- 1Ecology and Evolutionary Biology, University of Colorado Boulder, Boulder, CO, United States
- 2Hopkins Marine Station, Department of Biology, Stanford University, Pacific Grove, CA, United States
- 3Monterey Bay Aquarium Research Institute, Moss Landing, CA, United States
- 4Department of Fisheries, Wildlife, and Conservation Sciences, Oregon State University, Corvallis, OR, United States
- 5Cooperative Institute for Marine Ecosystems and Resources Studies, Oregon State University, Newport, OR, United States
- 6Office of Science and Technology, National Oceanic and Atmospheric Administration (NOAA) Fisheries, Silver Spring, MD, United States
- 7Pacific Marine Environmental Laboratory, National Oceanic and Atmospheric Administration (NOAA), Newport, OR, United States
- 8Cooperative Institute for Research in Environmental Sciences, University of Colorado Boulder, Boulder, CO, United States
- 9National Centers for Environmental Information, National Oceanic and Atmospheric Administration (NOAA), Boulder, CO, United States
The NOAA-NPS Ocean Noise Reference Station Network (NRS) is a passive acoustic monitoring effort to record the low-frequency (<2 kHz) sound field throughout the U.S. Exclusive Economic Zone. Data collection began in 2014 and spans 12 acoustic recording locations. To date, NRS datasets have been analyzed to understand spatial variation of large-scale sound levels, however, assessment of specific sound sources is an area where these datasets can provide additional insights. To understand seasonal patterns of blue whale, Balaenoptera musculus, and fin whale, B. physalus, sound production in the eastern North Pacific Ocean, this study explored data recorded between 2014 and 2020 from four NRS recording sites. A call index (CI) was used to quantify the intensity of blue whale B calls and fin whale 20 Hz pulses. Diel and seasonal patterns were then determined in the context of their migratory patterns. Most sites shared similar patterns in blue whale CI: persistent acoustic presence for 4–5 months starting by August and ending by February with a CI maximum in October or November. Fin whale patterns included persistent acoustic presence for 5–7 months starting by October and ending before April with a CI maximum between October and December. The diel patterning of blue whale song varied across the sites with the Gulf of Alaska, Olympic Coast, Cordell Bank, and Channel Islands (2014–2015) exhibiting a tendency towards nighttime song detection. However, this diel pattern was not observed at Channel Islands (2018–2020). Fin whale song detection was distributed evenly across day and night at most recording sites and months, however, a tendency toward nighttime song detection was observed in Cordell Bank during fall, and Gulf of Alaska and Olympic Coast during spring. Understanding call and migration patterns for blue and fin whales is essential for conservation efforts. By using passive acoustic monitoring and efficient detection methods, such as CI, it is possible to process large amounts of bioacoustic data and better understand the migratory behaviors of endangered marine species.
1 Introduction
Sound travels faster and farther through water than air, especially at low frequencies (Urick, 1983). This quality, paired with limited visibility underwater, makes sound an essential mode of communication for marine organisms (Au and Hastings, 2008). Passive acoustic monitoring (PAM) can be used to measure ambient sound levels and monitor animal sound production over long time periods and large spatial areas (Browning et al., 2017; Baumgartner et al., 2018). The U.S. National Oceanic and Atmospheric Administration (NOAA)-National Park Service Ocean Noise Reference Station Network (NRS) is an example of a long-term PAM study, in which 12 calibrated autonomous passive acoustic recorders have been deployed and maintained in all major regions of the U.S. Exclusive Economic Zone since 2014. This network provides multi-year, continuous recordings of low-frequency underwater sound between 10 Hz and 2 kHz (Haver et al., 2018). Data from this acoustic network have been analyzed to assess long-term patterns of the ocean soundscape across sites and to understand how bioacoustic activity, anthropogenic noise, and physical processes contribute to the ambient sound levels (Haver et al., 2018; Haver et al., 2019; Haver et al., 2020; Haver et al., 2021).
One common source of bioacoustic sound in marine environments is baleen whales. Baleen whales use sound to forage, communicate, avoid predators, and navigate (Clark, 1990; Tyack and Clark, 2000; Parks et al., 2014; Torres, 2017; Davis et al., 2020; Oestreich et al., 2020a; Cade et al., 2021). Blue (Balaenoptera musculus) and fin (B. physalus) whales produce the lowest frequency vocalizations among whales and it is believed that they use sound to communicate over very long distances. Although blue and fin whales are endangered, their vocalizations are quite ubiquitous (McDonald et al., 2006; Rankin et al., 2006; Gedamke et al., 2007; Stafford et al., 2009; Širović et al., 2009; Širović et al., 2015; Davis et al., 2020).
While on the feeding grounds, blue whales in the Northeast Pacific are acoustically active, producing at least three different types of vocalizations termed A, B, and D calls, which are linked to behaviors ranging from foraging to social interaction (McDonald et al., 2006; Oleson et al., 2007a; Širović and Hildebrand, 2011; Lewis et al., 2018; Lewis and Širović, 2018; Szesciorka et al., 2020). B calls are low frequency, long-duration tonal calls thought to be used primarily (and potentially exclusively) by males to communicate over long distances, suggesting reproductive utility (Oleson et al., 2007a). However, a recent study examining the ratio of B calls occurring at night compared to day along with tagging data determined that B calls can indicate behavioral transitions from foraging to migration (Oestreich et al., 2020a). Due to their intensity and prevalence, B calls are often used to detect blue whales acoustically.
Two blue whale populations are present in the data examined in this study: the Northeast Pacific and North Pacific populations. However, the vocalizations of the North Pacific populations do not include B calls (Stafford et al., 2001; McDonald et al., 2006). The blue whale population in the Northeast Pacific migrates seasonally from waters off the Gulf of Alaska to Central America (Mate et al., 1999; Burtenshaw et al., 2004; Calambokidis et al., 2009). The majority of the population can be found in the feeding grounds of the highly productive California Current Ecosystem from May to December (Abrahms et al., 2019; Szesciorka et al., 2020). The population then migrates south to the breeding grounds in the Costa Rica Dome, Pacific coast of Mexico, and the Gulf of California (Mate et al., 1999; Burtenshaw et al., 2004; Bailey et al., 2009; Širović et al., 2015). In the Gulf of Alaska, both Northeast Pacific and North Pacific blue whale calls have been recorded suggesting both populations use this area (Stafford, 2003).
In the Northeast Pacific Ocean, fin whales are present from at least 70°N (Chukchi Sea) to 32°N (off the California coast) with seven distinct productive feeding areas concentrating the species in the summer (Mizroch et al., 2009). In the winter, fin whales have been documented from 60°N (Bering Sea and Gulf of Alaska) to 23°N (off Baja California coast), with regular observations off southern California and sightings and acoustic detections north of 40°N (Mizroch et al., 2009). Of a variety of call types, fin whales are thought to produce 20 Hz and 40 Hz pulse calls, with 40 Hz calls associated with foraging and 20 Hz pulses potentially serving a reproductive function (McDonald and Fox, 1999; Croll et al., 2002; Širović et al., 2009; Širović et al., 2017; Romagosa et al., 2021).
The objective of this study was to quantify blue B calls and fin whale 20 Hz pulses, using an energy index, from previously collected NRS data across four sites in the Pacific Ocean to document diel and seasonal patterns. Such temporal patterns in call detections provide insight into foraging and migratory behaviors, which are key to informing effective conservation measures.
2 Materials and methods
This project analyzed data from four passive acoustic moorings from the NRS project (Haxel et al., 2013; Haver et al., 2018). Each mooring contained a single omnidirectional, autonomous underwater hydrophone (AUH) with an approximate sensitivity of −192 dB re 1 V/μPa and flat frequency response (±1 dB) between 10 Hz and 2 kHz. Each NRS AUH collected acoustic data at a sample rate of 5 kHz with a 2 kHz low-pass cutoff. Multiple years of data were collected across the Channel Islands, Olympic Coast, Gulf of Alaska, and Cordell Bank NRS sites between 2014 and 2020 (Figure 1; Table 1). The location of each NRS was determined in partnership with NOAA National Marine Fisheries Service, NOAA Office of National Marine Sanctuaries and NOAA Pacific Marine Environmental Laboratory (Haver et al., 2018). The sites examined here are considered baleen whale feeding grounds (Calambokidis et al., 2015).
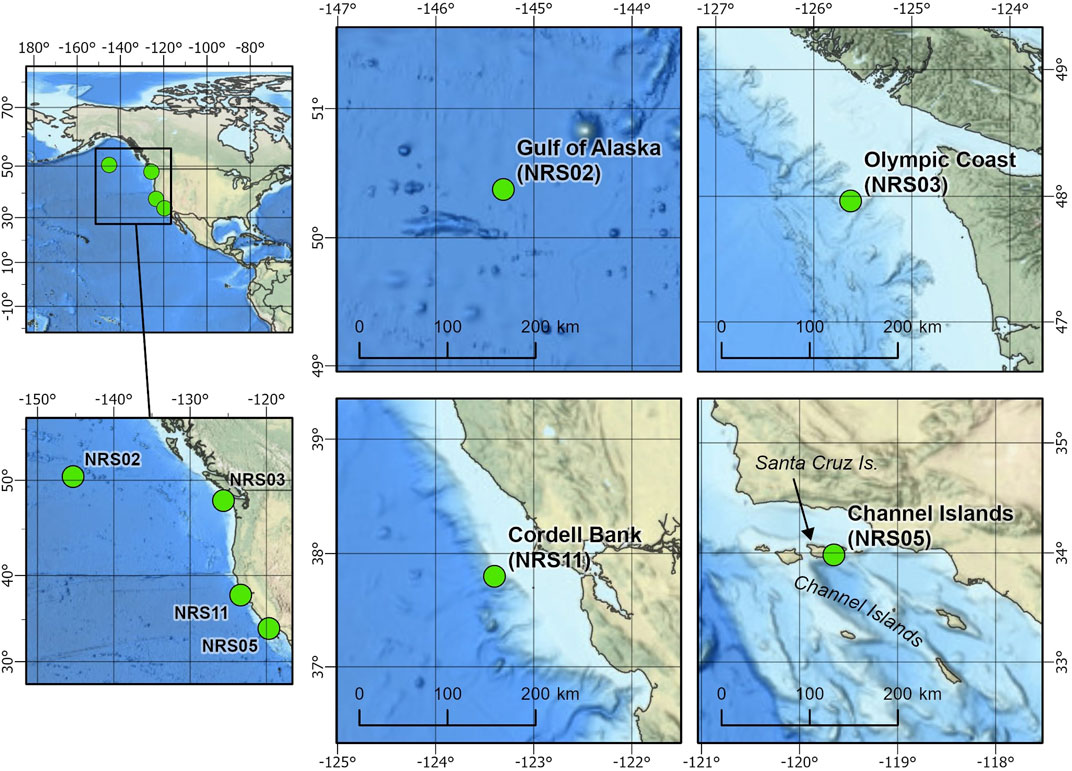
FIGURE 1. Locations of the NOAA-NPS Ocean Noise Reference Station Network (NRS) recording sites used for this study: Gulf of Alaska (NRS02), Olympic Coast (NRS03), Cordell Bank (NRS11), and Channel Islands (NRS05).
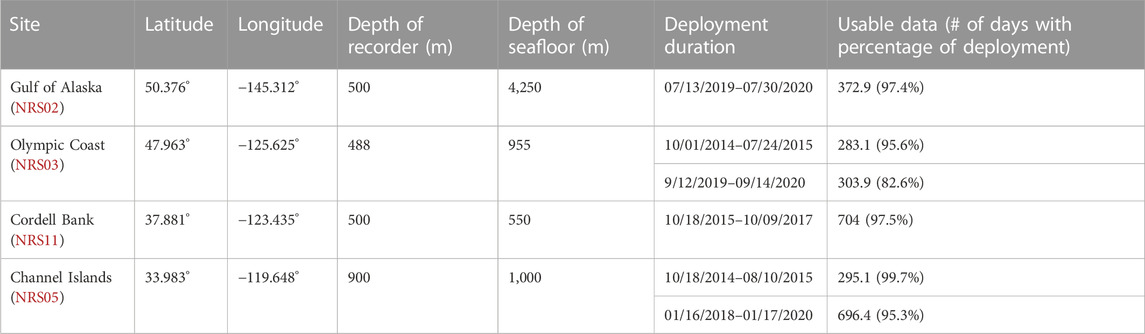
TABLE 1. Deployment details at each recording site, including location, recorder and seafloor depth, deployment start and end, and number of days and percentage of the deployment with usable data.
Some NRS deployments (Olympic Coast 2014–2015 and Channel Islands 2014–2015) were accessed from the NOAA National Centers for Environmental Information passive acoustic archive (NOAA OAR Pacific Marine Environmental Laboratory et al., 2014). The remaining deployments (Gulf of Alaska 2019–2020, Olympic Coast 2019–2020; Cordell Bank 2015–2017, and Channel Islands 2018–2020) were provided directly from NRS personnel. Data gaps are present due to equipment failure, data redacted by the U.S. Navy for national security concerns, and incomplete temporal coverage of deployments (Figure 2). Olympic Coast and Channel Islands sites contain two deployments. Each are analyzed separately due to interannual variability.
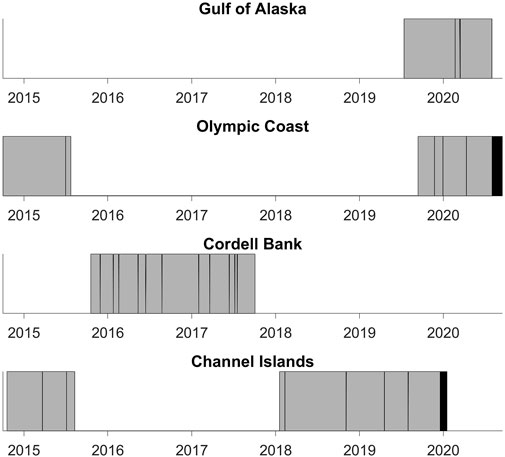
FIGURE 2. Duration of each deployment’s audio data. Gray indicates data used in this study. Black indicates data collected during the deployment that were not used in the study. White indicates data gaps. See Table 1 for the start and end dates of each deployment.
Daily long-term spectral averages (LTSAs) with a 1 Hz/1 min resolution were created from the calibrated sound files for each deployment. The documentation for Scripps Institution of Oceanography Triton software package contains detailed information on the creation of LTSAs1. LTSAs were then visually scrutinized to remove time periods with persistent noise artifacts resulting from recovery efforts or equipment failure. Periods of redactions, which can span a whole day or part of a day, were set to NaN. Leveraging established methods focused on acoustic power (Mellinger et al., 2009; Širović et al., 2009; Širović et al., 2015; Haver et al., 2020; Oestreich et al., 2020a), a call index (CI) approach was applied to the LTSAs to quantify blue whale B calls and fin whale 20 Hz pulses (Figure 3). CI calculates a signal-to-noise ratio using the target frequency range (signal) and nearby background frequencies (noise) for a given call type. The blue whale signal was calculated as the average of 43 Hz and 44 Hz (associated with the 3rd and strongest harmonic of the B call) while the fin whale signal was calculated as the average of 20 Hz and 21 Hz (associated with fin whale pulses). Noise was determined as the average of two background frequencies, one band below and one above the signal frequencies. For blue whale CI, the background frequencies were 37 and 50 Hz. For fin whale CI, 12 Hz and 34 Hz were used. CI for both blue and fin whales was aggregated from minute to daily and then to monthly resolution, represented as a statistical summary with percentiles (10th, 25th, 50th, 75th, 90th) and the mean. Results are presented as a single average seasonal cycle for each site, even if portions of the seasonal cycle were recorded in different years or if parts of the cycle were recorded across multiple years. Table 2 outlines the year usable data were collected aggregated by month to show the occurrence of non-consecutive data in each deployment. The two deployments for Olympic Coast and Channel Islands are discrete monitoring events separated by multiple years, and are kept separate for this analysis. For deployments that spanned multiple years (Channel Islands and Cordell Bank), the results for each separate year are reported in the supplementary section. The seasonal pattern results were confirmed by visual inspection of daily LTSAs for all sites. Acoustic presence of blue and fin whales is defined as a monthly median CI value exceeding 1.01. Oestreich et al. (2022) previously identified 1.01 as a robust threshold for presence vs. absence of B calls when applied to a 15-day running mean of call index in 6 years of recordings from Monterey Bay. For the monthly bins used here, a 1.01 CI threshold is potentially more conservative but minimizes the chance of false positives.
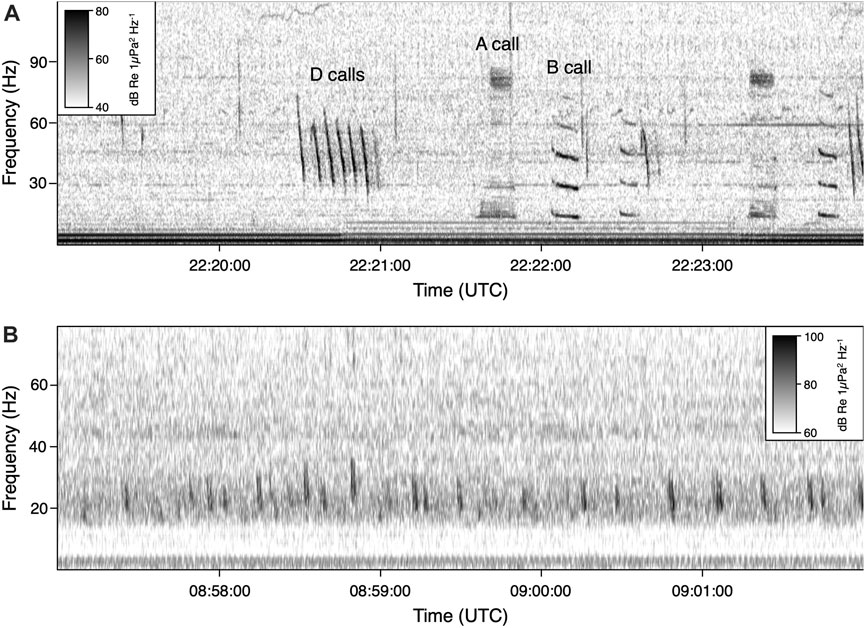
FIGURE 3. Spectrogram of (A) blue whale call types, including A, B, and D calls and (B) fin whale song pulses, produced near 20 Hz. Note the differences in scale. Spectrograms were created with the following parameters: 8,192 FFT window size, 50% overlap for blue whale; 4,096 FFT window size, 95% overlap for fin whale. The recording displayed in the top panel was taken on 18 June 2015 at the Channel Islands site. The recording in the bottom panel was taken on 3 October 2014 at the Olympic Coast site.
To examine diel patterns, CI values were categorized by day, night, and dusk/dawn. The Matlab SolarAzEl function (Koblick, 2020) was used to compute solar elevation for each minute of data using the location at the ocean surface above the NRS hydrophone. Solar elevations < −12° determined nighttime hours, −12° to 0° determined dusk/dawn hours, and >0° determined daytime hours. Categories for each 1-min CI measurement were binned to a daily resolution to calculate the ratio of CI values associated with nighttime to those associated with daytime (CInight:CIday). These daily values were then aggregated by month for statistical summary. CInight:CIday values above 1.0 indicate song detection was greater at night whereas values below 1.0 indicate song detection was greater during the day. Monthly CInight:CIday values were excluded from the data visualization when the corresponding monthly CI median was below 1.01.
To distinguish when CI signal and its diel patterns were caused by whale calling, the monthly spectrum levels associated with the target frequency bands and the background frequency bands were plotted for blue and fin whales overall (Supplementary Figures S1, S2) and separated by day and night (Supplementary Figures S3, S4). Note that the target frequency bands also contain background noise since the noise level was not subtracted. The monthly CI results were extracted and plotted for the individual years comprising the Cordell Bank 2015–2017 (Supplementary Figure S5) and Channel Islands 2018–2020 (Supplementary Figure S6) deployments.
All processing and plotting were completed using Matlab 2020a (Mathworks, Inc.). Custom scripts adapted from previous research (Oestreich et al., 2020a) were used to calculate CI and CInight:CIday.
3 Results
Each deployment’s LTSA depicts the overall soundscape for that site during the respective recording period (Figure 4). Blue whale B calls are visibly apparent in a narrow band slightly above 40 Hz for portions of every LTSA. Fin whale pulses are also apparent as a more broadband contribution around 20 Hz across the sites.
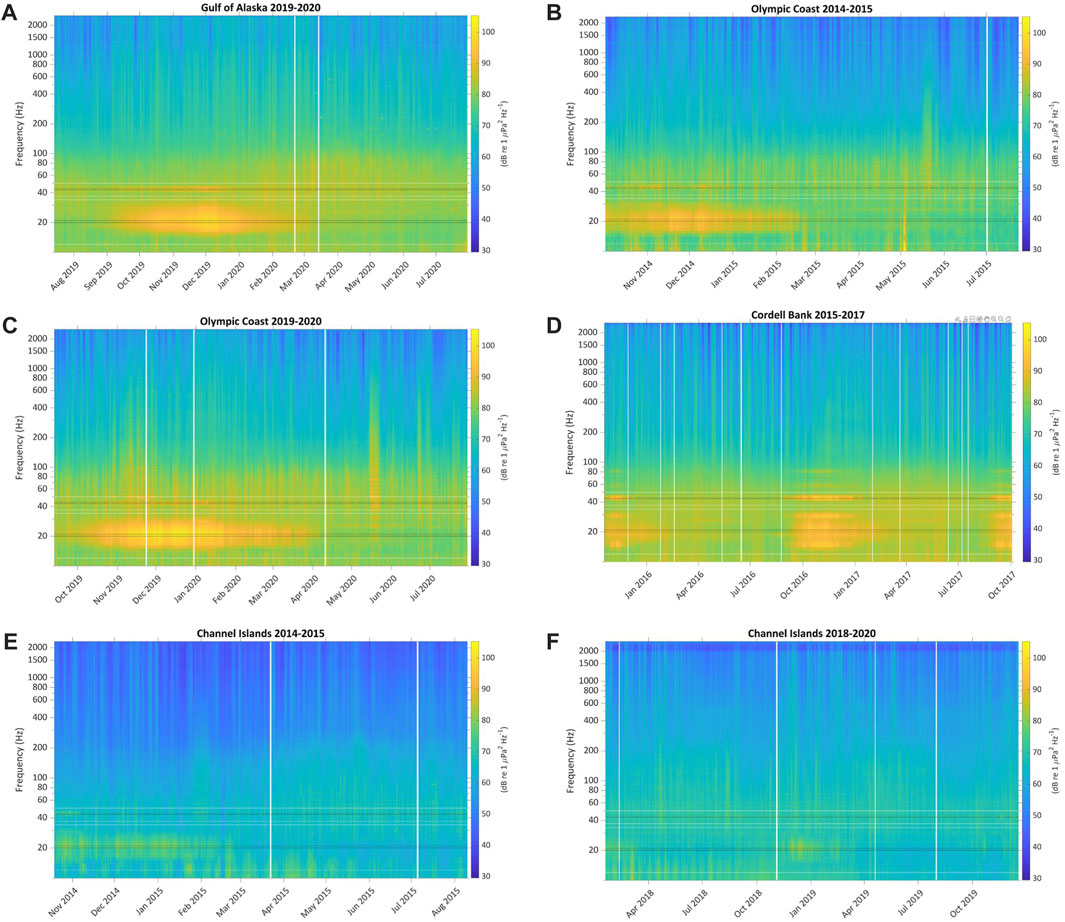
FIGURE 4. Calibrated 1 min/1 Hz LTSAs of (A) Gulf of Alaska 2019–2020, (B) Olympic Coast 2014–2015, (C) Olympic Coast 2019–2020, (D) Cordell Bank 2015–2017, (E) Channel Islands 2014–2015, and (F) Channel Islands 2018–2020 depicting each site’s overall soundscape. LTSAs were calculated using Welch’s Method (FFT length = 5,000 points, Hann window length = 5,000, FFT overlap = 50%). Blue whale B call 3rd harmonic frequencies (43 and 44 Hz) and fin whale 20 Hz pulse frequencies (20 and 21 Hz) used in the call index (CI) calculation are annotated in black. Additional B call harmonics are also visible at some sites. Background noise frequencies used in the CI calculations for blue whale (37 Hz and 50 Hz) and fin whale (12 and 34 Hz) are shown in white. Vertical white bars show time periods where data were redacted. See also Supplementary Figures S1, S2.
3.1 Blue whale calling patterns
Patterns in CI and associated night:day ratios (Figure 5) are supported by examination of the underlying signal and noise bands (Supplementary Figures S1, S3). Most recording sites shared similar patterns in blue whale CI: 1) persistent acoustic presence during a contiguous portion of the year (4–5 months), 2) rise in acoustic presence by August and absence by February, and 3) maximum CI in October or November (Figure 5; the maximum is indicated by the monthly median of daily values). The exceptions to these patterns were Olympic Coast 2019–2020, which indicated acoustic presence for only 2 months in December and January with December having the maximum CI (Figure 5C); Channel Islands 2014–2015 where data missing from September resulted in four non-contiguous months with CI values above 1.01 (Figure 5E), and Olympic Coast 2014–2015 where data missing in August and September precluded knowing which month blue whale CI values first exceeded 1.01 (Figure 5B). CI levels varied greatly across sites, with the strongest call signal at Cordell Bank (Figure 5D) and the weakest in the Channel Islands (Figure 5F). The two deployments recorded at Olympic Coast show a general presence of blue whale B calls in this area from as early as October to as late as January but also document interannual variability (Figures 5B, C). The two Channel Islands deployments (Figures 5E, F) also capture interannual variability. Median CInight:CIday values never fell below 1 for the Gulf of Alaska (Figure 5A), both Olympic Coast (Figures 5B, C), Cordell Bank (Figure 5D), and the Channel Islands 2014–2015 (Figure 5E) deployments, which suggests a tendency for greater intensity of blue whale song during the night compared to day. In addition to having the strongest CI signal, Cordell Bank is distinguished by the highest CInight:CIday values (Figure 5D). Along with having the lowest CI signals, Channel Islands 2018–2020 is distinguished by the lowest CInight:CIday values, with median values in all months below 1 (Figure 5F). The Channel Islands 2018–2020 median spectrum levels for the signal during the day were higher than those during the night from May to December (Supplementary Figure S3F). However, the high amount of overlap between day and night spectrum levels makes it difficult to distinguish a strong diel pattern for this deployment (Supplementary Figure S3F).
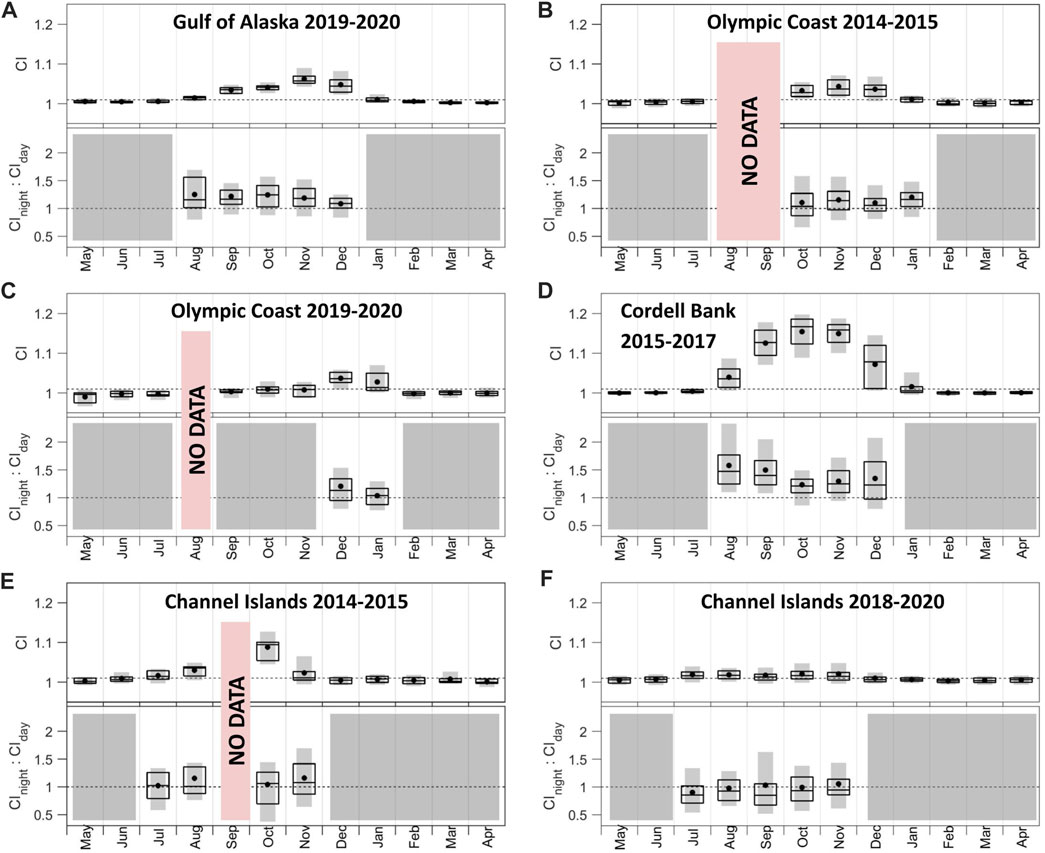
FIGURE 5. Call index (CI) and CInight:CIday associated with blue whale B calls across (A) Gulf of Alaska 2019–2020, (B) Olympic Coast 2014–2015, (C) Olympic Coast 2019–2020, (D) Cordell Bank 2015–2017, (E) Channel Islands 2014–2015, and (F) Channel Islands 2018–2020 sites. CInight:CIday values were only included if the monthly mean CI value was above 1.01. Months that did not meet this threshold are shown in gray. Periods of no data are noted in red. The dotted line in the top plots denotes the threshold for monthly mean CI (1.01). The dotted line in the bottom plots denotes the threshold for daytime song tendency (below than 1) and nighttime song tendency (greater than 1) calling. Boxplots show the 25th, 50th, and 75th percentiles of daily CI values from each month. Black dots indicate means. Gray bars indicate 10th-90th percentiles. Note the data shown are not necessarily chronologically. See also Supplementary Figures S1, S3.
3.2 Fin whale calling patterns
Patterns in CI and associated night:day ratios (Figure 6) are supported by examination of the underlying signal and noise bands (Supplementary Figures S2, S4). With the exception of Channel Islands 2018–2020, recording sites shared similar patterns in fin whale CI: 1) persistent acoustic presence during a contiguous portion of the year (5–7 months), 2) rise in acoustic presence by October and absence by April, and 3) maximum CI between October and December (Figure 6). Channel Islands 2018–2020 indicates 3 months of acoustic presence between December and February with a maximum CI in December (Figure 6F). CI levels varied across sites, with the strongest monthly median call signal at Olympic Coast (Figure 6C) and the weakest in the Channel Islands (Figure 6F). Similar to blue whale CI, inter-annual variability was observed at the two Olympic Coast (Figures 6B, C; peaks in November and December, respectively) and two Channel Island deployments (Figures 6E, F; peaks in October and December, respectively). The majority of months showed a median CInight:CIday value approximately equal to 1 indicating an even distribution across day and night. Gulf of Alaska (Figure 6A) and Olympic Coast (Figures 6B, C) showed a winter increase in median CInight:CIday from values below one to values above 1 as corresponding CI values declined while Cordell Bank (Figure 6D) showed CInight:CIday above one at the start of the calling period (September) followed by a continual downward trend in values falling down to and below 1.
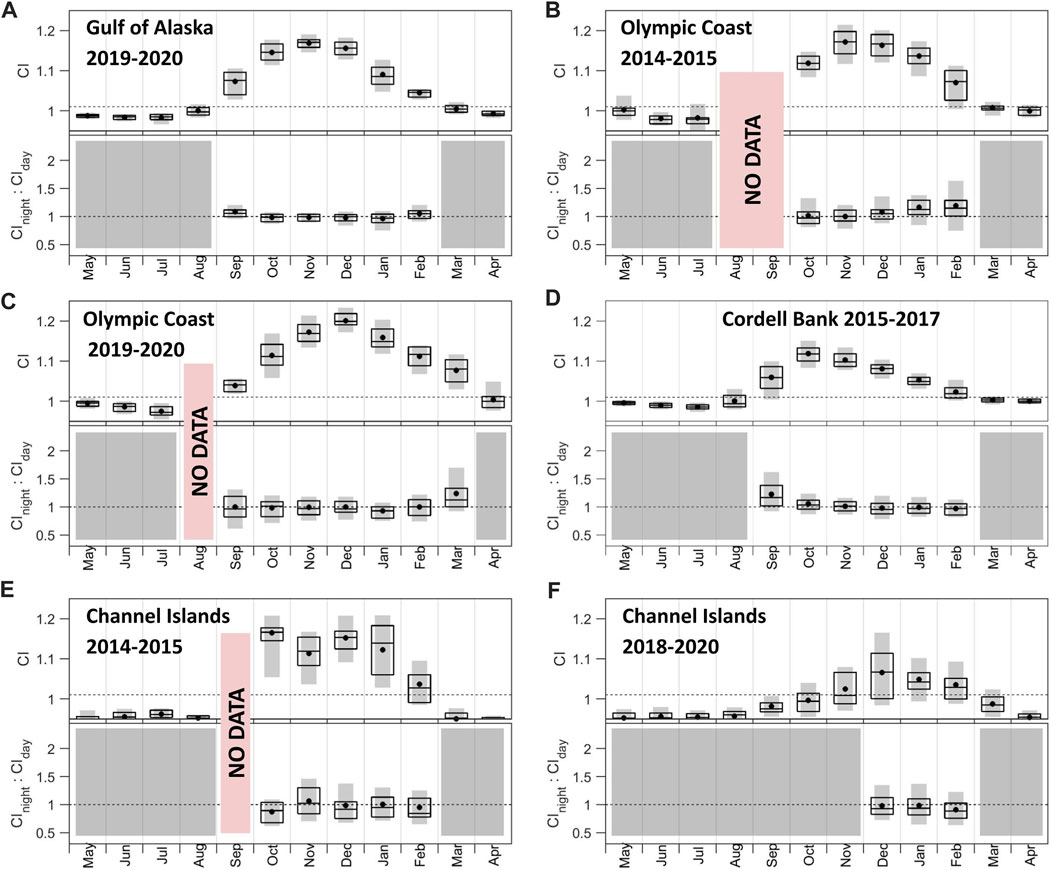
FIGURE 6. Call index (CI) and CInight:CIday associated with fin whale 20 Hz pulses across (A) Gulf of Alaska 2019–2020, (B) Olympic Coast 2014–2015, (C) Olympic Coast 2019–2020, (D) Cordell Bank 2015–2017, (E) Channel Islands 2014–2015, and (F) Channel Islands 2018–2020 sites. CInight:CIday values were only included if the mean CI value was above 1.01. Months that did not meet this threshold are shown in gray. Periods of no data are noted in red. The dotted line in the top plots denotes the threshold for monthly mean CI (1.01). The dotted line in the bottom plots denotes the threshold for daytime song tendency (below than 1) and nighttime song tendency (greater than 1) calling. Boxplots show the 25th, 50th, and 75th percentiles of daily CI values from each month. Black dots indicate means. Gray bars indicate 10th-90th percentiles. Note the data shown are not necessarily chronologically. See also Supplementary Figures S2, S4.
4 Discussion
In this project, four NRS recording sites located in the eastern Pacific Ocean were analyzed for the presence and timing of blue and fin whale song using call energy indices. While analyses have been previously conducted to assess specific regions and associated blue and fin whale populations (Širović et al., 2015; Haver et al., 2020; Oestreich et al., 2020a; Rice et al., 2021; Oestreich et al., 2022), this study expanded the application to a broader spatial scale.
4.1 Seasonal variation
The presence of blue whale B calls, as proxied by CI, varied across the sites and seasons. The late fall and early winter peak in blue whale CI at the Gulf of Alaska and Olympic Coast recording sites (Figure 5) is supported by the presence of blue whale B calls documented from October to February at sites off Oregon, Washington, and Vancouver Island (Burtenshaw et al., 2004). Burtenshaw et al. (2004) did not show discernable peaks. This could reflect a real change in blue whale distribution and/or behavior through time due to changes in ocean conditions that drive prey availability (Bailey et al., 2009; Calambokidis et al., 2009; Derville et al., 2022). Alternatively, this could result from differences in measurement locations and metrics.
Patterns detected at Gulf of Alaska and Olympic Coast during 2019–2020 (Figures 5A, C) show a lag in the disappearance of blue whale CI signal at the Olympic Coast (February) compared to the Gulf of Alaska (January). This could indicate southward movement of at least part of the NE Pacific population from the Gulf of Alaska down the eastern margin of the North Pacific as part of the expected winter migration, but could also arise from changes in vocal behavior rather than movement. Increased background noise, particularly at 50 Hz, in November for the Olympic Coast 2019–2020 deployment resulted in masking of a rise in signal during that month thereby driving down the CI value. It is likely the noise masked a rise in blue whale signal but it also reduced the detection area so the precise timing of peak calling is not known (See Supplementary Figure S1C). The onset of CI values above 1.01 in the summer at Cordell Bank (Figure 5D) and Channel Islands (Figures 5E, F, with the 2018–2020 deployment having a very slight trend) aligns with previous studies that observed blue whale B calls from approximately June to January off Southern California (Burtenshaw et al., 2004; Širović et al., 2015; Szesciorka et al., 2020) and July to January off Central California (Oestreich et al., 2020a; Oestreich et al., 2022). B calls recorded at these sites could capture 1) the northward migration of blue whales during late spring and early summer as well as the southward migration during late fall and early winter or 2) a single, extended migration period that spans late spring to early winter.
Cordell Bank is notable for the high magnitude of blue whale CI values relative to other recording sites (Figure 5), which could be a byproduct of one or multiple factors. For one, Cordell Bank and the nearby Gulf of the Farallones are critical foraging areas for the NE Pacific blue whale population (Calambokidis et al., 2015), meaning that higher CI values could result simply from a large number of singing individuals occupying this region during the summer-fall foraging season. However, the Channel Islands are also a notable foraging ground for this population (Calambokidis et al., 2015), yet relatively low CI values were recorded in this region (Figures 5E, F), indicating that differences in abundance between sites alone likely does not fully explain the high CI values documented at Cordell Bank. A more likely explanation is the relative proximity of singing individuals to each hydrophone, which can influence received levels in the target frequency bands used in the CI calculation. More specifically, the Cordell Bank hydrophone (NRS11) is located near the southern border of Cordell Bank National Marine Sanctuary close to Greater Farallones National Marine Sanctuary. The hydrophone is exposed to the open ocean and is likely well positioned to record the songs of blue whales foraging in both sanctuaries as well as offshore (Haver et al., 2020). In contrast, the Channel Islands hydrophone (NRS05) is located south of Santa Cruz Island that may block sound propagating from north of Santa Cruz Island, which has previously been observed as the most-frequented by blue whales via visual (Calambokidis et al., 2015), biologging (Bailey et al., 2009), and passive acoustic (Širović et al., 2015) methods. Therefore, the Channel Islands results are likely influenced by the placement of the hydrophone and surrounding bathymetric structure, which limits the detection range, as opposed to a true lack of blue whales producing B calls in this region (Helble et al., 2013).
Fin whale movement in the California Current System varies from established baleen whale patterns due to the high potential for individual variation, greater localized residency, and a shift offshore during the summer and onshore during the winter (Scales et al., 2017; Derville et al., 2022). Fin whale CI documented across the four sites aligns with established patterns of strong presence between September and March from the Gulf of Alaska to California (Mizroch et al., 2009; Stafford et al., 2009; Oleson et al., 2014; Haver et al., 2020; Rice et al., 2021). Fin whales regularly winter off the coast of California, and in the Southern California Bight, a common habitat, their 20 Hz pulses were previously detected year-round with greatest sound production occurring from September to December with a peak in November (Mizroch et al., 2009; Širović et al., 2015). Year-round use of the Southern California Bight by fin whales has also been documented by tracking via satellite tags (Scales et al., 2017). Here we observed consistently longer seasonal duration of fin whale vocalizations across all sites compared to blue whales with the exception of the Channel Islands 2018–2020 deployment. Similar to seasonal patterns observed for blue whale CI, concurrent (2019–2020) deployments documented a 1-month lag in the fin whale CI signal disappearance between Gulf of Alaska (March; Figure 6A) and Olympic Coast (April; Figure 6C). This could reflect seasonal latitudinal movements of this fin whale population progressing southward in the late fall and early winter months. It should be noted that the values between November and December at Gulf of Alaska are very close and a slight increase in the noise level was recorded in December. Visual observation surveys of fin whales in the nearshore waters of Oregon identified a similar seasonal peak spanning October to February (Derville et al., 2022). The seasonality documented both visually (Derville et al., 2022) and acoustically (outlined here) could point to latitudinal or longitudinal seasonal movements (Scales et al., 2017). Longitudinal dispersion, especially away from the Channel Islands recording site, could be a driving factor behind the relatively short duration of acoustic presence identified here.
4.2 Diel variation
Distinct diel patterns of blue whale B call production have previously been linked to regional population-level foraging and migration behaviors. Wiggins et al. (2005) observed a strong tendency toward nighttime B call production in the Southern California Bight during summer and fall, which was hypothesized to result from a tradeoff between blue whales’ singing and foraging, which occurs primarily during daytime at depth. More recently, Oestreich et al. (2020a) confirmed this hypothesis using a combination of passive acoustic and bio-logging analyses. Further, the transition from more intense nighttime song detection to more even diel distribution of song detection (i.e., a drop in CInight:CIday from values greater than 1.0 to near or below 1.0) has been linked to the transition from foraging to southward migration (Oestreich et al., 2020a) and was observed in the Monterey Bay region every year from 2015 to 2021 (Oestreich et al., 2022). These links between diel patterning in blue whale song detection (as measured via CI) and other behaviors make CInight:CIday a useful tool for further exploring the seasonal patterns in behavior described in the preceding section. While similar links between diel patterning of song and other behaviors have not been established for fin whales, we also explore CInight:CIday for fin whales in the present study to establish a baseline understanding that can provide a foundation for future investigations of the behavioral context of fin whale call production. For both blue and fin whale call types evaluated here, a wide spread of CInight:CIday values was recorded at all sites. As such, the trends outlined below should be interpreted cautiously and the differences between CI values at night and day likely depend on many factors.
For blue whales, seasonal patterns of CInight:CIday varied across sites though all but the Channel Islands 2018–2020 deployment showed a consistently higher intensity at night compared to day for months where CI was above 1.01 (Figure 5, Supplementary Figure S3). This pattern is supported by Oestreich et al. (2020a) where blue whale B calls in Monterey Bay tended toward nighttime song detection (CInight:CIday > 1) during the summer onset and fall peak in song intensity. Oestreich et al. (2020a), Oestreich et al. (2022) also noted a decrease in CInight:CIday toward 1 during the fall-winter drop in song intensity and associated this combination of seasonal and diel patterning to the regional population-level transition to migration, but noted that their findings from Monterey Bay might or might not apply to other foraging locations along the west coast of North America. The present study does not provide strong evidence of similar patterning for a drop in preferential nighttime song intensity as CI decreases in the fall-winter at the four recording sites. This lack of a clear acoustic indication of the transition from foraging to migration might result from one or multiple factors. For one, monthly variation in noise contributions including across day and night influence the CI and CInight:CIday patterns. This is particularly evident at the Olympic Coast 2019–2020 site. In addition to the influence of noise levels, the Channel Islands in particular are at a more central point along the migratory pathway of this blue whale population, and therefore are likely occupied simultaneously by animals foraging in, moving northward, and migrating southward through this region. This mix of behavioral modes across the singing individuals effectively sampled by this hydrophone would likely obscure clear diel patterns previously associated with distinct foraging and migratory behavioral states. Further, as noted previously, this Channel Islands hydrophone is not well-positioned to record the typical foraging habitat of blue whales in this region. This might result in a bias toward recording non-foraging, transiting blue whales that typically produce calls with a more even diel distribution (e.g., CInight:CIday near 1.0), which is consistent with our results for the Channel Islands (Figures 5E, F). This finding suggests that further individual-level investigation of diel patterning in blue whale singing and foraging behavior (Oestreich et al., 2020a) is warranted across foraging locations.
For fin whales, CInight:CIday values were consistently near 1.0 across most recording sites and seasons (Figure 6), indicating a relatively even distribution throughout the day and night across the year. The Olympic Coast sites were notable outliers to this trend, with elevated monthly median CInight:CIday values during the winter (Figure 6B) and early spring (Figure 6C), indicating greater fin whale song intensity at night compared to day during these periods. While less is currently known about the behavioral context of song detection diel patterns in fin whales relative to blue whales, Stimpert et al. (2015) previously showed that fin whales typically produce song on shallow non-foraging dives, including during the daytime. Combined with the lack of clear diel patterning in song detection shown here (Figure 6), this suggests that fin whale foraging behavior is not quite as diurnally-biased as blue whale foraging.
These findings on the lack of seasonality in diel song patterning by fin whales provide a foundation for future studies to further refine understanding of the behavioral context of fin whale song detection, likely via bio-logging analyses (Stimpert et al., 2015; Oestreich et al., 2020a).
4.3 Additional parameters to consider
PAM provides an opportunity to analyze soundscapes and soniferous species present over long periods of time (Au and Hastings, 2008). PAM stationary recording systems, such as the ones used here, support high temporal resolution (with continuous coverage in one area) while mobile PAM platforms have increased spatial coverage but record for a short window in a single location. The combination of both stationary and mobile recording systems would provide complimentary information that covers change in a single place as well as snapshots of a broader area during that time. This additional coverage could help to elucidate patterns that are potentially muted (or otherwise influenced) due to the placement of a stationary hydrophone.
The CI method applied here was previously developed to efficiently extract specific call types (B calls and 20 Hz pulses) simultaneously to maximize the chance of detecting whales in large volumes of passive acoustic data. Missed detections may occur when the selected background frequencies and/or the signal frequencies are overwhelmed by sounds from other sources. The influence of anthropogenic sound sources such as vessel noise is prevalent at the Olympic Coast, Cordell Bank, and Channel Islands recording locations, with large container ships being particularly dominant and producing sound at frequencies that overlap with blue and fin whale calls (McKenna et al., 2013; Haver et al., 2018; Haver et al., 2020; Haver et al., 2021). Therefore, the potential for masking could be high in these locations resulting in an underestimate of call presence and reduced communication space for these species (Clark et al., 2009; Hatch et al., 2012; Cholewiak et al., 2018). However, we do not expect that such masking biases the broader temporal patterns in blue and fin whale song described here (Figures 5, 6) due to the coarse temporal binning applied and the relatively constant background levels when averaged across months and between night and day for each month (Supplementary Figures S1–S4). This is further supported by previous work showing that, when present, blue whale song as represented by the CI target frequency bands is discernable above the CI background frequency bands (Oestreich et al., 2020a, Supplementary Figure S1). However, changes in the intensity of the background frequencies will influence the detection area and potentially impact calling behavior through varying call intensity and/or calling rate as well as the perceived pattern of calling using CI. Therefore, the site-specific and seasonal levels in background noise need to be heavily considered when applying this technique to different sites and interpreting those results. Cross-referencing blue and fin whale vocalizations, and vessel noise frequency bands with vessel tracking data could assist in a broader understanding of the low-frequency soundscape drivers at the NRS sites (Haver et al., 2020; Haver et al., 2021).
While CI documents the acoustic presence of a specific call type for each species, capturing the arrival at a site when whales are not acoustically active or producing a different call type would require the inclusion of other observational methods, such as visual sightings, and expanding to acoustic detection of additional call types. Blue whale D calls, which have been associated with foraging, have been recorded earlier in the year (April/May to September/November) compared to B calls (June/July to December/January) and could help to document a more complete presence of this species in an area (Oleson et al., 2007b; Szesciorka et al., 2020). In addition to the 20 Hz pulse calls that are associated with reproductive or social behavior, fin whales produce a call at 40 Hz more frequently when foraging (Širović et al., 2013). Inclusion of these additional call types would support monitoring of foraging and migratory timing. Still, CI is an excellent tool to pair with long-term continuous data (e.g., NRS and the Monterey Accelerated Research System cabled observatory) to efficiently extract reproductive function call types that can provide important information about whale presence, and in some cases, more specific information on foraging and migratory behavior.
For deployments covering multiple annual cycles (Cordell Bank 2015–2017 (Figures 5D, 6D) and Channel Islands 2018–2020 (Figures 5F, 6F), average annual trends were similar to the individual years that contributed to the full deployment for both blue and fin whale (Supplementary Figures S5, S6). Overall seasonal patterns were similar but CI intensity varied across the years. A notable exception was interannual variability observed for fin whale results at Channel Islands between 2018 and 2019 (Supplementary Figure S6). Across disparate deployments, Olympic Coast blue whale results varied between 2014–2015 and 2019–2020, and Channel Islands fin whale results varied between 2014–2015 and 2018–2019. A potential cause for this interannual variability is the change in oceanographic conditions. Variation in (sub)mesoscale biophysical influences (e.g., upwelling and fronts that drive prey availability and aggregation) and large scale variations in the atmosphere and ocean (e.g., the Pacific Decadal Oscillation and El Niño Southern Oscillation) impact blue and fin whale migration patterns and arrival at feeding grounds, which further influence acoustic presence and behavioral observations (Burtenshaw et al., 2004; Calambokidis et al., 2009; Stafford et al., 2009; Scales et al., 2017; Benoit-Bird et al., 2019; Szesciorka et al., 2020; Cade et al., 2021). Inclusion of environmental parameters that quantify habitat suitability and prey abundance in the context of conditions that deviate from an anticipated norm (e.g., anomalies derived from climatological data) in addition to longer time series of whale behavior are needed to understand drivers behind the interannual variation in the CI measured for blue and fin whales.
4.4 Long-term monitoring
Long-term PAM is essential in assessing spatio-temporal patterns of soundscapes, ecosystems, and species (e.g., Davis et al., 2020; McKenna et al., 2021; Rice et al., 2021). As such it is an important tool for NOAA and similar agencies to address conservation needs (Gedamke et al., 2016), and analyses like this study provide important information for reporting on conditions and evaluating changes over time. For example, continued monitoring of low-frequency soundscapes and whale vocalization activity, especially during periods of anthropogenic noise fluctuation, provides insight into the effects of increasing levels of background noise on the acoustic habitat of blue and fin whales (Ryan et al., 2021). Analysis tools, such as CI for blue and fin whale monitoring, are an important data stream for NRS and related long-term sound projects due to their efficiency, and can be used in tandem with other methods for processing long-term continuous data to monitor conditions and trends. Centralized repositories and global access to soundscape data and data products will in turn facilitate future research and collaboration (Wall et al., 2021).
4.5 Broader impacts
This paper used efficient acoustic metrics to study the seasonality of blue and fin whale song along the U.S. west coast, and in doing so contributes to the scientific knowledge on the presence and behavioral patterns of these endangered species. By studying these aspects of blue and fin whale activity, scientists can provide information to managers and policymakers and help inform management of the ecosystems that these endangered species inhabit (Agardy, 2000; Scholik-Schlomer et al., 2009; Wiley et al., 2011; Calambokidis et al., 2015; Haver et al., 2020; Guan et al., 2021). The areas sampled acoustically in this study include a number of marine protected areas (MPAs) including national marine sanctuaries (NMS). Anthropogenic threats to blue and fin whales across the U.S. west coast ecosystems sampled in this study include the impacts of anthropogenic noise on these soniferous species’ effective communication space, collisions between vessels and whales, and entanglement in fishing gear. The present study provides insight on the regional-scale seasonal patterns of blue and fin whale presence and behavior required for cross-NMS coordination and effective management strategies to mitigate these varied threats. More specifically, vessel speed reduction programs (Abramson et al., 2011; Silber and Bettridge, 2012; Conn and Silber, 2013; Pine et al., 2018) provide a management tool to help mitigate anthropogenic threats to blue and fin whales. Seasonal and location-specific dynamic management approaches (Lewison et al., 2015; Maxwell et al., 2015; Oestreich et al., 2020b), which implement vessel speed reductions only during the times of year when interaction with endangered whale populations is most likely (Figures 5, 6) can further enhance the efficacy and adoptability of such programs. Coordination of these actions across NMS covering the broad range and migratory routes of blue and fin whales along the U.S. west coast can assist in the movement toward Marine Protected Corridors, which are critical to the conservation of migratory marine populations (Hyrenbach et al., 2000; Johnson et al., 2022).
Author’s note
This project was made possible via publicly-available data (NRS) and code (Oestreich W. K. et al., 2020; https://github.com/woestreich/blue-whale-migration).
Data availability statement
Publicly available datasets were analyzed in this study. The data can be found here: https://www.ncei.noaa.gov/maps/passive-acoustic-data/.
Ethics statement
Ethical review and approval was not required for the animal study because hydrophones were stationary and did not interfere with animals of study or their environment.
Author contributions
RD and JG conceived and designed the NRS. CW, SH, WO, and JR designed the manuscript. EP and CW processed and analyzed data, and prepared figures. EP, CW, and WO drafted the manuscript. All authors contributed to the article and approved the submitted version.
Funding
This research was supported by NOAA Fisheries, the University of Colorado Undergraduate Research Opportunity, and NOAA cooperative agreement NAO17OAR4320101. Publication of this article was funded by the University of Colorado Boulder Libraries.
Acknowledgments
The authors thank Leila Hatch, Sofie Van Parijs, Joe Haxel, Dani Lipski, Lauren Roche, Haru Matsumoto, Megan McKenna, Lindsey Peavey, and Jenny Waddell for their contributions to the NRS project and acoustic data collection at the four NRS sites included in this analysis. This paper is NOAA-PMEL contribution number 5396. This paper was enhanced by the helpful contributions of two reviewers.
Conflict of interest
The authors declare that the research was conducted in the absence of any commercial or financial relationships that could be construed as a potential conflict of interest.
Publisher’s note
All claims expressed in this article are solely those of the authors and do not necessarily represent those of their affiliated organizations, or those of the publisher, the editors and the reviewers. Any product that may be evaluated in this article, or claim that may be made by its manufacturer, is not guaranteed or endorsed by the publisher.
Supplementary material
The Supplementary Material for this article can be found online at: https://www.frontiersin.org/articles/10.3389/frsen.2023.994518/full#supplementary-material
Footnotes
1https://github.com/MarineBioAcousticsRC/Triton.
References
Abrahms, B., Hazen, E. L., Aikens, E. O., Savoca, M. S., Goldbogen, J. A., Bograd, S. J., et al. (2019). Memory and resource tracking drive blue whale migrations. Proc. Natl. Acad. Sci. 116 (12), 5582–5587. doi:10.1073/pnas.1819031116
Abramson, L., Polefka, S., Hastings, S., and Bor, K. (2011). “Reducing the threat of ship strikes on large cetaceans in the Santa barbara channel region and Channel Islands national marine sanctuary,” in NOAA marine sanctuaries conservation series ONMS, 11-01 (Boulder). Available at: https://repository.library.noaa.gov/view/noaa/30771.
Agardy, T. (2000). Information needs for marine protected areas: Scientific and societal. Bull. Mar. Sci. 66 (3), 875–888.
Bailey, H., Mate, B. R., Palacios, D. M., Irvine, L., Bograd, S. J., and Costa, D. P. (2009). Behavioural estimation of blue whale movements in the Northeast Pacific from state-space model analysis of satellite tracks. Endanger. Species Res. 10, 93–106. doi:10.3354/esr00239
Baumgartner, M. F., Stafford, K. M., and Latha, G. (2018). “Near real-time underwater passive acoustic monitoring of natural and anthropogenic sounds,” in Observing the oceans in real time, 203–226.
Benoit-Bird, K. J., Waluk, C. M., and Ryan, J. P. (2019). Forage species swarm in response to coastal upwelling. Geophys. Res. Lett. 46 (3), 1537–1546. doi:10.1029/2018gl081603
Browning, E., Gibb, R., Glover-Kapfer, P., and Jones, K. E. (2017). Passive acoustic monitoring in ecology and conservation. WWF Conserv. Technol. Ser. 1 (2), 1–75.
Burtenshaw, J. C., Oleson, E. M., Hildebrand, J. A., McDonald, M. A., Andrew, R. K., Howe, B. M., et al. (2004). Acoustic and satellite remote sensing of blue whale seasonality and habitat in the Northeast Pacific. Deep Sea Res. Part II Top. Stud. Oceanogr. 51 (10-11), 967–986. doi:10.1016/s0967-0645(04)00095-5
Cade, D. E., Fahlbusch, J. A., Oestreich, W. K., Ryan, J., Calambokidis, J., Findlay, K. P., et al. (2021). Social exploitation of extensive, ephemeral, environmentally controlled prey patches by supergroups of rorqual whales. Anim. Behav. 182, 251–266. doi:10.1016/j.anbehav.2021.09.013
Calambokidis, J., Barlow, J., Ford, J. K., Chandler, T. E., and Douglas, A. B. (2009). Insights into the population structure of blue whales in the Eastern North Pacific from recent sightings and photographic identification. Mar. Mammal Sci. 25 (4), 816–832. doi:10.1111/j.1748-7692.2009.00298.x
Calambokidis, J., Steiger, G. H., Curtice, C., Harrison, J., Ferguson, M. C., Becker, E., et al. (2015). 4. Biologically important areas for selected cetaceans within US waters-west coast region. Aquat. Mamm. 41 (1), 39–53. doi:10.1578/am.41.1.2015.39
Cholewiak, D., Clark, C. W., Ponirakis, D., Frankel, A., Hatch, L. T., Risch, D., et al. (2018). Communicating amidst the noise: Modeling the aggregate influence of ambient and vessel noise on baleen whale communication space in a national marine sanctuary. Endanger. Species Res. 36, 59–75. doi:10.3354/esr00875
Clark, C. W., Ellison, W. T., Southall, B. L., Hatch, L., Van Parijs, S. M., Frankel, A., et al. (2009). Acoustic masking in marine ecosystems: Intuitions, analysis, and implication. Mar. Ecol. Prog. Ser. 395, 201–222. doi:10.3354/meps08402
Clark, C. W. (1990). “Acoustic behavior of mysticete whales,” in Sensory abilities of cetaceans (Boston, MA: Springer), 571–583.
Conn, P. B., and Silber, G. K. (2013). Vessel speed restrictions reduce risk of collision-related mortality for North Atlantic right whales. Ecosphere 4 (4), art43–16. doi:10.1890/es13-00004.1
Croll, D. A., Clark, C. W., Acevedo, A., Tershy, B., Flores, S., Gedamke, J., et al. (2002). Only male fin whales sing loud songs. Nature 417 (6891), 809. doi:10.1038/417809a
Davis, G. E., Baumgartner, M. F., Corkeron, P. J., Bell, J., Berchok, C., Bonnell, J. M., et al. (2020). Exploring movement patterns and changing distributions of baleen whales in the Western North Atlantic using a decade of passive acoustic data. Glob. change Biol. 26 (9), 4812–4840. doi:10.1111/gcb.15191
Derville, S., Barlow, D. R., Hayslip, C., and Torres, L. G. (2022). Seasonal, annual, and decadal distribution of three rorqual whale species relative to dynamic ocean conditions off Oregon, USA. Front. Mar. Sci. 9, 868566. doi:10.3389/fmars.2022.868566
Gedamke, J., Gales, N., Hildebrand, J., and Wiggins, S. (2007). Seasonal occurrence of low frequency whale vocalisations across eastern Antarctic and southern Australian waters. White Pap. Present. Sci. Comm. Int. Whal. Comm. 21, 30.
Gedamke, J., Harrison, J., Hatch, L., Angliss, R., Barlow, J., Berchok, C., et al. (2016). Ocean noise strategy roadmap. Silver Spring: US National Oceanic and Atmospheric Administration.
Guan, S., Brookens, T., and Vignola, J. (2021). Use of underwater acoustics in marine conservation and policy: Previous advances, current status, and future needs. J. Mar. Sci. Eng. 9 (2), 173. doi:10.3390/jmse9020173
Hatch, L. T., Clark, C. W., Van Parijs, S. M., Frankel, A. S., and Ponirakis, D. W. (2012). Quantifying loss of acoustic communication space for right whales in and around a US National Marine Sanctuary. Conserv. Biol. 26 (6), 983–994. doi:10.1111/j.1523-1739.2012.01908.x
Haver, S. M., Gedamke, J., Hatch, L. T., Dziak, R. P., Van Parijs, S., McKenna, M. F., et al. (2018). Monitoring long-term soundscape trends in US waters: The NOAA/NPS Ocean Noise reference station network. Mar. Policy 90, 6–13. doi:10.1016/j.marpol.2018.01.023
Haver, S. M., Fournet, M. E., Dziak, R. P., Gabriele, C., Gedamke, J., Hatch, L. T., et al. (2019). Comparing the underwater soundscapes of four US national Parks and marine sanctuaries. Front. Mar. Sci. 6, 500. doi:10.3389/fmars.2019.00500
Haver, S. M., Rand, Z., Hatch, L. T., Lipski, D., Dziak, R. P., Gedamke, J., et al. (2020). Seasonal trends and primary contributors to the low-frequency soundscape of the Cordell Bank National marine sanctuary. J. Acoust. Soc. Am. 148 (2), 845–858. doi:10.1121/10.0001726
Haver, S. M., Adams, J. D., Hatch, L. T., Van Parijs, S. M., Dziak, R. P., Haxel, J., et al. (2021). Large vessel activity and low-frequency underwater sound benchmarks in United States waters. Front. Mar. Sci. 8. doi:10.3389/fmars.2021.669528
Haxel, J. H., Dziak, R. P., and Matsumoto, H. (2013). Observations of shallow water marine ambient sound: The low frequency underwater soundscape of the central Oregon coast. J. Acoust. Soc. Am. 133 (5), 2586–2596. doi:10.1121/1.4796132
Helble, T. A., D'Spain, G. L., Hildebrand, J. A., Campbell, G. S., Campbell, R. L., and Heaney, K. D. (2013). Site specific probability of passive acoustic detection of humpback whale calls from single fixed hydrophones. J. Acoust. Soc. Am. 134 (3), 2556–2570.
Hyrenbach, K. D., Forney, K. A., and Dayton, P. K. (2000). Marine protected areas and ocean basin management. Aquatic conservation Mar. Freshw. Ecosyst. 10 (6), 437–458.
Johnson, C. M., Reisinger, R. R., Palacios, D. M., Friedlaender, A. S., Zerbini, A. N., Willson, A., et al. (2022). Protecting Blue Corridors - challenges and solutions for migratory whales navigating national and international seas. Zenodo. doi:10.5281/zenodo.6196131
Koblick, D. (2020). Vectorized solar azimuth and elevation estimation, MATLAB Cent. File Exch. Available at: https://www.mathworks.com/matlabcentral/fileexchange/23051-vectorized-solar-azimuth-and-elevation-estimation.
Lewis, L. A., and Širović, A. (2018). Variability in blue whale acoustic behavior off southern California. Mar. Mammal Sci. 34 (2), 311–329.
Lewis, L. A., Calambokidis, J., Stimpert, A. K., Fahlbusch, J., Friedlaender, A. S., McKenna, M. F., et al. (2018). Context-dependent variability in blue whale acoustic behaviour. R. Soc. open Sci. 5 (8), 180241. doi:10.1098/rsos.180241
Lewison, R., Hobday, A. J., Maxwell, S., Hazen, E., Hartog, J. R., Dunn, D. C., et al. (2015). Dynamic ocean management: Identifying the critical ingredients of dynamic approaches to ocean resource management. BioScience 65 (5), 486–498. doi:10.1093/biosci/biv018
Mate, B. R., Lagerquist, B. A., and Calambokidis, J. (1999). Movements of North Pacific blue whales during the feeding season off southern California and their southern fall migration 1. Mar. Mammal Sci. 15 (4), 1246–1257. doi:10.1111/j.1748-7692.1999.tb00888.x
Maxwell, S. M., Hazen, E. L., Lewison, R. L., Dunn, D. C., Bailey, H., Bograd, S. J., et al. (2015). Dynamic ocean management: Defining and conceptualizing real-time management of the ocean. Mar. Policy 58, 42–50. doi:10.1016/j.marpol.2015.03.014
McDonald, M. A., and Fox, C. G. (1999). Passive acoustic methods applied to fin whale population density estimation. J. Acoust. Soc. Am. 105 (5), 2643–2651. doi:10.1121/1.426880
McDonald, M. A., Mesnick, S. L., and Hildebrand, J. A. (2006). Biogeographic characterization of blue whale song worldwide: Using song to identify populations. J. cetacean Res. Manag. 8 (1), 55–65.
McKenna, M. F., Wiggins, S. M., and Hildebrand, J. A. (2013). Relationship between container ship underwater noise levels and ship design, operational and oceanographic conditions. Sci. Rep. 3 (1), 1760–1810. doi:10.1038/srep01760
McKenna, M. F., Baumann-Pickering, S., Kok, A., Oestreich, W. K., Adams, J. D., Barkowski, J., et al. (2021). Advancing the interpretation of shallow water marine soundscapes. Front. Mar. Sci. 8. doi:10.3389/fmars.2021.719258
Mellinger, D. K., Küsel, E. T., Thomas, L., and Marques, T. A. (2009). Taming the Jez monster: Estimating fin whale spatial density using acoustic propagation modeling. J. Acoust. Soc. Am. 126 (4), 2229. doi:10.1121/1.3248985
Mizroch, S. A., Rice, D. W., Zwiefelhofer, D., Waite, J., and Perryman, W. L. (2009). Distribution and movements of fin whales in the North Pacific ocean. Mammal. Rev. 39 (3), 193–227. doi:10.1111/j.1365-2907.2009.00147.x
NOAA OAR Pacific Marine Environmental LaboratoryNational Marine Fisheries ServiceNOS Office of National Marine SanctuariesDOI NPS Natural Resource Stewardship and Science Directorate (2014). NOAA Ocean Noise reference station network raw passive acoustic data. Boulder: NOAA National Centers for Environmental Information.
Oestreich, W. K., Fahlbusch, J. A., Cade, D. E., Calambokidis, J., Margolina, T., Joseph, J., et al. (2020a). Animal-borne metrics enable acoustic detection of blue whale migration. Curr. Biol. 30 (23), 4773–4779.e3. doi:10.1016/j.cub.2020.08.105
Oestreich, W. K., Chapman, M. S., and Crowder, L. B. (2020b). A comparative analysis of dynamic management in marine and terrestrial systems. Front. Ecol. Environ. 18 (9), 496–504. doi:10.1002/fee.2243
Oestreich, W. K., Abrahms, B., McKenna, M. F., Goldbogen, J. A., Crowder, L. B., and Ryan, J. P. (2022). Acoustic signature reveals blue whales tune life-history transitions to oceanographic conditions. Funct. Ecol. 36, 882–895. doi:10.1111/1365-2435.14013
Oleson, E. M., Calambokidis, J., Burgess, W. C., McDonald, M. A., LeDuc, C. A., and Hildebrand, J. A. (2007a). Behavioral context of call production by eastern North Pacific blue whales. Mar. Ecol. Prog. Ser. 330, 269–284. doi:10.3354/meps330269
Oleson, E. M., Wiggins, S. M., and Hildebrand, J. A. (2007b). Temporal separation of blue whale call types on a southern California feeding ground. Anim. Behav. 74 (4), 881–894. doi:10.1016/j.anbehav.2007.01.022
Oleson, E. M., Širović, A., Bayless, A. R., and Hildebrand, J. A. (2014). Synchronous seasonal change in fin whale song in the North Pacific. PloS one 9 (12), e115678. doi:10.1371/journal.pone.0115678
Parks, S. E., Cusano, D. A., Stimpert, A. K., Weinrich, M. T., Friedlaender, A. S., and Wiley, D. N. (2014). Evidence for acoustic communication among bottom foraging humpback whales. Sci. Rep. 4 (1), 7508–7517. doi:10.1038/srep07508
Pine, M. K., Hannay, D. E., Insley, S. J., Halliday, W. D., and Juanes, F. (2018). Assessing vessel slowdown for reducing auditory masking for marine mammals and fish of the Western Canadian Arctic. Mar. Pollut. Bull. 135, 290–302. doi:10.1016/j.marpolbul.2018.07.031
Rankin, S., Barlow, J., and Stafford, K. M. (2006). Blue whale (Balaenoptera musculus) sightings and recordings south of the Aleutian Islands. Mar. Mammal Sci. 22 (3), 708–713. doi:10.1111/j.1748-7692.2006.00054.x
Rice, A., Širović, A., Trickey, J. S., Debich, A. J., Gottlieb, R. S., Wiggins, S. M., et al. (2021). Cetacean occurrence in the Gulf of Alaska from long-term passive acoustic monitoring. Mar. Biol. 168 (5), 72–29. doi:10.1007/s00227-021-03884-1
Romagosa, M., Pérez-Jorge, S., Cascão, I., Mouriño, H., Lehodey, P., Pereira, A., et al. (2021). Food talk: 40-Hz fin whale calls are associated with prey biomass. Proc. R. Soc. B 288, 20211156. doi:10.1098/rspb.2021.1156
Ryan, J. P., Joseph, J. E., Margolina, T., Hatch, L. T., Azzara, A., Reyes, A., et al. (2021). Reduction of low-frequency vessel noise in Monterey Bay national marine sanctuary during the COVID-19 pandemic. Front. Mar. Sci. 8, 587. doi:10.3389/fmars.2021.656566
Scales, K. L., Schorr, G. S., Hazen, E. L., Bograd, S. J., Miller, P. I., Andrews, R. D., et al. (2017). Should I stay or should I go? Modelling year-round habitat suitability and drivers of residency for fin whales in the California current. Divers. Distributions 23 (10), 1204–1215. doi:10.1111/ddi.12611
Scholik-Schlomer, A. R., Guan, S., and Harrison, J. (2009). Role of the national marine Fisheries Service in underwater acoustics: Integration of science and policy. J. Acoust. Soc. Am. 125 (4), 2518. doi:10.1121/1.4783464
Silber, G. K., and Bettridge, S. O. M. (2012). An assessment of the final rule to implement vessel speed restrictions to reduce the threat of vessel collisions with North Atlantic right whales. NOAA technical memorandum NMFS-OPR, 48. Available at: https://repository.library.noaa.gov/view/noaa/4207.
Širović, A., and Hildebrand, J. A. (2011). Using passive acoustics to model blue whale habitat off the Western Antarctic Peninsula. Deep Sea Res. Part II Top. Stud. Oceanogr. 58 (13-16), 1719–1728. doi:10.1016/j.dsr2.2010.08.019
Širović, A., Hildebrand, J. A., Wiggins, S. M., and Thiele, D. (2009). Blue and fin whale acoustic presence around Antarctica during 2003 and 2004. Mar. Mammal Sci. 25 (1), 125–136. doi:10.1111/j.1748-7692.2008.00239.x
Širović, A., Williams, L. N., Kerosky, S. M., Wiggins, S. M., and Hildebrand, J. A. (2013). Temporal separation of two fin whale call types across the eastern North Pacific. Mar. Biol. 160 (1), 47–57. doi:10.1007/s00227-012-2061-z
Širović, A., Rice, A., Chou, E., Hildebrand, J. A., Wiggins, S. M., and Roch, M. A. (2015). Seven years of blue and fin whale call abundance in the Southern California Bight. Endanger. Species Res. 28 (1), 61–76. doi:10.3354/esr00676
Širović, A., Oleson, E. M., Buccowich, J., Rice, A., and Bayless, A. R. (2017). Fin whale song variability in southern California and the Gulf of California. Sci. Rep. 7 (1), 10126–10211. doi:10.1038/s41598-017-09979-4
Stafford, K. M., Nieukirk, S. L., and Fox, C. G. (2001). Geographic and seasonal variation of blue whale calls in the North Pacific. J. Cetacean Res. Manag. 3 (1), 65–76.
Stafford, K. M., Citta, J. J., Moore, S. E., Daher, M. A., and George, J. E. (2009). Environmental correlates of blue and fin whale call detections in the North Pacific Ocean from 1997 to 2002. Mar. Ecol. Prog. Ser. 395, 37–53. doi:10.3354/meps08362
Stafford, K. M. (2003). Two types of blue whale calls recorded in the Gulf of Alaska. Mar. Mammal Sci. 19 (4), 682–693. doi:10.1111/j.1748-7692.2003.tb01124.x
Stimpert, A. K., DeRuiter, S. L., Falcone, E. A., Joseph, J., Douglas, A. B., Moretti, D. J., et al. (2015). Sound production and associated behavior of tagged fin whales (Balaenoptera physalus) in the Southern California Bight. Anim. Biotelemetry 3 (1), 23–12. doi:10.1186/s40317-015-0058-3
Szesciorka, A. R., Ballance, L. T., Širović, A., Rice, A., Ohman, M. D., Hildebrand, J. A., et al. (2020). Timing is everything: Drivers of interannual variability in blue whale migration. Sci. Rep. 10 (1), 7710–7719. doi:10.1038/s41598-020-64855-y
Torres, L. G. (2017). A sense of scale: Foraging cetaceans' use of scale-dependent multimodal sensory systems. Mar. Mammal Sci. 33 (4), 1170–1193. doi:10.1111/mms.12426
Tyack, P. L., and Clark, C. W. (2000). “Communication and acoustic behavior of dolphins and whales,” in Hearing by whales and dolphins (New York, NY: Springer), 156–224.
Wall, C. C., Haver, S. M., Hatch, L. T., Miksis-Olds, J., Bochenek, R., Dziak, R. P., et al. (2021). The next wave of passive acoustic data management: How centralized access can enhance science. Front. Mar. Sci. 8, 873. doi:10.3389/fmars.2021.703682
Wiggins, S. M., Oleson, E. M., McDonald, M. A., and Hildebrand, J. A. (2005). Blue whale (Balaenoptera musculus) diel call patterns offshore of Southern California. Aquat. Mamm. 31 (2), 161–168. doi:10.1578/am.31.2.2005.161
Keywords: passive acoustic monitoring, blue whale, fin whale, sound production, Northeast Pacific Ocean, ocean noise reference station network, marine bioacoustics, migration
Citation: Pearson EJ, Oestreich WK, Ryan JP, Haver SM, Gedamke J, Dziak RP and Wall CC (2023) Widespread passive acoustic monitoring reveals spatio-temporal patterns of blue and fin whale song vocalizations in the Northeast Pacific Ocean. Front. Remote Sens. 4:994518. doi: 10.3389/frsen.2023.994518
Received: 14 July 2022; Accepted: 18 January 2023;
Published: 03 February 2023.
Edited by:
Susan E. Parks, Syracuse University, United StatesReviewed by:
Mingming Liu, Institute of Deep-Sea Science and Engineering (CAS), ChinaRegina A. Guazzo, SPAWAR Systems Center Pacific (SSC Pacific), United States
Copyright © 2023 Pearson, Oestreich, Ryan, Haver, Gedamke, Dziak and Wall. This is an open-access article distributed under the terms of the Creative Commons Attribution License (CC BY). The use, distribution or reproduction in other forums is permitted, provided the original author(s) and the copyright owner(s) are credited and that the original publication in this journal is cited, in accordance with accepted academic practice. No use, distribution or reproduction is permitted which does not comply with these terms.
*Correspondence: Carrie C. Wall, carrie.wall@noaa.gov
†These authors have contributed equally to this work and share first authorship
‡Present address: Monterey Bay Aquarium Research Institute, Moss Landing, CA, United States