniques to analyze Gulf coastal systems was published in 1971 (Szekielda et al., 1971), as part of a global assessment. The second paper was not published until 1986, focusing on the Iranian coast (Asanuma et al., 1986). This was followed by an additional study in 1987 centered on the KSA coast (Al-Hinai et al., 1987). After a 4-year gap, one paper in 1991 examined oil spills in KSA and Kuwait coasts (Cross, 1992), succeded by a paper on marine habitats in KSA in 1992 (Khan et al., 1992). The first paper on the UAE and Bahrain coasts was published in 1993 (Jensen et al., 1993; Zainal et al., 1993), and from 2003 to 2006, studies on Gulf coasts solely concentrated on the UAE (n = 6) (Al Jawdar et al., 2005; Althausen et al., 2003; Essa et al., 2005; Howari, 2004; Saito et al., 2003; Yagoub and Kolan, 2006). The first paper on Qatar’s coast appeared in 2007 (Al-Manni et al., 2007), while the inaugural study on Iraq was in 2009 (Hadeel et al., 2009). Temporally, the data illustrates a significant increase in published studies that utilize RS techniques to analyze Gulf coasts over the past five decades, indicating overall growth in research output on the topic within the Gulf region (Figure 3). Spatially, RS-based studies predominantly focused on the coasts of Iran (22.6%) and the UAE (22.6%), followed by KSA (11.8%) and Kuwait (11.3%). Qatar, Bahrain, and Iraq are the least studied of the Gulf nations. Additionally, 15.6% of papers take a broad perspective, analyzing the entire Gulf region. Figure 4 shows a word map of common themes identified in article text and titles.
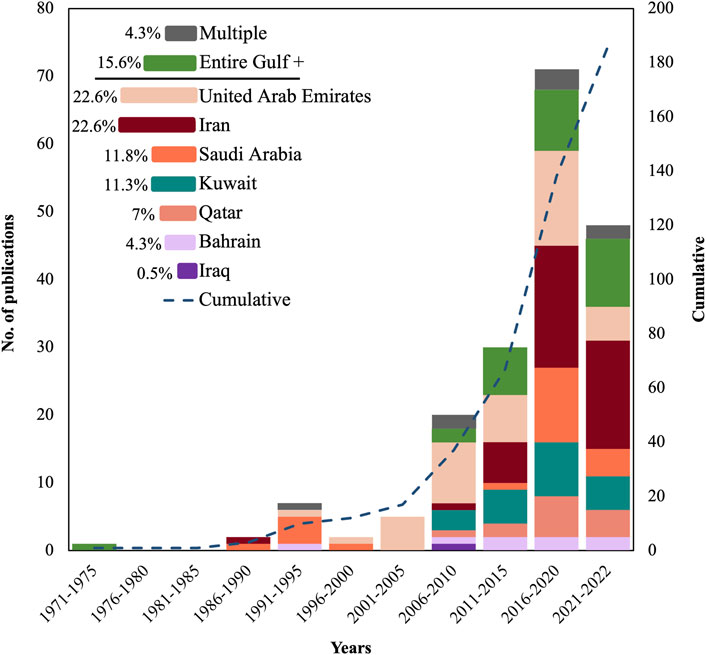
Figure 3. Historical overview of RS publications on the Gulf waters over time by country. Note: ‘Entire Gulf+' denotes study areas covering either the entire Gulf or the entire Gulf along with multiple water bodies. ‘Multiple’ signifies study areas spanning multiple countries.
In terms of topics explored, the most common focus among the 186 papers (35%) was environmental pressures. A significant portion of these papers were centered around Iran, the UAE, and the entire Gulf region. Over a quarter of papers (27%) focused on habitats, mainly originating from Iran and the UAE. A fifth of the papers (20%) were on coastal urbanization, with the UAE and Qatar having the highest number of papers on this topic. Nearly half (43.5%) of the papers used GIS techniques in their RS studies, with most being related to habitats and environmental pressures (Figure 5). The first paper to utilize GIS techniques was published in 1993 by Jensen et al. (1993) (Jensen et al., 1993), and since then, there has been a significant increase in research complementing this geospatial tool with RS. Regarding accuracy assessments of the produced maps, 37.1% of the papers evaluated the accuracy, with most of them being related to habitats, followed by coastal urbanization (Figure 5). The first paper that assessed the accuracy of maps produced using RS was published in 2003 (Saito et al., 2003). Over half (55.9%) of studies supplemented RS with field surveys, ground-truthing, or in situ data (Figure 5), primarily in papers concerning environmental pressures and habitats. The majority of the papers utilized optical sensors (50.5%), leveraging the strengths of different satellite platforms to analyze changes (Figure 5); remaining papers used multi-sensors (47.3%), radar (1.6%), and Lidar (0.5%).
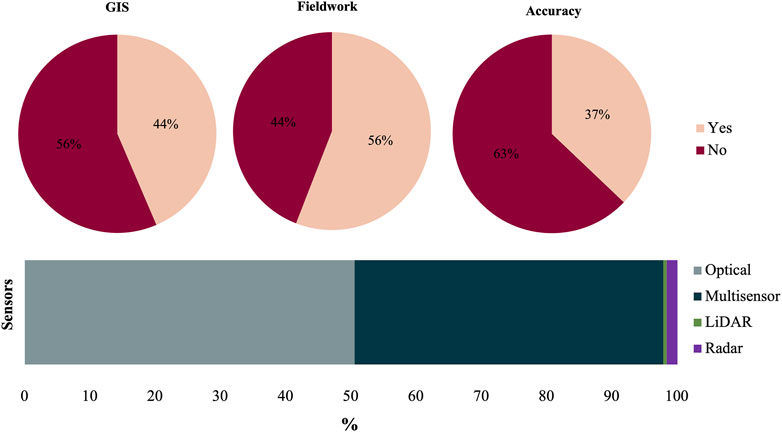
Figure 5. Summary of RS-based studies in the Gulf region. The distribution is based on (A) inclusion of a GIS component, (B) integration with field measurements, (C) reporting accuracy assessments, and (D) the types of sensors utilized.
For mapping the coastal urbanization, the most commonly used RS data were Landsat products with moderate spatial resolution, namely, Multispectral Scanner (MSS) (80 m), Thematic Mapper (TM) (30 m), Enhanced Thematic Mapper (ETM+) (30 m), and Operational Land Imager (OLI) (30 m) (Abdi and Nandipati, 2010; Abulibdeh et al., 2019; Al-Manni et al., 2007; Al-Ruzouq et al., 2014; AlDousari et al., 2022; Algharib, 2008; Aljaddani et al., 2022; Alqurashi et al., 2016; Baby, 2015; El-Kholei et al., 2019; Elhag, 2016; Hadeel et al., 2009; Jaad and Abdelghany, 2021; Kourosh Niya et al., 2019; Kwarteng and Chavez Jr, 1998; Liu et al., 2016; Loughland et al., 2007; Rahman, 2016; Rahman et al., 2017; Yagoub and Kolan, 2006). Some studies used other moderate spatial resolution sensors such as Satellite Pour l'Observation de la Terre (SPOT) (10 m) (Hashem and Balakrishnan, 2015) or a combination of Sentinel-2 (10 m) with Landsat (Subraelu et al., 2022), or Linear Imaging Self-Scanning Sensor (LISS-3) (24 m) with Landsat (Al Kuwari and Kaiser, 2011). Some studies used high spatial resolution RS data (Bualhamam, 2009), or combined high spatial resolution RS data, such as IKONOS (4 m) (Aljenaid S. S. et al., 2022), QuickBird (2.6 m) (Shandas et al., 2017), GeoEye-1 (0.46 m) (Dadras et al., 2015), DubaiSat (4 m) (Nassar et al., 2014), and aerial photos (Baby and Al-Sarawi, 2014; Dadras et al., 2015; Nassar et al., 2014), along with moderate spatial resolution RS data. Night-time light data, combined with Landsat imageries, can provide a valuable source of information for mapping the space-time dynamics of urban growth across very large areas (Alahmadi and Atkinson, 2019). The utilization of multi-source/multi-sensor data for the study of coastal urbanization in the Gulf can be observed as a prevailing trend in publications over the past decade (Al-Ruzouq et al., 2022; Al-Ruzouq et al., 2019; Alahmadi and Atkinson, 2019; Dadras et al., 2015; Hussein et al., 2017; Nassar et al., 2014; Shandas et al., 2017; Subraelu et al., 2022). Most studies utilized GIS techniques alongside RS techniques, with a smaller subset relying solely on GIS methodology (Hashem and Balakrishnan, 2015; Zainal et al., 2012).
Technically, when extracting the urban areas, caution is necessary when using RS data and approaches, a matter that has garnered significant attention among regional researchers. Harris (1985) suggested a statistical model to improve the classification of RS data in arid and urban areas in Al Jahra-Shuwaikh, Kuwait (Harris, 1985). Others have focused on enhancing high-frequency information in both bright desert and dark urban areas and utilizing specific band combinations to handle urban pixels (Kwarteng and Chavez Jr, 1998). Additionally, researchers have worked on selecting suitable change-detection techniques for the region (Sohl, 1999), applying advanced statistical image processing techniques to identify specific upland and coastal features (Althausen et al., 2003), proposing novel approaches to predict urban expansion (Boulila et al., 2021; Paul et al., 2021), and extracting road networks and urban objects (Ghandorh et al., 2022; Manandhar et al., 2019) using time-series satellite images.
A significant number of studies have utilized the conventional classification method, relying on pixel-based classification (PBC) techniques focusing on specific bands and/or retrieved indices. PBC encompasses diverse technical approaches, with the supervised classification method being predominantly favored by many researchers (Abdi and Nandipati, 2010; Aljenaid S. et al., 2022; Chouari, 2022; Hadeel et al., 2009; Hashem, 2015; Hussein et al., 2017; Kourosh Niya et al., 2019; Rahman, 2016; Rahman et al., 2017). The unsupervised classification method has also been employed (Algharib, 2008; Loughland et al., 2007), but there have been instances where researchers have harnessed the benefits of both supervised and unsupervised PBC techniques, thereby applying hybrid classification methods (Al Kuwari and Kaiser, 2011; Nassar et al., 2014; Shandas et al., 2017; Yagoub and Kolan, 2006). It is worth noting that the on-screen digitizing classification method, despite being a traditional approach, continues to be employed even in recent studies, particularly when the study area is relatively small in size (Al-Ruzouq et al., 2019; Kourosh Niya et al., 2019) or digitizing the coastlines (Subraelu et al., 2022). A limited number of recent studies have employed non-conventional classification methods, such as the object-oriented classification method (OBC) (Al-Ruzouq et al., 2022; Alqurashi et al., 2016) and machine learning classifiers (Al-Dousari et al., 2023; Jaad and Abdelghany, 2021). These methods go beyond considering solely the spectral attributes of urban features, as in traditional pixel-based classification methods. Instead, they also consider other relevant attributes such as shape, spatial relationships, and topographic characteristics of the urban features. For change detection, nearly all studies included in this review utilized the post-classification comparison method (PCC). However, a few exceptions were observed where researchers employed alternative approaches such as image differencing (Al-Ruzouq et al., 2014; Elmahdy and Mohamed, 2018; Loughland et al., 2007) and the Continuous Change Detection and Classification (CCDC) algorithm (Aljaddani et al., 2022).
3.2 Remote sensing of coastal urbanization and LULC
The Gulf region is home to 26 major urban centers, with a coastal population of about 15 million. This population represents approximately 8% of the total number of people living within 50 km of the coast across the entire region. The Arabian coastal zones of the Gulf, particularly in Kuwait, Bahrain, Qatar, and the UAE, are heavily urbanized, with most of the population residing here (Figure 6). These urban centers, once modest fishing communities that also held significance as trading hubs and pearl markets, have undergone transformational development into modern metropolises.
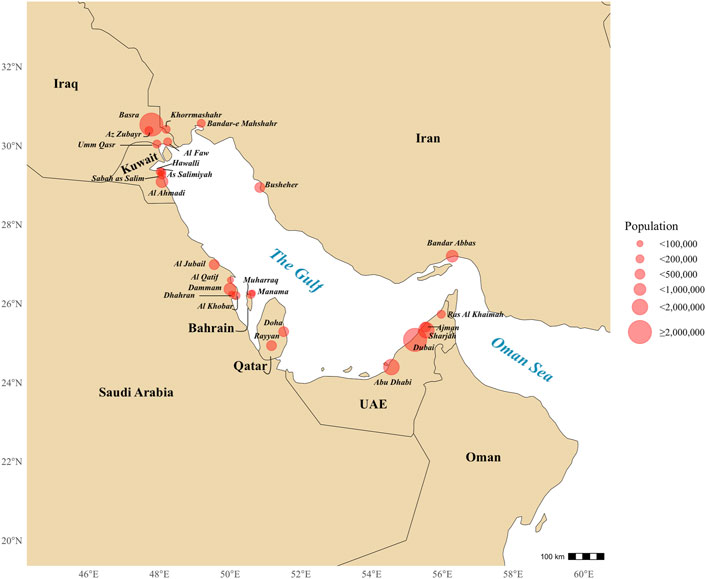
Figure 6. Populations of the major urban centers (≥100,000) located along the coastal zone of the Arabian Gulf.
3.2.1 Southern Gulf coast
The southern coast stretches from the Musandam peninsula in Oman across the UAE to Qatar. Despite only constituting 20% of the Gulf coastline, the southern coast stands out with a remarkable contribution to coastal urban change research in the region. The southern coast acquired most RS-based studies addressing coastal urbanization (39.5%) of all sub-regions. RS-based studies concentrated on seven urban centers situated along the southern coast of the Gulf, including Ras Al Khaimah (RAK), Ajman, Sharjah, Dubai, Abu Dhabi in the UAE, and Al Khore and Doha in Qatar (Supplementary Table S1).
Different time frames, sensors, and RS classification approaches were used in the aforementioned studies, making comparison of urban growth among urban centers difficult. Despite this, the collective period of studies covered an extensive range from 1971 to 2021, most relying on freely archived Landsat imagery and applying the PBC approach to map and/or detect changes in urban class. Study scopes were limited to city borders or local coasts. One study covered the entire UAE coast (Subraelu et al., 2022), while another compared the patterns of two cities on the southern coast, Dubai and Doha, after technically unifying the RS data and approach (Jaad and Abdelghany, 2021). Overall, all studies indicate urban growth in the southern coast’s urban centers, correlating with population trends. The pattern of the temporal increase in built-up areas across coastal zone sections shows that the ‘at-coastal’ section (∼2.5 km distance from the coast) exhibited the highest level of urbanization compared to the ‘near-coastal’ (∼20 km distance from the coast) and ‘far-coastal’ (∼46 km distance from the coast) sections. Urbanization began earlier in the ‘at-coastal’ and ‘near-coastal’ sections (Al-Ruzouq et al., 2022).
In terms of urbanization indicators or urban growth metrics, Ajman’s built-up areas experienced approximately a 12-fold increase over 40 years (1975–2015) (Al-Ruzouq et al., 2019). Abu Dhabi City expanded nearly seven and a half-fold over 31 years (1972–2003) (Loughland et al., 2007), while Doha’s built-up areas increased around six and a half times over 27 years (1990–2017) (Abulibdeh et al., 2019). The actual urban areas in square kilometers in the southern coast can provide further insights into urbanization in this coastal zone. For example, from the UAE’s federation in 1972–2000, the built-up and areas under development in the capital, Abu Dhabi, increased from approximately 20 km2–257 km2 in 2000 (Yagoub and Kolan, 2006). The built-up area in Dubai grew from 96 km2 in 2000 to 624 km2 in 2015 in just 15 years (2000–2015) (Elmahdy and Mohamed, 2018), while the urban area of Doha expanded from only 106 km2 in 1987 to approximately 421 km2 in 2013, gaining around 315 km2 over 26 years (1987–2013) (Shandas et al., 2017). In terms of annual urban growth, Dubai averaged an annual growth rate of 10% over 39 years (1972–2011) (Nassar et al., 2014), while the Doha metropolitan area exhibited an annual growth rate of approximately 5.5% in urban area over 26 years (1987–2013), with a peak growth rate of more than 13% over a 5-year period (1998–2003) (Shandas et al., 2017).
A significant surge in urban and industrial development along the southern coast of the Gulf has been observed since the turn of the millennium. In Dubai, the majority of urban growth occurred after 2000, with an average annual increase of 31 km2 per year over 11 years (2000–2011) (Nassar et al., 2014). Elmahdy and Mohamed (2018) calculated Dubai’s urban growth in the first 5 years of the new millennium to be approximately 234 km2, representing a growth rate of around 6% (2000–2005) (Elmahdy and Mohamed, 2018). Before the new millennium, Doha’s built-up area increased only by approximately 35 km2 over 30 years (1972–2002) (Al-Manni et al., 2007). However, the built-up areas of Doha increased substantially by around three times over only 13 years (1997–2010), and recreational spaces increased by more than four times over the same 13 years (Hashem and Balakrishnan, 2015).
Urbanization in the southern coast drives the demand for coastal land reclamation. Subraelu et al. (2022) revealed that cumulative land reclamation reached 119 km2 along the entire UAE coast, with Dubai accounting for over 70% (68 km2), including the development of Palm Deira and waterfront islands, followed by Abu Dhabi with 35 km2 and RAK with approximately 7 km2 (Subraelu et al., 2022). In another RS-based study conducted in Abu Dhabi in 2001, urban expansion was observed on new islands, including Lulu, Saadiyat, Yas, and Al Reem (Hussein et al., 2017). Coastal land reclamation in the southern coast of the Gulf before the new millennium was also investigated. For example, Abdi and Nandipati (2010) found that between 1972 and 2000, new land areas in Abu Dhabi waters amounted to around 16% of the total land area (Abdi and Nandipati, 2010). In Doha, reclamation and dredging activities added 10 km2 to coastal area between 1972 and 2002 (Al-Manni et al., 2007).
3.2.2 Western Gulf coast
The western coast includes the eastern KSA and Bahrain coasts. The RS-based papers addressing coastal urbanization in the western coast of the Gulf focused on five urban centers: Muharraq and the main island of Bahrain, and Al Khobar, Dammam, and Ras Tanura in KSA (Supplementary Table S1). Two papers conducted on the Bahrain landscape have the longest study periods, allowing for the investigation of more historical LULC classes: The first spans 45 years (1963–2008) (Zainal et al., 2012), and the other spans 56 years (1951–2007) (Modara et al., 2014). Most of the studies applied GIS tools for spatial analysis of coastal urbanization and retrieving urban growth metrics and was extensively used in the last two mentioned papers. Additionally, they used old aerial photographs and converted scanned analog images to digital data, then geo-referenced the images to understand historical dynamics in LULC changes and track urbanization trajectories. Similar to RS-based studies applied in the southern coast, most studies in the western coast utilized optical sensors, mainly the moderate spatial resolution of Landsat, and employed PBC methods to classify the images. However, one paper utilized high spatial resolution imagery from IKONOS (Modara et al., 2014), and another utilized calibrated DMSP-OLS nighttime lights imagery to extract more details (Alahmadi and Atkinson, 2019). Additionally, papers published in recent years applied OBC methods in their studies (Aljenaid S. S. et al., 2022; Alqurashi & Kumar, 2016; Chouari, 2022; Rahman, 2016; Rahman et al., 2017).
The built-up areas on Bahrain’s main islands exhibited an annual increasing trend of 7.5% over 34 years (1986–2020), covering approximately 40% of the main islands—specifically, Bahrain, Muharraq, Sitra, and Nabih Saleh (Aljenaid S. et al., 2022). Similarly, the urban expansion rate in Dammam was calculated to be 7.8% over 34 years (1985–2019) (Aljaddani et al., 2022). Dammam, the most populated city on the eastern KSA coast, saw its built-up area increase to approximately 29% of the total area over 24 years (1990–2014), and it is predicted that the urban area will encompass 55% of the city by 2026 (Rahman et al., 2017). In general, urban expansion can be observed in other western coast human settlements as well. For instance, Al Aba Oasis’ built-up area, west of Ras Tanura, increased from 1 km2 in 1985 to 2.5 km2 in 2000, and to more than 14 km2 by 2021 (Chouari, 2022).
The urban expansion pattern of Dammam has primarily grown inland towards the west, given its geographic constraints between Gulf Saihat to the north and Al Khobar and Dhahran to the south, limiting coastal growth (Aljaddani et al., 2022). Unlike the pattern observed in the southern coast (Subsection 3.2.1), the onset of the new millennium did not trigger extensive coastal urbanization on the Gulf’s western coast. In Al-Khobar, built-up areas saw a 117% increase over 11 years (1990–2001) and a 43.5% increase over the subsequent 12 years (2001–2013) (Rahman, 2016), while Dammam experienced a total urban expansion of approximately 42.5 km2 over 34 years (1985–2019), with more than 25 km2 occurring during the last 4 years interval (2015–2019) (Aljaddani et al., 2022). Broader geographical scope and specific time intervals enable an accurate analysis of coastal urbanization trajectories in the western coast of the Gulf. A study encompassing the entire eastern coast of KSA (1992–2013) with 7-year intervals revealed that urban areas increased by 31% (1992–1999), 19% (1999–2006), and 37% (2006–2013) (Alahmadi and Atkinson, 2019).
Coastal land reclamation studies were only reported for Bahrain on the western coast. The total extent of reclaimed coastal areas amounted to 91 km2 (1963–2008), with accelerated growth observed between 1997 and 2007, averaging 21 km2 annually (Zainal et al., 2012). The land area of Bahrain increased from 695 km2 (1989) to 814 km2 (2018) due to accelerated sea reclamation (El-Kholei et al., 2019). Specifically, Muharraq island’s coastline expanded from 35 km2 to 166 km2 (1951–2007) (Modara et al., 2014).
3.2.3 Northern Gulf coast
The northern coast comprises the Kuwait and Iraq coasts, with a total coastal length of 557 km (499 km for Kuwait and 58 km for Iraq). Most RS-based studies on urban growth in the northern Gulf coast used Landsat imagery and the PBC method to detect LULC classes in urban centers in Kuwait City and Basra City, Iraq. One study employed UAVs (Hassan et al., 2020), while Al-Dousari et al. (2022) utilized Sentinel-2 with a machine learning-based classification algorithm (AlDousari et al., 2022).
Three papers covered relatively short study periods ranging from 5 to 12 years (AlDousari et al., 2022; Algharib, 2008; Hadeel et al., 2009), while the three others covered longer durations of 30–40 years (AlDousari et al., 2022; Baby, 2015; Tao et al., 2023) (Supplementary Table S1). The main difference in urbanization patterns between Kuwait City and Basra is that urbanization in Kuwait is more likely to involve the conversion of bare soils to urban areas, similar to other GCC cities, while urban expansion in Basra involves a reduction in vegetation areas (Tao et al., 2023). Also, the annual urban growth in Basra was calculated at 1.2% over 13 years (1990–2003) (Hadeel et al., 2009), which is relatively limited and does not follow the rates observed in the southern and western coasts of the Gulf (see Subsections 3.2.1 and 3.2.2). Over 30 years (1990–2020), the built-up area in Basra increased by approximately 218 km2 (Tao et al., 2023), while Kuwait’s built-up area increased by 205 km2 over 39 years (1972–2011) (Baby, 2015). Considering the significant difference in population and area between the two countries, this represents a relatively large amount of urbanization in Kuwait. Baby (2015) found that urban expansion in Kuwait is polarizing towards the Gulf coasts, encompassing approximately 157 km2 over 18 years (1972–1990). Additionally, about 1.5 km2 of water was converted to urban areas, notably around Kuwait Tower and the artificial green island, which became the first artificial island in the Gulf and opened to the public in 1988.
In Kuwait, the urban area constitutes 32.5% of total area and has increased by 10% over only 13 years (1989–2001) (Algharib, 2008). By 2016, the urban area of Kuwait was 329 km2, expanding to 455 km2, which accounted for 53% of total area in 2021. The projected increase in the built-up area is expected to reach 582 km2 (68%) by 2026 (AlDousari et al., 2022).
3.2.4 Iranian Gulf coast
Iranian Gulf coastal cities have lower population densities and slower growth compared to most urban centers in the mainland of the Islamic Republic of Iran. Apart from Bandar Abbas, with a population exceeding half a million, only three other coastal towns—Bushehr, Bandar-e Mahshahr, and Khorramshahr—have populations exceeding 100,000 (Figure 6). RS-based studies dedicated to coastal urbanization along the Iranian Gulf coast have focused solely on two urban centers: one in Bandar Abbas (Dadras et al., 2015), the largest Iranian city on the Gulf, and another on Qeshm Island (Kourosh Niya et al., 2019), the largest island in the Gulf. The first paper covers a period of 56 years (1956–2012), while the other spans only 18 years (1996–2014) (Supplementary Table S1). The long-term study utilized multi-source/multi-sensor data with varying spatial resolutions, including aerial photos (Dadras et al., 2015). The other study employed time-series Landsat imagery and traditional on-screen digitizing techniques suitable for studying small areas like Qeshm Island (Kourosh Niya et al., 2019). Both studies demonstrated significant growth in the built-up class across the Iranian coast and over the study periods. Bandar Abbas’ built-up areas expanded from approximately 4 km2 in 1956 to around 50 square kilometers in 2012, representing a more than ten-fold increase (Dadras et al., 2015). The highest urban growth reached 123% between (1975–1987), with a minimum of 29% observed between (2001–2012). Overall, the main drivers of urbanization along the Iranian coast include high demand for construction, population growth, and migration from rural areas to urban centers.
3.3 Remote sensing of coastal and marine habitats
RS is applied to address environmental impacts of urbanization, including mapping and detecting the changes of coastal and marine habitats in the Gulf. Habitats such as mangrove forests (43%) have received the most attention, followed by a focus on multiple habitats (29%) (e.g., seagrass and mangroves and sabkha), nearshore subtidal habitats like coral reefs (20%), and lastly algal mats (sabkha) and tidal vegetation such as saltmarsh (8%) (Figure 7).
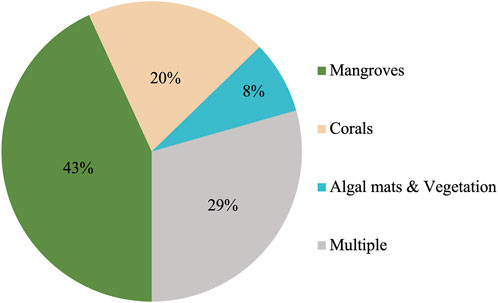
Figure 7. Percentage of type of coastal habitats assessed in the review. Seagrass beds were consistently studied alongside multiple habitats.
Supplementary Table S2 summarizes RS-based studies under review aimed to map and detect changes in habitats (mangroves, corals, algal mats, and seagrasses) along the coastal zones of the Gulf.
3.3.1 Mangroves
Mangrove forests are vital, as they provide wildlife habitat, store blue carbon in sediment, and protect shorelines (FAO, 2007; Kristensen et al., 2008). Regarding the geographic distribution of mangrove forests in the Gulf, Almahasheer (2018) identified and mapped the spatial coverage of mangroves along the entire coastlines of the Gulf in 2017, achieving an accuracy of 90.6%. The study revealed that there are around 7,000 mangrove communities in the region, with Iran accounting for 48% of the mangrove extent, followed by the UAE with 40%, Qatar with 5.6%, KSA with 4.5%, Kuwait with 0.6%, and Bahrain with 0.4% (Almahasheer, 2018). Urban development has led to increased nutrient and water exchange through dredged channels, benefiting mangroves and expanding habitat area (Burt, 2014; Burt et al., 2021). The majority of studies have focused on the Iranian coast (n = 15) and the UAE (n = 15) (Supplementary Table S2). The eastern coasts of KSA (Li et al., 2019; Sardi et al., 2020; Zayed Abdalla, 2022) and Bahrain (Aljenaid S. et al., 2022) have received relatively less attention in terms of RS-based research on mangroves, whereas there is currently no available RS-based research focused on the northern coast (Kuwait and Iraq), where mangroves do not naturally occur, although introduction efforts have been initiated in Kuwait (Loughland et al., 2020).
All RS-based studies of mangroves employed optical sensors, with few studies integrating active sensors performed in Qatar Peninsula (Butler et al., 2021; Butler et al., 2020) and Qeshm Island (Ghorbanian et al., 2022; Ghorbanian et al., 2021). The predominant sensor used was Landsat imagery (30 m resolution) (n = 25), followed by high-resolution data from satellites like worldview-2 and IKONOS (n = 11), and aerial photos (n = 3). A multi-source/multi-sensor approach to improve mapping and change detection in Gulf mangroves was evident in 13 papers. RS approaches for mangrove mapping and investigating environmental interactions in the region have been employed since the early 1990s (Jensen et al., 1993). This approach has successfully produced habitat maps for the northern Emirates of UAE (Sharjah, Ajman, Umm Al Quwain, and Ras Al Khaimah) (Mateos-Molina et al., 2020) and the northeastern region of Qatar (Warren et al., 2016), resources of value to coastal environmental and planning managers. Additionally, using the RS approach has successfully identified environmental threats to mangrove forests in Qatar (Butler et al., 2021; Butler et al., 2020).
The efforts of mapping and classifying local mangroves in the Gulf using RS approach were relatively focused on UAE’s and Iran’s coasts. Loughland et al. (2007) mapped Abu Dhabi’s coastal vegetation area and classified mangroves under broader vegetation classes together with intertidal vegetation and salt marsh. However, advanced RS techniques allow for more precise identification and mapping of mangroves, making them easily distinguishable from other coastal vegetation classes. These methods can quantify changes using various approaches such as single-band analysis or combined with single indices (e.g., narrow-band NDVI) and multi-indices approach (Hajjdiab et al., 2017; Li et al., 2019). Furthermore, mangrove forests can be categorized into more detailed subclasses based on their density (high, moderate, low) (Elmahdy and Mohamed, 2013), flooded/not flooded conditions (Saito et al., 2003), and levels of disturbances (Toosi et al., 2022). Using RS and GIS, mangrove areas in the northwestern Emirates were calculated to be approximately 20 km2 (Mateos-Molina et al., 2020). RS approach successfully detected and mapped even small mangrove areas (approximately 0.11 km2) on the coast of Bushehr using aerial photos combined with moderate spatial resolution Landsat imagery (Etemadi et al., 2021). Additionally, high overall accuracy mangrove maps (>93%) were provided using automated ML algorithms in the Google Earth Engine (GEE) cloud (Ghorbanian et al., 2021). ML classifiers have been reported to demonstrate robustness compared to traditional classifiers, achieving good overall accuracy in distinguishing between dense and dispersed mangroves in the UAE (Elmahdy et al., 2020). Furthermore, incorporating spatial landscape metrics enabled a more detailed classification of Qeshm Island’s mangroves, affected by human population growth, industrialization, and economic development, into five levels of disturbances: 202 km2 (very low), 276 km2 (low), 251 km2 (medium), 120 km2 (high), and 35 km2 (very high disturbance) (Toosi et al., 2022).
The majority of RS-based studies indicated a significant reduction in mangroves along the Gulf coasts, except in the area of Khor Al Bazam in the southwestern part of the UAE, where an increase likely due to plantation activities, closure of nearby shipyards, and increased public awareness regarding mangrove preservation (Howari et al., 2009). However, in Abu Dhabi’s coastal zone, there has been a reduction in wetlands, including mangroves, from approximately 1.2 km2–2.8 km2 over 28 years (1972–2000) due to coastal human activities such as reclamation and dredging (Yagoub and Kolan, 2006). Another RS-based study confirmed the reduction of mangroves in Abu Dhabi, indicating a decrease in mangrove area by −0.104% (∼2.9 km2) over 10 years (1990–2000) and a continued decrease of −0.091% (∼2.2 km2) over the next 6 years (2000–2006) (Elmahdy and Mohamed, 2013). Additionally, in the northern Emirates of the UAE, mangroves along the Gulf have shown a slight decrease over 3 decades (1990–2019) compared to those along the Gulf of Oman (Elmahdy et al., 2020). A recent study in Bahrain, covering a long period of 53 years (1967–2020), showed that the total mangrove area of Ras Tubli, Ras Sanad, and Sitra declined by 95%, from approximately 3.3 km2–0.5 km2 (Aljenaid S. et al., 2022). In the vicinity of Bahrain islands, particularly Tarut Bay, Qatif, another recent study investigated anthropogenic-induced geomorphological changes on mangroves. It observed a substantial reduction in mangroves from around 13 km2–4.5 km2 over 35 years (1986–2021), with certain mangrove sites experiencing complete loss due to continuous mangrove tree cutting (Zayed Abdalla, 2022).
Human-induced actions have adversely affected the distribution and density of mangroves, as observed in other RS-based studies. These include local cutting activities along the Qeshm island coast (Kourosh Niya et al., 2019), coastal urbanization activities along the UAE coast (Elmahdy et al., 2020; Subraelu et al., 2021), landfilling and reclamation activities from Al-Khafji to Dammam coast (Sardi et al., 2020), and oil transportation and refining activities in Ras Tanura port. Significant reduction in mangrove forests along the western Gulf coast, particularly in the northern Jazirat Al Kazzami and southern Gurmah island regions, has been observed, attributed to the 1991 Gulf war’ oil spill (Sardi et al., 2020). Worth mentioning, RS-based studies of Gulf mangroves have revealed their environmental benefits, including cooling surfaces in surrounding areas during daytime along the Dubai and Abu Dhabi coasts (Frey et al., 2007), and enabled estimation of carbon storage in the total biomass of mangroves in Bushehr, Iran (Amiri, 2021).
3.3.2 Coral reefs
Out of the 11 papers focused on coral reef habitats in the Gulf, six were from Iran (Kabiri et al., 2013a; Kabiri et al., 2013b; Kabiri et al., 2018; 2020; Kabiri et al., 2014; Mousavi et al., 2015), three from the UAE (Ben-Romdhane et al., 2016; Ben-Romdhane et al., 2020; Riegl and Purkis, 2009), and one each from Qatar (Riegl and Purkis, 2009), and Kuwait (Gholoum et al., 2019) (Supplementary Table S2). Most RS-based studies of Gulf coral reefs utilized high spatial resolution data from airborne and satellite-borne sensors such as worldview-2, IKONOS, QuickBird, and DubaiSat, as well as commercial drones. Limited studies adopted a multi-source/multi-sensor approach, with two incorporating active sensors like LiDAR to enhance reef and water property detail. Additionally, only a small number of papers extensively utilized GIS, one to assess the suitability for coral artificial reefs in Kish Island (Mousavi et al., 2015), and the other to conduct spatial analysis of Musandam’s coral reef distribution and proximity to human influence and activities (Mansour et al., 2017).
The first paper explicitly focusing on corals in the Gulf emerged in 2005, where coral in Dubai was classified into dense live, dense dead, and sparse coral (Riegl and Purkis, 2005). Subsequently, coral reefs, along with other delineated habitats, have been mapped using RS approaches in the northern Emirates of the UAE (Mateos-Molina et al., 2020) and Qatar (Warren et al., 2016). Additionally, along the southern coast of the Gulf, RS techniques revealed that reef communities collectively represent only 2% of the sea bottom in Qatar, with a significant presence on Halul Island (Butler et al., 2020). In the Iranian coast, considerable attention has been directed towards mapping and monitoring changes in the corals of Kish Island using RS. High spatial resolution imagery enabled the classification of corals at the species level rather than solely distinguishing between dense or sparse coral. The results of the RS approach revealed both the covered areas of Kish Island corals (∼4,500 m2 of the surveyed area) and the dominant coral types (Kabiri et al., 2014). Additionally, the RS approach has not only confirmed the presence of previously known coral areas in Qatar but also provided new details where corals had not been previously mapped (Warren et al., 2016).
The accuracy of coral maps depends not only on the spatial resolution of RS data but also on the spectral resolution. Mapping coral density on Kubbar Island in Kuwait, the sole RS-based reef study conducted on the northern coast of the Gulf, demonstrated that accuracy could be improved by incorporating high spectral resolution RS data and ancillary data (e.g., water turbidity, temperature, and salinity) (Gholoum et al., 2019). In Bahrain, coral habitats, among other marine habitats, were mapped with approximately 84% overall accuracy using moderate-resolution RS data from Landsat (Al-Jenaid et al., 2017). The use of high spatial resolution QuickBird data enabled mapping of the coral environment of Kish Island with an accuracy of 89.2% (Kabiri et al., 2013b). Utilizing images from commercial drones achieved higher accuracy compared to other methods and proved capable of differentiating between various coral types, visually detecting bleached corals, and cost-effectively identifying larger corals (Kabiri et al., 2020).
Detecting changes in corals, especially after coral bleaching and mortality events, is a significant research area. Butler et al. (2021) identified high-risk coral reef habitats in Qatar, including Al Zubarah, Al Ruwais, Al Khor, Al Dhakira, Mesaieed, Jazirat al Bushayriyah, and Halul Island (Butler et al., 2021). Analyzing reflectance changes in pre and post-bleaching images over 3 years (2005–2008) in Kish Island successfully identified 28% of bleached corals (Kabiri et al., 2013a). Change detection analysis of Delma Island corals in UAE suggested that the coral reefs in the UAE may exhibit greater resilience against thermal stress caused by global warming events, such as El Niño episodes (Ben-Romdhane et al., 2018).
3.3.3 Algal habitats
Macroalgae and cyanobacterial mats (together called algal mats here), as a land cover class, have typically been investigated alongside other coastal classes such as mangrove forests along the coasts of the Gulf, particularly the southern coast (UAE, Qatar) (n = 9) and in western coast (n = 3) (Supplementary Table S2). Algal communities have been assessed within sabkha ecosystems (salt flats) in Abu Dhabi using a multispectral visible/near-infrared camera (Hajjdiab et al., 2017). The study characterized two types of algae, namely, active and non-active algae, based on their spectral reflectance properties. Active algae exhibited high near-infrared (NIR) band reflectance and lower red band reflectance, while inactive algae showed low NIR band reflectance and higher red band reflectance (Hajjdiab et al., 2017).
Algal habitats have been mapped using moderate spatial resolution sensors such as Landsat (Al-Jenaid et al., 2017; Al-Thukair and Al-Hinai, 1993; Althausen et al., 2003; Elmahdy and Mohamed, 2013; Jensen et al., 1993; Khan et al., 1992). In the Abu Dhabi-Dubai coast, two classes of algal mats were distinguished (cyanophyceae and algal deposit), facing threats from industrial and urban development as well as oil spills, using a multi-source multi-sensor approach that included SPOT4 (20 m) and ASTER (15 m) (Saito et al., 2003). High spatial resolution RS data (<5 m) offer greater detail on algal mats distribution (shallow/deep algae) (Riegl and Purkis, 2005) and enhance separability of algal mats from other habitats (algae with coral/dense algae) (Warren et al., 2016). Ben-Romdhane et al. (2018) used DubaiSat imagery (5 m) to analyze changes in macroalgae-dominant habitats on Dalma Island between 2014 and 2016 (Ben-Romdhane et al., 2018), while Butler et al. (2020) combined worldview-2 (1.84 m) with LiDAR to distinguish between dense and sparse algae habitats along the coast of Qatar and Halul Island (Butler et al., 2020).
3.3.4 Seagrass
Seagrass beds in the Gulf have been examined alongside multiple other ecosystems in RS-based studies but lacked dedicated analyses despite being identified as a high-risk habitat in recent studies along the Qatar coast (Butler et al., 2021; Butler et al., 2020). Land reclamation and dredging activities in Bahrain, close to Qatar, have had a significant impact on seagrass beds, resulting in a 23.5% decrease, and on a mixed class of sands/seagrass/algae, causing a 34% reduction from 1963 to 2008 (Zainal et al., 2012). RS-based studies on seagrass were limited to the southern coast (n = 6) and the western coast (n = 4), with no RS paper published for seagrass in other Gulf coasts (Supplementary Table S2). A comprehensive RS-based approach along the coasts of the northern emirates of UAE revealed a seagrass coverage of approximately 21.6 km2 in Ras Al Khaimah and Umm Al Quwain (Mateos-Molina et al., 2020).
The resolution (spatial/spectral) of the sensors used is crucial for mapping and detecting changes in seagrass habitats. Howari et al. (2009) utilized a combination of high and moderate spatial resolution from two multispectral sensors and observed potential expansion of seagrass beds in the Khor Al Bazam area (Abu Dhabi) over 9 years (1994–2003), although accurately quantifying this increase posed challenges. Also, sparse and dense seagrass in Qatar on the southern Gulf coast were successfully mapped using worldview-2 satellite data (Warren et al., 2016). Utilizing worldview-2 satellite imagery with airborne LiDAR bathymetry, it was found that sparse seagrass covers around 14% of the Qatar shelf area, while dense seagrass occupies approximately 13% of the shelf (Butler et al., 2021; Butler et al., 2020).
3.4 Remote sensing of environmental pressures and state changes
In this section, we collate RS-based studies that examined the pressures and state changes in the Gulf marine environment linked to natural phenomena or anthropogenic activities. These studies encompass diverse aspects, including chlorophyll concentration and harmful algal blooms (29.3%), shoreline changes (15.4%), oil spills (15.4%), sea surface temperature (SST) changes (13.9%), water quality indicators (9.3%) such as turbidity and clarity, salinity (1.6%) and paper examining multiple pressures (7.7%) (Figure 8). Most chlorophyll concentration and harmful algal bloom studies were done on the entire Gulf region, while majority of the oil spill studies were done on KSA and Iran, with most shoreline change studies focused on Iran, followed by Kuwait (Supplementary Table S3).
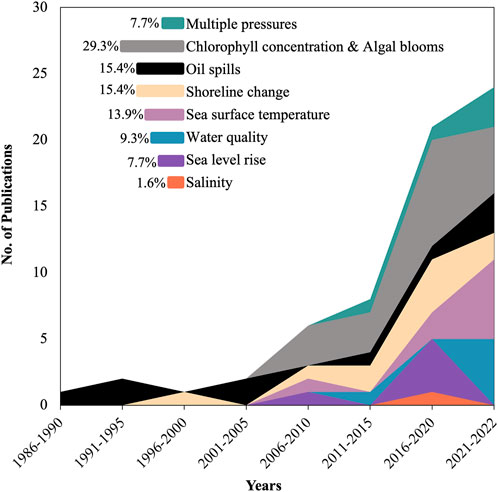
Figure 8. Number of peer-reviewed journal articles using RS for studying the pressure and state changes in the environment within the Gulf waters.
3.4.1 Oil spills in Gulf waters
The significant oil spill off the Kuwait coasts in January 1991, during the first Gulf War, heightened environmental awareness among researchers in the region regarding marine oil pollution. The resulting pollution posed a significant threat to various species and habitats, including birds and mangrove ecosystems, dugongs (sea cows), offshore coral islands, green turtles, shrimping grounds, fisheries, and humpback dolphins (Price et al., 1993; Sheppard et al., 2010). The Gulf War oil spill emphasized the necessity for practical RS methods to assess oil slick extents resulting from spills (Cross, 1992), develop environmental sensitivity indices for clean-up operations (Jensen et al., 1993), establish early warning systems to protect the marine environment of the Gulf States from oil pollution (Essa et al., 2005), and create spatial predictive models for oil spill probability (Mokhtari et al., 2015).
Addressing oil spill incidents and their environmental impacts using RS in the Gulf is primarily focused within the national borders of countries, namely, Iran (n = 4), KSA (n = 3), UAE (n = 3), Qatar (n = 1), and one paper addressed Kuwait and KSA maritime borders together (Cross, 1992). In terms of sensor usage, AVHRR data (1 km spatial resolution) was found to be a suitable information source during oil spill events (Asanuma et al., 1986; Cross, 1992). Utilizing various types of satellite imagery and employing a multi-source, multi-sensor approach appears to be a feasible and practical method for marine oil spill surveillance (Essa et al., 2005). GIS techniques have proven effective in constructing early warning systems to safeguard the Gulf marine environment from oil pollution, modeling oil spill probabilities, and identifying high-risk areas with active oil facilities and heavy ship traffic (Essa et al., 2005; Mokhtari et al., 2015). This aids in environmental risk assessment and detailed oil spill simulations. Recent studies along the KSA coast, from Al-Khafji to Dammam, including Abu Ali and Tarut islands, identified Safaniyah, Khursaniyah-Jubail, Tarut Bay, and the offshore islands as areas of highest priority for protection in the event of an oil spill (Sardi et al., 2020).
3.4.2 Turbidity and suspended sediments
RS-based studies on suspended sediments in the Gulf have been relatively recent, with only a few papers published in the last decade. These studies have primarily focused on the waters of Iran (n = 4), UAE (n = 1), and Kuwait (n = 1). The sensors used in these studies include moderate spatial resolution RS data such as Landsat and Sentinel-2 (n = 3), as well as coarse resolution sensors like MODIS and MERIS (n = 4), which provide pixel sizes sufficient for studying suspended sediments. Two papers highlighted the impact of coastal urbanization activities and changes in LULC on the increase of suspended sediments in Gulf waters. The first paper focused on coastal sedimentary processes in the Zohreh River delta, Iran, revealing that 80% of the sediment is transported to the subtidal zone of the Gulf, while 20% is deposited at the river mouth due to changes in land use and river bank erosion (Gharibreza et al., 2014). The second paper demonstrated that the construction of Palm Jumeirah artificial island in Dubai led to an increase in water reflectance within the 0.5–0.8 µm wavelength range, indicating elevated levels of suspended sediments (Mansourmoghaddam et al., 2022).
Mapping water depth in the northern Gulf coast using RS data offered valuable insights into water transparency and quality dynamics in extensive coastal environments (Alsahli and Nazeer, 2022). Kabiri (2022) utilized Landsat OLI and Sentinel-2 to assess water clarity indicators in the near-shore zone of Kish Island, Iran, estimating Secchi disk depth (Zsd) values as a measure of water clarity with satellite data. These findings are significant for monitoring and managing coastal water quality (Kabiri, 2022).
3.4.3 Chlorophyll concentration and harmful algal blooms
Regional and local factors like dust storms and anthropogenic activities can have a significant impact on transparency levels and influence phytoplankton growth in Gulf waters (Moradi, 2021). RS monitoring techniques for chlorophyll concentration and harmful algal blooms provide high-frequency and large-scale observations, enabling the assessment of nutrient levels and water quality (Al Shehhi et al., 2017a; Mahrad et al., 2020). Eight papers (out of 21) consider the entire Gulf in their RS approach, and nine papers covered the southern coast (with three of them in Dubai), Iran (n = 2), and Kuwait (n = 1). Using high spatial resolution RS data for mapping chlorophyll seems effective and can eliminate the need for costly in situ monitoring, as shown in Dubai Creek (Bachir, 2015); however, applying in large water coverage is not practical. Free satellite-derived products of chlorophyll concentrations offer valuable environmental insights into the factors influencing phytoplankton variability in the Gulf. Chlorophyll concentration information extracted over 15 years (2004–2019) across the Gulf using satellite-derived products revealed that the temporal seasonal averages change in annual cycles and that the trend decreased from 2004 to 2019, by about 0.7 mg/m3 (Albanai, 2022). Another study spanning 23 years (1998–2020) successfully identified phytoplankton bloom growth metrics, including the timings of initiation, peak, termination, and duration across the Gulf using satellite-derived ocean color chlorophyll (Zoljoodi et al., 2022). A recent study, supported by field observations, observed that the surface waters in the inner part of Kuwait Bay exhibited a dark brownish color, indicating the occurrence of a red tide in that area (Saburova et al., 2022).
However, satellite-derived chlorophyll concentration data overestimated field observations by up to ∼170–270% in Iranian coastal areas (Moradi, 2021). With its low spatial resolution, MODIS was used in 15 out of 21 papers for deriving chlorophyll concentration products. However, the coarse resolution of MODIS thermal bands (1 km) makes it less sensitive to local variations in temperature, which can influence chlorophyll estimation (Al Muhairi et al., 2009). To address this issue, one of the initial RS-based studies combined MODIS-derived chlorophyll data and high-resolution DubaiSat data (5 m) for detecting and monitoring red tide outbreaks along the southern coast of the Gulf (Muhairi et al., 2010). Additionally, Al Shehhi et al. (2017b) introduced a customized atmospheric correction model specifically designed for MODIS images, adapted to the dusty atmosphere of the region, to enhance chlorophyll concentration estimation across the entire Gulf (Al Shehhi et al., 2017a).
In a recent study in the Bushehr region, Iran, Tehrani et al. (2021) merged Landsat OLI with MODIS data and applied a chlorophyll extraction algorithm, successfully determining water surface chlorophyll concentration in the region. They noted higher chlorophyll levels in Bushehr Port compared to the Bushehr coast (Tehrani et al., 2021). It is worth mentioning that the fluorescence-based method using MERIS outperformed the chlorophyll anomaly approach in detecting harmful algal blooms and distinguishing between different algal species, successfully aiding in monitoring a prolonged harmful algal bloom outbreak in the Gulf region (Zhao et al., 2015).
3.4.4 Shoreline changes
A considerable number of shoreline change studies in the Gulf region have employed RS techniques (n = 15) to develop shoreline management plans (Dibajnia et al., 2012), assess and map vulnerabilities to environmental hazards (Mafi-Gholami et al., 2019), and measure coastal erosion and accretion (Aldogom et al., 2020). RS-based studies on Gulf shoreline changes focused on the Iranian coast (n = 6), Kuwait (n = 5), UAE (n = 3), and Tarut Island in the western coast of the Gulf (n = 1). The predominant studies utilized optical moderate spatial resolution RS data, particularly Landsat products, with two studies applying GIS functions for spatial analysis of coastal characteristics. Geospatial technologies, including RS, employ various techniques such as simple band subtraction, principal component analysis, and fuzzy logic to retrieve and measure detailed shoreline change metrics and coastal land dynamics (Ghanavati et al., 2008).
Based on coastal slope classification, it was found that about 25% of total shorelines in the Hormozgan province (∼2,240 km) exhibited high and very high vulnerability to environmental hazards and high risk of erosion (Mafi-Gholami et al., 2019). Based on erosion and accretion rates, the UAE shoreline was classified into five categories (high erosion, erosion, low erosion, accretion, and high accretion). It was found that the maximum erosion was measured at Umm Al Quwain and Ras Al Khaimah coasts, while the maximum accretion was measured at the Abu Dhabi coast (Aldogom et al., 2020). In the Hendijan River delta in Iran, the shoreline has migrated approximately 4 km into the Gulf, with an average annual advance rate of 90 m, while approximately 82 km2 of water near the delta has been filled with sediment (Ghanavati et al., 2008). In another Iranian river delta, the shoreline regression has remained relatively constant, with a rate of 0.6 m per year, as revealed in a study aimed at mapping and analyzing coastal sedimentary processes in the Zohreh River delta (Ghanavati et al., 2008). In the northern coast of the Gulf, the initial length of Kuwait’s coastline increased from ∼339 km to ∼479 km over a span of 39 years (1972–2011) (Baby, 2013). Another study found that over 40–50 years, sea levels rose by approximately 120–150 mm, exacerbating coastal erosion in southern Kuwait due to beach house construction near the high-water line and slipways blocking sand movement (Neelamani, 2018). In the southern coast of the Gulf, detailed coastal land dynamics over 31 years (1990–2021) across the seven emirates of the UAE have revealed observed retreat in Ajman and Umm Al Quwain, spanning 22 km of coastline. This area experienced a net erosion of 300 m2 and an annual erosion rate of 30 m2 per year over the past 21 years (2000–2021). Meanwhile, Dubai, Abu Dhabi, and Ras-Al Khaimah have undertaken extensive coastal reclamation projects, with Dubai leading the way by expanding its coastal areas by over 68 km2 since the early 2000s (Subraelu et al., 2022). In the western coast, significant transformations were observed in Tarut Bay and Tarut Island, KSA, leading to a notable decrease in the aquatic area from ∼393 km2 to ∼346 km2 over 35 years (1986–2021). This increase expanded the area of Tarut Island from about ∼22 km2–∼32 km2 during the same period due to human activities, particularly the extensive filling of shallow waters to facilitate urban development (Zayed Abdalla, 2022).
3.4.5 Sea surface temperature (SST)
RS technologies are effective for monitoring sea surface temperature (SST) to assess its impacts on coastal and marine ecosystems. Five out of 14 RS-based studies focused on SST across the entire Gulf, while the remainder examined the southern coast (n = 5), Iran (n = 3), and Kuwait (n = 1). Although it is low in spatial resolution, MODIS, and their derived SST products were used in eight out of 14 studies, while two studies employed high spatial resolution RS data such as DubaiSat, and three utilized moderate spatial resolution data from Landsat. Recently, 17 years of high spatial resolution SST data were compared with SST measurements from ten offshore locations in the shallow coastal waters of the UAE, revealing significant agreement between the two datasets (Nesterov et al., 2021).
Utilizing the thermal bands of RS data is crucial for extracting SST information and often requires calibration with ground measurements, particularly for low spatial resolution data, which can make the thermal bands less sensitive to local temperature variations (Al Muhairi et al., 2009). Atmospheric correction was applied to the thermal bands of AVHRR (NOAA-7 satellite) to obtain accurate SST measurements of the Gulf (Asanuma et al., 1986). MODIS’ SST products have been validated in Gulf waters, showing lower negative bias for both Aqua and Terra. MODIS-Aqua exhibited greater sensitivity to SST signals at night, while MODIS-Terra was more sensitive during the day (Saleh and Al-Anzi, 2021). In Kuwait and the northwest Gulf waters, it was found that the thermal band 10 of Landsat OLI is more accurate than thermal band 11 in monitoring SST, with a difference of ±1.1° (5.8%) (Albanai and Abdelfatah, 2022).
RS-based studies in the Gulf have observed a relationship between SST and other environmental pressures, noting that the high temperature of desalination brine has a local impact on surrounding water (Al Muhairi et al., 2009). A positive spatial correlation was found between satellite-derived chlorophyll concentrations and SST in shallow regions of the Iranian coast without any time lag. However, the correlation was negative in deeper regions with a time offset of 3–5 months (Moradi and Moradi, 2020). RS approaches are useful to detect the relationship between Gulf marine and coastal habitats and SST. A study employed high spatial resolution satellite images to detect changes in UAE coral reefs, concluding that live coral habitats exhibit tolerance to extremely high SST, suggesting resilience in the face of such temperature anomalies (Ben-Romdhane et al., 2018).
3.4.6 Water salinity
Salinity can change due to natural and human factors, and RS techniques can measure and assess these variations in sea surface salinity (SSS) (Kao et al., 2018). Only one RS-based study in the hyper-saline environment of the Gulf focused on SSS (Zhao et al., 2017). This study used Landsat OLI with 30 m spatial resolution to avoid limitations associated with retrieving products of coarse resolutions RS data. These limitations include viewing factors such as adjacent effects, mixed water-land pixels, and stray light contamination (Vanhellemont and Ruddick, 2015). Therefore, higher-resolution satellite imagery, like Landsat, is more suited to monitor water quality in the Gulf, especially in coastal areas. The researchers proposed an algorithm that showed good agreement between satellite-derived and in situ measurements, enabling the assessment of sea surface salinity (SSS) changes during extreme events compared to normal conditions (Zhao et al., 2017).
4 Discussion
With the number of people living in global coastal zones expected to grow from 2.15 billion in 2000 to 2.9 billion by 2,100, current pathways for the future development, research, and management of coastal zones, resources, and environments needs to be reformed. This increase in population pressures will be most acute near growing urban centers and, by consequence, lead to adverse effects for surrounding coastal ecosystems. To manage this complex and evolving dynamic, governments need reliable data that can be easily communicated to inform marine spatial management. Remote sensing, unlike traditional techniques, which can be intrusive, costly, and restricted in coverage, has been employed to monitor areas connected to coastal and marine environments globally; however, limited information has been available on the use of RS in the Arabian Gulf. Considering this knowledge gap, this systematic review synthesized published RS literature that focused on coastal zones of the eight states surrounding the Gulf, spanning five decades from 1971 through 2022.
This review offers the first comprehensive interpretation of how RS has been utilized along the coast of this rapidly developing region. The results of this review will serve as a useful reference tool for stakeholders from across a variety of disciplines, including environmental scientists, RS specialists, urban planners, coastal managers, and real estate developers. This review mapped the spatiotemporal patterns of the 186 publications extracted from three research databases and then subsequently categorized and sub-categorized how these publications used RS through the following four overarching categories: 1) coastal urbanization, 2) coastal and marine habitats, 3) environmental pressures and state changes, and 4) other. Results revealed that the largest proportion of papers applied RS techniques to explore topics related to environmental pressures and state changes (35%), with comparably lower proportions on coastal and marine habitats (27%) and coastal urbanization (20%). This greater concentration on environmental pressures and state changes can be attributed to several factors, such as major oil spill events (e.g., Gulf War), which elicited the first RS papers, and the region’s role as a major maritime conduit for global energy supplies which necessitated the monitoring of several environmental hazards (Yacoubi et al., 2024). The availability of open-access satellite data, along with its derived products, has also advantageously provided researchers with high-temporal frequency and large coverage scales to document environmental pressures more extensively. The smaller proportion of papers on coastal and marine habitats may be associated with the need for higher spatial resolution (Hedley et al., 2016), and the even smaller concentration of papers on coastal urbanization may be explained by the relatively recent emergence of coastal development projects in the Gulf within the past two decades.
4.1 Temporal and spatial trends
Temporally, the results illustrated that, despite slow growth from 1971 to the late 1990s, the number of peer-reviewed RS publications in the Gulf dramatically increased since 2001, signaling that this field has been gaining acceptance and credibility among the scientific community and with regional decision-makers. More than a third of RS-based studies (45.7%) were published in the last 3 years under review (2019–2022), the quantity of which will be expected to rise even further thanks to NASA and Sentinel’s open and free data access policy launched in 2008 and 2016, respectively, allowing access to Landsat and Sentinel products and archived images. This observed regional expansion aligns with the observations of Zhuang et al. (2013), who analyzed global RS literature from 1991 to 2010, revealing that the annual number of publications increased by 24.6% in those 2 decades, with a concentration in research in North America, Western Europe and East Asia (Zhuang et al., 2013). Our review indicates that research in the Gulf region is rapidly gaining ground and aligning with global trends in RS. This review extends the temporal range to 2022, expands knowledge on this understudied region, and introduces a coastal dimension to the discourse on urban RS which is often missing in the literature (Wentz et al., 2014). Additionally, our findings are consistent with those of de Araujo Barbosa et al. (2015), who, in their systematic review of RS applications in ecosystem services from 1960 to 2013, observed growth from 115 to 5,920 scientific publications and noted a relative deficiency in research within the Middle East, including the Arabian Gulf (de Araujo Barbosa et al., 2015). With the recent rapid growth in regional research, this data deficit is likely to be filled soon.
This temporal increase in RS publications appeared to be largely spatially siloed within national borders. Nearly half of the publications were split between Iran (22.6%) and the UAE (22.6%), with the remainder relating to KSA (11.8%) and Kuwait (11.3%). Qatar, Bahrain, Oman, and Iraq held the fewest publications. The review further demonstrated that most studies were conducted on the southern and western coasts of the Gulf, indicating that the large volume of RS publications may be connected to higher rates of coastal urbanization in populated centers along the coastlines of the UAE, Qatar, Bahrain, and KSA relative to the less populated northern and Iranian coasts. There are merits to national, city, and district-level applications of RS, as it can contextualize studies within their unique political, economic, and environmental conditions; however, scholars have consistently called for greater Gulf-wide RS applications to account for the shared nature of this water body (Zhao et al., 2017). Of the 15.6% RS-based studies on the wider Gulf region, most investigated large-scale variation in chlorophyll concentration and SST. The tendency to examine these two parameters at a broad scale may be connected to the availability of open-source RS data and derived products from NASA’s MODIS; but they fall short of producing sufficient transboundary papers on other environmental pressures and parameters and, therefore, restricts the production of a synoptic and regional view of the Gulf. Regional analyses would also enhance the accuracy of observations as estimations would be averaged over larger areas thereby reducing the impact of local anomalies and increasing the reliability of the data (Pitarch et al., 2016).
4.2 Coastal urbanization
This review illustrated that RS researchers have managed to extract coastal urban areas as pixels or objects in an arid and semi-arid region. While optical RS data in such an arid environment is minimally affected by cloud cover for most of the year, the ability to extract coastal urban areas serves as a great step forward in this field, given the considerable challenge associated with the spectral separability of urban areas from their surrounding soil and exposed rock (Althausen et al., 2003; Boulila et al., 2021; Ghandorh et al., 2022; Harris, 1985; Kwarteng and Chavez Jr, 1998; Manandhar et al., 2019; Paul et al., 2021; Sohl, 1999). Publications using RS in Gulf coastal urban zones relied on several RS techniques, including statistical modeling, change-detection methods, and advanced image processing to improve classification, predict urban expansion, and identify features such as road networks and urban objects from time-series satellite images. In terms of topics, studies highlighted a direct correlation between intensified human activities (e.g., reclamation, dredging, tree cutting) and widespread mangrove reduction: in Abu Dhabi from ∼1.2 km2 to ∼2.8 km2 over 28 years (1972–2000) (Yagoub and Kolan, 2006), in Tarut Bay, KSA from ∼13 km2 to ∼4.5 km2 over 35 years (1986–2021) (Zayed Abdalla, 2022), and in Ras Tubli, Ras Sanad, and Sitra, Bahrain by 95%, from 3.3 km2 to 0.5 km2 53 years (1967–2020) (Aljenaid S. et al., 2022). These findings echo that of Burt and Bartholomew (2019), who contend that coastal development has significantly degraded critical ecosystems that have historically offered goods and services to coastal communities for centuries (Burt and Bartholomew, 2019). This notion signals the growing demand for tools such as RS that can inform more effective and sustainable urban planning and development. Although RS applications on coastal urbanization constituted a fifth of all papers analyzed in this review, the number of suitability studies used for urban planning was alarmingly low at merely two papers (Mousavi et al., 2015). In practice, suitability studies such as environmental impact assessments (EIAs) are integrated into the management structure of nations surrounding the Gulf, but due to data confidentiality purposes, there is a lower inclination to publish the results. Organizations should strive to publicly distribute all data while also conducting cost-effective EIAs aided by RS.
4.3 Coastal and marine habitats
Land reclamation and dredging activities have adversely affected marine habitats in the Gulf, degrading the nursery areas for fish, feeding sites for both resident and migratory birds, and important habitats for key species such as dugongs, green turtles, and commercially important fishes (Bualhamam, 2009; Friis and Killilea, 2023; Zainal et al., 2012). Underscoring calls by scholars such as De Leeuw et al. (2010), Heumann (2011), and Pettorelli et al. (2014) to better employ remote sensing technologies in environmental management and conservation, this review demonstrated a significant variation in coverage among ecosystems (De Leeuw et al., 2010; Heumann, 2011; Pettorelli et al., 2014). More publications used RS methods to assess mangrove forest habitats (43%) compared to multiple habitats (29%), coral reefs (20%), and algal mats and vegetation (8%). This variation likely reflects both the ease of satellite detection (e.g., mangrove forests) and biodiversity value (e.g., coral reefs), leaving extremely extensive and biologically important habitats such as seagrass and algal mats understudied. To date, no regional reviews have examined the use of RS across multiple habitats in the Gulf. A notable review by Rondon et al. (2023), however, presents an insightful synthesis into remote sensing applications on mangroves in the Gulf Cooperation Council (GCC) from 2010 to 2022, revealing a decrease in mangrove cover in Saudi Arabia, Oman, Bahrain, and Kuwait, while noting an increase in Qatar and stable populations in the UAE due to afforestation and restoration initiatives (Howari et al., 2009). To develop a more comprehensive understanding of environmental change, an integrated monitoring program focusing on multiple habitat types across various spatial and temporal scales is warranted. Other notable barriers towards the integration of this technology in environmental management and conservation may also need to be addressed, including concerns around the cost and accuracy of data products, lack of interdisciplinary collaboration, as well as an absence of publicly accessible portals to facilitate informed decision-making (McCarthy et al., 2017; Schaeffer et al., 2013).
4.4 Environmental pressures and state changes
The current review demonstrated that RS literature in the Gulf has been applied to study a diverse range of topics on environmental pressures and state changes ranging from water quality (9%), including aspects such as turbidity and clarity, oil spills (15%), chlorophyll concentration and harmful algal blooms (29%) to shoreline changes (15%) and SST variability (14%), with a number of papers examining multiple pressures (8%) and salinity (2%). There were also noticeable geographic disparities on where these pressures were studied; for example, research on chlorophyll/harmful algal blooms and Gulf water quality received the most attention when considering the entire Arabian Gulf as the study area, followed by RS-based studies on SST. Additionally, oil spill studies were primarily conducted in Iran and KSA, with SST studies concentrated in the southern coast, specifically the UAE. Satellite-derived concentrations of chlorophyll, like that produced by MODIS, offer valuable environmental insights into the factors influencing phytoplankton variability in the Gulf (Alsahli and Nazeer, 2022). However, RS researchers in the Gulf identified errors in MODIS-derived chlorophyll mapping in coastal waters and proposed solutions by combining modeling techniques to improve accuracy.
Discharge from thermal desalination plants, which are widespread in the Gulf, has an impact not only on water quality but also on the thermal properties of the water (SST) and its trends, leading to adverse environmental impacts caused by heated effluents (Mahrad et al., 2020; Nesterov et al., 2021). Moreover, climate change will affect the Gulf region, as shown in recent RS-based studies that used MODIS data to map SST (Hereher, 2022). Of the reviewed papers, 15% investigated shoreline changes or sea level rise, contributing to understanding multi-decadal drivers of coastal change, evaluating potential climate change impacts, and supporting urban adaptation strategies in coastal areas (Vitousek et al., 2023). Certain studies created coastal models that predicted shoreline changes, which were subsequently used as guides for urban construction. Such guidelines enhance the efficacy of tackling problems like reduced sediment supply, extensive beach loss, and sea level rise, serving as a crucial manual for coastal managers. This integration aligns with the overarching development goals of promoting sustainable coastal management and enhancing resilience to environmental pressures. Adopting a synoptic regional approach to comprehensively study this semi-enclosed Gulf is crucial given the interconnectedness of its processes, such as river sediment dispersal from Iraq and Iran affecting water quality (Ghanavati et al., 2008; Gharibreza et al., 2014), and desalination plant discharges altering temperature and salinity profiles (Dawoud, 2012), consequently impacting habitats across boundaries.
4.5 Remote sensing techniques for coastal research in the gulf
The review also demonstrated that most papers used optical sensors (50.2%), while others used multi-sensors (47.5%), radar (1.6%), and Lidar (0.5%). Methodologically and technically, multi-source and multi-sensor data should be developed to maximize the benefits of any available RS data. Rondon et al. (2023), in their review on mangroves in the GCC, reported that results on the country-level distribution and cover change of mangroves varied among the literature due to the absence of a standardized methodology, variations in the resolution of satellite imagery and classification approaches. Over half of the studies complemented RS with field surveys, ground-truthing, or in situ data, primarily in papers concerning environmental pressures and habitats. This current review also highlighted the need for UAV ground truthing to validate satellite data. A third of all papers (37%) evaluated some component of accuracy; however, they frequently did not report the error matrix. This omission makes it challenging to evaluate the accuracy of the results and the subsequent measurements, comparisons, and recommendations that rely on maps produced from the data.
In general, the findings of this review cement the immense value geospatial technologies offer in deriving valuable ecological information from spectral information at a distance, at a low cost, and by eliminating in situ expenses, enabling the survey of areas difficult to access and providing repetitive coverage for periodic monitoring. RS is the only approach that can feasibly produce consistent and frequent data on large-scale settings such as the ocean and coastal provinces, but it does not negate the value of related approaches, such as in situ monitoring which is necessary to validate RS output. Only a few recent studies have utilized non-conventional classification techniques, such as the object-oriented classification method (OBC) (93, 109) and machine learning classifiers (91, 122). These approaches extend beyond the consideration of spectral attributes alone, as seen in traditional pixel-based classification methods. Rather, they also incorporate additional pertinent attributes such as shape, spatial relationships, and topographic characteristics of urban features. Scientists and practitioners should strive to use multiple methods as a complementary approach that can yield more robust insights that can also more effectively map complex phenomena like ecosystem services (Amani et al., 2021).
5 Conclusion and recommendations
With increasing coastal populations, growing investments in infrastructure and the continued use of land reclamation and dredging for constructing coastal mega-projects, RS technologies, including satellite, aerial, and drone-based tools, can ensure coastal managers can keep pace with the impact of rising population pressures and urbanization on coastal resources and habitats. To address the prior lack of comprehenive data on the use of RS applications to monitor coastal change in the Arabian Gulf, this study presents the first systematic review on the uses and applications of remote sensing along the coastal zones of the eight countries bordering the Gulf from 1971 to 2022. The review extracted, synthesized, categorized, and sub-categorized published peer-reviewed literature over the past five decades by searching through three of the largest research databases: Web of Science, Scopus, and ScienceDirect. The spatiotemporal patterns of the 186 included publications revealed a slow progression in RS coastal research in the first 3 decades, and a dramatic increase in productivity after 2001. Specifically, nearly half of all literature (46%) was published in the most recent 3 years alone (2019–2022), with around two-thirds (65%) published since 2002. Most publications, however, were spatially clustered in Iran and the UAE, followed by Saudi Arabia and Kuwait, with fewer studies focused on Qatar, Bahrain, Oman, and Iraq. Among the categories of coastal urbanization, coastal and marine habitats, and environment pressures and state changes, most RS applications were used to investigate the latter, and primarily on pressures related to harmful algal blooms, shoreline changes, and oil spills. Additionally, most papers used remote sensing to evaluate a single ecosystem (mangroves), with relatively less focused on coral reefs, algal mats, or multiple habitats. Overall, this review suggests the need to adopt policies and management tools that mitigate the negative impacts and risks associated with urbanization in the Gulf’s coastal and marine ecosystems.
As a result, the authors of this review encourage all stakeholders, from RS specialists and environmentalists to urban planners and policymakers, to collaboratively take advantage of this technology through the following 15 recommendations for policy and research (Figure 9). In terms of policies, countries around the Gulf should establish long-term regional monitoring systems using a combination of GIS and RS techniques coupled with in situ approaches for the iterative and transboundary mapping and conservation of coastal areas and hotspot zones (Hussein et al., 2017; Loughland et al., 2007; Yagoub and Kolan, 2006) as well as for the assessment of unsustainable land use patterns (Al Kuwari and Kaiser, 2011; Alqurashi & Kumar, 2016; Chouari, 2022; Hashem, 2015; Rahman, 2016; Shandas et al., 2017; Zainal et al., 1993; Zainal et al., 2012). Along with frequent synoptic monitoring, these eight countries should set up local interventions at ecologically suitable spatial scales to establish more effective systems for coastal management (Sale et al., 2014), which can inform decision-making processes seeking to minimize and mitigate anthropogenic-induced social and ecological consequences like dredging and reclamation (Abdi and Nandipati, 2010; Loughland et al., 2007; Yagoub and Kolan, 2006). These RS observations can then be used to corroborate regulation standards and set preventative measures (e.g., stop-work turbidity thresholds near dredging) for a range of industries involved in coastal development, including the real estate, maritime, and manufacturing industries.
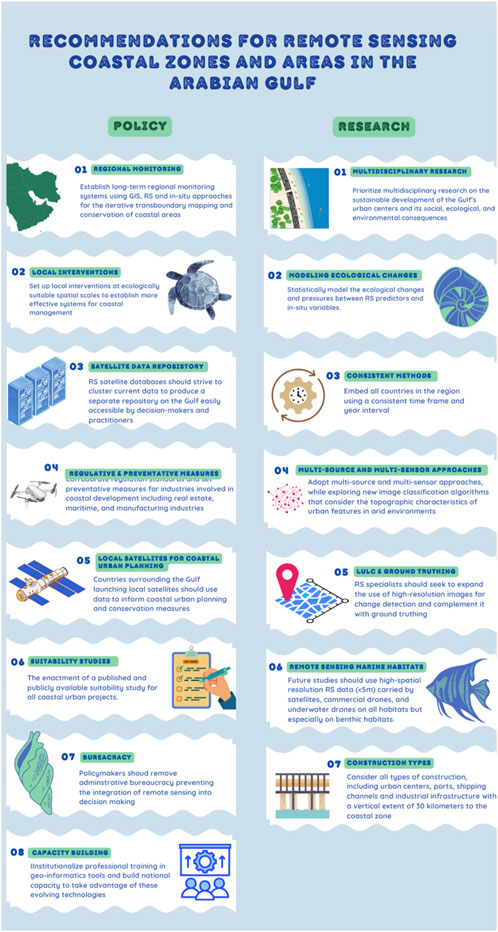
Figure 9. Policy and research recommendations for remote sensing coastal zones and areas in the Arabian Gulf.
Moreover, RS satellite databases should strive to consolidate current data to produce an open-access Gulf-focused repository that is easily accessible by decision-makers and practitioners (Al Kuwari and Kaiser, 2011) and compatible with GIS systems, which would be an invaluable resource for conservation and spatial planning (Mateos-Molina et al., 2021). To complement this repository, the recent emergence of space agencies in the countries surrounding the Gulf that are involved in developing and launching local satellites (Rubin, 2024) should use them to inform coastal urban planning and conservation measures and support sustainable development (Al Mansoori et al., 2019). Contingent for the increased deployment of RS to understand urban spatial planning and development would be the enactment of a published and publicly available suitability study for all coastal urban projects. As RS data becomes more available, policymakers should remove administrative bureaucracy to allow the integration of RS into decision-making processes for effective environmental governance (Cerbaro et al., 2020). Lastly, countries should institutionalize professional training in geo-informatics tools (Bualhamam, 2009) and build national capacity to take advantage of these evolving technologies to ensure the sustainable management of Gulf coasts.
While this review has shown that there has been rapid growth and evolution of RS-based research in the Gulf region, there remain knowledge gaps and areas in need of deeper attention that can be addressed through research. Concerning research methods and approaches, academia must prioritize multidisciplinary research on the sustainable development of the Gulf’s urban centers and its social, ecological, and environmental consequences. Scholars should venture beyond measuring, mapping, and monitoring coastal urbanization to statistically model environmental changes and pressures, particularly between RS predictors and in situ variables. This transition from descriptive to predictive models will enable the development of future scenarios for coastal vulnerability and the selection of sites for restoration of coastal and marine habitats and/or for urban expansion that are environmentally sustainable (Alahmadi and Atkinson, 2019; Alqurashi & Kumar, 2016; Hashem, 2015).
RS studies investigating coastal urbanization in the Gulf should strive to embed all coasts as one study area using a consistent time frame and year interval. This standardized approach would allow for a more reasonable comparison of the rates of change and the additions/losses of urban areas across the region. Additionally, they should accelerate the adoption of a multi-source and multi-sensor approach while exploring new image classification algorithms such as ML and DL that go beyond pixel-based classification to consider the spectral, shape, spatial, and topographic characteristics of urban features for more accurate LULC maps and predictions in arid environments (Aljaddani et al., 2022). RS experts are encouraged to broaden the utilization of high-resolution imagery for change detection within the ‘coastal urban’ classification, supplementing it with ground truthing. Simultaneously, there is a call for introducing a cost-effective remote sensing approach that upholds accurate LULC classification while monitoring coastal urbanization (Al-Ruzouq et al., 2014; Elmahdy and Mohamed, 2018). Future studies should use high-spatial resolution RS data (<5 m) carried by satellites, commercial drones, or even underwater drones on all habitats, especially focusing on benthic habitats. Such studies should also consider all types of construction, including urban centers, ports, shipping channels, industrial infrastructure, and marine construction facilities, with a vertical extent of 30 km to the coastal zone, to comprehensively understand the impact of human activity on the Gulf coastlines and sea. These research pursuits should, ultimately, foster a comprehensive understanding of historical urban growth dynamics, identify the causes and drivers by utilizing available historical aerial photos for LULC classification, quantify spatial and temporal growth, and address the demands for residential, commercial, and industrial lands (Alahmadi and Atkinson, 2019; Aljaddani et al., 2022; Chouari, 2022).
The implementation of the aforementioned policy and research recommendations can contribute to the sustainable management of the Gulf’s coastal and marine ecosystems while fostering the socio-economic development of its inhabitants residing along the coast. The information derived will not only aid in promoting collaboration among industrial and scientific communities within the Gulf region but also will act as a diplomatic catalyst towards enhancing relationships among nations surrounding the Gulf and facilitate their active participation in global environmental protection efforts.
Data availability statement
The raw data supporting the conclusions of this article will be made available by the authors upon request, without undue reservation.
Author contributions
BD: Conceptualization, Formal Analysis, Investigation, Methodology, Project administration, Software, Supervision, Validation, Visualization, Writing–original draft, Writing–review and editing. MA-M: Conceptualization, Formal Analysis, Investigation, Methodology, Project administration, Software, Validation, Visualization, Writing–original draft, Writing–review and editing. AA-G: Conceptualization, Formal Analysis, Investigation, Methodology, Project administration, Software, Visualization, Writing–original draft, Writing–review and editing. JB: Conceptualization, Funding acquisition, Supervision, Writing–original draft, Writing–review and editing.
Funding
The author(s) declare that financial support was received for the research, authorship, and/or publication of this article. This research was supported by the CITIES and Mubadala ACCESS Centers (grants 73-71210-ADHPG-CG001 and 73-71210-ADHPG-CG009, respectively), through funding from Tamakeen. MA-M and AA-G were also supported by a Kawader Research Assistantship from NYUAD.
Conflict of interest
The authors declare that the research was conducted in the absence of any commercial or financial relationships that could be construed as a potential conflict of interest.
Publisher’s note
All claims expressed in this article are solely those of the authors and do not necessarily represent those of their affiliated organizations, or those of the publisher, the editors and the reviewers. Any product that may be evaluated in this article, or claim that may be made by its manufacturer, is not guaranteed or endorsed by the publisher.
Supplementary material
The Supplementary Material for this article can be found online at: https://www.frontiersin.org/articles/10.3389/frsen.2024.1422910/full#supplementary-material
References
Abdelfattah, M. A., Shahid, S. A., and Othman, Y. R. (2009). Soil salinity mapping model developed using RS and GIS—a case study from Abu Dhabi, United Arab Emirates. Eur. J. Sci. Res. 26 (3), 342–351.
Abdi, A. M., and Nandipati, A. (2010). Abu Dhabi island: analysis of development and vegetation change using remote sensing (1972–2000). Adv. Earth Observation Glob. Change, 43–53. doi:10.1007/978-90-481-9085-0_4
Abulibdeh, A., Al-Awadhi, T., and Al-Barwani, M. (2019). Comparative analysis of the driving forces and spatiotemporal patterns of urbanisation in Muscat, Doha, and Dubai. Dev. Pract. 29 (5), 606–618. doi:10.1080/09614524.2019.1598335
Al-Abdulrazzak, D., and Pauly, D. (2014). Managing fisheries from space: google Earth improves estimates of distant fish catches. ICES J. Mar. Sci. 71 (3), 450–454. doi:10.1093/icesjms/fst178
Alahmadi, M., and Atkinson, P. M. (2019). Three-fold urban expansion in Saudi Arabia from 1992 to 2013 observed using calibrated DMSP-OLS night-time lights imagery. Remote Sens. 11 (19), 2266. doi:10.3390/rs11192266
Alanazi, H. A., and Ghrefat, H. A. (2013). Spectral analysis of multispectral Landsat 7 ETM+ and ASTER data for mapping land cover at Qurayah Sabkha, Northern Saudi Arabia. J. Indian Soc. Remote Sens. 41, 833–844. doi:10.1007/s12524-013-0291-2
Albanai, J. A. (2021). Mapping Kuwait bathymetry using passive multispectral remote sensing. Kuwait J. Sci. 48 (4). doi:10.48129/kjs.v48i4.8978
Albanai, J. A. (2022). Trend and dynamic of chlorophyll-a concentration over the Arabian Gulf: a long-term study using MODIS data (2004–2019). J. Eng. Res. 10, 2307–1877. doi:10.36909/jer.12213
Albanai, J. A., and Abdelfatah, S. A. (2022). Accuracy assessment for Landsat 8 thermal bands in measuring sea surface temperature over Kuwait and North West Arabian Gulf. Kuwait J. Sci. 49 (1). doi:10.48129/kjs.v49i1.9549
Aldogom, D., Albesher, S., Al Mansoori, S., and Nazzal, T. (2020). Assessing coastal land dynamics along UAE shoreline using GIS and remote sensing techniques. IOP Conf. Ser. Earth Environ. Sci. 540, 012031. doi:10.1088/1755-1315/540/1/012031
AlDousari, A. E., Kafy, A. A., Saha, M., Fattah, M. A., Almulhim, A. I., Al Rakib, A., et al. (2022). Modelling the impacts of land use/land cover changing pattern on urban thermal characteristics in Kuwait. Sustain. Cities Soc. 86, 104107. doi:10.1016/j.scs.2022.104107
Al-Dousari, A. E., Mishra, A., and Singh, S. (2023). Land use land cover change detection and urban sprawl prediction for Kuwait metropolitan region, using multi-layer perceptron neural networks (MLPNN). Egypt. J. Remote Sens. Space Sci. 26 (2), 381–392. doi:10.1016/j.ejrs.2023.05.003
Algharib, S. M. (2008). Spatial patterns of urban expansion in Kuwait city between 1989 and 2001 Kent State University.
Al-Hinai, K. G., Moore, J. M., and Bush, P. R. (1987). LANDSAT image enhancement study of possible submerged sand-dunes in the Arabian Gulf. Int. J. remote Sens. 8 (2), 251–258. doi:10.1080/01431168708948639
Al Hurban, A., El-Gamily, H., and El-Sammak, A. (2008). Geomorphic changes in Ras Al-Subiyah area, Kuwait. Environ. Geol. 54, 1377–1390. doi:10.1007/s00254-007-0919-z
Al-Hurban, A. E. (2014). Effects of recent anthropogenic activities on the surface deposits of Kuwait. Arabian J. Geosciences 7, 665–691. doi:10.1007/s12517-013-0866-9
Aljaddani, A. H., Song, X.-P., and Zhu, Z. (2022). Characterizing the patterns and trends of urban growth in Saudi arabia’s 13 capital cities using a landsat time series. Remote Sens. 14 (10), 2382. doi:10.3390/rs14102382
Al Jawdar, M. Y., Shiobara, M., and Onuma, T. (2005). Monitoring of coastal environment using satellite images in the United Arab Emirates. Dev. Earth Environ. Sci. 3, 13–29. doi:10.1016/S1571-9197(05)80026-6
Aljenaid, S., Abido, M., Redha, G. K., AlKhuzaei, M., Marsan, Y., Khamis, A. Q., et al. (2022a). Assessing the spatiotemporal changes, associated carbon stock, and potential emissions of mangroves in Bahrain using GIS and remote sensing data. Regional Stud. Mar. Sci. 52, 102282. doi:10.1016/j.rsma.2022.102282
Al-Jenaid, S., Ghoneim, E., Abido, M., AlWedhai, K., Mohammed, G., Mansoor, S., et al. (2017). Integrating remote sensing and field survey to map shallow water benthic habitat for the Kingdom of Bahrain. J. Environ. Sci. Eng. 6, 176–200. doi:10.17265/2162-5263/2017.04.002
Aljenaid, S. S., Kadhem, G. R., AlKhuzaei, M. F., and Alam, J. B. (2022b). Detecting and assessing the spatio-temporal land use land cover changes of Bahrain island during 1986–2020 using remote sensing and GIS. Earth Syst. Environ. 6 (4), 787–802. doi:10.1007/s41748-022-00315-z
Al Kuwari, N., and Kaiser, M. (2011). Impact of North Gas Field development on landuse/landcover changes at Al Khore, North Qatar, using remote sensing and GIS. Appl. Geogr. 31 (3), 1144–1153. doi:10.1016/j.apgeog.2011.03.001
Almahasheer, H. (2018). Spatial coverage of mangrove communities in the Arabian Gulf. Environ. Monit. Assess. 190 (2), 85. doi:10.1007/s10661-018-6472-2
Al-Manni, A. A. A., Abdu, A. S. A., Mohammed, N. A., and Al-Sheeb, A. E. (2007). Urban growth and land use change detection using remote sensing and geographic information system techniques in Doha City, State of Qatar. Arab Gulf J. Sci. Res. 25 (4), 190–198.
Al Mansoori, S., Al Shamsi, M., Al Maazmi, A., AlMarzouqi, F., and AlBesher, S. (2019). “Applications of khalifasat mission,” in IGARSS 2019-2019 IEEE International Geoscience and Remote Sensing Symposium, Yokohama, Japan, 28 July 2019 - 02 August 2019.
Al Muhairi, A., Ghedira, H., Al-Ahmad, H., Dawood, A., and Al-Mualla, M. (2009). “Exploring the potential of MODIS visible and thermal channels in monitoring and assessing the impact of desalination plant discharges in the Arabian Gulf. 2009,” in IEEE International Geoscience and Remote Sensing Symposium, Cape Town, South Africa, 12-17 July 2009.
Alqurashi, A. F., and Kumar, L. (2016). Spatiotemporal patterns of urban change and associated environmental impacts in five Saudi Arabian cities: a case study using remote sensing data. Habitat Int. 58, 75–88. doi:10.1016/j.habitatint.2016.10.001
Alqurashi, A. F., Kumar, L., and Sinha, P. (2016). Urban land cover change modelling using time-series satellite images: a case study of urban growth in five cities of Saudi Arabia. Remote Sens. 8 (10), 838. doi:10.3390/rs8100838
Al-Rashidi, S., and Al-Hurban, A. (2019). Geomorphological changes along the coastline of as Sabrina, northern Kuwait. Arabian J. Geosciences 12, 290–319. doi:10.1007/s12517-019-4466-1
Al-Ruzouq, R., and Shanableh, A. (2014). Multi-temporal satellite imagery for urban expansion assessment at Sharjah city/UAE. IOP Conf. Ser. Earth Environ. Sci. 20, 012010. doi:10.1088/1755-1315/20/1/012010
Al-Ruzouq, R., Shanableh, A., Khalil, M. A., Zeiada, W., Hamad, K., Abu Dabous, S., et al. (2022). Spatial and temporal inversion of land surface temperature along coastal cities in arid regions. Remote Sens. 14 (8), 1893. doi:10.3390/rs14081893
Al-Ruzouq, R., Yilmaz, A. G., Shanableh, A., Boharoun, Z. A., Khalil, M. A., and Imteaz, M. A. (2019). Spatio-temporal analysis of urban growth and its impact on floods in Ajman City, UAE. Environ. Monit. Assess. 191, 1–21. doi:10.1007/s10661-019-7854-9
Alsahli, M. M., and Nazeer, M. (2022). Modeling Secchi disk depth over the north arabian gulf waters using MODIS and MERIS images. PFG–Journal Photogrammetry, Remote Sens. Geoinformation Sci. 90 (2), 177–189. doi:10.1007/s41064-021-00189-2
Al Shehhi, M. R., Gherboudj, I., and Ghedira, H. (2017a). Satellites-based monitoring of harmful algal blooms for sustainable desalination. Desalination Sustain., 341–366. doi:10.1016/b978-0-12-809791-5.00009-2
Al Shehhi, M. R., Gherboudj, I., Zhao, J., and Ghedira, H. (2017b). Improved atmospheric correction and chlorophyll-a remote sensing models for turbid waters in a dusty environment. ISPRS J. Photogrammetry Remote Sens. 133, 46–60. doi:10.1016/j.isprsjprs.2017.09.011
Althausen, J., Kendall, C., Lakshmi, V., Alsharhan, A., and Whittle, G. (2003). “Using satellite imagery and GIS in the mapping of coastal landscapes in an arid environment: Khor Al Bazam, Western Abu Dhabi, United Arab Emirates,” in Desertification in the third millennium (Rotterdam: AA Balkema/Swets&Zeitlinger).
Al-Thukair, A. A., and Al-Hinai, K. (1993). Preliminary damage assessment of algal mats sites located in the western Gulf following the 1991 oil spill. Mar. Pollut. Bull. 27, 229–238. doi:10.1016/0025-326x(93)90029-j
Amani, M., Ghorbanian, A., Asgarimehr, M., Yekkehkhany, B., Moghimi, A., Jin, S., et al. (2021). Remote sensing systems for ocean: a review (Part 1: passive systems). IEEE J. Sel. Top. Appl. Earth Observations Remote Sens. 15, 210–234. doi:10.1109/jstars.2021.3130789
Amiri, F. (2021). Carbon storage potential of Avicennia marina as influenced by soil factors in National Park Nayband, South Coast of Iran. Acta Ecol. Sin. 41 (6), 566–574. doi:10.1016/j.chnaes.2021.06.005
Aromataris, E., and Pearson, A. (2014). The systematic review: an overview. AJN Am. J. Nurs. 114 (3), 53–58. doi:10.1097/01.naj.0000444496.24228.2c
Asanuma, I., Muneyama, K., Sasaki, Y., Iisaka, J., Yasuda, Y., and Emori, Y. (1986). Satellite thermal observation of oil slicks on the Persian Gulf. Remote Sens. Environ. 19 (2), 171–186. doi:10.1016/0034-4257(86)90070-2
Asghar Pilehvar, A. (2021). Spatial-geographical analysis of urbanization in Iran. Humanit. Soc. Sci. Commun. 8 (1), 1–12. doi:10.1057/s41599-021-00741-w
Babineau, J. (2014). Product review: covidence (systematic review software). J. Can. Health Libr. Association/Journal de l'Association des bibliothèques de la santé du Can. 35 (2), 68–71. doi:10.5596/c14-016
Baby, S. (2013). Findings and discussions on coastal evolution of Kuwait, review of laws and perspective of developed strategies. J. Image Graph. 1 (1), 17–33. doi:10.12720/joig.1.1.17-33
Baby, S. (2015). Monitoring the coastal land use land cover changes (LULCC) of Kuwait from spaceborne LANDSAT sensors.
Baby, S., and Al-Sarawi, M. (2014). Visual interpretation of pictorial data and reconnaissance survey to extract information on Kuwait’s coastal landscape.
Bachir, A. R. (2015). Modeling of chlorophyll-a and eutrophication indicators in the Dubai Creek area using remote sensing
Bakirman, T., Gumusay, M. U., and Tuney, I. (2016). “Mapping of the seagrass cover along the Mediterranean coast of Turkey using Landsat 8 OLI images,” in The International Archives of the Photogrammetry, Remote Sensing and Spatial Information Sciences 41, 1103–1105. doi:10.5194/isprs-archives-XLI-B8-1103-2016
Beegum, S. N., Gherboudj, I., Chaouch, N., Temimi, M., and Ghedira, H. (2018). Simulation and analysis of synoptic scale dust storms over the Arabian Peninsula. Atmos. Res. 199, 62–81. doi:10.1016/j.atmosres.2017.09.003
Bennett, M. K., Younes, N., and Joyce, K. (2020). Automating drone image processing to map coral reef substrates using google earth engine. Drones 4 (3), 50. doi:10.3390/drones4030050
Ben-Romdhane, H., Al-Musallami, M., Marpu, P. R., Ouarda, T. B., and Ghedira, H. (2018). Change detection using remote sensing in a reef environment of the UAE during the extreme event of El Niño 2015–2016. Int. J. remote Sens. 39 (19), 6358–6382. doi:10.1080/01431161.2018.1460502
Ben-Romdhane, H., Marpu, P. R., Ouarda, T. B., and Ghedira, H. (2016). Corals and benthic habitat mapping using DubaiSat-2: a spectral-spatial approach applied to Dalma Island, UAE (Arabian Gulf). Remote Sens. Lett. 7 (8), 781–789. doi:10.1080/2150704x.2016.1187317
Ben-Romdhane, H., Ouarda, T. B., Marpu, P., Rajan, A., Bugla, I., Perry, R. J., et al. (2020). Studying coral reef patterns in UAE waters using panel data analysis and multinomial logit and probit models. Ecol. Indic. 112, 106050. doi:10.1016/j.ecolind.2019.106050
Boulila, W., Ghandorh, H., Khan, M. A., Ahmed, F., and Ahmad, J. (2021). A novel CNN-LSTM-based approach to predict urban expansion. Ecol. Inf. 64, 101325. doi:10.1016/j.ecoinf.2021.101325
Bualhamam, M. R. (2009). The study of urban growth impact in tourism area using remote sensing and GIS technique for north part of the UAE. J. Geogr. Regional Plan. 2 (6), 166.
Bugnot, A., Mayer-Pinto, M., Airoldi, L., Heery, E., Johnston, E., Critchley, L., et al. (2021). Current and projected global extent of marine built structures. Nat. Sustain. 4 (1), 33–41. doi:10.1038/s41893-020-00595-1
Burt, J. A. (2014). The environmental costs of coastal urbanization in the Arabian Gulf. City 18 (6), 760–770. doi:10.1080/13604813.2014.962889
Burt, J. A., and Bartholomew, A. (2019). Towards more sustainable coastal development in the Arabian Gulf: opportunities for ecological engineering in an urbanized seascape. Mar. Pollut. Bull. 142, 93–102. doi:10.1016/j.marpolbul.2019.03.024
Burt, J. A., Ben-Hamadou, R., Abdel-Moati, M. A., Fanning, L., Kaitibie, S., Al-Jamali, F., et al. (2017). Improving management of future coastal development in Qatar through ecosystem-based management approaches. Ocean and Coast. Manag. 148, 171–181. doi:10.1016/j.ocecoaman.2017.08.006
Burt, J. A., Feary, D. A., Bauman, A. G., Usseglio, P., Cavalcante, G. H., and Sale, P. F. (2011). Biogeographic patterns of reef fish community structure in the northeastern Arabian Peninsula. ICES J. Mar. Sci. 68 (9), 1875–1883. doi:10.1093/icesjms/fsr129
Burt, J. A., Killilea, M., and Rademacher, A. (2021). Unexpected nature? Proliferating mangroves on the coast of Abu Dhabi.
Burt, J. A., and Paparella, F. (2023). “The marine environment of the emirates,” in A natural history of the emirates (Springer), 95–117.
Butler, J. D., Purkis, L. M., Purkis, S. J., Yousif, R., and Al-Shaikh, I. (2021). A benthic habitat sensitivity analysis of Qatar's coastal zone. Mar. Pollut. Bull. 167, 112333. doi:10.1016/j.marpolbul.2021.112333
Butler, J. D., Purkis, S. J., Yousif, R., Al-Shaikh, I., and Warren, C. (2020). A high-resolution remotely sensed benthic habitat map of the Qatari coastal zone. Mar. Pollut. Bull. 160, 111634. doi:10.1016/j.marpolbul.2020.111634
Cerbaro, M., Morse, S., Murphy, R., Lynch, J., and Griffiths, G. (2020). Challenges in using earth observation (EO) data to support environmental management in Brazil. Sustainability 12 (24), 10411. doi:10.3390/su122410411
Chen, J., Theller, L., Gitau, M. W., Engel, B. A., and Harbor, J. M. (2017). Urbanization impacts on surface runoff of the contiguous United States. J. Environ. Manag. 187, 470–481. doi:10.1016/j.jenvman.2016.11.017
Chouari, W. (2022). Land use/land cover change detection in the wetlands. A case study: Al-Aba Oasis, west of Ras Tanura, kingdom of Saudi Arabia. J. Water Land Dev., 229–237. doi:10.24425/jwld.2022.140802
Cross, A. (1992). Monitoring marine oil pollution using AVHRR data: observations off the coast of Kuwait and Saudi Arabia during January 1991. Int. J. remote Sens. 13 (4), 781–788. doi:10.1080/01431169208904152
Cunning, R., Silverstein, R. N., Barnes, B. B., and Baker, A. C. (2019). Extensive coral mortality and critical habitat loss following dredging and their association with remotely-sensed sediment plumes. Mar. Pollut. Bull. 145, 185–199. doi:10.1016/j.marpolbul.2019.05.027
Dadras, M., Shafri, H. Z., Ahmad, N., Pradhan, B., and Safarpour, S. (2015). Spatio-temporal analysis of urban growth from remote sensing data in Bandar Abbas city, Iran. Egypt. J. Remote Sens. Space Sci. 18 (1), 35–52. doi:10.1016/j.ejrs.2015.03.005
Dahy, B., Issa, S., and Saleous, N. (2021). “A review of land change modelling techniques using remote sensing and gis,” in 42nd Asian Conference on Remote Sensing, ACRS 2021.
Dahy, B., Issa, S., and Saleous, N. (2022). Random forest for classifying and monitoring 50 Years of vegetation dynamics in three desert cities of the uae. The international Archives of the photogrammetry. Remote Sens. Spatial Inf. Sci. 43, 69–76. doi:10.5194/isprs-archives-XLIII-B3-2022-69-2022
Daoudi, M., and Niang, A. J. (2021). Detection of shoreline changes along the coast of Jeddah and its impact on the geomorphological system using GIS techniques and remote sensing data (1951–2018). Arabian J. Geosci. 14 (13), 1265. doi:10.1007/s12517-021-07605-2
Dawoud, M. A. (2012). Environmental impacts of seawater desalination: arabian Gulf case study. Int. J. Environ. Sustain. 1 (3). doi:10.24102/ijes.v1i3.96
de Araujo Barbosa, C. C., Atkinson, P. M., and Dearing, J. A. (2015). Remote sensing of ecosystem services: a systematic review. Ecol. Indic. 52, 430–443. doi:10.1016/j.ecolind.2015.01.007
De Leeuw, J., Georgiadou, Y., Kerle, N., De Gier, A., Inoue, Y., Ferwerda, J., et al. (2010). The function of remote sensing in support of environmental policy. Remote Sens. 2 (7), 1731–1750. doi:10.3390/rs2071731
Dibajnia, M., Soltanpour, M., Vafai, F., Shoushtari, S. M. H. J., and Kebriaee, A. (2012). A shoreline management plan for Iranian coastlines. Ocean and Coast. Manag. 63, 1–15. doi:10.1016/j.ocecoaman.2012.02.012
Duan, H., Zhang, H., Huang, Q., Zhang, Y., Hu, M., Niu, Y., et al. (2016). Characterization and environmental impact analysis of sea land reclamation activities in China. Ocean and Coast. Manag. 130, 128–137. doi:10.1016/j.ocecoaman.2016.06.006
El-Arifi, S. A. (1986). The nature of urbanization in the gulf countries. GeoJournal 13, 223–235. doi:10.1007/bf00190508
Elhag, M. (2016). Detection of temporal changes of eastern coast of Saudi Arabia for better natural resources management.
El-Kholei, A. O., Aljenaid, S. S., and Kadhem, G. M. (2019). Urban transformations and contested spaces in the kingdom of Bahrain. Open House Int. 44 (4), 55–63. doi:10.1108/ohi-04-2019-b0008
Elmahdy, S. I., Ali, T. A., Mohamed, M. M., Howari, F. M., Abouleish, M., and Simonet, D. (2020). Spatiotemporal mapping and monitoring of mangrove forests changes from 1990 to 2019 in the Northern Emirates, UAE using random forest, Kernel logistic regression and Naive Bayes Tree models. Front. Environ. Sci. 8, 102. doi:10.3389/fenvs.2020.00102
Elmahdy, S. I., and Mohamed, M. M. (2013). Change detection and mapping of mangrove using multi-temporal remote sensing data: a case study of Abu Dhabi, UAE. J. Geomatics 7 (1), 41–45.
Elmahdy, S. I., and Mohamed, M. M. (2018). Monitoring and analysing the Emirate of Dubai’s land use/land cover changes: an integrated, low-cost remote sensing approach. Int. J. Digital Earth 11 (11), 1132–1150. doi:10.1080/17538947.2017.1379563
Elso, M. Z., Manent, P., Luque, A., Ramdani, M., and Robaina, R. R. (2017). Genetic description and remote sensing techniques as management tools for Zostera noltii seagrass populations along the Atlantic Moroccan coast. J. Coastal Res. 33 (1), 78–87. doi:10.2112/JCOASTRES-D-15-00111.1
Essa, S., Harahsheh, H., Shiobara, M., and Nishidai, T. (2005). Operational remote sensing for the detection and monitoring of oil pollution in the Arabian Gulf: case studies from the United Arab Emirates. Dev. earth Environ. Sci. 3, 31–48. doi:10.1016/S1571-9197(05)80027-8
Etemadi, H., Smoak, J. M., and Abbasi, E. (2021). Spatiotemporal pattern of degradation in arid mangrove forests of the Northern Persian Gulf. Oceanologia 63 (1), 99–114. doi:10.1016/j.oceano.2020.10.003
Frey, C., Rigo, G., and Parlow, E. (2007). Urban radiation balance of two coastal cities in a hot and dry environment. Int. J. remote Sens. 28 (12), 2695–2712. doi:10.1080/01431160600993389
Friis, G., and Burt, J. A. (2020). Evolution of mangrove research in an extreme environment: historical trends and future opportunities in Arabia. Ocean and Coast. Manag. 195, 105288. doi:10.1016/j.ocecoaman.2020.105288
Friis, G., and Killilea, M. E. (2023). “Mangrove ecosystems of the United Arab Emirates,” in A natural history of the emirates (Springer), 217–240.
Ghanavati, E., Firouzabadi, P., Jangi, A., and Khosravi, S. (2008). Monitoring geomorphologic changes using Landsat TM and ETM+ data in the Hendijan River delta, southwest Iran. Int. J. remote Sens. 29 (4), 945–959. doi:10.1080/01431160701294679
Ghandorh, H., Boulila, W., Masood, S., Koubaa, A., Ahmed, F., and Ahmad, J. (2022). Semantic segmentation and edge detection—approach to road detection in very high resolution satellite images. Remote Sens. 14 (3), 613. doi:10.3390/rs14030613
Gharibreza, M., Habibi, A., Imamjomeh, S. R., and Ashraf, M. A. (2014). Coastal processes and sedimentary facies in the Zohreh River Delta (northern Persian gulf). Catena 122, 150–158. doi:10.1016/j.catena.2014.06.010
Gholoum, M., Bruce, D., and Alhazeem, S. (2019). A new image classification approach for mapping coral density in State of Kuwait using high spatial resolution satellite images. Int. J. remote Sens. 40 (12), 4787–4816. doi:10.1080/01431161.2019.1574991
Ghorbanian, A., Ahmadi, S. A., Amani, M., Mohammadzadeh, A., and Jamali, S. (2022). Application of artificial neural networks for mangrove mapping using multi-temporal and multi-source remote sensing imagery. Water 14 (2), 244. doi:10.3390/w14020244
Ghorbanian, A., Zaghian, S., Asiyabi, R. M., Amani, M., Mohammadzadeh, A., and Jamali, S. (2021). Mangrove ecosystem mapping using Sentinel-1 and Sentinel-2 satellite images and random forest algorithm in Google Earth Engine. Remote Sens. 13 (13), 2565. doi:10.3390/rs13132565
Hadeel, A., Jabbar, M., and Chen, X. (2009). Application of remote sensing and GIS to the study of land use/cover change and urbanization expansion in Basrah province, southern Iraq. Geo-spatial Inf. Sci. 12 (2), 135–141. doi:10.1007/s11806-009-0244-7
Hajjdiab, H., Farok, H., and Howari, F. M. (2017). “The application of narrow-band NDVI as a tool for mapping mangrove environment in Abu Dhabi,” in Proceedings of the 9th International Conference on Machine Learning and Computing.
Hamidi, M., Kavianpour, M. R., and Shao, Y. (2014). Numerical simulation of dust events in the Middle East. Aeolian Res. 13, 59–70. doi:10.1016/j.aeolia.2014.02.002
Harris, R. (1985). Contextual classification post-processing of Landsat data using a probabilistic relaxation model. Int. J. remote Sens. 6 (6), 847–866. doi:10.1080/01431168508948509
Hashem, N. (2015). Assessing spatial equality of urban green spaces provision: a case study of Greater Doha in Qatar. Local Environ. 20 (3), 386–399. doi:10.1080/13549839.2013.855182
Hashem, N., and Balakrishnan, P. (2015). Change analysis of land use/land cover and modelling urban growth in Greater Doha, Qatar. Ann. GIS 21 (3), 233–247. doi:10.1080/19475683.2014.992369
Hassan, A., G Almatar, M., Torab, M., and Allen, C. D. (2020). Environmental urban plan for Failaka Island, Kuwait: a study in urban geomorphology. Sustainability 12 (17), 7125. doi:10.3390/su12177125
Hedley, J. D., Roelfsema, C. M., Chollett, I., Harborne, A. R., Heron, S. F., J. Weeks, S., et al. (2016). Remote sensing of coral reefs for monitoring and management: a review. Remote Sens. 8 (2), 118. doi:10.3390/rs8020118
Heidarzadeh, M., Šepić, J., Rabinovich, A., Allahyar, M., Soltanpour, A., and Tavakoli, F. (2020). Meteorological tsunami of 19 March 2017 in the Persian Gulf: observations and analyses. Pure Appl. Geophys. 177, 1231–1259. doi:10.1007/s00024-019-02263-8
Hereher, M. E. (2022). Climate change during the third millennium—the gulf cooperation Council countries. Sustainability 14 (21), 14181. doi:10.3390/su142114181
Heumann, B. W. (2011). Satellite remote sensing of mangrove forests: recent advances and future opportunities. Prog. Phys. Geogr. 35 (1), 87–108. doi:10.1177/0309133310385371
Hill, V. J., Zimmerman, R. C., Bissett, W. P., Dierssen, H., and Kohler, D. D. (2014). Evaluating light availability, seagrass biomass, and productivity using hyperspectral airborne remote sensing in Saint Joseph’s Bay, Florida. Estuaries coasts 37, 1467–1489. doi:10.1007/s12237-013-9764-3
Howari, F. (2004). Investigation of hydrocarbon pollution in the vicinity of United Arab Emirates coasts using visible and near infrared remote sensing data. J. Coast. Res. 20 (4), 1089–1095. doi:10.2112/03-0023r.1
Howari, F. M., Jordan, B. R., Bouhouche, N., and Wyllie-Echeverria, S. (2009). Field and remote-sensing assessment of mangrove forests and seagrass beds in the northwestern part of the United Arab Emirates. J. Coast. Res. 25 (1), 48–56. doi:10.2112/07-0867.1
Hussein, H., and Hussein, M. (2017). The study of multi-temporal analysis of urban development and environmental changes of the city of Abu Dhabi. Glob. Changes Nat. Disaster Manag. Geo-information Technol., 17–32. doi:10.1007/978-3-319-51844-2_2
Issa, S., Al Ameeri, M., Abouhaligah, H., Alkaabi, N., Sultan, M., and Dahy, B. (2023). Modelling and visualizing urban growth trajectory in Abu Dhabi using time series satellite imagery. Int. Archives Photogrammetry, Remote Sens. Spatial Inf. Sci. 48, 145–151. doi:10.5194/isprs-archives-xlviii-m-1-2023-145-2023
Issa, S., Dahy, B., Saleous, N., and Shamsi, M. A. (2021). “A remote sensing based time-series land use land cover change (LULCC) analysis: a case study from the United Arab Emirates (UAE),” in Sixth international conference on engineering geophysics, virtual, 25–28 october 2021.
Jaad, A., and Abdelghany, K. (2021). The story of five MENA cities: urban growth prediction modeling using remote sensing and video analytics. Cities 118, 103393. doi:10.1016/j.cities.2021.103393
Jaber, S. M. (2020). Is there a relationship between human population distribution and land surface temperature? Global perspective in areas with different climatic classifications. Remote Sens. Appl. Soc. Environ. 20, 100435. doi:10.1016/j.rsase.2020.100435
Jensen, J. R., Narumalani, S., Weatherbee, O., Murday, M., Sexton, W. J., and Green, C. J. (1993). Coastal environmental sensitivity mapping for oil spills in the United Arab Emirates using remote sensing and GIS technology. Geocarto Int. 8 (2), 5–13. doi:10.1080/10106049309354404
Kabiri, K. (2022). Estimation of the Secchi disk depth from the NASA MODIS-Aqua diffuse attenuation coefficient data in the northern Persian Gulf and the Gulf of Oman: a spatiotemporal assessment. Regional Stud. Mar. Sci. 52, 102359. doi:10.1016/j.rsma.2022.102359
Kabiri, K., Pradhan, B., Samimi-Namin, K., and Moradi, M. (2013a). Detecting coral bleaching, using QuickBird multi-temporal data: a feasibility study at Kish Island, the Persian Gulf. Estuar. Coast. Shelf Sci. 117, 273–281. doi:10.1016/j.ecss.2012.12.006
Kabiri, K., Pradhan, B., Shafri, H. Z. M., Mansor, S. B., and Samimi-Namin, K. (2013b). A novel approach to estimate diffuse attenuation coefficients for QuickBird satellite images: a case study at Kish Island, the Persian Gulf. J. Indian Soc. Remote Sens. 41, 797–806. doi:10.1007/s12524-013-0293-0
Kabiri, K., Rezai, H., and Moradi, M. (2018). Mapping of the corals around Hendorabi Island (Persian Gulf), using Worldview-2 standard imagery coupled with field observations. Mar. Pollut. Bull. 129 (1), 266–274. doi:10.1016/j.marpolbul.2018.02.045
Kabiri, K., Rezai, H., and Moradi, M. (2020). A drone-based method for mapping the coral reefs in the shallow coastal waters–case study: Kish Island, Persian Gulf. Earth Sci. Inf. 13 (4), 1265–1274. doi:10.1007/s12145-020-00507-z
Kabiri, K., Rezai, H., Moradi, M., and Pourjomeh, F. (2014). Coral reefs mapping using parasailing aerial photography-feasibility study: Kish Island, Persian Gulf. J. Coast. conservation 18, 691–699. doi:10.1007/s11852-014-0345-9
Kao, H.-Y., Lagerloef, G. S., Lee, T., Melnichenko, O., Meissner, T., and Hacker, P. (2018). Assessment of aquarius sea surface salinity. Remote Sens. 10 (9), 1341. doi:10.3390/rs10091341
Karami, S., Hamzeh, N. H., Alam, K., Noori, F., and Abadi, A. R. S. (2021). Spatio-temporal and synoptic changes in dust at the three islands in the Persian Gulf region. J. Atmos. Solar-Terrestrial Phys. 214, 105539. doi:10.1016/j.jastp.2021.105539
Khan, M., Fadlallah, Y., and Al-Hinai, K. (1992). Thematic mapping of subtidal coastal habitats in the western arabian gulf using landsat TM data—abu Ali Bay, Saudi Arabia. Int. J. remote Sens. 13 (4), 605–614. doi:10.1080/01431169208904140
Khan, M. A., and Kumar, A. (2009). Impact of urban development on mangrove forests along the west coast of the Arabian Gulf. Earth Sci. India 2, 206–216.
Klemas, V. (2013). Fisheries applications of remote sensing: an overview. Fish. Res. 148, 124–136. doi:10.1016/j.fishres.2012.02.027
Kourosh Niya, A., Huang, J., Karimi, H., Keshtkar, H., and Naimi, B. (2019). Use of intensity analysis to characterize land use/cover change in the biggest Island of Persian Gulf, Qeshm Island, Iran. Sustainability 11 (16), 4396. doi:10.3390/su11164396
Kristensen, E., Bouillon, S., Dittmar, T., and Marchand, C. (2008). Organic carbon dynamics in mangrove ecosystems: a review. Aquat. Bot. 89 (2), 201–219. doi:10.1016/j.aquabot.2007.12.005
Kwarteng, A. Y., and Chavez, P. (1998). Change detection study of Kuwait City and environs using multi-temporal Landsat Thematic Mapper data. Int. J. remote Sens. 19 (9), 1651–1662. doi:10.1080/014311698215162
Lazzarini, M., Marpu, P. R., and Ghedira, H. (2013). Temperature-land cover interactions: the inversion of urban heat island phenomenon in desert city areas. Remote Sens. Environ. 130, 136–152. doi:10.1016/j.rse.2012.11.007
Li, W., El-Askary, H., Qurban, M. A., Li, J., ManiKandan, K., and Piechota, T. (2019). Using multi-indices approach to quantify mangrove changes over the Western Arabian Gulf along Saudi Arabia coast. Ecol. Indic. 102, 734–745. doi:10.1016/j.ecolind.2019.03.047
Liu, Y., Hu, C., Dong, Y., Xu, B., Zhan, W., and Sun, C. (2019). Geometric accuracy of remote sensing images over oceans: the use of global offshore platforms. Remote Sens. Environ. 222, 244–266. doi:10.1016/j.rse.2019.01.002
Liu, Y., Sun, C., Yang, Y., Zhou, M., Zhan, W., and Cheng, W. (2016). Automatic extraction of offshore platforms using time-series Landsat-8 Operational Land Imager data. Remote Sens. Environ. 175, 73–91. doi:10.1016/j.rse.2015.12.047
Loughland, R., Butt, S. J., and Nithyanandan, M. (2020). Establishment of mangrove ecosystems on man-made islands in Kuwait: sustainable outcomes in a challenging and changing environment. Aquat. Bot. 167, 103273. doi:10.1016/j.aquabot.2020.103273
Loughland, R. A., Saenger, P., Luker, G., Siddiqui, K., Saji, B., Belt, M., et al. (2007). Changes in the coastal zone of Abu Dhabi determined using satellite imagery (1972–2003). Aquatic Ecosyst. Health and Manag. 10 (3), 301–308. doi:10.1080/14634980701512988
Lu, D., Mausel, P., Brondizio, E., and Moran, E. (2004). Change detection techniques. Int. J. remote Sens. 25 (12), 2365–2401. doi:10.1080/0143116031000139863
Mafi-Gholami, D., Zenner, E. K., Jaafari, A., Bakhtyari, H. R. R., and Bui, D. T. (2019). Multi-hazards vulnerability assessment of southern coasts of Iran. J. Environ. Manag. 252, 109628. doi:10.1016/j.jenvman.2019.109628
Mahrad, B. E., Newton, A., Icely, J. D., Kacimi, I., Abalansa, S., and Snoussi, M. (2020). Contribution of remote sensing technologies to a holistic coastal and marine environmental management framework: a review. Remote Sens. 12 (14), 2313. doi:10.3390/rs12142313
Manandhar, P., Marpu, P. R., Aung, Z., and Melgani, F. (2019). Towards automatic extraction and updating of VGI-based road networks using deep learning. Remote Sens. 11 (9), 1012. doi:10.3390/rs11091012
Mancini, S., Olsen, R. C., Abileah, R., and Lee, K. R. (2012). Automating nearshore bathymetry extraction from wave motion in satellite optical imagery. Algorithms and Technologies for Multispectral, Hyperspectral, and Ultraspectral Imagery XVIII.
Mannino, A. M., Borfecchia, F., and Micheli, C. (2021). Tracking marine alien macroalgae in the Mediterranean Sea: the contribution of citizen science and remote sensing. J. Mar. Sci. Eng. 9 (3), 288. doi:10.3390/jmse9030288
Mansour, S., Al-Awadhi, T., Hatrushi, S., and Buloshi, A. (2017). The anthropogenic effects on coral reefs across northern coasts of Oman: a GIS based modeling. Geoinfor geostat: an overview 5: 2. of 6, 2.
Mansourmoghaddam, M., Ghafarian Malamiri, H. R., Rousta, I., Olafsson, H., and Zhang, H. (2022). Assessment of Palm Jumeirah Island’s construction effects on the surrounding water quality and surface temperatures during 2001–2020. Water 14 (4), 634. doi:10.3390/w14040634
Mateos-Molina, D., Antonopoulou, M., Baldwin, R., Bejarano, I., Burt, J. A., García-Charton, J. A., et al. (2020). Applying an integrated approach to coastal marine habitat mapping in the north-western United Arab Emirates. Mar. Environ. Res. 161, 105095. doi:10.1016/j.marenvres.2020.105095
Mateos-Molina, D., Pittman, S., Antonopoulou, M., Baldwin, R., Chakraborty, A., García-Charton, J., et al. (2021). An integrative and participatory coastal habitat mapping framework for sustainable development actions in the United Arab Emirates. Appl. Geogr. 136, 102568. doi:10.1016/j.apgeog.2021.102568
McCarthy, M. J., Colna, K. E., El-Mezayen, M. M., Laureano-Rosario, A. E., Méndez-Lázaro, P., Otis, D. B., et al. (2017). Satellite remote sensing for coastal management: a review of successful applications. Environ. Manag. 60, 323–339. doi:10.1007/s00267-017-0880-x
Meline, T. (2006). Selecting studies for systemic review: inclusion and exclusion criteria. Contemp. issues Commun. Sci. Disord. 33, 21–27. doi:10.1044/cicsd_33_s_21
Miliaresis, G. C. (2013). Terrain analysis for active tectonic zone characterization: a new application for MODIS night LST (MYD11C3) data set. Int. J. Geogr. Inf. Sci. 27 (7), 1417–1432. doi:10.1080/13658816.2012.685172
Mirakhorlou, K., Teimouri, S., and Abadeh, M. (2017). Mapping potential of mangrove forests based on site demands (Geomorphological factors and physico-chemical characteristics of soil and water). Environ. Conserv. 23, 90–97.
Modara, M., Ait Belaid, M., and AlJenaid, S. (2014). Assessment of land-use/land-cover change in Muharraq Island using multi-temporal and multi-source geospatial data. Int. J. Image Data Fusion 5 (3), 210–225. doi:10.1080/19479832.2014.904446
Moeinkhah, A., Shakiba, A., and Azarakhsh, Z. (2019). Assessment of regression and classification methods using remote sensing technology for detection of coastal depth (case study of Bushehr Port and Kharg Island). J. Indian Soc. Remote Sens. 47, 1019–1029. doi:10.1007/s12524-019-00959-x
Mokhtari, S., Hosseini, S. M., Danehkar, A., Azad, M. T., Kadlec, J., Jolma, A., et al. (2015). Inferring spatial distribution of oil spill risks from proxies: case study in the north of the Persian Gulf. Ocean and Coast. Manag. 116, 504–511. doi:10.1016/j.ocecoaman.2015.08.017
Moradi, M. (2021). Evaluation of merged multi-sensor ocean-color chlorophyll products in the Northern Persian Gulf. Cont. Shelf Res. 221, 104415. doi:10.1016/j.csr.2021.104415
Moradi, M., and Moradi, N. (2020). Correlation between concentrations of chlorophyll-a and satellite derived climatic factors in the Persian Gulf. Mar. Pollut. Bull. 161, 111728. doi:10.1016/j.marpolbul.2020.111728
Mousavi, S. H., Danehkar, A., Shokri, M. R., Poorbagher, H., and Azhdari, D. (2015). Site selection for artificial reefs using a new combine Multi-Criteria Decision-Making (MCDM) tools for coral reefs in the Kish Island–Persian Gulf. Ocean and Coast. Manag. 111, 92–102. doi:10.1016/j.ocecoaman.2015.03.004
Muhairi, A., Ghedira, H., and Shaheen, A. (2010). “Combining satellite-derived chlorophyll-a data and high-resolution dubaisat-1 data to detect and monitor red tide outbreaks in the Arabian Gulf,” in 2010 IEEE International Geoscience and Remote Sensing Symposium.
Naimaee, R., Kiani, A., Jarahizadeh, S., Haji Seyed Asadollah, S. B., Melgarejo, P., and Jodar-Abellan, A., (2024). Long-term water quality monitoring: using satellite images for temporal and spatial monitoring of thermal pollution in water resources. Sustainability 16 (2), 646. doi:10.3390/su16020646
Nassar, A. K., Blackburn, G. A., and Whyatt, J. D. (2014). Developing the desert: the pace and process of urban growth in Dubai. Comput. Environ. Urban Syst. 45, 50–62. doi:10.1016/j.compenvurbsys.2014.02.005
Nassar, A. K., Blackburn, G. A., and Whyatt, J. D. (2016). Dynamics and controls of urban heat sink and island phenomena in a desert city: development of a local climate zone scheme using remotely-sensed inputs. Int. J. Appl. earth observation geoinformation 51, 76–90. doi:10.1016/j.jag.2016.05.004
Neelamani, S. (2018). Coastal erosion and accretion in Kuwait–problems and management strategies. Ocean and Coast. Manag. 156, 76–91. doi:10.1016/j.ocecoaman.2017.05.014
Nesterov, O., Temimi, M., Fonseca, R., Nelli, N. R., Addad, Y., Bosc, E., et al. (2021). Validation and statistical analysis of the group for high resolution sea surface temperature data in the arabian gulf. Oceanologia 63 (4), 497–515. doi:10.1016/j.oceano.2021.07.001
Nodej, T. M., and Rezazadeh, M. (2018). The spatial distribution of critical wind erosion centers according to the dust event in Hormozgan province (south of Iran). Catena 167, 340–352. doi:10.1016/j.catena.2018.05.008
Page, M. J., McKenzie, J. E., Bossuyt, P. M., Boutron, I., Hoffmann, T. C., Mulrow, C. D., et al. (2021). The PRISMA 2020 statement: an updated guideline for reporting systematic reviews. Int. J. Surg. 88, 105906. doi:10.1016/j.ijsu.2021.105906
Paul, J., Shankar, B. U., Bhattacharyya, B., and Datta, A. K. (2021). Unsupervised change detection in remote sensing images using CNN based transfer learning. Adv. Comput. Data Sci. 1440, 463–474. doi:10.1007/978-3-030-81462-5_42
Pettorelli, N., Laurance, W. F., O'Brien, T. G., Wegmann, M., Nagendra, H., and Turner, W. (2014). Satellite remote sensing for applied ecologists: opportunities and challenges. J. Appl. Ecol. 51 (4), 839–848. doi:10.1111/1365-2664.12261
Pitarch, J., Volpe, G., Colella, S., Krasemann, H., and Santoleri, R. (2016). Remote sensing of chlorophyll in the Baltic Sea at basin scale from 1997 to 2012 using merged multi-sensor data. Ocean Sci. 12 (2), 379–389. doi:10.5194/os-12-379-2016
Price, A., Sheppard, C., and Roberts, C. (1993). The Gulf: its biological setting. Mar. Pollut. Bull. 27, 9–15. doi:10.1016/0025-326x(93)90004-4
Purkis, S. J. (2018). Remote sensing tropical coral reefs: the view from above. Annu. Rev. Mar. Sci. 10, 149–168. doi:10.1146/annurev-marine-121916-063249
Radhi, H., Fikry, F., and Sharples, S. (2013). Impacts of urbanisation on the thermal behaviour of new built up environments: a scoping study of the urban heat island in Bahrain. Landsc. Urban Plan. 113, 47–61. doi:10.1016/j.landurbplan.2013.01.013
Rahman, M. T. (2016). Detection of land use/land cover changes and urban sprawl in Al-Khobar, Saudi Arabia: an analysis of multi-temporal remote sensing data. ISPRS Int. J. Geo-Information 5 (2), 15. doi:10.3390/ijgi5020015
Rahman, M. T., Aldosary, A. S., and Mortoja, M. G. (2017). Modeling future land cover changes and their effects on the land surface temperatures in the Saudi Arabian eastern coastal city of Dammam. Land 6 (2), 36. doi:10.3390/land6020036
Ramachandra, T., Aithal, B. H., and Sanna, D. D. (2012). Insights to urban dynamics through landscape spatial pattern analysis. Int. J. Appl. earth observation geoinformation 18, 329–343. doi:10.1016/j.jag.2012.03.005
Ramadan, E. (2015). Sustainable urbanization in the arabian gulf region: problems and challenges. Arts Soc. Sci. J. 6 (2), 1–4. doi:10.4172/2151-6200.1000109
Riegl, B. M., and Purkis, S. J. (2005). Detection of shallow subtidal corals from IKONOS satellite and QTC View (50, 200 kHz) single-beam sonar data (Arabian Gulf; Dubai, UAE). Remote Sens. Environ. 95 (1), 96–114. doi:10.1016/j.rse.2004.11.016
Riegl, B. M., and Purkis, S. J. (2009). Model of coral population response to accelerated bleaching and mass mortality in a changing climate. Ecol. Model. 220 (2), 192–208. doi:10.1016/j.ecolmodel.2008.09.022
Rondon, M., Ewane, E. B., Abdullah, M. M., Watt, M. S., Blanton, A., Abulibdeh, A., et al. (2023). Remote sensing-based assessment of mangrove ecosystems in the Gulf Cooperation Council countries: a systematic review. Front. Mar. Sci. 10. doi:10.3389/fmars.2023.1241928
Rubin, L. (2024). A Middle East space race? Motivations, trajectories, and regional politics. Space Policy 101608, 101608. doi:10.1016/j.spacepol.2023.101608
Saburova, M., Al-Kandari, M., Polikarpov, I., Akbar, A., Hussain, S., Rahmeh, R., et al. (2022). Alien toxic dinoflagellate Heterocapsa circularisquama from the western pacific in Kuwait, NW Indian ocean. Deep Sea Res. Part II Top. Stud. Oceanogr. 196, 105027. doi:10.1016/j.dsr2.2022.105027
Saito, H., Bellan, M., Al-Habshi, A., Aizpuru, M., and Blasco, F. (2003). Mangrove research and coastal ecosystem studies with SPOT-4 HRVIR and TERRA ASTER in the Arabian Gulf. Int. J. remote Sens. 24 (21), 4073–4092. doi:10.1080/0143116021000035030
Sale, P. F., Agardy, T., Ainsworth, C. H., Feist, B. E., Bell, J. D., Christie, P., et al. (2014). Transforming management of tropical coastal seas to cope with challenges of the 21st century. Mar. Pollut. Bull. 85 (1), 8–23. doi:10.1016/j.marpolbul.2014.06.005
Saleh, A. K., and Al-Anzi, B. S. (2021). Statistical validation of MODIS-based sea surface temperature in shallow semi-enclosed marginal sea: a comparison between direct matchup and triple collocation. Water 13 (8), 1078. doi:10.3390/w13081078
Sardi, S. S., Qurban, M. A., Li, W., Kadinjappalli, K. P., Manikandan, P. K., Hariri, M. M., et al. (2020). Assessment of areas environmentally sensitive to oil spills in the western Arabian Gulf, Saudi Arabia, for planning and undertaking an effective response. Mar. Pollut. Bull. 150, 110588. doi:10.1016/j.marpolbul.2019.110588
Schaeffer, B. A., Schaeffer, K. G., Keith, D., Lunetta, R. S., Conmy, R., and Gould, R. W. (2013). Barriers to adopting satellite remote sensing for water quality management. Int. J. remote Sens. 34 (21), 7534–7544. doi:10.1080/01431161.2013.823524
Shandas, V., Makido, Y., and Ferwati, S. (2017). Rapid urban growth and land use patterns in Doha, Qatar: opportunities for sustainability. Eur. J. Sustain. Dev. Res. 1. doi:10.20897/ejosdr.201711
Sheppard, C., Al-Husiani, M., Al-Jamali, F., Al-Yamani, F., Baldwin, R., Bishop, J., et al. (2010). The Gulf: a young sea in decline. Mar. Pollut. Bull. 60 (1), 13–38. doi:10.1016/j.marpolbul.2009.10.017
Sohl, T. L. (1999). Change analysis in the United Arab Emirates: an investigation of techniques. Photogrammetric Eng. remote Sens. 65 (4), 475–484.
Subraelu, P., Ebraheem, A. A., Sherif, M., Sefelnasr, A., Yagoub, M., and Rao, K. N. (2022). Land in water: the study of land reclamation and artificial islands formation in the UAE coastal zone: a remote sensing and GIS perspective. Land 11 (11), 2024. doi:10.3390/land11112024
Subraelu, P., Yagoub, M., Sefelnasr, A., Nageswara, K., Allamsatti, R., Ebraheem, A., et al. (2021). Sea-Level rise and coastal vulnerability: a preliminary assessment of UAE coast through remote sensing and GIS. J. Coast. Zone Mang 24, 477–480.
Sultana, R., and Nasrollahi, N. (2018). Evaluation of remote sensing precipitation estimates over Saudi Arabia. J. Arid Environ. 151, 90–103. doi:10.1016/j.jaridenv.2017.11.002
Szekielda, K.-H., Mitchell, W., and Usn, L. (1971). Oceanographic applications of color-enhanced satellite imageries. Remote Sens. Environ. 2, 71–76. doi:10.1016/0034-4257(71)90079-4
Tao, H., Hashim, B. M., Heddam, S., Goliatt, L., Tan, M. L., Sa’adi, Z., et al. (2023). Megacities’ environmental assessment for Iraq region using satellite image and geo-spatial tools. Environ. Sci. Pollut. Res. 30 (11), 30984–31034. doi:10.1007/s11356-022-24153-8
Tariq, S., Mariam, A., and Mehmood, U. (2022). Spatial and temporal variations in PM2. 5 and associated health risk assessment in Saudi Arabia using remote sensing. Chemosphere 308, 136296. doi:10.1016/j.chemosphere.2022.136296
Tehrani, N. A., Janalipour, M., and Babaei, H. (2021). Estimating water surface chlorophyll-a concentration by big remote sensing data in the Persian gulf, bushehr. Remote Sens. Earth Syst. Sci. 4 (1-2), 87–95. doi:10.1007/s41976-021-00045-2
Theriault, C., Scheibling, R., Hatcher, B., and Jones, W. (2006). Mapping the distribution of an invasive marine alga (Codium fragile spp. tomentosoides) in optically shallow coastal waters using the compact airborne spectrographic imager (CASI). Can. J. Remote Sens. 32 (5), 315–329. doi:10.5589/m06-027
Toosi, N. B., Soffianian, A. R., Fakheran, S., and Waser, L. T. (2022). Mapping disturbance in mangrove ecosystems: incorporating landscape metrics and PCA-based spatial analysis. Ecol. Indic. 136, 108718. doi:10.1016/j.ecolind.2022.108718
Traganos, D., Aggarwal, B., Poursanidis, D., Topouzelis, K., Chrysoulakis, N., and Reinartz, P. (2018). Towards global-scale seagrass mapping and monitoring using Sentinel-2 on Google Earth Engine: the case study of the aegean and ionian seas. Remote Sens. 10 (8), 1227. doi:10.3390/rs10081227
Uddin, S., Al Ghadban, A. N., Al Dousari, A., Al Murad, M., and Al Shamroukh, D. (2010). A remote sensing classification for land-cover changes and micro-climate in Kuwait. Int. J. Sustainable Dev. Plann. 5 (4), 367–377. doi:10.2495/SDP-V5-N4-367-377
U.S. Department of Energy (2019). The Strait of Hormuz is the world's most important oil transit chokepoint. Available at: https://www.eia.gov/todayinenergy/detail.php?id=39932# (Accessed May 15, 2023).
Vanhellemont, Q., and Ruddick, K. (2015). Advantages of high quality SWIR bands for ocean colour processing: examples from Landsat-8. Remote Sens. Environ. 161, 89–106. doi:10.1016/j.rse.2015.02.007
van Lavieren, H., Burt, J., Feary, D. A., Cavalcante, G., Marquis, E., Benedetti, L., et al. (2011). Managing the growing impacts of development on fragile coastal and marine ecosystems: lessons from the Gulf: policy report. International Network on Water, Environment: United Nations University.
Vaughan, G. O., Al-Mansoori, N., and Burt, J. A. (2019). “The arabian gulf,” in World seas: an environmental evaluation (Elsevier), 1–23.
Vitousek, S., Buscombe, D., Vos, K., Barnard, P. L., Ritchie, A. C., and Warrick, J. A. (2023). The future of coastal monitoring through satellite remote sensing. Camb. Prisms Coast. Futur. 1, e10. doi:10.1017/cft.2022.4
Wang, L., Jia, M., Yin, D., and Tian, J. (2019). A review of remote sensing for mangrove forests: 1956–2018. Remote Sens. Environ. 231, 111223. doi:10.1016/j.rse.2019.111223
Warren, C., Dupont, J., Abdel-Moati, M., Hobeichi, S., Palandro, D., and Purkis, S. (2016). Remote sensing of Qatar nearshore habitats with perspectives for coastal management. Mar. Pollut. Bull. 105 (2), 641–653. doi:10.1016/j.marpolbul.2015.11.036
Wentz, E. A., Anderson, S., Fragkias, M., Netzband, M., Mesev, V., Myint, S. W., et al. (2014). Supporting global environmental change research: a review of trends and knowledge gaps in urban remote sensing. Remote Sens. 6 (5), 3879–3905. doi:10.3390/rs6053879
Wong, B. A., Thomas, C., and Halpin, P. (2019). Automating offshore infrastructure extractions using synthetic aperture radar and Google Earth Engine. Remote Sens. Environ. 233, 111412. doi:10.1016/j.rse.2019.111412
Wu, W., Zhi, C., Gao, Y., Chen, C., Chen, Z., Su, H., et al. (2022). Increasing fragmentation and squeezing of coastal wetlands: status, drivers, and sustainable protection from the perspective of remote sensing. Sci. Total Environ. 811, 152339. doi:10.1016/j.scitotenv.2021.152339
Xu, J., and Zhao, D. (2014). Review of coral reef ecosystem remote sensing. Acta Ecol. Sin. 34 (1), 19–25. doi:10.1016/j.chnaes.2013.11.003
Yacoubi, L., Savoca, D., El Zrelli, R. B., Gopalan, J., Nazal, M., Lin, Y.-J., et al. (2024). Trace element levels in the muscles of three tern species (Aves: laridae) from the western Arabian Gulf: environmental assessment and implications for conservation. Environ. Monit. Assess. 196 (3), 235. doi:10.1007/s10661-024-12385-9
Yagoub, M., and Kolan, G. R. (2006). Monitoring coastal zone land use and land cover changes of Abu Dhabi using remote sensing. J. Indian Soc. Remote Sens. 34, 57–68. doi:10.1007/bf02990747
Yancho, J. M. M., Jones, T. G., Gandhi, S. R., Ferster, C., Lin, A., and Glass, L. (2020). The google earth engine mangrove mapping methodology (Geemmm). Remote Sens. 12 (22), 3758. doi:10.3390/rs12223758
Zainal, A. J., Dalby, D., and Robinson, I. (1993). Monitoring marine ecological changes on the east coast of Bahrain with Landsat TM. Photogrammetric Eng. Remote Sensing;(United States) 59 (3).
Zainal, K., Al-Madany, I., Al-Sayed, H., Khamis, A., Al Shuhaby, S., Al Hisaby, A., et al. (2012). The cumulative impacts of reclamation and dredging on the marine ecology and land-use in the Kingdom of Bahrain. Mar. Pollut. Bull. 64 (7), 1452–1458. doi:10.1016/j.marpolbul.2012.04.004
Zanchetta, A., and Zecchetto, S. (2021). Wind direction retrieval from Sentinel-1 SAR images using ResNet. Remote Sens. Environ. 253, 112178. doi:10.1016/j.rse.2020.112178
Zayed Abdalla, A. (2022). The effects of Anthropogenic Induced geomorphological changes on mangrove forests in Tarut Bay, on the east coast of Saudi Arabia. Sci. J. Sch. Arts-University Assiut 25 (82), 1171–1200. doi:10.21608/aakj.2022.239817
Zhang, J., Su, F., and Ding, Z. (2017). Sea reclamation status of countries around the South China Sea from 1975 to 2010. Sustainability 9 (6), 878. doi:10.3390/su9060878
Zhao, J., Temimi, M., and Ghedira, H. (2015). Characterization of harmful algal blooms (HABs) in the Arabian Gulf and the Sea of Oman using MERIS fluorescence data. ISPRS J. Photogrammetry Remote Sens. 101, 125–136. doi:10.1016/j.isprsjprs.2014.12.010
Zhao, J., Temimi, M., and Ghedira, H. (2017). Remotely sensed sea surface salinity in the hyper-saline Arabian Gulf: application to landsat 8 OLI data. Estuar. Coast. Shelf Sci. 187, 168–177. doi:10.1016/j.ecss.2017.01.008
Zhuang, Y., Liu, X., Nguyen, T., He, Q., and Hong, S. (2013). Global remote sensing research trends during 1991–2010: a bibliometric analysis. Scientometrics 96, 203–219. doi:10.1007/s11192-012-0918-z
Keywords: change detection, coastal and marine habitats, coastal cities, coastal management, earth observation systems (EOS), environmental pressure, sustainable urban planning, land use/land cover (LULC)
Citation: Dahy B, Al-Memari M, Al-Gergawi A and Burt JA (2024) Remote sensing of 50 years of coastal urbanization and environmental change in the Arabian Gulf: a systematic review. Front. Remote Sens. 5:1422910. doi: 10.3389/frsen.2024.1422910
Received: 24 April 2024; Accepted: 20 August 2024;
Published: 12 September 2024.
Edited by:
Ahmet Cilek, Arizona State University, United StatesReviewed by:
Dimitris Poursanidis, Terrasolutions Marine Environment Research, GreeceVarun Narayan Mishra, Amity University, India
Sajjad Hussain, COMSATS Institute of Information Technology, Pakistan
Copyright © 2024 Dahy, Al-Memari, Al-Gergawi and Burt. This is an open-access article distributed under the terms of the Creative Commons Attribution License (CC BY). The use, distribution or reproduction in other forums is permitted, provided the original author(s) and the copyright owner(s) are credited and that the original publication in this journal is cited, in accordance with accepted academic practice. No use, distribution or reproduction is permitted which does not comply with these terms.
*Correspondence: Basam Dahy, YmFzYW0uZGFoeUBueXUuZWR1, ZG9oYWliYXNzYW1AZ21haWwuY29t