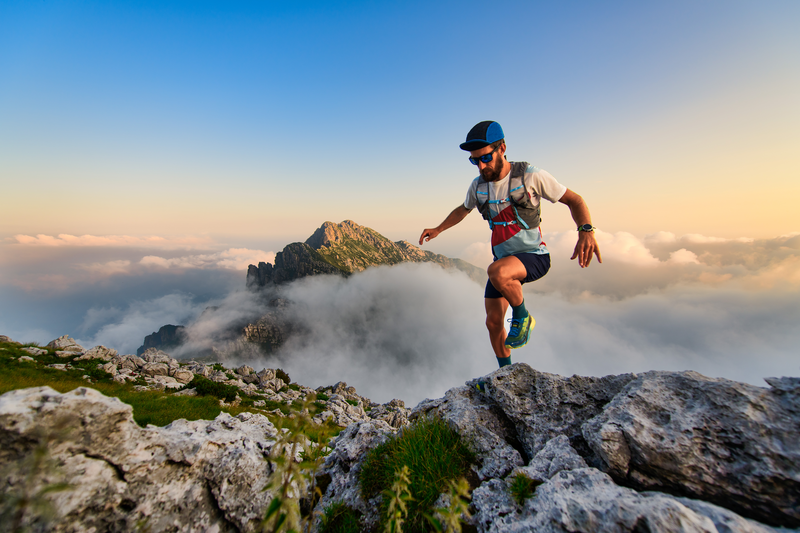
94% of researchers rate our articles as excellent or good
Learn more about the work of our research integrity team to safeguard the quality of each article we publish.
Find out more
CASE REPORT article
Front. Rehabil. Sci.
Sec. Rehabilitation in Children and Youth
Volume 6 - 2025 | doi: 10.3389/fresc.2025.1545105
This article is part of the Research Topic Exoskeleton Gait Training View all 10 articles
The final, formatted version of the article will be published soon.
You have multiple emails registered with Frontiers:
Please enter your email address:
If you already have an account, please login
You don't have a Frontiers account ? You can register here
Recently, robot-assisted gait training (RAGT) has attracted attention as a rehabilitation method to efficiently improve walking function. The purpose of this case report is to examine whether there is a change in gait function after RAGT with HAL in children with cerebral palsy (CP). Methods: Three children with CP participated in this study. Case 1 was an 8-year-old boy with Gross Motor Function Classification System (GMFCS) level II. Case 2 involved a 9-yearold girl with a class IV GMFCS. Case 3 was that of a 10-year-old boy with class IV GMFCS. RAGT was conducted once a day for 20 min for a total of 11-12 sessions. Gait was assessed before and after RAGT. We assessed using three-dimensional motion analysis and surface electromyography (sEMG). The sEMG signals were recorded from the bilateral tensor fascia lata, gluteus maximus, semitendinosus, and rectus femoris. Results: All cases showed changes in the joint angle and muscle activity in the lower limbs before and after RAGT. In Case 1, the maximum hip extension angle increased from -10.6° to -4.1° at the terminal stance, and the average muscle activity of the gluteus maximus in the right stance phase increased from 22.4% to 30.2%. In Case 2, the maximum extension angle of the left knee joint increased from -43.0° to -26.9°. In Case 3, the maximum hip extension angle increased from -39.9° to -25.9° on the left side and from -35.1° to -18.7° on the right side; the maximum knee extension angle increased from -55.7° to -38.8° on the left side and from -52.1° to -36.9° on the right side. Discussion: A Case 1 had significant hip flexion during gait, but RAGT with HAL emphasized hip extension motion and enabled an efficient gait. As a result, the maximum hip extension angle increased, and the activity of the gluteus maximus muscle in the stance phase increased. Cases 2 and 3 had greater hip and knee joint flexion angles, however increased knee extension angles after RAGT. The increased hip and knee joint extension angles during the stance phase increased the propulsive force propelling the walker forward.
Keywords: Robot-assisted gait training, Hybrid Assistive Limb, Cerebral Palsy, Three-dimensional gait analysis, Electromyography
Received: 14 Dec 2024; Accepted: 10 Mar 2025.
Copyright: © 2025 Takahashi, Mutsuzaki, NAKAYAMA, Kuroda, Koseki, Yoshikawa, Nakayama, Oguro, Takeuchi, Mizukami, Watanabe and Marushima. This is an open-access article distributed under the terms of the Creative Commons Attribution License (CC BY). The use, distribution or reproduction in other forums is permitted, provided the original author(s) or licensor are credited and that the original publication in this journal is cited, in accordance with accepted academic practice. No use, distribution or reproduction is permitted which does not comply with these terms.
* Correspondence:
Hirotaka Mutsuzaki, Ibaraki Prefectural University of Health Sciences, Ami, Ibaraki, Japan
Disclaimer: All claims expressed in this article are solely those of the authors and do not necessarily represent those of their affiliated organizations, or those of the publisher, the editors and the reviewers. Any product that may be evaluated in this article or claim that may be made by its manufacturer is not guaranteed or endorsed by the publisher.
Research integrity at Frontiers
Learn more about the work of our research integrity team to safeguard the quality of each article we publish.