- 1Scottish Association for Marine Science (SAMS), Oban, United Kingdom
- 2APEM Limited, Heaton Mersey, United Kingdom
- 3Marine Scotland Science, Aberdeen, United Kingdom
- 4University of Plymouth, Plymouth, United Kingdom
The characterisation of marine soundscapes allows observation of spatiotemporal distribution of vocalising species and human activities, which can inform an assessment of their interactions. Such data are important for monitoring the ecological status of marine habitats. The Scottish Atlantic Frontier is an important habitat for a variety of cetacean species. Historic whaling has heavily impacted several species inhabiting these waters and current comprehensive information about seasonal occurrence and distribution is lacking for all species. This study presents year-round passive acoustic monitoring data from ten sites in this understudied region. The three most offshore sites were examined for baleen whale vocalisations, and four species were regularly detected. Fin whale detections peaked from October to January and were at their lowest during May and June. Humpback whale song was detected as early as January but showed a strong seasonal peak in March and April. In contrast, minke whales were detected regularly throughout the year but with a peak in detections from October to November, when sei whales were also detected. All monitoring sites showed frequent occurrence of odontocete echolocation clicks and whistles. Comprised mainly of delphinid vocalisations, whistles and clicks were detected on an almost-daily basis among the offshore sites, with a slight reduction in detections from May to July particularly among the more inshore sites. Ambient sound levels (root-mean-square sounds pressure level; SPL) varied by site and season in relation to species presence, anthropogenic contributions, and environmental conditions. Monthly median SPL across the array varied up to 18 dB within 1/3-octave bands. Throughout the year, variability in median SPL was lowest in the higher frequency bands (>10 kHz), while highest variability was found between January to July in specific lower frequency bands (<1 kHz). Results from this study demonstrate the value of passive acoustic data in providing novel baseline information about cetacean occurrence and distribution in Scottish offshore habitats where data are limited and outdated. The results will feed into statutory reporting on underwater noise, support the identification and designation of future marine protected areas for cetaceans, and help guide management of future human-marine mammal interactions in Scottish offshore waters.
1 Introduction
Geographical range shifts of marine species in response to global climate change and other human impacts are now widely recognised and have been described in several taxa (Pinsky et al., 2013; Gulland et al., 2022; Orgeret et al., 2022). In the North Atlantic, changes in prey distribution underlying the movements of top predators such as marine mammals have been linked to changes in the Atlantic Meridional Overturning Circulation (AMOC), which in turn are linked to climate change (Record et al., 2019). In mid-latitudes of the eastern North Atlantic there is evidence of northward range expansion for more temperate-water species, such as short-beaked common dolphins (Delphinus delphis) and striped dolphins (Stenella coeruleoalba), and possibly a range reduction of cold-water species such as white-beaked dolphins (Lagenorhynchus albirostris) due to climate change (MacLeod et al., 2008; Evans and Waggitt, 2020). Modelling of cetaceans stranded in the United Kingdom (UK) over the last 30 years showed a general proportional increase in strandings of warm-water adapted species (Williamson et al., 2021), indicating ongoing changes in species distribution as part of wider ecological regime shifts due to climate change-driven environmental changes in the Northeast Atlantic (Vollset et al., 2022).
The marine region to the west and north of Scotland and Ireland, also referred to as the Atlantic Frontier, is oceanographically and topographically complex (Pollock et al., 2000). The region is influenced by the North Atlantic Current, and the slope current at the shelf edge, which show mixing with the upper layers of the eastern Rockall Trough (Ellett et al., 1986; Jones et al., 2018). Mixing and upwelling, due to diverging currents and water mass interactions with the ocean floor, collectively transport nutrients from deeper waters to the surface and in turn create highly productive marine habitats. Together, the complex bathymetry and diverse oceanographic features of the Scottish shelf edge create productive habitats and regions of increased prey availability for a variety of species groups, including cetaceans (Weir et al., 2001). At least 20 species of cetaceans have been documented in the area, although information about their seasonal occurrence and abundance is largely absent or outdated (Charif and Clark, 2000; Charif and Clark, 2009).
Many cetacean species recorded in this region were hunted from seven shore-based whaling stations located in Shetland, the Hebrides and Ireland during the first part of the 20th century (Thompson, 1928), which led to the severe depletion of several baleen whale species and the likely extinction of the North Atlantic right whale (Eubalaena glacialis) in this region (Clapham et al., 1999).
While “Atlantic Frontier surveys” to the north and west of Scotland have been carried out since 1979 (Pollock et al., 2000; Weir et al., 2001), due to the remote location of the region, understanding of cetacean distribution in these areas is still comparatively poor and large-scale cetacean abundance surveys of the region have been rare and seasonally restricted. Some data were collected during the European wide SCANS-II and SCANS-III surveys (in 2005 and 2016 respectively), as well as the 2007 CODA survey. These surveys used ships and planes to survey waters both on the continental shelf and beyond the shelf edge (Hammond et al., 2009; Hammond et al., 2013; Hammond et al., 2021). These snapshot surveys provided useful data on species presence and abundance on a multi-year to decadal timescale but were not designed to assess shorter-term (e.g. seasonal) changes in species distribution. With the exception of acoustic monitoring for baleen whales in deep offshore waters (Charif and Clark, 2009), no further large-scale data collection efforts have been carried out in this region since the delivery of these surveys.
From these surveys, it appears that the most commonly encountered species in the deep waters along the Scottish shelf edge during the summer include humpback whales (Megaptera novaeangliae), fin whales (Balaenoptera physalus), sei whales (Balaenoptera borealis), northern bottlenose whales (Hyperoodon ampullatus), sperm whales (Physeter macrocephalus), long-finned pilot whales (Globicephala melas), Cuvier’s (Ziphius cavirostris) and Sowerby’s (Mesoplodon bidens) beaked whales, as well as Atlantic white-sided (Lagenorhynchus acutus) and common dolphins. Minke whales, white-beaked dolphins, and harbour porpoises (Phocoena phocoena) on the other hand are more commonly recorded within the 200 m depth contour (Northridge et al., 1995; Harwood and Wilson, 2001; Weir et al., 2001; Reid et al., 2003). Killer whales (Orcinus orca), Risso’s (Grampus griseus) and bottlenose dolphins (Tursiops truncatus) are also frequently encountered in the Atlantic Frontier marine region and use deep offshore areas as well as more shallow shelf waters (Evans, 1987).
Passive acoustic monitoring (PAM) of vocally active marine mammals has become a valuable tool for continuous assessments of species occurrence, distribution, relative abundance, and behaviour (Zimmer, 2011; Marques et al., 2013; Risch et al., 2014a). It allows cost-effective, autonomous, and increasingly real-time data collection over long periods, independent of inclement weather and without disturbance (van Parijs et al., 2009; van Parijs et al., 2021). While aerial and ship-board visual surveys can provide valuable information on species abundance and spatial distribution, most of these surveys only provide summer snapshots on relatively coarse temporal scales (yearly, decadal) and during daylight hours (Gilles et al., 2016; Nachtsheim et al., 2021), with effort typically spanning several days/weeks per survey. In contrast, while spatially limited, static PAM can provide high-resolution temporal data and has been used in multiple studies to investigate long-term (e.g. seasonal) and diurnal trends in species occurrence, and to explore habitat association (e.g. Kyhn et al., 2012; Pirotta et al., 2014; El-Gabbas et al., 2021).
Long-term PAM can also provide information about human activities generating underwater noise such as shipping, military activities, and marine energy industries (Nieukirk et al., 2012; Haver et al., 2018). Information about these human activities and their influences on marine soundscapes and species, and their variability in space and time, can inform effective management of offshore habitats (e.g. Duarte et al., 2021; Warren et al., 2021).
Several studies have employed PAM to study habitat use and the effects of offshore construction on cetaceans in UK waters (e.g. Simon et al., 2010; Risch et al., 2019; Thompson et al., 2020; Benhemma-Le Gall et al., 2021). A recent large-scale PAM survey (ObSERVE) off the Irish continental shelf edge, to the south of the Scottish Atlantic Frontier region, obtained new information about the seasonal distribution of 13 cetacean species (eight odontocetes and five mysticetes), and documented offshore seismic survey activity and its effect on marine mammal acoustic detections (Berrow et al., 2018).
The primary aim of this present study was the collection and analysis of year-round data on cetacean seasonal occurrence patterns and variability in ambient sound levels in the shelf waters west of the Outer Hebrides. Results from this study will feed into statutory reporting on underwater noise and will provide a valuable evidence base for future designation for Marine Protected Areas (MPAs) and Special Areas of Conservation (SACs) for cetaceans, and provide context for status assessment of neighbouring currently existing protected areas. Additionally, the results will contribute to assessments of future human impacts in the region, for example from shipping and offshore renewable energy developments.
2 Methodology
2.1 Passive acoustic data collection
Passive acoustic moorings were deployed at 10 sites on the continental shelf to the west of the Outer Hebrides, Scotland (Table 1; Figure 1). Moorings were deployed with a sub-surface mooring recovery system (VR2AR acoustic release; Innovasea, Canada, with an ARC rope canister; RS Aqua, UK), and did not have a surface buoy to reduce the amount of vertical line in the water column. Mooring depths ranged between 61 and 174 m. The mooring locations were selected based on existing knowledge of cetacean distribution and migration patterns influenced by oceanographic and bathymetric features, and to represent relative distribution and intensity of anthropogenic pressures (especially vessel presence and fishing pressure). The array was designed to provide good spatial coverage from north to south across the continental shelf west of the Outer Hebrides, representing coastal, continental shelf and near-continental shelf slope habitats (Figure 1). Further, the array was placed such as to extend westward from an inshore passive acoustic array deployed from 2017-2022 as part of the COMPASS project1.
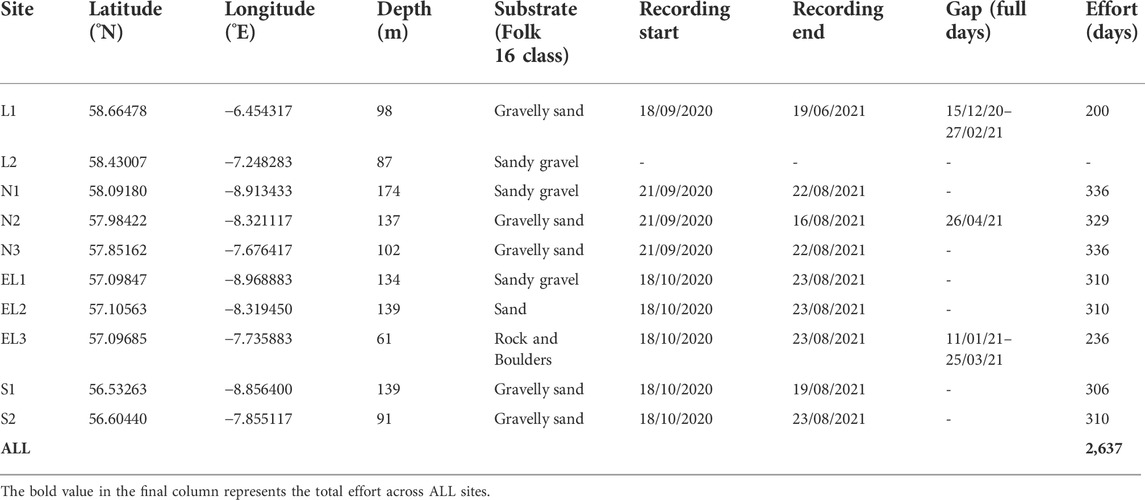
TABLE 1. Deployment and effort information for all acoustic moorings deployed during this study. Substrate data source: Seabed substrate 1:250,000 - Europe © EMODnet Geology, European Commission, 2021.
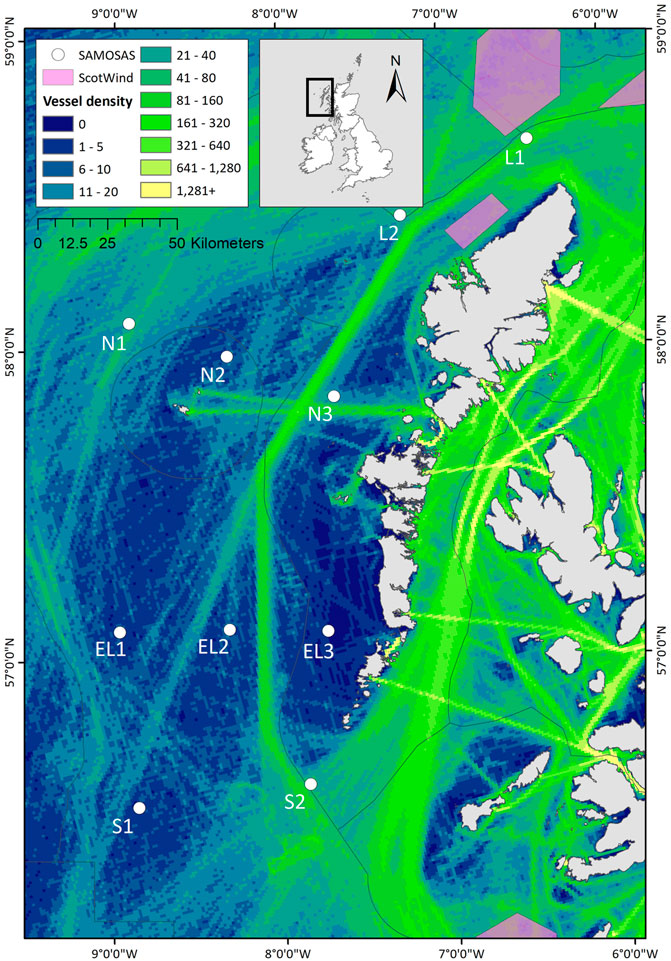
FIGURE 1. Map of the study area off western Scotland, showing the passive acoustic monitoring locations (i.e. the SAMOSAS array) analysed in this project. The black box in the inset map shows the study area in relation to Britain and Ireland. The Outer Hebrides refers to the chain of islands oriented from NNE to SSW west of the Scottish mainland as presented in the main plot; Shetland is the northernmost island grouping visible in the inset. Background colours represent the 2019 Automated Identification System (AIS) ship traffic data. Vessel densities are represented as the annual number of routes per km2. AIS data source: EMODnet Human Activities - European Maritime Safety Agency (EMSA) Route Density Map. The ScotWind 1 leasing areas2 are indicated as pink polygons.
Acoustic data were collected between September 2020 and August 2021 using broadband acoustic recorders (Sylence-LP-440; RTSYS, France) with a pre-amplified hydrophone (GP1516M-LP; Co.l.mar, Italy) positioned approximately 5 m above the seabed and sampling at 64 kS/s (16 bit; +15 dB gain). The system had a mean end-to-end sensitivity of −162.1 dB re 1 V/μPa. Recordings were collected with a 25/35 min on/off cycle every hour2.
2.2 Data analysis
2.2.1 Cetacean occurrence
Acoustic recordings were analysed using a semi-automated detection approach. To aid and speed up the mostly manual analysis process, we used automated detection algorithms customised in XBAT Version R5 (Figueroa and Robbins, 2008) and PAMGuard Version 2.01.05 (Gillespie et al., 2008) for first pass detection of species for which either species-specific (e.g. minke and fin whales) or species group-specific detectors (e.g. delphinids) exist. All detection results were then manually validated by experienced analysts (NvG, CS, SB, BW, DR), and species occurrence summarised as daily (delphinids) or hourly presence (baleen whales). All hours/days without detections were manually reviewed to identify missed presence of the target species. For species for which no automated detectors were readily available (e.g. humpback and sei whales), all sound files were scanned manually by experienced analysts (NvG, DR).
2.2.1.1 Baleen whales
Presence of baleen whales was investigated for the most westerly moorings of the array (N1, EL1, S1; Figure 1) using a combination of automated call detectors and manual validation. To focus the analysis on the lower frequency bands of baleen whale sound production, data for this analysis were downsampled to 2 kHz using the SoX library.
An energy sum detector available in PAMGuard was customised (Supplementary Table S1, Supplementary Material)3 to detect fin whale song (20 Hz notes, often associated with 125–130 Hz precursor notes (Watkins et al., 1987); Figure 2A). Results of the detector were manually verified. All hours without positive detections were manually evaluated for fin whale presence by noting either the presence of 20 Hz song notes missed by the detector or downsweeps in the 40–60 Hz frequency range, which have recently been associated with fin whale foraging behaviour in other parts of the North Atlantic (Romagosa et al., 2021).
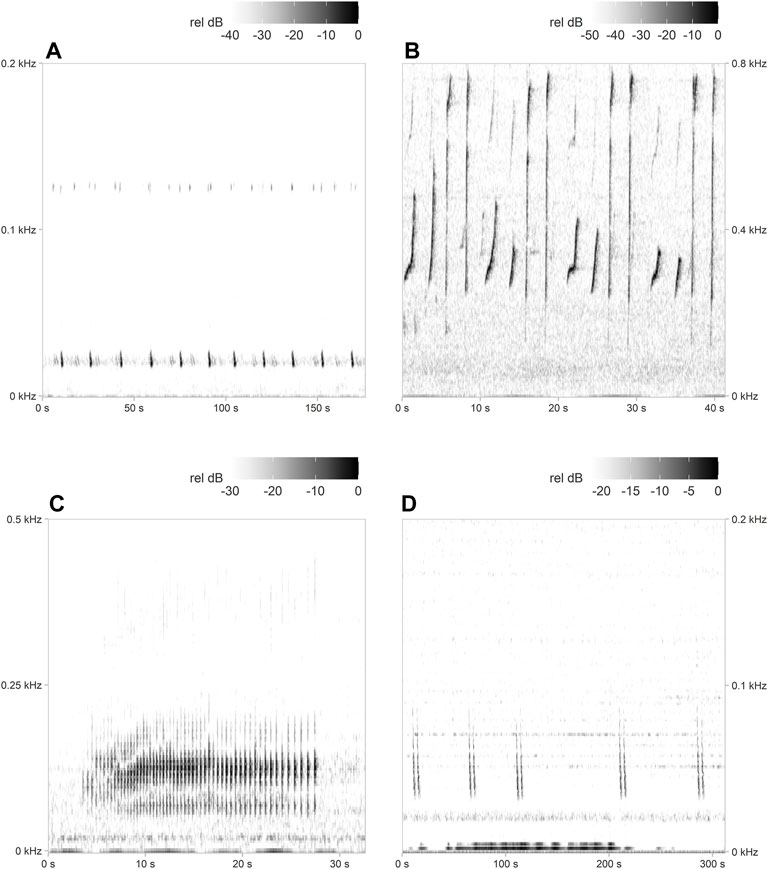
FIGURE 2. Example spectrograms of (A) fin whale song, (B) humpback whale song, (C) minke whale song, and (D) sei whale downsweeps, which were all detected in the current study. Note the different time (x-axis) and frequency scales (y-axis). Spectrogram parameters: fast Fourier transform (FFT) size = 1,024 (B,C) and 2048 (A,D) points, overlap = 95%, sample rate = 2000 Hz, frequency resolution = 1.95 Hz (B,C) and 0.98 Hz (A,D), and time resolution = 25.6 ms (B,C) and 51.2 ms (A,D).
An automated pulse train detector, originally developed for the western North Atlantic (Popescu et al., 2013; Risch et al., 2013), was used to detect minke whale song (Figure 2C). Minke whale detection results were also manually validated, and hours without detections were manually scanned to clarify that no positive detections were missed.
Hourly presence of humpback whale song (Figure 2B), and sei whale downsweeps (Figure 2D), were determined by manually scanning all recording hours for the three targeted recording sites.
2.2.1.2 Delphinids and other odontocetes
Daily presence of odontocete whistles and clicks were determined for the whole array and the entire recording period.
The PAMGuard whistle-and-moan and generic click detectors were customised (Supplementary Table S2, Supplementary Material) to detect odontocetes, including mostly delphinids (Figure 3) but also potentially beaked whales (see Discussion), at all sites. Since reliable species-specific classifiers for Northeast Atlantic dolphin species are not available, neither whistles nor echolocation clicks were classified to species level. All whistle-and-moan and click detector outputs were manually validated, and days without whistle or click detections were manually checked to confirm odontocete absence.
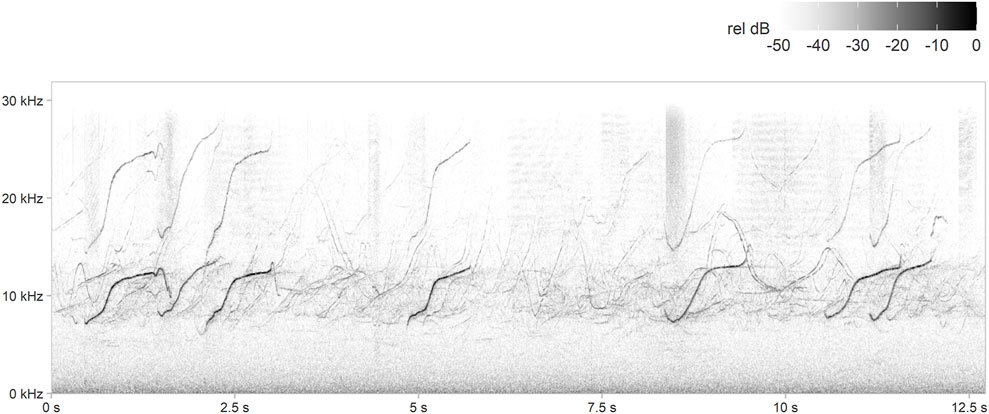
FIGURE 3. Example spectrogram of dolphin whistles and echolocation clicks detected during this study. Spectrogram parameters: fast Fourier transform (FFT) size = 1,024 points, overlap = 95%, sample rate = 64,000 Hz, frequency resolution = 62.5 Hz and time resolution = 0.8 ms.
2.2.2 Ambient sound levels
Using the third-octave level (TOL) function in PAMGuide (Merchant et al., 2015), root-mean-square sound pressure levels (Lp,rms (ISO, 2017); in dB re 1 μPa - hereafter referred to as SPL) were quantified in 1/3-octave bands (Hanning window, 0% overlap, 1 s resolution) across the full available frequency range (i.e. nominal centre frequencies 25 Hz–25 kHz). While susceptible to bias from short, loud transient sounds, this metric has been recommended for quantifying continuous sounds (Robinson et al., 2014). It has been widely used in assessments of underwater soundscapes which are often dominated by continuous sound sources such as wind and vessel traffic (e.g. Haver et al., 2019; Mustonen et al., 2019; Zhang et al., 2020; Basan et al., 2021). For each of the monitoring locations, SPLs were summarized across the entire deployment and per month across the full frequency range. Variability between sites was further investigated by comparing monthly median (i.e. 50th percentile) SPL for each 1/3-octave band across the whole array and plotting the maximum difference between sites.
3 Results
3.1 Monitoring effort
Over the full deployment period (September/October 2020–August 2021), a total of 2,637 days with recordings (25 min/h) were collected across 9 of the 10 monitoring sites (Figure 1; Table 1). Site L2 did not yield any useable data due to a technical failure during deployment. Most sites provided acoustic data for the entire deployment duration and seven sites had over 300 days of data coverage spanning all seasons (Table 1). Data gaps at sites L1, N2 and EL3, lasting from 1 day to over 2 months, occurred due to read/write failures of the recorders (Table 1).
3.2 Baleen whales
Baleen whales, including fin, humpback, minke and sei whales, were regularly recorded on the three western-most moorings of the array (N1, EL1, S1), which were analysed for these species specifically. Fin whales were the most frequently detected baleen whale species, while sei whales were detected on the fewest number of days (Figure 4, Supplementary Figures S1–S2). While efforts were made to search for North Atlantic blue whale song, none was detected in the data analysed from these three moorings.
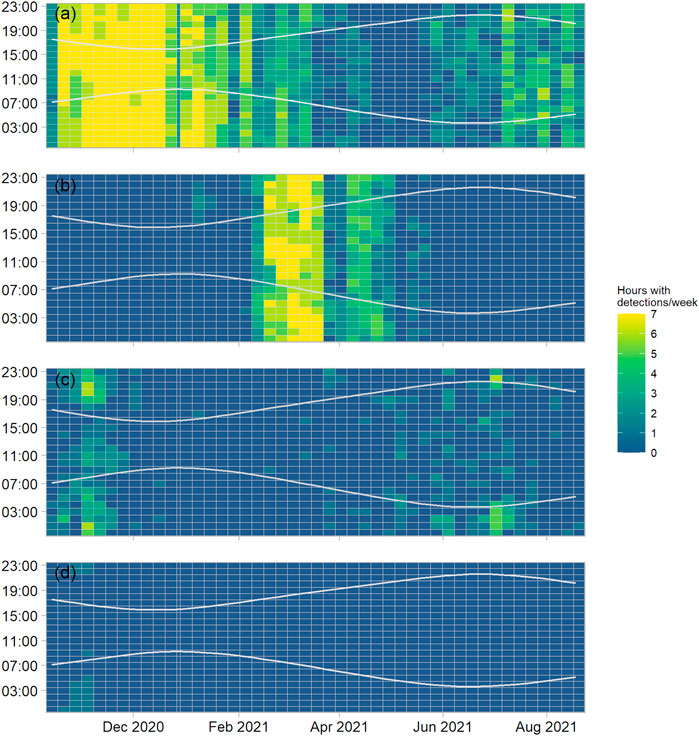
FIGURE 4. Hours with a minimum of one detection (detection positive hours) for site EL1, aggregated by week (x-axis, tick marks indicate start of month) and plotted by hour of day (y-axis), for (A) fin whales (song and downsweeps), (B) humpback whale (song), (C) minke whale (song), (D) sei whale (downsweeps). The “sun-methods” function of the R (R Core Team, 2021) maptools (Bivand and Lewin-Koh, 2013) library was used to determine sunrise and sunset times at the site, as indicated by the grey lines.
Fin whale vocalisations were present almost year-round. Fin whale song or downsweeps were detected on 70.5%, 80.0%, and 70.6% of all monitored days at N1, EL1 and S1, respectively. Most fin whale song detections occurred between November 2020 and January 2021. Fewer songs were recorded from April to June 2021, while the detection of 40 Hz downsweeps increased during that period. Fin whale song detections started to increase again towards the end of the summer (July-August). This general seasonal pattern was similar at all three offshore monitoring sites (Figure 4A, Supplementary Figures S1A–S2A). The 20 Hz song was consistently recorded both in the vicinity of the recorders, as identified by loud (>15 dB signal-to-noise ratio) received levels, and from more distant locations as indicated by lower received levels. Multiple singers were detected simultaneously on a regular basis. No clear diel pattern in vocal activity was detected at any of the monitoring sites.
Humpback whale song was recorded on all three western-most moorings. While first detected in January (EL1, S1) and February (N1), most humpback whale detections occurred during the spring months, from March to May 2021 (Figure 4B; Supplementary Figures S1B–S2B), when humpback whale song became a dominant part of the soundscape below 2 kHz, particularly at night. Humpback whale song detections became very scarce or absent after May. At sites EL1 and S1, humpback whale song decreased from the end of March 2021 for a 2-week period and increased again thereafter. Several singers were recorded simultaneously on numerous occasions, particularly at mooring N1.
Minke whale song was detected year-round with a clear peak during autumn (October-November) at sites EL1 and N1 (Figure 4C, Supplementary Figure S2C). This peak in autumn detections was less clear at site S1 (Supplementary Figure S1C). Detections decreased at all sites during winter (December 2020 to February 2021) and increased again in March/April 2021. Compared to the other species, minke whale song detections showed a stronger diel pattern, particularly during autumn, where more detection positive hours were recorded after sunset (Figure 4C, Supplementary Figures S1C–S2C).
Sei whale downsweeps were detected at sites EL1 and N1, mostly in October and November 2020 (Figure 4D, Supplementary Figure S2D). Most detections (13 detection positive days) were made at site N1 (Supplementary Figure S2D). At site S1 possible sei whale calls were detected on 19 April 2021; however, no detections of sei whales were made at this site during the autumn period when the species was detected at the other two sites (Supplementary Figure S1D).
3.3 Delphinids
Whistle and echolocation click detections were very common across the whole PAM array. Many sites showed near daily presence of whistles and clicks. At sites L1, N1-2, EL1-2 and S1-2, whistles and clicks were detected on more than 90% of all effort days. Lowest detection rates were found at sites N3 and EL3, with 83% and 66% detection positive days, respectively (Figure 5). These two sites were also deployed closest to shore and represented the shallowest sites of the array (Figure 1). However, even at these sites, whistles and clicks were still regularly detected throughout the year. A marked drop in detections was observed at sites L1, N1-3, EL1 and to a lesser extent S1 from about mid-May to mid-June 2021, after which detection rates increased again to nearly 100% detection positive days until the end of the deployment period (Figure 5).
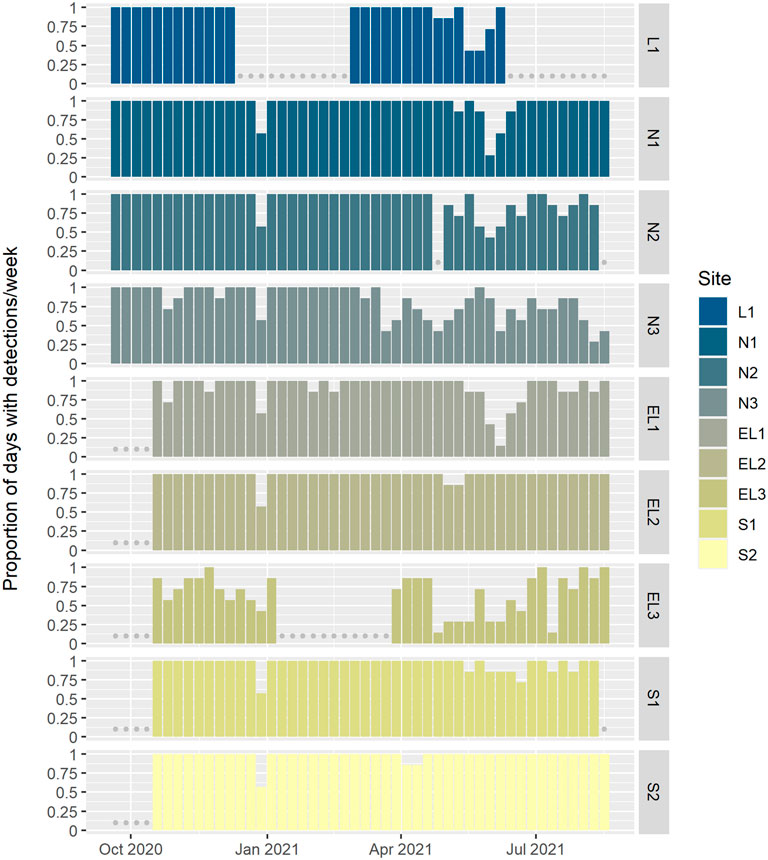
FIGURE 5. Proportion of days per week with whistle and click detections, plotted by site (arranged north to south, and offshore to coastal; see Figure 1). Detections were not classified to species and while mainly comprised of delphinid detections, they might include beaked whales too. Grey dots indicate weeks with missing data.
3.4 Ambient sound
Ambient sound levels (Lp,rms) differed between sites and time of year. Overall, median SPL in the lower frequency (e.g. 1/3-octave bands centred at 25 and 125 Hz) were highest at the most offshore recording sites (N1, EL1, S1), as well as L1 and EL2, and lowest at the inshore sites (N3, EL3, and S2; Figures 6, 7). For the higher frequency bands (e.g. 1/3-octave bands centred at 2 and 10 kHz), SPLs were more uniform across the whole array (Figure 6).
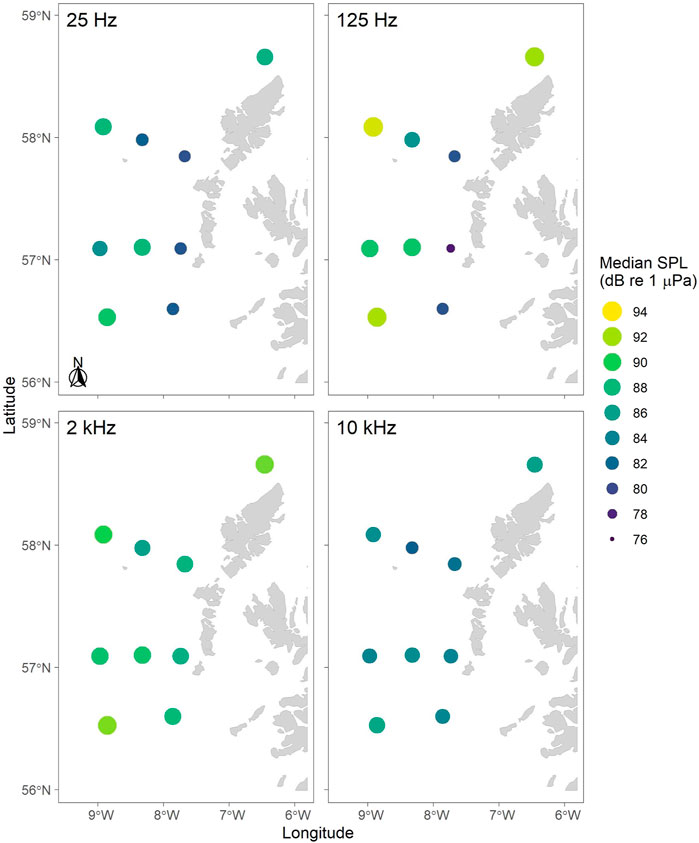
FIGURE 6. Median root-mean-square sound pressure levels (Lp,rms; dB re 1 μPa) mapped across the whole recording period (i.e. 8-12 months; see Table 1) for four 1/3-octave bands centred at 25 Hz, 125 Hz, 2 kHz and 10 kHz mapped by recording location. Colours (dark to light) and circle size (small to big) indicate increase in sound pressure levels.
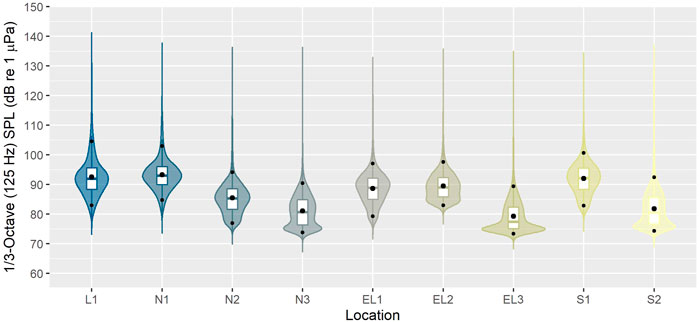
FIGURE 7. Root-mean-square sound pressure level (Lp,rms; dB re 1 μPa) distributions for the 1/3-octave band centred at 125 Hz across the whole recording period (i.e. 8–12 months; see Table 1), plotted by site (see Figure 1). Lower and upper bounds of boxes represent lower and upper quartiles, respectively. Solid middle box lines represent the median and the larger black dot the mean. Small black dots indicate the 5th and 95th percentiles. The probability distribution of the data is shown as kernel density.
When aggregated across the array, the largest variability in the monthly median SPL across the whole deployment period was for the 200 Hz centred 1/3-octave band (i.e. a maximum of 18 dB between sites), with all bands from about 50–400 Hz demonstrating maximal monthly differences of over 10 dB for most months (Figure 8). In general, monthly variability was relatively low for 1/3-octave bands up to approximately 40 Hz, highest for frequency bands centred between 100 Hz and 1.25 kHz and decreased again for the higher 1/3-octave bands where the lowest variability was present both within and between months. Variability in SPLs measured for frequency bands from 200 Hz to 1 kHz tended to increase starting from about January 2021 to the end of the deployment period (Figure 8).
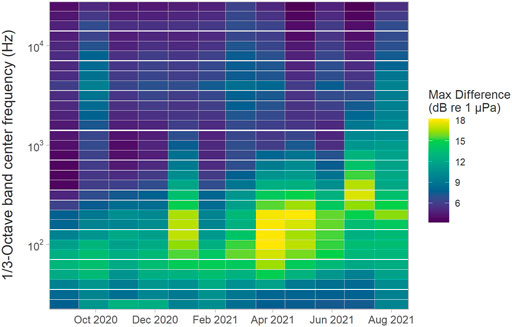
FIGURE 8. Maximum difference in median root-mean-square sound pressure levels (Lp,rms; dB re 1 μPa) comparing all sites of the entire array for each month (x-axis) and 1/3-octave band (y-axis).
4 Discussion
This study presents year-round data on cetacean presence and ambient sound levels in the UK shelf area to the west of the Outer Hebrides, Scotland. Except for some large-scale surveys such as the SCANS and CODA surveys, this area has been poorly surveyed for cetaceans in recent decades and most available datasets date back over 20 years (Charif and Clark, 2000; Charif et al., 2001; Harwood and Wilson, 2001; Weir et al., 2001; Reid et al., 2003; Charif and Clark, 2009).
Four baleen whale species (minke, fin, humpback and sei whales) were detected across the array (Figure 4; Supplementary Figures S1–S2). Except for fin whale 40 Hz downsweeps (Boisseau et al., 2008; Romagosa et al., 2021), the analysis for the detection of these species was directed at the most prominent vocal signal of these species, namely their song (Payne and McVay, 1971; Watkins et al., 1987; Mellinger et al., 2000; Tremblay et al., 2019). In the future, the detection of additional call types, such as humpback whale non-song vocalisations, could broaden the observed seasonal patterns described here. Detection of whistles and echolocation clicks were used to identify presence of delphinids. Acoustic detection probability is, among other aspects, influenced by vocalisation type (e.g. frequency and source level), as well as factors influencing sound propagation and transmission loss characteristics (e.g. temperature and bathymetry) and ambient sound level (i.e. signal-to-noise ratio). Typically, detection ranges of fin whales are likely to be larger than those of minke and humpback whales. Fin whales have been detected out to distances of over 100 km in deep waters off Ireland (McCauley, 2015; Berrow et al., 2018). In the relatively high traffic coastal waters of the Gulf of Maine, United States, detections ranges of 10 and 29 km have been estimated for minke (Risch et al., 2014b), and humpback whales (Clark and Clapham, 2004) respectively. Due to their omni-directionality and lower frequency content, dolphin whistles typically travel larger distances than their clicks (Lammers and Au, 2003), and may be detected up to a maximum of a few tens of kilometres off Scotland and Ireland (Janik, 2000; Berrow et al., 2018).
Fin whale song was detected year-round, but predominantly during the winter months from November 2020 to February 2021 (Figure 4A, Supplementary Figures S1A–S2A with fewest detections during May and June 2021. This general pattern of singing behaviour is similar to that detected in other parts of the North Atlantic (Morano et al., 2012; Romagosa et al., 2020) and singing has been linked exclusively to male reproductive behaviour in this species (Croll et al., 2002).
Humpback whale song was detected extensively during spring months (March-May 2021) but was detected from late January and early February 2021 at some sites (Figure 4B, Supplementary Figures S1B–S2B). The ObSERVE passive acoustic study in Irish waters reported similar seasonality in humpback whale song detection (Berrow et al., 2018), indicating the importance of this marine region for the spring (presumed northward) migration of humpback whales. In an earlier passive acoustic study in offshore waters of the Northeast Atlantic, humpback whale song detections were found both from October to December and from January to March with no detections recorded in this region in April (Charif et al., 2001). Since this earlier study was carried out further offshore than the current one, the combined results from both studies suggest that some humpback whales migrate closer to the British shelf edge during spring and that whales might be distributed further offshore when migrating southward during autumn, which would explain why no humpback whales were detected in the present study during autumn. Interestingly, after a peak in detections during early and mid-March 2021, there was a second peak in humpback whale song detections in April 2021 at all three offshore sites (Figure 4B, Supplementary Figures S1B–S2B), indicating a staggered migration.
Recognised humpback whale breeding areas in the North Atlantic are Silver Bank in the Dominican Republic, the southeastern Caribbean around Guadeloupe and a small remnant of a once larger population around Cape Verde (Stevick et al., 2018; Wenzel et al., 2020). While the western North Atlantic humpback whale population has been increasing post-whaling, the Cape Verde population is still of concern (Wenzel et al., 2020). Using photo-identification, three individual humpback whales from Cape Verde were recently identified in Irish waters, but no matches were found with the Caribbean (Berrow et al., 2021). While the origin of humpback whales using the Scottish shelf region is currently unknown, it is possible that animals from both the Caribbean and the Cape Verde populations might use this region and that individual animals from either population might take different routes or migrate at slightly different times of year, resulting in the seasonal patterns observed here. This potential mix of animals from recovering and non-recovering populations is important to consider when planning new human activity and conservation interventions.
Contrary to humpback whale song occurrence, minke whale song was primarily detected during autumn at EL1 and N1 (October-November 2020). After an absence of detections for most of the winter months, minke whales were again detected during spring and summer 2021 (Figure 3C, Supplementary Figure S2C). This general pattern matches visual observations of minke whale occurrence in Scottish inshore waters from April to November (Macleod et al., 2004; Dolman et al., 2013).
While only detected sporadically, sei whale downsweeps (Tremblay et al., 2019) were recorded over several weeks from October to November at sites EL1 and N1 and possibly 1 day in April at site S1 (Figure 4D, Supplementary Figures S1D–S2D). An autumn peak in detection and generally low detection rates for sei whale vocalisations was also described from the northern acoustic moorings of the ObSERVE array, mainly to the southwest of the Porcupine Shelf (Berrow et al., 2018). Given low sighting rates during visual surveys in offshore Scottish waters, passive acoustic monitoring might be a very useful tool to detect sei whale presence and preferred habitat. Given that sei whale vocalisations and song have been described only relatively recently (Baumgartner et al., 2008; Tremblay et al., 2019), there remains a lot to learn about this species’ vocalisations and their behavioural context. Such knowledge will improve our ability to use passive acoustic recordings to infer the distribution and behaviour of this species in the eastern North Atlantic, which is poorly understood at present.
Delphinid whistles and clicks were detected extensively throughout the year across most of the array (Figure 5). On the most offshore moorings (N1, EL1 and S1), delphinids were detected almost daily, while fewer detections were made at the inshore moorings (EL3, N3). These results show the year-round importance of this marine region for delphinids. To date, most work on classification of delphinid vocalisations in the North Atlantic has focused on populations in the western North Atlantic (Baron et al., 2008; Oswald, 2013; Cohen et al., 2022). Given that a multi-species classification system for the delphinid community inhabiting the eastern North Atlantic is not yet available, no attempt was made to distinguish individual delphinid species in this study. However, future work should include classification by species to allow interpretation of the seasonal distribution of the various species, which, based on other data sources and earlier studies, may include common, bottlenose, white-beaked, Risso’s and Atlantic white-sided dolphins, as well as long-finned pilot whales and killer whales (Northridge et al., 1995; Harwood and Wilson, 2001; Weir et al., 2001; Reid et al., 2003; Ball et al., 2021).
Several beaked whale species might occur in the study area (Kowarski et al., 2018). While Sowerby’s beaked whale clicks are likely too high frequency to be detected using a sample rate of 64 kS/s (Cholewiak et al., 2013), northern bottlenose whales and Cuvier’s beaked whales could be detectable (Stanistreet et al., 2017; Kowarski et al., 2018; Clarke et al., 2019). No attempt was made here to classify detected echolocation clicks to investigate the presence of beaked whales. It is therefore possible that northern bottlenose whales as well as Cuvier’s beaked whales might form part of the detections reported in this study, particularly at the deep-water sites near the shelf edge.
A marked reduction in whistle and echolocation click detections was observed at sites L1, N1-3, EL1 from mid-May to early June 2021 (Figure 5). This period directly follows the spring military exercise Joint Warrior (1–20 May 2021), during which military sonar and firing was recorded on several sites across the array. Given the possible implications of these regular naval activities and otherwise frequent dolphin presence, the potential for a link between the timing of the exercise and the reduction of detections of delphinid vocalisations warrants further investigation.
Passive acoustic data can also provide information on ambient sound levels and wider soundscape composition. Underwater sound is produced by a variety of sources, including natural sound sources (earthquakes, tidal turbulence, wind, and rain) as well as anthropogenic sources such as shipping, recreational vessel traffic, seismic exploration, military sonars, echosounders, offshore construction, and operational noise from marine industries (Hildebrand, 2009; Duarte et al., 2021). These sounds vary in loudness and frequency, as well as over time and space, and many sources overlap with one another, thereby creating complex soundscapes especially in coastal environments close to human population centres (Hatch et al., 2008; Farcas et al., 2020).
The underwater soundscape west of the Outer Hebrides has not been described in detail to date. Given the study area’s remote location and comparatively low levels of human activity, the soundscapes observed here can be expected to differ from those recorded in more heavily used areas nearer the coast. Indeed, median SPLs for the 125 Hz centred 1/3-octave band across the array were in the range of 75–95 dB re 1 µPa. These levels are generally lower than those reported for monitoring sites in the Scottish part of the North Sea (Merchant et al., 2016; van Geel et al., 2020), which experiences a lot more human activity than the current study area. Additionally, while not further investigated in this study, it is of interest to note that the period of data collection fell within the COVID-19 pandemic, which was associated with global reductions in marine vessel traffic and associated decreased noise emissions (e.g. March et al., 2021; Sertlek, 2021). It should also be acknowledged that the SPLs presented here were not corrected for acoustic self-noise (i.e. tide/current related mooring noise particularly affecting the lower frequencies (<400 Hz) at some locations), and sound measurements were affected by the noise floor of the acoustic recorders (affecting the highest frequencies (≥20 kHz)). The combination of these two effects has, for some frequency bands, resulted in higher reported levels than were present in the environment.
Overall, SPLs in the 125 Hz 1/3-octave band were highest at the offshore recording sites and lowest at the inshore sites (Figures 6, 7). While the factors underlying this pattern have not yet been further analysed, weather events and offshore shipping traffic are likely driving noise levels in these frequencies, which has also been observed in other marine regions with similar sound levels in this frequency band, such as the Baltic Sea (Mustonen et al., 2019).
Variability in SPLs between 1/3-octave bands centred at 200 Hz to 1 kHz increased from late winter throughout spring and summer. Some of this increased variability is likely due to increased biological activity, including the extended vocal presence of humpback whales. Other vocal species which were not analysed but were observed during data analysis and whose sounds thus contribute to the overall soundscape include sperm whales, grey seals (Halichoerus grypus), and various fish and invertebrate species, such as snapping shrimps, cod (Gadus morhua), or haddock (Melanogrammus aeglefinus). Another factor which might have affected increased variability in ambient sound levels in spring and summer are contributions from inshore vessel traffic, including fishing and recreational vessels.
The EU Marine Strategy Framework Directive (MSFD; EC, 2008; EC, 2017) requires member states to maintain a Good Environmental Status (GES) of European waters, defined as “ecologically diverse and dynamic oceans and seas which are clean, healthy and productive.” The MSFD has been transposed and implemented into UK legislation via the UK Marine Strategy Regulations. Additionally, all cetacean species are listed on Annex IV of the EU Habitats Directive (EC, 1992). In the UK, the Habitats Directive has originally been transposed into UK law by the Conservation (Natural Habitats, &c.) Regulations 1994, meaning they are given protection in Scotland as European Protected Species. Two species, the harbour porpoise and bottlenose dolphin, are also listed on Annex II, requiring that Favourable Conservation Status of the species be maintained or restored through the designation of Special Areas of Conservation (SACs). In addition, the Marine (Scotland) Act 2010 grants the Scottish Government powers to designate Marine Protected Areas (MPAs). In recent years, these powers, coupled with the requirements under the Habitats Directive have led to the Scottish Government designating a SAC for harbour porpoises (Inner Hebrides and the Minches SAC), and two MPAs; one for Risso’s dolphins (North-east Lewis MPA), and one for minke whales (Sea of the Hebrides MPA) on the west coast of Scotland. All of these neighbour the current study area.
The selection process for these protected areas was based on best available data (e.g. Paxton et al., 2016). However, a lack of information on cetacean distribution and density in offshore areas, particularly during winter, means that current and proposed protected areas are largely located in inshore waters. An offshore deep sea marine reserve, the West of Scotland MPA (the closest boundary of which sits approximately 50 km to the west of N1) was designated by the Scottish Government in 2020. While the habitat and species protection at this site is supposed to support a healthy ecosystem, including the prey consumed by cetacean species that are known to occur in this area, no cetacean species have yet been put forward as protected features of this reserve. The primary reason is a lack of data to determine whether the reserve might provide important habitat for these species. Additional data on cetacean occurrence, like those presented in this study, may provide the evidence required to support future inclusion of cetaceans as protected features and the formulation of effective conservation objectives and management options.
Strategic assessments of offshore habitats are particularly important now given the increased interest in these regions with respect to the generation of renewable energy. Offshore wind energy has reached maturity as an industry and plays an important role in the energy systems of many European countries (DeCastro et al., 2019). The industry is now assessing expansion opportunities into more offshore waters and even the high seas (Elsner and Suarez, 2019). While an important factor in the strategy to reduce future climate change, potential impacts associated with this industry must be carefully assessed in the context of other ongoing cumulative human impacts in offshore habitats, which have also been significantly increasing in recent decades (Halpern et al., 2019).
More generally, these data are also needed to assess and predict ecological changes in the wider eastern North Atlantic caused by the global climate crisis which affects marine mammals as crucial parts of the ecosystem, for which they can act as sentinel species (Moore, 2008; Williamson et al., 2021; Gulland et al., 2022). Large-scale, climate-driven distributional shifts in several cetacean species have been documented in the western North Atlantic, where a shift in distribution of the North Atlantic right whale in response to changes in their primary copepod prey (Davis et al., 2017) had profound conservation implications, such as increased mortality from ship strike and fishing gear entanglement in their new habitat. Increased entanglements of whales in fishing gear following shifts in prey species distribution driven by climate change have also been recently documented in the North Pacific Ocean (Santora et al., 2020).
Similar distributional changes might be ongoing in the eastern North Atlantic but might go unnoticed unless effective year-round and long-term monitoring strategies for marine mammals are implemented in offshore waters of Britain and Ireland and the wider Northeast Atlantic. The results of the current study highlight the value of long-term observational data and emphasize the value of better understanding species’ current distribution and abundance patterns to accurately identify and predict future changes, so that effective long-term conservation measures can be implemented.
Data availability statement
Enquiries about the data should be made to Marine Scotland Science. Requests to access the datasets should be directed to Kate Brookes: S2F0ZS5Ccm9va2VzQHNjb3QuZ292.
Author contributions
RC, NvG, DR, SB, and BW conceptualised and designed the study. Data collection was undertaken by NvG, DR, and TB, and data were analysed by NvG, DR, SB, CS, and BW. DR and NvG wrote the manuscript. All authors reviewed the manuscript and contributed to the writing.
Funding
This study was funded by the European Marine Fisheries Fund (EMFF).
Acknowledgments
We thank John Beaton for his help with the mooring design, and the Seatrek Marine crew for their help deploying and retrieving the equipment.
Conflict of interest
RC is currently employed by APEM Ltd., but was employed by Marine Scotland Science for the duration of the project.
All authors declare that the research was conducted in the absence of any commercial or financial relationships that could be construed as a potential conflict of interest.
Publisher’s note
All claims expressed in this article are solely those of the authors and do not necessarily represent those of their affiliated organizations, or those of the publisher, the editors and the reviewers. Any product that may be evaluated in this article, or claim that may be made by its manufacturer, is not guaranteed or endorsed by the publisher.
Supplementary Material
The Supplementary Material for this article can be found online at: https://www.frontiersin.org/articles/10.3389/frsen.2022.934681/full#supplementary-material
Footnotes
1COMPASS, Collaborative Oceanography and Monitoring for Protected Areas and Species; https://compass-oceanscience.eu/.
2https://www.offshorewindscotland.org.uk/news-events/2022/january/scotwind-1-results/.
References
Ball, R. J., Kitchiner, A., Davison, N. J., Brownlow, A., Berrow, S., McKeown, N. J., et al. (2021). New haplotypes found in stranded long-finned pilot whales (Globicephala melas) in the eastern North Atlantic and adjacent waters. Mar. Mamm. Sci. (In press). doi:10.1111/mms.12893
Baron, S. C., Martinez, A., Garrison, L. P., and Keith, E. O. (2008). Differences in acoustic signals from delphinids in the western North Atlantic and northern Gulf of Mexico. Mar. Mamm. Sci. 24, 42–56. doi:10.1111/j.1748-7692.2007.00168.x
Basan, F., Fischer, J. G., and Kühnel, D. (2021). Soundscapes in the German Baltic Sea before and during the COVID-19 pandemic. Front. Mar. Sci. 8, 689860. doi:10.3389/fmars.2021.689860
Baumgartner, M. F., van Parijs, S. M., Wenzel, F. W., Tremblay, C. J., Carter Esch, H., Warde, A. M., et al. (2008). Low frequency vocalizations attributed to sei whales (Balaenoptera borealis). J. Acoust. Soc. Am. 124, 1339–1349. doi:10.1121/1.2945155
Benhemma-Le Gall, A., Graham, I. M., Merchant, N. D., and Thompson, P. M. (2021). Broad-Scale responses of harbor porpoises to pile-driving and vessel activities during offshore windfarm construction. Front. Mar. Sci. 8, 664724. doi:10.3389/fmars.2021.664724
Berrow, S. D., Massett, N., Whooley, P., Jann, B. V. M., Lopez-Suarez, P., Stevick, P. T., et al. (2021). Resightings of humpback whales (Megaptera novaeangliae) from Ireland to a known breeding ground: Cabo Verde, West Africa. Aquat. Mamm. 47, 63–70. doi:10.1578/AM.47.1.2021.63
Berrow, S. D., O’Brien, J., Meade, R., Delarue, J., Kowarski, K., Martin, B., et al. (2018). Acoustic surveys of cetaceans in the Irish Atlantic Margin in 2015–2016: Occurrence, distribution and abundance. Dublin, Ireland: Department of Communications, Climate Action & Environment and the National Parks and Wildlife Service (NPWS), Department of Culture, Heritage and the Gaeltacht, 348. Available at: https://www.gov.ie/en/publication/12374-observe-programme/#2015-2016-acoustic-survey (Accessed April 23, 2022).
Bivand, R., and Lewin-Koh, N. (2013). maptools: Tools for reading and handling spatial objects. Available at: http://cran.r-project.org/package=maptools.
Boisseau, O., Gillespie, D., Leaper, R., and Moscrop, A. (2008). Blue (Balaenoptera musculus) and fin (B. physalus) whale vocalisations measured from northern latitudes of the Atlantic Ocean. J. Cetacean Res. Manage. 10, 23–30.
Charif, R., Clapham, P., and Clark, C. (2001). Acoustic detections of singing humpback whales in deep waters off the British Isles. Mar. Mamm. Sci. 17, 751–768. doi:10.1111/j.1748-7692.2001.tb01297.x
Charif, R. A., and Clark, C. W. (2000). Acoustic monitoring of large whales off north and west Britain and Ireland: A two-year study, October 1996-September 1998. Joint Nature Conservation Committee (JNCC) Report No. 313.
Charif, R., and Clark, C. W. (2009). Acoustic monitoring of large whales in deep waters north and west of the British Isles: 1996-2005. Preliminary Technical Report 08-07 by Cornell Lab of Ornothology – Bioacoustic Research Program for the UK Department of Energy and Climate Change’s offshore energy Strategic Environmental Assessment programme.
Cholewiak, D., Baumann-Pickering, S., and van Parijs, S. (2013). Description of sounds associated with Sowerby’s beaked whales (Mesoplodon bidens) in the western North Atlantic Ocean. J. Acoust. Soc. Am. 134, 3905–3912. doi:10.1121/1.4823843
Clapham, P. J., Young, S. B., and Brownell, R. L. (1999). Baleen whales: Conservation issues and the status of the most endangered populations. Mamm. Rev. 29, 37–62. doi:10.1046/j.1365-2907.1999.00035.x
Clark, C. W., and Clapham, P. J. (2004). Acoustic monitoring on a humpback whale (Megaptera novaeangliae) feeding ground shows continual singing into late spring. Proc. R. Soc. Lond. B 271, 1051–1057. doi:10.1098/rspb.2004.2699
Clarke, E., Feyrer, L. J., Moors-Murphy, H., and Stanistreet, J. (2019). Click characteristics of northern bottlenose whales (Hyperoodon ampullatus) and Sowerby’s beaked whales (Mesoplodon bidens) off eastern Canada. J. Acoust. Soc. Am. 146, 307–315. doi:10.1121/1.5111336
Cohen, R. E., Frasier, K. E., Baumann-Pickering, S., Wiggins, S. M., Rafter, M. A., Baggett, L. M., et al. (2022). Identification of western North Atlantic odontocete echolocation click types using machine learning and spatiotemporal correlates. PloS ONE 17, e0264988. doi:10.1371/journal.pone.0264988
Croll, D., Clark, C. W., Acevedo, A., Tershy, B., Flores, S., Gedamke, J., et al. (2002). Only male fin whales sing loud songs. Nature 417, 809. doi:10.1038/417809a
Davis, G. E., Baumgartner, M. F., Bonnell, J. M., Bell, J., Berchok, C., Bort Thornton, J., et al. (2017). Long-term passive acoustic recordings track the changing distribution of North Atlantic right whales (Eubalaena glacialis) from 2004 to 2014. Sci. Rep. 7, 13460. doi:10.1038/s41598-017-13359-3
DeCastro, M., Salvador, S., Gómez-Gesteira, M., Costoya, X., Carvalho, D., Sanz-Larruga, F. J., et al. (2019). Europe, China and the United States: Three different approaches to the development of offshore wind energy. Renew. Sustain. Energy Rev. 109, 55–70. doi:10.1016/j.rser.2019.04.025
Dolman, S. J., Hodgins, N. K., Macleod, C. D., Pierce, G. J., and Weir, C. R. (2013). Harbour porpoises (Phocoena phocoena) and minke whales (Balaenoptera acutorostrata) observed during land-based surveys in the Minch, north-west Scotland. J. Mar. Biol. Assoc. U. K. 94, 1185–1194. doi:10.1017/S0025315413000507
Duarte, C. M., Chapuis, L., Collin, S. P., Costa, D. P., Devassy, R. P., Eguiluz, V. M., et al. (2021). The soundscape of the Anthropocene ocean. Science 371, eaba4658. doi:10.1126/science.aba4658
EC (European Commission) (2008). Directive 2008/56/EC of the European Parliament of the Council of 17 June 2008 establishing a framework for community action in the field of marine environmental policy (Marine Strategy Framework Directive) (Text with EEA relevance). Available at: https://eur-lex.europa.eu/legal-content/EN/TXT/?qid=1567028582748&uri=CELEX:32008L0056.
EC (European Commission) (2017). Commission Decision (EU) 2017/848 of 17 May 2017 laying down criteria and methodological standards on good environmental status of marine waters and specifications and standardised methods for monitoring and assessment, and repealing Decision 2010/477/EU. Available at: https://eur-lex.europa.eu/legal-content/EN/TXT/?uri=CELEX%3A32017D0848.
EC (European Council) (1992). Council Directive 92/43/EEC of 21 May 1992 on the conservation of natural habitats and of wild fauna and flora. Available at: https://eur-lex.europa.eu/legal-content/EN/TXT/?uri=CELEX:31992L0043.
El-Gabbas, A., van Opzeeland, I., Burkhardt, E., and Boebel, O. (2021). Dynamic species distribution models in the marine realm: Predicting year-round habitat suitability of baleen whales in the Southern Ocean. Front. Mar. Sci. 8, 802276. doi:10.3389/fmars.2021.802276
Ellett, D. J., Edwards, A., and Bowers, R. (1986). The hydrography of the Rockall Channel – An overview. Proc. Roy. Soc. Edinb. B 88, 61–81. doi:10.1017/S0269727000004474
Elsner, P., and Suarez, S. (2019). Renewable energy from the high seas: Geo-spatial modelling of resource potential and legal implications for developing offshore wind projects beyond the national jurisdiction of coastal States. Energy Policy 128, 919–929. doi:10.1016/j.enpol.2019.01.064
Evans, P., and Waggitt, J. (2020). “Impacts of climate change on Marine Mammals, relevant to the coastal and marine environment around the UK,” in Marine climate change impacts partnership science review 2020, 421–455. Available at: http://www.mccip.org.uk/media/2022/19_marine_mammals_2020.pdf (Accessed April 23, 2022).
Farcas, A., Powell, C. F., Brookes, K. L., and Merchant, N. D. (2020). Validated shipping noise maps of the Northeast Atlantic. Sci. Total Environ. 735, 139509. doi:10.1016/j.scitotenv.2020.139509
Figueroa, H. K., and Robbins, M. (2008). “Xbat: An open-source extensible platform for bioacoustic research and monitoring,” in Computational bioacoustics for assessing biodiversity. Editors K.-H. Frommolt, R. Bardeli, and M. Clausen (Bonn: Bundesamt für Naturschutz), 143–155.
Gilles, A., Viquerat, S., Becker, E. A., Forney, K. A., Geelhoed, S. C. V., Haelters, J., et al. (2016). Seasonal habitat-based density models for a marine top predator, the harbor porpoise, in a dynamic environment. Ecosphere 7, e01367. doi:10.1002/ecs2.1367
Gillespie, D., Mellinger, D. K., Gordon, J., Mclaren, D., Redmond, P., McHugh, R., et al. (2008). PAMGUARD: Semiautomated, open source software for real-time acoustic detection and localisation of cetaceans. J. Acoust. Soc. Am. 30, 54–62. doi:10.1121/1.4808713
Gulland, F. M. D., Baker, J. D., Howe, M., LaBrecque, E., Leach, L., Moore, S. E., et al. (2022). A review of climate change effects on marine mammals in United States waters: Past predictions, observed impacts, current research and conservation imperatives. Clim. Change Ecol. 3, 100054. doi:10.1016/j.ecochg.2022.100054
Halpern, B. S., Frazier, M., Afflerbach, J., Lowndes, J. S., Micheli, F., O’Hara, C., et al. (2019). Recent pace of change in human impact on the world’s ocean. Sci. Rep. 9, 11609. doi:10.1038/s41598-019-47201-9
Hammond, P. S., Lacey, C., Gilles, A., Viquerat, S., Borjesson, P., Herr, H., et al. (2021). Estimates of cetacean abundance in European Atlantic waters in summer 2016 from the SCANS-III aerial and shipboard surveys. Available at: https://synergy.st-andrews.ac.uk/scans3/files/2021/06/SCANS-III_design-based_estimates_final_report_revised_June_2021.pdf (Accessed April 23, 2022).
Hammond, P. S., Macleod, K., Berggren, P., Borchers, D. L., Burt, L., Cañadas, A., et al. (2013). Cetacean abundance and distribution in European Atlantic shelf waters to inform conservation and management. Biol. Conserv. 164, 107–122. doi:10.1016/j.biocon.2013.04.010
Hammond, P. S., Macleod, K., Gillespie, D., Swift, R. J., Winship, A., Burt, M. L., et al. (2009). Cetacean offshore distribution and abundance in European Atlantic waters (CODA) - Final Report. Available at: http://randd.defra.gov.uk/Default.aspx?Module=More&Location=None&ProjectID=13943 (Accessed April 23, 2022).
Harwood, J., and Wilson, B. (2001). The implications of developments on the Atlantic Frontier for marine mammals. Cont. Shelf Res. 21, 1073–1093. doi:10.1016/S0278-4343(00)00125-4
Hatch, L., Clark, C., Merrick, R., van Parijs, S., Ponirakis, D., Schwehr, K., et al. (2008). Characterizing the relative contribution of large vessels to total ocean noise fields: A case study using the Gerry E. Studds Stellwagen Bank National Marine Sanctuary. Environ. Manage. 42, 735–752. doi:10.1007/s00267-008-9169-4
Haver, S. M., Fournet, M. E., Dziak, R. P., Gabriele, C., Gedamke, J., Hatch, L. T., et al. (2019). Comparing the underwater soundscapes of four US national parks and marine sanctuaries. Front. Mar. Sci. 6, 500. doi:10.3389/fmars.2019.00500
Haver, S. M., Gedamke, J., Hatch, L. T., Dziak, R. P., van Parijs, S., McKenna, M. F., et al. (2018). Monitoring long-term soundscape trends in US waters: The NOAA/NPS Ocean Noise Reference Station Network. Mar. Policy 90, 6–13. doi:10.1016/j.marpol.2018.01.023
Hildebrand, J. A. (2009). Anthropogenic and natural sources of ambient noise in the ocean. Mar. Ecol. Prog. Ser. 395, 5–20. doi:10.3354/meps08353
ISO (International Organization for Standardization) (2017). ISO 18405:2017 Underwater Acoustics - Terminology. Available at: https://www.iso.org/obp/ui/#iso:std:iso:18405:ed-1:v1:en.
Janik, V. M. (2000). Source levels and the estimated active space of bottlenose dolphin (Tursiops truncatus) whistles in the Moray Firth, Scotland. J. Comp. Physiol. A 186, 673–680. doi:10.1007/s003590000120
Jones, S., Cottier, F., Inall, M., and Griffiths, C. (2018). Decadal variability on the northwest European continental shelf. Prog. Oceanogr. 161, 131–151. doi:10.1016/j.pocean.2018.01.012
Kowarski, K., Delarue, J., Martin, B., O’Brien, J., Meade, R., Ó. Cadhla, O., et al. (2018). Signals from the deep: Spatial and temporal acoustic occurrence of beaked whales off western Ireland. PLoS ONE 13, e0199431. doi:10.1371/journal.pone.0199431
Kyhn, L. A., Tougaard, J., Thomas, L., Duve, L. R., Stenback, J., Amundin, M., et al. (2012). From echolocation clicks to animal density – Acoustic sampling of harbor porpoises with static dataloggers. J. Acoust. Soc. Am. 131, 550–560. doi:10.1121/1.3662070
Lammers, M. O., and Au, W. W. L. (2003). Directionality in the whistles of Hawaiian spinner dolphins (Stenella longirostris): A signal feature to cue direction of movement? Mar. Mamm. Sci. 19, 249–264. doi:10.1111/j.1748-7692.2003.tb01107.x
MacLeod, C. D., Weir, C. R., Santos, M. B., and Dunn, T. E. (2008). Temperature-based summer habitat partitioning between white-beaked and common dolphins around the United Kingdom and Republic of Ireland. J. Mar. Biol. Assoc. U. K. 88, 1193–1198. doi:10.1017/S002531540800074X
Macleod, K., Fairbairns, R., Gill, A., Fairbairns, B., Gordon, J., Blair-Myers, C., et al. (2004). Seasonal distribution of minke whales Balaenoptera acutorostrata in relation to physiography and prey off the Isle of Mull, Scotland. Mar. Ecol. Prog. Ser. 277, 263–274. doi:10.3354/meps277263
March, D., Metcalfe, K., Tintoré, J., and Godley, B. J. (2021). Tracking the global reduction of marine traffic during the COVID-19 pandemic. Nat. Commun. 12, 2415. doi:10.1038/s41467-021-22423-6
Marques, T. A., Thomas, L., Martin, S. W., Mellinger, D. K., Ward, J. A., Moretti, D. J., et al. (2013). Estimating animal population density using passive acoustics. Biol. Rev. 88, 287–309. doi:10.1111/brv.12001
McCauley, R. (2015). Offshore Irish noise logger program (March to September 2014): Analysis of cetacean presence, and ambient and anthropogenic noise sources. Project CMST 1296 Report R2015-01 for RPS MetOcean/Woodside Energy (Ireland) Pty Ltd. Available at: https://cmst.curtin.edu.au/wp-content/uploads/sites/4/2015/06/CMST-2015-01-1296-Ireland-DRIMS_10574111.pdf (Accessed April 23, 2022).
Mellinger, D. K., Carson, C. D., and Clark, C. W. (2000). Characteristics of minke whale (Balaenoptera acutorostrata) pulse trains recorded near Puerto Rico. Mar. Mamm. Sci. 16, 739–756. doi:10.1111/j.1748-7692.2000.tb00969.x
Merchant, N. D., Brookes, K. L., Faulkner, R. C., Bicknell, A. W. J., Godley, B. J., Witt, M. J., et al. (2016). Underwater noise levels in UK waters. Sci. Rep. 6, 36942. doi:10.1038/srep36942
Merchant, N. D., Fristrup, K. M., Johnson, M. P., Tyack, P. L., Witt, M. J., Blondel, P., et al. (2015). Measuring acoustic habitats. Methods Ecol. Evol. 6, 257–265. doi:10.1111/2041-210X.12330
Miller, B. S., Balcazar, N., Nieukirk, S., Leroy, E. C., Aulich, M., Shabangu, F. W., et al. (2021). An open access dataset for developing automated detectors of Antarctic baleen whale sounds and performance evaluation of two commonly used detectors. Sci. Rep. 11, 806. doi:10.1038/s41598-020-78995-8
Moore, S. E. (2008). Marine mammals as ecosystem sentinels. J. Mammal. 89, 534–540. doi:10.1644/07-MAMM-S-312R1.1
Morano, J. E., Salisbury, D. P., Rice, A. N., Conklin, K. L., Falk, K. L., Clark, C. W., et al. (2012). Seasonal and geographical patterns of fin whale song in the western North Atlantic Ocean. J. Acoust. Soc. Am. 132, 1207–1212. doi:10.1121/1.4730890
Mustonen, M., Klauson, A., Andersson, M., Clorennec, D., Folegot, T., Koza, R., et al. (2019). Spatial and temporal variability of ambient underwater sound in the Baltic Sea. Sci. Rep. 9, 13237. doi:10.1038/s41598-019-48891-x
Nachtsheim, D. A., Viquerat, S., Ramírez-Martínez, N. C., Unger, B., Siebert, U., Gilles, A., et al. (2021). Small cetacean in a human high-use area: Trends in harbor porpoise abundance in the North Sea over two decades. Front. Mar. Sci. 7, 1135. doi:10.3389/fmars.2020.606609
Nieukirk, S. L., Mellinger, D. K., Moore, S. E., Klinck, K., Dziak, R. P., Goslin, J., et al. (2012). Sounds from airguns and fin whales recorded in the mid-Atlantic Ocean, 1999-2009. J. Acoust. Soc. Am. 131, 1102–1112. doi:10.1121/1.3672648
Northridge, S. P., Tasker, M. L., Webb, A., and Williams, J. M. (1995). Distribution and relative abundance of harbour porpoises (Phocoena phocoena L.), white-beaked dolphins (Lagenorhynchus albirostris Gray), and minke whales (Balaenoptera acutorostrata Lacepède) around the British Isles. ICES J. Mar. Sci. 52, 55–66. doi:10.1016/1054-3139(95)80015-8
Orgeret, F., Thiebault, A., Kovacs, K. M., Lydersen, C., Hindell, M. A., Thompson, S. A., et al. (2022). Climate change impacts on seabirds and marine mammals: The importance of study duration, thermal tolerance and generation time. Ecol. Lett. 25, 218–239. doi:10.1111/ele.13920
Oswald, J. (2013). Development of a classifier for the acoustic identification of delphinid species in the Northwest Atlantic Ocean. Encinitas, CA: Bio-Waves, Inc. Final Report. Prepared for Naval Facilities Engineering Command Atlantic, Norfolk, Virginia, under HDR Environmental, Operations and Construction, Inc. Norfolk, Virginia Contract No. CON005-4394-009, Subproject 164744, Task Order 003, Agreement # 105067. Available at: https://www.navymarinespeciesmonitoring.us/files/5013/9422/1082/Atlantic_Classifier_Report_FINAL.pdf (Accessed April 23, 2022).
Paxton, C. G. M., Scott-Hayward, L. A. S., McKenzie, M., Rexstad, E. A., and Thomas, L. (2016). Revised phase III data analysis of Joint Cetacean Protocol data resources. Joint Nature Conservation Committee (JNCC). Report No. 517. Available at: https://hub.jncc.gov.uk/assets/01adfabd-e75f-48ba-9643-2d594983201e (Accessed April 23, 2022).
Payne, R. S., and McVay, S. (1971). Songs of humpback whales. Science 173, 585–597. doi:10.1126/science.173.3997.585
Pinsky, M. L., Worm, B., Fogarty, M. J., Sarmiento, J. L., and Levin, S. A. (2013). Marine taxa track local climate velocities. Science 341, 1239–1242. doi:10.1126/science.1239352
Pirotta, E., Thompson, P. M., Miller, P. I., Brookes, K. L., Cheney, B., Barton, T. R., et al. (2014). Scale-dependent foraging ecology of a marine top predator modelled using passive acoustic data. Funct. Ecol. 28, 206–217. doi:10.1111/1365-2435.12146
Pollock, C. M., Mavor, R., Weir, C. R., Reid, A., White, R. W., Tasker, M. L., et al. (2000). The distribution of seabirds and marine mammals in the Atlantic Frontier, north and west of Scotland. Joint Nature Conservation Committee (JNCC), Aberdeen, Scotland. Available at: https://www.vliz.be/imisdocs/publications/ocrd/125355.pdf.
Popescu, M., Dugan, P. J., Pourhomayoun, M., Risch, D., Lewis, H. W., and Clark, C. W. (2013). “Bioacoustical periodic pulse train signal detection and classification using spectrogram intensity binarization and energy projection,” in ICML 2013 workshop on machine learning for bioacoustics (Atlanta, GA).
R Core Team (2021). R: A language and environment for statistical computing. Available at: https://www.r-project.org/.
Record, N. R., Runge, J. A., Pendleton, D. E., Balch, W. M., Davies, K. T. A., Pershing, A. J., et al. (2019). Rapid climate-driven circulation changes threaten conservation of endangered North Atlantic right whales. Oceanogr. 32, 162–169. doi:10.5670/oceanog.2019.201
Reid, J. B., Evans, P. G. H., and Northridge, S. (2003). Atlas of cetacean distribution in north-west European waters. Peterborough: Joint Nature Conservation Committee (JNCC). Available at: https://data.jncc.gov.uk/data/a5a51895-50a1-4cd8-8f9d-8e2512345adf/atlas-cetacean-distribution-web.pdf (Accessed April 23, 2022).
Risch, D., Castellote, M., Clark, C. W., Davis, G. E., Dugan, P. J., Hodge, L. E. W., et al. (2014a). Seasonal migrations of North Atlantic minke whales: Novel insights from large-scale passive acoustic monitoring networks. Mov. Ecol. 2, 24. doi:10.1186/s40462-014-0024-3
Risch, D., Clark, C. W., Dugan, P. J., Popescu, M., Siebert, U., van Parijs, S. M., et al. (2013). Minke whale acoustic behavior and multi-year seasonal and diel vocalization patterns in Massachusetts Bay, USA. Mar. Ecol. Prog. Ser. 489, 279–295. doi:10.3354/meps10426
Risch, D., Siebert, U., and van Parijs, S. M. (2014b). Individual calling behaviour and movements of North Atlantic minke whales (Balaenoptera acutorostrata). Behav. 151, 1335–1360. doi:10.1163/1568539X-00003187
Risch, D., Wilson, S. C., Hoogerwerf, M., van Geel, N. C. F., Edwards, E. W. J., Brookes, K. L., et al. (2019). Seasonal and diel acoustic presence of North Atlantic minke whales in the North Sea. Sci. Rep. 9, 3571. doi:10.1038/s41598-019-39752-8
Robinson, S. P., Lepper, P. A., and Hazelwood, R. A. (2014). Good practice guide for underwater noise measurement. NPL good practice guide No. 133. National Measurement Office, Marine Scotland, The Crown Estate. Available at: https://www.npl.co.uk/special-pages/guides/gpg133underwater (Accessed June 12, 2022).
Romagosa, M., Baumgartner, M., Cascão, I., Lammers, M. O., Marques, T. A., Santos, R. S., et al. (2020). Baleen whale acoustic presence and behaviour at a Mid-Atlantic migratory habitat, the Azores Archipelago. Sci. Rep. 10, 4766. doi:10.1038/s41598-020-61849-8
Romagosa, M., Pérez-Jorge, S., Cascão, I., Mouriño, H., Lehodey, P., Pereira, A., et al. (2021). Food talk: 40-Hz fin whale calls are associated with prey biomass. Proc. R. Soc. B 288, 20211156. doi:10.1098/rspb.2021.1156
Santora, J. A., Mantua, N. J., Schroeder, I. D., Field, J. C., Hazen, E. L., Bograd, S. J., et al. (2020). Habitat compression and ecosystem shifts as potential links between marine heatwave and record whale entanglements. Nat. Commun. 11, 536. doi:10.1038/s41467-019-14215-w
Sertlek, H. Ö. (2021). Hindcasting soundscapes before and during the COVID-19 pandemic in selected areas of the North Sea and the Adriatic Sea. J. Mar. Sci. Eng. 9, 702. doi:10.3390/jmse9070702
Simon, M., Nuuttila, H., Reyes-Zamudio, M. M., Ugarte, F., Verfuss, U., Evans, P. G. H., et al. (2010). Passive acoustic monitoring of bottlenose dolphin and harbour porpoise, in Cardigan Bay, Wales, with implications for habitat use and partitioning. J. Mar. Biol. Assoc. U. K. 90, 1539–1545. doi:10.1017/S0025315409991226
Stanistreet, J. E., Nowacek, D. P., Baumann-Pickering, S., Bell, J. T., Cholewiak, D. M., Hildebrand, J. A., et al. (2017). Using passive acoustic monitoring to document the distribution of beaked whale species in the western North Atlantic Ocean. Can. J. Fish. Aquat. Sci. 74, 2098–2109. doi:10.1139/cjfas-2016-0503
Stevick, P. T., Bouveret, L., Gandilhon, N., Rinaldi, C., Rinaldi, R., Broms, F., et al. (2018). Migratory destinations and timing of humpback whales in the southeastern Caribbean differ from those off the Dominican Republic. J. Cetacean Res. Manage. 18, 127–133.
Thompson, D’A. W. (1928). On whales landed at the Scottish whaling stations during the years 1908-1914 and 1920-1927. Fish. Board Scotl. Sci. Investigations 3, 1–40.
Thompson, P. M., Graham, I. M., Cheney, B., Barton, T. R., Farcas, A., Merchant, N. D., et al. (2020). Balancing risks of injury and disturbance to marine mammals when pile driving at offshore windfarms. Ecol. Solutions Evid. 1, e12034. doi:10.1002/2688-8319.12034
Tremblay, C. J., van Parijs, S. M., and Cholewiak, D. (2019). 50 to 30-Hz triplet and singlet down sweep vocalizations produced by sei whales (Balaenoptera borealis) in the western North Atlantic Ocean. J. Acoust. Soc. Am. 145, 3351–3358. doi:10.1121/1.5110713
van Geel, N. C. F., Merchant, N. D., Culloch, R. M., Edwards, E. W. J., Davies, I. M., O’Hara Murray, R. B., et al. (2020). Exclusion of tidal influence on ambient sound measurements. J. Acoust. Soc. Am. 148, 701–712. doi:10.1121/10.0001704
van Parijs, S. M., Baker, K., Carduner, J., Daly, J., Davis, G. E., Esch, C., et al. (2021). NOAA and BOEM minimum recommendations for use of passive acoustic listening systems in offshore wind energy development monitoring and mitigation programs. Front. Mar. Sci. 8, 760840. doi:10.3389/fmars.2021.760840
van Parijs, S. M., Clark, C. W., Sousa-Lima, R. S., Parks, S. E., Rankin, S., Risch, D., et al. (2009). Management and research applications of real-time and archival passive acoustic sensors over varying temporal and spatial scales. Mar. Ecol. Prog. Ser. 395, 21–36. doi:10.3354/meps08123
Vollset, K. W., Urdal, K., Utne, K., Thorstad, E. B., Sægrov, H., Raunsgard, A., et al. (2022). Ecological regime shift in the Northeast Atlantic Ocean revealed from the unprecedented reduction in marine growth of Atlantic salmon. Sci. Adv. 8, eabk2542. doi:10.1126/sciadv.abk2542
Warren, V. E., McPherson, C., Giorli, G., Goetz, K. T., and Radford, C. A. (2021). Marine soundscape variation reveals insights into baleen whales and their environment: A case study in central New Zealand. R. Soc. Open Sci. 8, 201503. doi:10.1098/rsos.201503
Watkins, W. A., Tyack, P. L., Moore, K. E., and Bird, J. E. (1987). The 20-Hz signals of finback whales (Balaenoptera physalus). J. Acoust. Soc. Am. 82, 1901–1912. doi:10.1121/1.395685
Weir, C. R., Pollock, C., Cronin, C., and Taylor, S. (2001). Cetaceans of the Atlantic Frontier, north and west of Scotland. Cont. Shelf Res. 21, 1047–1071. doi:10.1016/S0278-4343(00)00124-2
Wenzel, F. W., Broms, F., López-Suárez, P., Lopes, K., Veiga, N., Yeoman, K., et al. (2020). Humpback whales (Megaptera novaeangliae) in the Cape Verde Islands: Migratory patterns, resightings, and abundance. Aquat. Mamm. 46, 21–31. doi:10.1578/AM.46.1.2020.21
Williamson, M. J., ten Doeschate, M. T. I., Deaville, R., Brownlow, A. C., and Taylor, N. L. (2021). Cetaceans as sentinels for informing climate change policy in UK waters. Mar. Policy 131, 104634. doi:10.1016/j.marpol.2021.104634
Zhang, G., Forland, T. N., Johnsen, E., Pedersen, G., and Dong, H. (2020). Measurements of underwater noise radiated by commercial ships at a cabled ocean observatory. Mar. Pollut. Bull. 153, 110948. doi:10.1016/j.marpolbul.2020.110948
Keywords: passive acoustic monitoring, soundscape, cetaceans, seasonal occurrence, ambient sound
Citation: van Geel NCF, Risch D, Benjamins S, Brook T, Culloch RM, Edwards EWJ, Stevens C and Wilson B (2022) Monitoring cetacean occurrence and variability in ambient sound in Scottish offshore waters. Front. Remote Sens. 3:934681. doi: 10.3389/frsen.2022.934681
Received: 02 May 2022; Accepted: 04 July 2022;
Published: 31 August 2022.
Edited by:
Marc Lammers, Hawaiian Islands Humpback Whale National Marine Sanctuary, United StatesReviewed by:
Zhao Zhao, Nanjing University of Science and Technology, ChinaElias Fakiris, University of Patras, Greece
Giacomo Giorli, National Institute of Water and Atmospheric Research (NIWA), New Zealand
Copyright © 2022 van Geel, Risch, Benjamins, Brook, Culloch, Edwards, Stevens and Wilson. This is an open-access article distributed under the terms of the Creative Commons Attribution License (CC BY). The use, distribution or reproduction in other forums is permitted, provided the original author(s) and the copyright owner(s) are credited and that the original publication in this journal is cited, in accordance with accepted academic practice. No use, distribution or reproduction is permitted which does not comply with these terms.
*Correspondence: Nienke C. F. van Geel, TmllbmtlLnZhbkdlZWxAc2Ftcy5hYy51aw==